- 1Marine Biomedical Research Institute, Guangdong Medical University, Zhanjiang, China
- 2Department of Breast Surgery, Affiliated Hospital of Guangdong Medical University, Zhanjiang, China
- 3Department of Breast Surgical Oncology, National Cancer Center/National Clinical Research Center for Cancer, Chinese Academy of Medical Sciences and Peking Union Medical College, Shenzhen, China
Invasive micropapillary carcinoma of the breast (IMPC) exhibits a unique micropapillary structure and “inside-out” growth pattern. Despite its extremely low incidence, IMPC has attracted considerable attention owing to its poor prognosis. Since Siriaunkgul and Tavassoli first proposed the term IMPC in 1993 to describe its morphological characteristics, with tumor cell clusters arranged in a pseudopapillary structure within the glandular cavity, its diagnostic rate has substantially increased. Based on the in-depth study of IMPC, a more comprehensive understanding of its epidemiology, clinicopathological features, and diagnostic criteria has been achieved in recent years. The pathogenesis and specific therapeutic targets of IMPC remain unclear. However, numerous studies have delved into its high-risk biological behavior. This review discusses the opportunities and challenges associated with IMPC.
Background
Invasive micropapillary carcinoma (IMPC) is a rare type of breast cancer with distinct histological and biological features. Morphologically, IMPC is composed of nested, morula-like, or pseudopapillary structures of neoplastic clusters devoid of fibrovascular cores surrounded by clear stromal spaces (1). In addition, micropapillary pattern (MP), associated with aggressive biological behavior and poor prognosis (2–4), has been reported in several organs, such as the lung (5), bladder (6), pancreas (7), alimentary tract (8, 9), salivary gland (10), thyroid (11), ovary (12), cervix (13), and kidney (14).
In 1980, Fisher et al. first described “the exfoliative appearance structure” in breast tissue (15). Later, in 1993, Siriaunkgul and Tavassoli first proposed the term “invasive micropapillary carcinoma of the breast” and provided a detailed description of its histological features (16). Until 2003, the World Health Organization (WHO) classified IMPC as a special histological subtype of breast cancer, a classification still in use today (17–19). IMPC accounts for 2%–8% of all breast cancers (4, 19–21). However, its occurrence is usually associated with lymph node metastasis (LNM), lymphovascular invasion (LVI), and locoregional recurrence (LRR) (22, 23). Through the in-depth study of IMPC, a more comprehensive understanding of its epidemiology, clinicopathological features, and diagnostic criteria has been achieved in recent years (Figure 1).
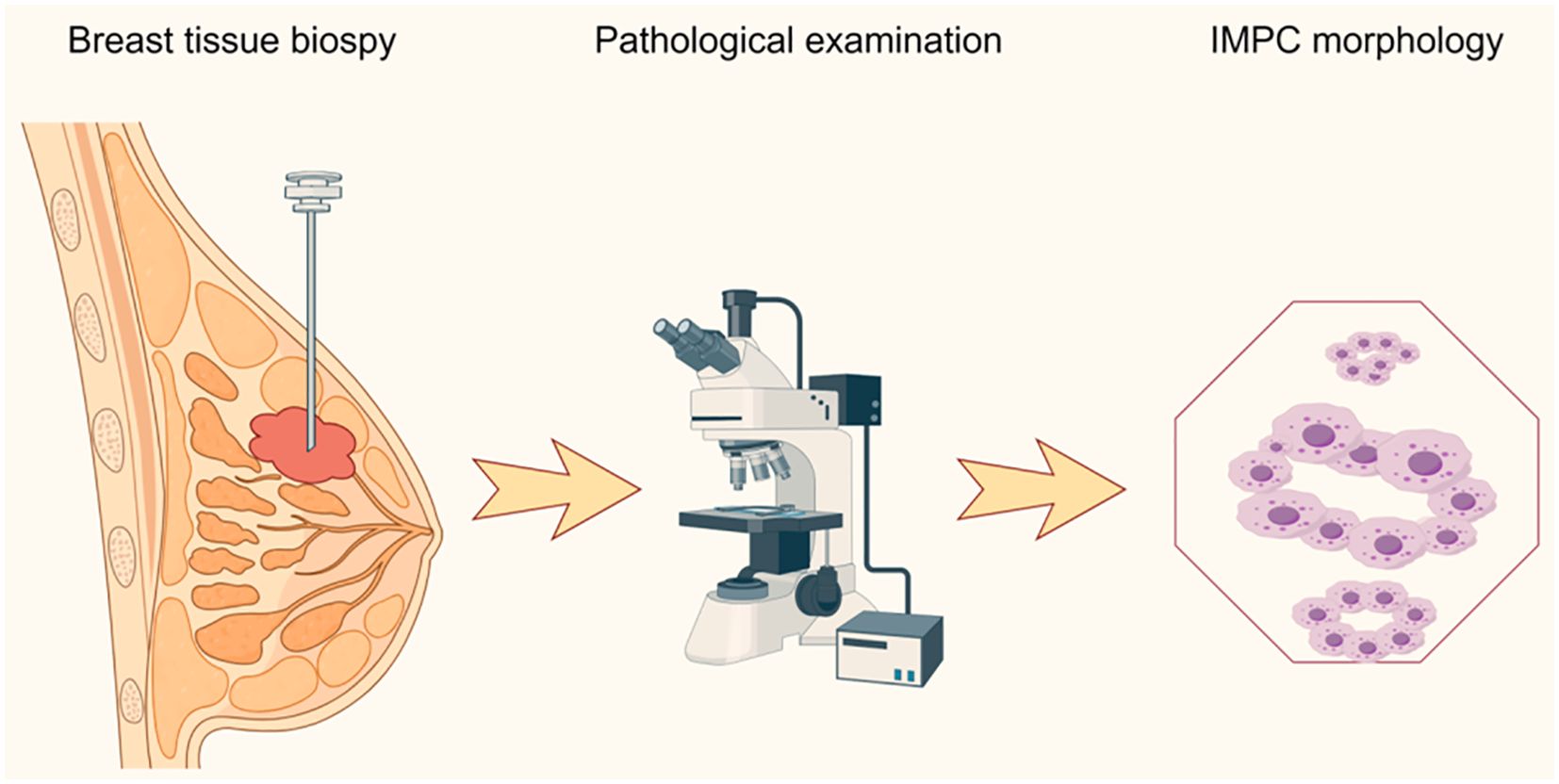
Figure 1. Pathological diagnostic procedure of invasive micropapillary carcinoma. IMPC, invasive micropapillary carcinoma of the breast.
Nevertheless, the high-risk biological behavior of IMPC, including its biological hallmarks, pathogenesis, and specific therapeutic targets, remains unclear. Therefore, individualized treatment for this subtype of breast cancer has not been improved. This review focuses on the research progress of IMPC and discusses its opportunities and challenges.
Pathological features of IMPC
Histological features
Cancerous cells typically have serrated edges and granular or eosinophilic cytoplasm when viewed under an optical microscope. Within nests, these cells form mulberry-like clusters and pseudopapillary structures, which are notable for the absence of endothelial cells and fibrovascular cores. Cancerous cells are typically enveloped by transparent fibrocollagen stromal space, resembling a swimming ring floating in the sea (17). Under an electron microscope, microvilli can be observed on the outer surface of cancerous cells, exhibiting secretory activity within the surrounding stroma (24). Furthermore, under a multiphoton microscope, six different morphologies of IMPC clusters can be detected: mulberry-like, chrysanthemum-like, tubular glandular, circular cluster, elliptical cluster, and irregular masses (25).
Invasive mucinous carcinoma or neuroendocrine carcinoma may also be accompanied by micropapillary (MP) features that are difficult to distinguish from IMPC. However, compared with pure invasive mucinous carcinoma or neuroendocrine carcinoma, the presence of MP features, such as LNM, LVI, and deteriorated differentiation, increases the probability of poor prognosis (22, 26–29). Pareja et al. reported that there was no significant genomic difference between IMPC and mucinous carcinoma with MP features. Both carcinomas exhibited recurrent gains in 1q, 6p, 8q, and 10q, along with recurrent losses in 16q, 11q, and 13q. Additionally, recurrent 8p12–8p11.2 amplification was observed in FGFR1 (30).
Previously, the diagnostic criteria for the proportion of IMPC have not been clearly defined because of its rarity as a pure form, often accompanied by invasive ductal carcinoma (IDC) component (31). Thus, three different classification methods of pure IMPC have been proposed: MP proportion > 50%, MP proportion > 75%, and MP diameter > 5 mm. Subsequently, studies have shown that the poor prognosis of IMPC is only related to the presence of MP features rather than their proportion (32–37). Based on these findings, Fu et al. proposed that IMPC can be diagnosed as long as MP features are present in IDC and their proportion should be noted in the pathological report (38). However, pure IMPC is a rare phenomenon, found in approximately 1%–2% of breast carcinomas. The authors suggest a cutoff percentile value of 75% IMPC component to histologically identify a carcinoma as pure IMPC (39).
When distributed focally, IMPC mostly exhibits the “inside-out” growth pattern and reversal of neoplastic cell polarity, distinguishing it from other breast cancer subtypes (40). Cytologically, the inside-out growth pattern induces a reversal of cell polarity, causing the apical surface of cells to face the stroma instead of the lumen. This alteration confers highly invasive and metastatic potential (41). Furthermore, the “inside-out” growth pattern can be confirmed through staining for MUC1/epithelial membrane antigen (EMA), sialyl Lewis X, p120 catenin, or Annexin A2 on the cell membrane (42–44). Nonetheless, their staining emerges almost exclusively on the peripheral membrane, with no apical staining, also known as “goblet staining” (45).
Immunohistochemical features
Among the molecular subtypes of IMPC, luminal subtypes account for about 70%−80%, while HER2-overexpressing and triple-negative subtypes account for only 10%−20% (46, 47). However, the criteria for evaluating the HER2 status of IMPC are controversial. According to the 2018 American Society of Clinical Oncology and College of American Pathology (ASCO/CAP) HER2 testing recommendations, moderate to intense incomplete membrane staining of HER2 can be scored as 2+ but not as 3+ (48).
Because of the inside-out growth pattern of neoplastic cell clusters, IMPC often exhibits incomplete membrane staining intensity of HER2. Stewart et al. and Perron et al. recommended the incorporation of fluorescence in situ hybridization testing to confirm HER2 amplification (49). However, Zhou et al. demonstrated that if the basolateral membrane showed intense, clear, linear, and incomplete staining of HER2, IMPC should be classified as HER2 3+ rather than HER2 2+. This approach helps avoid the need for additional fluorescence in situ hybridization testing procedures, saving considerable time, manpower, and economic costs.
Previously, HER2 expression was considered clinically insignificant in ductal carcinoma in situ; however, Francesk et al. reported that HER2 over-expression in DCIS is correlated with poorer clinicopathological parameters (50). Moreover, a clinical study showed that trastuzumab treatment in high-risk patients with HER2-positive DCIS reduced the recurrence rate (51). There are no studies on whether HER2 expression affects the prognosis of patients with IMPC; thus, this data need to be further explored.
Clinical examination of IMPC
IMPC exhibits distinct characteristics in different radiological examinations (52–55). On mammography, most lesions are irregular or lobulated, with vague or spiculated margins and scattered calcifications. On ultrasonography, lesions mostly demonstrate longitudinal growth, internal hypoechoic appearance, irregular shape, lobulated margins, and echogenic posterior features. Lesions observed on magnetic resonance imaging (MRI) typically have irregular shapes with spiculated margins. They exhibit heterogeneity in contrast enhancement and demonstrate a type III time-signal intensity curve. On positron emission tomography-computed tomography (PET-CT), the mean maximum standardized uptake value (SUVmax) of the lesions is >10, which distinguishes it from other breast cancer subtypes (Table 1).
Kubota et al. demonstrated that IMPC is particularly specific in MRI examination and is easy to identify and that ultrasound examination has high sensitivity for LNM of IMPC (56). However, the mammographic and ultrasound imaging characteristics of IMPC are reported to be difficult to distinguish from those of other breast cancer subtypes (57). Moreover, among the 29 cases enrolled in Akgun et al.’s study (58), 13 (13/29,44.82%) had MP component <75% and 16 (16/29,55.18%) had MP component >75%. The data revealed no significant correlation between the SUVmax of PET-CT and the proportion of MP component.
Although IMPC is highly invasive, sentinel lymph node biopsy (SLNB) remains the gold standard for determining the need for axillary lymph node dissection. To date, indocyanine green (ICG) is considered to have a good application prospect in SLNB for IMPC (59).
Clinical features of IMPC
The median age at IMPC onset is 48.0–61.4 years, with a median diameter of approximately 2.0–5.0 cm (60–62), consistent with the previous findings of Fu et al. (38). However, another study suggests that IMPC tends to be larger than IDC (63).
Liu et al. reported that IMPC had a significantly higher rate of estrogen receptor (ER) positivity (P = 0.037) and LVI (P = 0.005) than matched IDC (64). Mercogliano et al. further explained that ER expression in IMPC was positively correlated with tumor size but inversely correlated with patient age (65). Yu et al. found that IMPC had a higher nuclear grade III ratio than IDC by comparing the clinicopathological features between 267 IDC and 267 IMPC cases (P < 0.001) (66). Aker et al. reported that IMPC is more likely to have LNM than IDC (67). The presence of MP features increases the probability of LNM, LVI, and poor nuclear differentiation. This is closely related to the disordered arrangement and polarity reversal of neoplastic cell clusters (Table 2).
Eren et al. found that the positive rate of progesterone receptor (PR) in the pure IMPC group was significantly lower than that in the mixed IMPC group (66.7% vs. 83.3%, P = 0.024) (31). Further analysis by Wang et al. revealed a significantly higher proportion of stage IIIc cases in the pure IMPC group than in the mixed IMPC group (38.3% vs. 17.8%, P = 0.003) (68). However, Kaya et al. reported no significant difference between pure and mixed IMPC in terms of PR status, tumor size, LNM, LVI, and histological grade (69). Combined with the relevant literature, the incidence of pure IMPC was only 1%–2% (62, 70). The variation in the results among the aforementioned studies may be attributed to the relatively small number of pure IMPC cases, potentially introducing bias (Table 3).
In their retrospective analysis, Verras et al. found that genomic sequencing was more likely to detect the luminal B subtype in IMPC than the immunohistochemical method (71). Furthermore, although the triple-negative subtype is less common in IMPC, it is associated with higher histological grade and more advanced disease stage in pathological diagnosis (72–74), indicating that the triple-negative subtype of IMPC is more aggressive. Upon diagnosis, surgery or neoadjuvant therapy is necessary to prevent rapid disease progression.
Biological hallmark of IMPC
Biological hallmarks are important for diagnosis, treatment information, and predicting the prognosis of IMPC of the breast. Nassar et al. found that mucin1 (MUC1), a glycoprotein encoded by MUC1 on chromosome 1q21, also known as EMA, is normally expressed on the apical surface of glandular epithelial cells. It is crucial for maintaining lumen formation (75). In IDC, MUC1 was mainly expressed in the cytoplasm, intercellular space, and apical regions. However, in IMPC, MUC1 was predominantly expressed on the basal surface of the cells, forming a prominent linear staining band. This band highlighted the outline of the micropapillary structure, providing a basis for diagnosis. MUC1 has antiadhesive and immunosuppressive properties and can protect against infections. These attributes also position MUC1 as a potential therapeutic target for IMPC (76).
Notably, the staining patterns of sialyl Lewis X (sLeX), MUC4, β1 integrin, and RAC1 reflect the polarity reversal characteristic of IMPC and serve as diagnostic markers for IMPC. Their positive expression is closely related to poor prognosis among patients with IMPC (42, 65). However, Sozzani et al. reported that sLex expression was not a prognostic factor of IMPC (77). Song et al. reported that high sLex expression on the tumor cell membrane in IMPC was associated with shorter overall survival (OS) and disease-free survival (DFS) (P = 0.030, P < 0.001, respectively) (42). Mercogliano et al. demonstrated that high MUC4 expression was associated with shorter DFS in patients with HER2-overexpressing IMPC (P = 0.019) (65). Liu et al. showed that overexpression of β1 integrin and RAC1 was associated with LNM and shorter DFS in IMPC (78).
CD44, a cell surface transmembrane glycoprotein, is involved in tumor cell differentiation, invasion, and metastasis. Badyal et al. showed that the loss of CD44 in IMPC is correlated with LNM, indicating its potential as a marker to predict LNM. This phenomenon is attributed to the re-expression of CD44 in lymph nodes, which guides the homing of tumor cells to lymph nodes. Umeda et al. validated this finding (79, 80).
Meng et al. (81) reported that high expression of prostate stem cell antigen (PSCA), located on chromosome 8q24 and a glycosylphosphatidylinositol-anchored cell surface protein, was significantly associated with shorter DFS among patients with IMPC (P = 0.0003). Liu et al. subsequently reported that Jagged1 expression in IMPC was linked to large tumor size, LVI, and Ki67, and it emerged as an independent prognostic factor for DFS and OS (63).
AT-rich interaction domain 1A (ARID1A), a novel tumor suppressor gene, is a part of the multiprotein SWI/SNF chromatin remodeling complex and plays an important role in inhibiting the proliferation, differentiation, and invasion of tumor cells. Onder et al. reported that low ARID1A expression was a predictor of shorter OS and DFS in IMPC (HR = 15.9, 95% CI: 3.5–71.5, P < 0.0001; HR = 7.2, 95% CI: 2–25.4, P = 0.002). Additionally, ARID1A expression exhibits a similar trend in both pure and mixed IMPC (82).
Moreover, cyclooxygenase-2 (COX-2), lymphoid enhancer-binding factor 1 (LEF1), β-catenin, galectin-3, interleukin-1beta (IL-1β), and autocrine motility factor receptor (AMFR) were associated with poor prognosis in IMPC (46, 83–87) (Table 4 and Figure 2).
Underlying pathogenesis of IMPC
The key to reducing the high-risk biological behavior of IMPC lies in obtaining a thorough understanding of its pathogenesis and developing individualized treatment approaches for patients with IMPC. In recent years, with advances in research, the onset of IMPC is linked to tumor cell polarity and the tumor-immune microenvironment, including tumor-associated macrophages (TAMs), metabolic reprograming, post-translational modification (PTMs), related signaling pathways, genomic mutations, and copy number variations (CNV) (Figure 3).
Tumor cell polarity of IMPC
The cell polarity reversal pattern is a unique morphological feature of IMPC and is associated with poor prognosis. Uncovering its underlying molecular biological mechanism has become a topic of interest. According to Gruel et al. (88), LIN7A was identified as one of the most differentially expressed genes in IDC and IMPC via genome sequencing. LIN7A overexpression in IMPC was further confirmed at the mRNA and protein levels. Meanwhile, LIN7A overexpression caused cell polarity reversal and tube formation in MCF-10A and MDA-MB-231 3D cell culture models. Additionally, the experimental results demonstrated that tumor cells exhibiting reversed cell polarity displayed stronger proliferation, invasion, and metastatic abilities than those with normal polarity. To our knowledge, this is the first report highlighting the significant role of LIN7A in the regulation of tumor cell polarity. However, its upstream and downstream regulatory mechanisms are yet to be explored.
In the study by Liu et al. (78), RAC1 overexpression induced the reversal of tumor cell polarity and affected the prognosis of patients with IMPC. Following the silencing of the β1 integrin gene via RNA interference (RNAi), there was a significant downregulation in β1 integrin expression and RAC1 expression. In contrast, inhibition of RAC1 expression by RNAi did not downregulate β1 integrin expression, leading to the speculation that β1 integrin positively regulates RAC1. Furthermore, AIIB2, a β1 integrin inhibitor, abrogated the reversal of tumor cell polarity induced by RAC1 overexpression. Therefore, β1 integrin induces the reversal of tumor cell polarity by positively regulating RAC1 expression, thereby influencing IMPC prognosis.
Although MUC1, MUC4, and sLex are also considered to be associated with tumor cell polarity reversal and poor prognosis, further in-depth molecular mechanisms have not been reported (42, 65, 75).
Tumor microenvironment of IMPC
TAMs
TAMs, important mediators of tumor growth, are functionally categorized into two contrasting subtypes, classical activated M1 macrophages and alternatively activated M2 macrophages (89). The former typically exerts antitumor effects, whereas the latter can promote the occurrence and metastasis of tumor cells. The distinctive feature of M2 macrophages is the expression of the scavenger receptor CD163 (90).
TAM differentiation is reported to depend on Notch signaling modulation (91). Upregulation of Notch-1 and its signaling following macrophage activation modulate gene expression patterns, affecting antigen-presenting capacity and cytotoxic activity. Jagged1, a Notch receptor ligand, is also important in the regulation of tumor occurrence and development of tumors (92).
Liu et al. (63) reported that Jagged1 was highly expressed in IMPC compared with IDC and served as an independent prognostic factor for DFS. Jagged1 expression was positively correlated with the infiltration of CD163+ M2 macrophages in the tumor stroma.
Moreover, PJA2, which regulates the intensity and duration of cAMP signaling via protein kinase A (PKA), enhances the accumulation of ubiquitinated malignant fibrous histiocytoma amplified sequence 1, thereby promoting M1 macrophage polarization and M2 to M1 macrophage transformation (93, 94). Aberrant expression of PJA2 in IMPC promotes tumor invasion via M2 macrophage polarization (95).
TAMs are hypothesized to be influenced by various factors in the IMPC environment, causing a decrease in M1 macrophages and an increase in the number of M2 macrophages, thereby promoting tumor invasion. Hence, targeting jagged1 and PJA2 to reduce the formation of M2 macrophages could represent a potential therapeutic approach for IMPC.
Natural killer T cells
Kanomata et al. demonstrated that CD1d was expressed at abnormally low levels in IMPC (95). CD1d is a lipid antigen that activates natural killer T cells (NKT) by interacting with T-cell receptors on the cell membrane (96). Hix et al. found that low expression of CD1d compromised the immune function of NKT toward tumor cells and promoted the metastasis of breast cancer in vitro and in vivo (97). The decreased expression of CD1d in IMPC may enable tumor cells to evade immune system regulation and enhance the metastatic potential of IMPC. Improving the immune surveillance function of NTK may be another effective treatment for IMPC.
Metabolic reprograming of IMPC
Using spatial transcriptome sequencing, Lv et al. (98) mapped the transcriptional profile of IMPC for the first time. IMPC heterogeneity is associated with metabolic reprograming involving unsaturated fatty acid metabolism, long-chain fatty acid metabolism, amino acid metabolism, carbohydrate metabolism, and glycolysis.
Furthermore, their data revealed that SREBF1 expression was significantly higher in IMPC clusters than in IDC clusters. Interestingly, FASN, a target gene of SREBF1, was also highly expressed in IMPC clusters. Increased expression of SREBF1 and FASN is closely related to IMPC survival. SREBF1 is a key transcription factor regulating FASN in lipid metabolism. FASN is a key enzyme involved in the de novo synthesis of long-chain fatty acids (99).
SREBF1/FASN affects the heterogeneity of IMPC through lipid metabolism and may be a potential therapeutic target of IMPC.
Post-translational modifications of IMPC
PTMs induce structural changes in existing proteins to participate in multiple biological processes, including tumor initiation, progression, and invasion (100). There are up to 600 types of post-translational modifications of human proteins (101), with common types including acetylation, methylation, phosphorylation, propionylation, butyrylation, crotonylation, 2-hydroxyisobutyrylation, malonylation, and succinylation.
Methylation
Protein arginine methyl-transferases play an important role in arginine methylation and are involved in metabolic reprograming during tumorigenesis (102, 103).
Zhi et al. (104), through a comparison of metabolomic differences between IMPC and IDC, identified aberrant arginine methylation and overexpression of protein arginine methyl-transferases 3 (PRMT3) in IMPC. PRMT3, a methylation “writer,” is closely related to arginine methylation. Subsequently, FLAG-tag, CO-IP, and bioinformatics analyses revealed that PRMT3 interacts with histone H4 to increase H4R3me2a levels and mediates the expression of ER stress-related genes. Meanwhile, PRMT3 overexpression promoted tumor proliferation, whereas PRMT3 knockdown inhibited tumor growth in vitro and in vivo.
Wu et al. (23) found that high expression of protein arginine methyl-transferases 1 (PRMT1) was associated with shorter DFS in IMPC. In cellular experiments, PRMT1 also upregulated H4R3me2a to induce tumor cell proliferation and promote tumor cell metastasis via the tumor necrosis factor signaling pathway.
Phosphorylation
Protein phosphorylation, a crucial PTM, occurs mainly on serine, threonine, and tyrosine residues and is a reversible process regulated by kinases and phosphatases. Kinases play a significant role in the growth, migration, and invasion of malignant tumors (105, 106).
Chen et al. (107) analyzed the proteomic and phosphoproteomic characteristics of IMPC by LC–MS/MS. Sequencing data revealed that 589 phosphosites on 479 phosphoproteins were considered to be highly phosphorylated in IMPC, whereas 267 phosphosites on 176 phosphoproteins were down-regulated. Enrichment analysis of differentially phosphorylated proteins through GO and KEGG revealed that upregulated phosphoproteins were primarily associated with enzyme activator activity. Kinase enrichment analysis (KSEA) indicated that cyclin-dependent kinases and p90 ribosomal S6 kinases (RSKs) were highly activated, whereas protein kinase A (PKA) and protein kinase C (PKC) families were significantly inhibited in IMPC. Simultaneously, the tumor-specific mTORC1/S6K2 signaling pathway was significantly activated in IMPC. Unfortunately, despite significant attention to the proteome and phosphoproteome profiles of IMPC, the phosphorylation sites of specific proteins associated with IMPC have not been validated by in vitro and in vivo experiments.
This is the first in-depth proteomic and phosphoproteomic study to explore the pathogenesis of IMPC. The findings of this study suggest that IMPC mediates the activation of proto-oncogenes or the repression of tumor suppressor genes through protein phosphorylation. This process can influence cell cycle regulation and enhance proliferative growth signals to promote tumorigenesis and rapid progression.
Other modifications
PTMs are considered closely related to breast cancer. In triple-negative breast cancer, Krug et al. reported (108) that dysregulation of SIRT3 protein extensively affected mitochondrial acetylation in BRCA, leading to increased aerobic glycolysis and impaired tricarboxylic acid cycle. Ding et al. demonstrated that GPX4 ubiquitination upregulates EGR1 to induce mitochondrial-mediated apoptosis in triple-negative breast cancer cells (109). Pandkar et al. (110) reported that increased lactate production leads to histone H3 lysine 18 lactylation (H3K18la)-mediated upregulation of c-Myc expression, enhancing the invasion of hormone receptor-positive breast cancer. While the modification omics of acetylation, ubiquitination, and lactacylation of IMPC have not been reported, these modifications may play crucial roles in the heterogeneity of IMPC and serve as potential therapeutic targets for IMPC.
Genomic mutations and copy number variation
Genomic mutations and CNV are considered to drive tumorigenesis and initiate intratumor heterogeneity (111, 112). As reported by Shi et al. (113), CNV in IMPC were positively correlated with LNM by whole exome sequencing and whole genome sequencing of IMPC samples and paired normal tissues, along with cell cluster sequencing of primary IMPC lesions and paired lymph nodes. PRDM16 and IGSF9 copy number losses and ALDH2 copy number gains were observed in IMPC. Furthermore, COX regression analysis confirmed that low expression of PRDM16 and IGSF9 and high expression of ALDH2 were associated with LNM and poor survival in patients with IMPC.
Related signaling pathways of IMPC
PI3K/Akt/Bcl-2 axis
Plakoglobin, a member of the armadillo protein family, is an important component of adhesion junctions and delaminating bodies and can promote tumor cell aggregation and metastasis (114, 115). Huang et al. (116) found that in vivo and in vitro data showed that plakoglobin was overexpressed in the cell membrane and cytoplasm of IMPC. Plakoglobin knockout resulted in cluster depolymerization, whereas plakoglobin overexpression activated the PI3K/Akt/Bcl-2 signaling pathway and reduced cluster apoptosis in cell models. Furthermore, plakoglobin knockout inhibited tumor proliferation in animal models.
Wnt/β-catenin Axis
Dolezal et al. (46) reported that lymphoid enhancer-binding factor 1 (LEF1) and β-catenin expression were significantly increased in lymph node lesions compared with primary lesions in IMPC. Wnt/β-catenin plays a crucial role in the invasion of malignant tumors, and LEF1 is a specific marker of this signaling pathway (117, 118). Therefore, LEF1 overexpression may activate the Wnt/β-catenin pathway and contribute to lymph node tropism in IMPC. However, further basic experiments are required to validate this finding.
Survival prediction models and prognosis
The survival prediction model integrates the information of clinical, imaging, and pathological characteristics of the disease to establish an evaluation system for predicting the individual prognosis of patients. This model helps clinicians develop individualized treatment measures (119).
Meng et al. enrolled 388 patients diagnosed with IMPC and who underwent surgery between 2013 and 2017. A nomogram revealed that factors such as age, LNM, hormone receptor status, adjuvant radiotherapy, vascular invasion of lymph nodes, and postoperative radiotherapy significantly influenced LRR (120). Chen et al. used the Surveillance, Epidemiology, and End Results (SEER) database to construct a nomogram from 1,885 surgically treated patients with IMPC. They discovered that age ≥62 years at diagnosis, estrogen receptor negativity, and tumor stage were adverse independent factors for OS. Conversely, married patients and those treated with chemotherapy or radiotherapy had longer postoperative survival (121). In the retrospective study by Wang et al. (122), patients with IMPC who underwent breast-conserving surgery had better OS and breast cancer-specific survival (BCSS) than those in the mastectomy group. However, patients in the breast-conserving group had a smaller tumor diameter, fewer LNMs, and higher ER- and PR-receptor positivity. In the retrospective study by Wang et al. (122), breast-conserving surgery demonstrated better OS and BCSS than mastectomy for IMPC. However, patients in the breast-conserving group had smaller tumor diameters, fewer LNMs, and higher hormone receptor positivity. Additionally, Lewis et al. screened 2,660 patients from the US National Cancer Database to construct a COX model. They discovered that the survival time of patients with ≥4 positive lymph nodes was significantly shorter than that of patients with negative lymph nodes (P < 0.001).
However, the survival time of patients with 1–3 positive lymph nodes was similar to that of patients with negative lymph nodes (P = 0.883), indicating that N2 is an independent prognostic factor for IMPC (123). These studies guide patients with IMPC who exhibit the aforementioned high-risk factors before and after surgery. This approach may include expanding the scope of surgery, intensifying systemic therapy, and increasing the dose of radiotherapy.
The presence of MP component within no special type tumors is a more frequent occurrence, and much deliberation has been made on its clinical significance. Multiple studies have reported an association between the presence of a MP element within a tumor and a poorer prognosis, along with a recurring lymphotropic pattern (39). Chen et al. retrospectively analyzed 100 patients with IMPC and found lower 5- and 10-year OS than those with IDC; however, the reliability of their findings was questionable because of the small number of patients enrolled (34). Li et al. (124) used propensity score matching to eliminate the difference between IMPC and IDC during the screening period and revealed that MP was a favorable prognostic factor. However, more studies have suggested that compared with IDC, IMPC has significantly higher relapse-free and local-regional recurrence-free survival rates, with no difference in OS. The varying outcomes of the aforementioned studies may be attributed to a lack of understanding of IMPC in the past, with numerous cases being misdiagnosed, leading to an underestimation of the disease’s impact. However, as the understanding of IMPC improves, its diagnostic rate is gradually increasing. This enables more cases to be treated effectively at an early stage.
Conclusions
While most current studies suggest that IMPC does not affect OS, its highly aggressive behavior increases the risk of LNM and local recurrence, significantly affecting patients’ quality of life. Moreover, most of the current studies on IMPC are retrospective analyses, making it challenging to correct biases related to tumor stage, surgical methods, and systemic treatments. As a result, there are significant differences between the findings of these studies. Hence, assessing whether IMPC affects OS requires extensive large-scale prospective studies. From a histological perspective, the “inside-out” growth pattern reflects the external manifestation of IMPC’s high invasiveness. However, from a pathogenesis perspective, IMPC heterogeneity is driven by multiple factors, such as tumor-immune microenvironment, TAMs, and metabolic reprogramming. In this review, we focused on recent advances in the biomarkers, pathogenesis, and survival prediction models of IMPC. Additionally, we aimed to deepen our understanding of tumor heterogeneity, provide valuable insights into potential treatment targets, and identify the underlying mechanisms of IMPC to improve treatment strategies.
Author contributions
PQ: Writing – original draft, Data curation, Software. QC: Formal analysis, Writing – review & editing. SH: Visualization, Writing – review & editing. YZ: Writing – review & editing, Supervision. HZ: Supervision, Writing – review & editing, Writing – original draft. HL: Supervision, Writing – review & editing.
Funding
The author(s) declare financial support was received for the research, authorship, and/or publication of this article. This study was supported by National Natural Science Foundation of China (grant number: 82102987), and GuangDong Basic and Applied Basic Research Foundation (grant number: 2022A1515012420).
Acknowledgments
We thank Figdraw (www.figdraw.com) for figure drawing.
Conflict of interest
The authors declare that the research was conducted in the absence of any commercial or financial relationships that could be construed as a potential conflict of interest.
Publisher’s note
All claims expressed in this article are solely those of the authors and do not necessarily represent those of their affiliated organizations, or those of the publisher, the editors and the reviewers. Any product that may be evaluated in this article, or claim that may be made by its manufacturer, is not guaranteed or endorsed by the publisher.
Abbreviations
IMPC, Invasive micropapillary carcinoma of the breast; MP, micropapillary pattern; LNM, lymph node metastasis; LVI, lymphovascular invasion; LRR, locoregional recurrence; IDC, invasive ductal carcinoma; EMA, epithelial membrane antigen; MRI, magnetic resonance imaging; PET-CT, positron emission tomography-computed tomography; SUVmax, maximum standardized uptake value; ER, estrogen receptor; PR, progesterone receptor; OS, overall survival; MUC1, mucin1; sLeX, sialyl Lewis X; DFS, disease-free survival; PSCA, prostate stem cell antigen; AMFR, autocrine motility factor receptor; TAM, tumor-associated macrophage; PTM, post-translational modification; CNV, copy number variation; PKA, protein kinase A; NKT, killer T cells; KSEA, kinases enrichment analysis; RSK, ribosomal S6 kinase; PKC, protein kinase C; WES, whole exome sequencing; WGS, whole genome sequencing; SEER, Surveillance, Epidemiology, and End Results; BCSS, breast cancer-specific survival.
References
1. Hoda SA, Brogi E, Koerner FC, Rosen PP. Rosen’s Breast Pathology, America: Lippincott Williams & Wilkins (LWW) 4th ed. (2014).
2. Li YM, He YY, Sun PL, Gao HW. Correlation of micropapillary patten, cribriform pattern and retraction clefting of endocervical adenocarcinoma with the silva classification system and prognosis. Zhonghua Bing Li Xue Za Zhi. (2022) 51:596–601. doi: 10.3760/cma.j.cn112151-20220326-00225
3. Smith Sehdev AE, Sehdev PS, Kurman RJ. Noninvasive and invasive micropapillary (Low-grade) serous carcinoma of the ovary: A clinicopathologic analysis of 135 cases. Am J Surg Pathol. (2003) 27:725–36. doi: 10.1097/00000478-200306000-00003
4. Liu J, Xi W, Zhou J, Gao W, Wu Q. Nomogram predicting overall prognosis for invasive micropapillary carcinoma of the breast: A seer-based population study. BMJ Open. (2023) 13:e072632. doi: 10.1136/bmjopen-2023-072632
5. Kuroda N, Hamaguchi N, Ohara M, Hirouchi T, Miyzaki E, Mizuno K. Intracytoplasmic lumina in invasive micropapillary carcinoma of the lung. Diagn Cytopathol. (2006) 34:224–6. doi: 10.1002/dc.20427
6. Lopez-Beltran A, Montironi R, Blanca A, Cheng L. Invasive micropapillary urothelial carcinoma of the bladder. Hum Pathol. (2010) 41:1159–64. doi: 10.1016/j.humpath.2009.11.018
7. Kitagawa H, Nakamura M, Tani T, Tajima H, Nakagawara H, Ohnishi I, et al. A pure invasive micropapillary carcinoma of the pancreatic head: long disease-free survival after pancreatoduodenectomy and adjuvant chemotherapy with gemcitabine. Pancreas. (2007) 35:190–2. doi: 10.1097/01.mpa.0000250142.02768.c7
8. Sakamoto K, Watanabe M, de la Cruz C, Honda H, Ise H, Mitsui K, et al. Primary invasive micropapillary carcinoma of the colon. Histopathology. (2005) 47:479–84. doi: 10.1111/j.1365-2559.2005.02241.x
9. Guzińska-Ustymowicz K, Niewiarowska K, Pryczynicz A. Invasive micropapillary carcinoma: A distinct type of adenocarcinomas in the gastrointestinal tract. World J Gastroenterol. (2014) 20:4597–606. doi: 10.3748/wjg.v20.i16.4597
10. Tomihara K, Miwa S, Takazakura T, Kamisaki Y, Noguchi M. Invasive micropapillary salivary duct carcinoma mixed with mucin-rich salivary duct carcinoma in minor salivary gland: A rare case report. Oral Surg Oral Med Oral Pathol Oral Radiol. (2016) 121:e162–7. doi: 10.1016/j.oooo.2015.10.012
11. Yu Y, Wang R, Deng J, Zhou J, Zhou H, Wang J. Invasive micropapillary carcinoma of mixed breast cancer metastasizing to the cervical region and thyroid: report of a rare case. Gland Surg. (2021) 10:3141–6. doi: 10.21037/gs-21-506
12. Young RH. Ovarian tumors: A survey of selected advances of note during the life of this journal. Hum Pathol. (2020) 95:169–206. doi: 10.1016/j.humpath.2019.09.002
13. Alvarado-Cabrero I, McCluggage WG, Estevez-Castro R, Pérez-Montiel D, Stolnicu S, Ganesan R, et al. Micropapillary cervical adenocarcinoma: A clinicopathologic study of 44 cases. Am J Surg Pathol. (2019) 43:802–9. doi: 10.1097/pas.0000000000001245
14. Xu ZY, Wang JP, Zhang Y, Wu SW, Ma L, Qin YZ, et al. Xp11.2 translocation/tfe3 gene fusion renal cell carcinoma with a micropapillary pattern: cases report and literature review. Am J Trans Res. (2019) 11:327–39.
15. Fisher ER, Sass R, Fisher B, Gregorio R, Brown R, Wickerham L. Pathologic findings from the national surgical adjuvant breast project (Protocol 6). II. Relation of local breast recurrence to multicentricity. Cancer. (1986) 57:1717–24. doi: 10.1002/1097-0142(19860501)57:9<1717::aid-cncr2820570902>3.0.co;2-h
16. Siriaunkgul S, Tavassoli FA. Invasive micropapillary carcinoma of the breast. Modern Pathol. (1993) 6:660–2.
17. Yang WT, Bu H. Updates in the 5(Th) edition of who classification of tumours of the breast. Zhonghua Bing Li Xue Za Zhi. (2020) 49:400–5. doi: 10.3760/cma.j.cn112151-20200303-00163
18. Yang WT, Zhu XZ. the introduction of 2012 who classification of tumours of the breast. Zhonghua Bing Li Xue Za Zhi. (2013) 42:78–80. doi: 10.3760/cma.j.issn.0529-5807.2013.02.002
19. Hanby AM, Walker C. Tavassoli fa, devilee P: pathology and genetics: tumours of the breast and female genital organs. Who classification of tumours series - volume IV. Lyon, France: Iarc Press. Breast Cancer Res. (2004) 6:133. doi: 10.1186/bcr788
20. Cheng LH, Yu XJ, Zhang H, Zhang HJ, Jia Z, Wang XH. Advances in invasive micropapillary carcinoma of the breast research: A review. Medicine. (2024) 103:e36631. doi: 10.1097/md.0000000000036631
21. Li W, Han Y, Wang C, Guo X, Shen B, Liu F, et al. Precise pathologic diagnosis and individualized treatment improve the outcomes of invasive micropapillary carcinoma of the breast: A 12-year prospective clinical study. Modern Pathol. (2018) 31:956–64. doi: 10.1038/s41379-018-0024-8
22. Liu F, Yang M, Li Z, Guo X, Lin Y, Lang R, et al. Invasive micropapillary mucinous carcinoma of the breast is associated with poor prognosis. Breast Cancer Res Treat. (2015) 151:443–51. doi: 10.1007/s10549-015-3413-4
23. Wu K, Li W, Liu H, Niu C, Shi Q, Zhang J, et al. Metabolome sequencing reveals that protein arginine-N-methyltransferase 1 promotes the progression of invasive micropapillary carcinoma of the breast and predicts a poor prognosis. Am J Pathol. (2023) 193:1267–83. doi: 10.1016/j.ajpath.2023.05.010
24. Sagnak Yilmaz Z, Sarioglu S. Molecular pathology of micropapillary carcinomas: is characteristic morphology related to molecular mechanisms? Appl Immunohistochem Mol Morphol. (2023) 31:267–77. doi: 10.1097/pai.0000000000001123
25. He J, Kang D, Shen T, Zheng L, Zhan Z, Xi G, et al. Label-free detection of invasive micropapillary carcinoma of the breast using multiphoton microscopy. J Biophotonics. (2023) 16:e202200224. doi: 10.1002/jbio.202200224
26. Kawasaki T, Tashima T, Muramatsu C, Fujimoto A, Usami Y, Kodama H, et al. Neuroendocrine tumor of the breast showing invasive micropapillary features and multiple lymph node metastases. Cancer Rep (Hoboken). (2023) 6:e1775. doi: 10.1002/cnr2.1775
27. Sun Y, Gu W, Wang G, Zhou X. The clinicopathological and prognostic characteristics of mucinous micropapillary carcinoma of the breast. Histol Histopathol. (2022) 37:691–8. doi: 10.14670/hh-18-436
28. Asano Y, Kashiwagi S, Nagamori M, Tanaka S, Kuwae Y, Amano R, et al. Pure mucinous breast carcinoma with micropapillary pattern (Mumpc): A case report. Case Rep Oncol. (2019) 12:554–9. doi: 10.1159/000501766
29. Collins K, Ricci A Jr. Micropapillary variant of mucinous breast carcinoma: A distinct subtype. Breast J. (2018) 24:339–42. doi: 10.1111/tbj.12935
30. Pareja F, Selenica P, Brown DN, Sebastiao APM, da Silva EM, Da Cruz Paula A, et al. Micropapillary variant of mucinous carcinoma of the breast shows genetic alterations intermediate between those of mucinous carcinoma and micropapillary carcinoma. Histopathology. (2019) 75:139–45. doi: 10.1111/his.13853
31. Eren Kupik G, Altundağ K. The clinicopathological characteristics of pure and mixed invasive micropapillary breast carcinomas: A single center experience. Balkan Med J. (2022) 39:275–81. doi: 10.4274/balkanmedj.galenos.2022.2022-4-7
32. Acs G, Esposito NN, Rakosy Z, Laronga C, Zhang PJ. Invasive ductal carcinomas of the breast showing partial reversed cell polarity are associated with lymphatic tumor spread and may represent part of a spectrum of invasive micropapillary carcinoma. Am J Surg Pathol. (2010) 34:1637–46. doi: 10.1097/PAS.0b013e3181f5539c
33. Guo X, Chen L, Lang R, Fan Y, Zhang X, Fu L. Invasive micropapillary carcinoma of the breast: association of pathologic features with lymph node metastasis. Am J Clin Pathol. (2006) 126:740–6. doi: 10.1309/axyy-4ajt-mnw6-frmw
34. Chen L, Fan Y, Lang RG, Guo XJ, Sun YL, Cui LF, et al. Breast carcinoma with micropapillary features: clinicopathologic study and long-term follow-up of 100 cases. Int J Surg Pathol. (2008) 16:155–63. doi: 10.1177/1066896907307047
35. Walsh MM, Bleiweiss IJ. Invasive micropapillary carcinoma of the breast: eighty cases of an underrecognized entity. Hum Pathol. (2001) 32:583–9. doi: 10.1053/hupa.2001.24988
36. Ide Y, Horii R, Osako T, Ogura K, Yoshida R, Iwase T, et al. Clinicopathological significance of invasive micropapillary carcinoma component in invasive breast carcinoma. Pathol Int. (2011) 61:731–6. doi: 10.1111/j.1440-1827.2011.02735.x
37. Al-Ahmadie H, Iyer G. Molecular Alterations in The pathogenesis of Bladder Cancer Subtypes and Urothelial Carcinoma Variants. In: Hansel DE, Lerner SP, editors. Precision Molecular Pathology of Bladder Cancer. Springer International Publishing, Cham (2018). p. 65–83.
38. Yang YL, Liu BB, Zhang X, Fu L. Invasive micropapillary carcinoma of the breast: an update. Arch Pathol Lab Med. (2016) 140:799–805. doi: 10.5858/arpa.2016-0040-RA
39. Verras GI, Mulita F, Tchabashvili L, Grypari IM, Sourouni S, Panagodimou E, et al. A rare case of invasive micropapillary carcinoma of the breast. Prz Menopauzalny. (2022) 21:73–80. doi: 10.5114/pm.2022.113834
40. Hui Y, Lombardo KA, Quddus MR, Matoso A. Cell polarity reversal distinguishes true micropapillary growth from retraction artifact in invasive urothelial carcinoma. Appl Immunohistochem Mol Morphol. (2018) 26:e1–6. doi: 10.1097/pai.0000000000000566
41. Kuba S, Ohtani H, Yamaguchi J, Hayashi H, Uga T, Kanematsu T, et al. Incomplete inside-out growth pattern in invasive breast carcinoma: association with lymph vessel invasion and recurrence-free survival. Virchows Arch. (2011) 458:159–69. doi: 10.1007/s00428-010-1033-2
42. Song Y, Sun H, Wu K, Lyu J, Zhang J, Gu F, et al. Sle(X) expression in invasive micropapillary breast carcinoma is associated with poor prognosis and can be combined with muc1/ema as a supplementary diagnostic indicator. Cancer Biol Med. (2021) 18:477–89. doi: 10.20892/j.issn.2095-3941.2020.0422
43. Lepe M, Kalife ET, Ou J, Quddus MR, Singh K. [amp]]lsquo;Inside-out’ P120 immunostaining pattern in invasive micropapillary carcinoma of the breast; additional unequivocal evidence of reversed polarity. Histopathology. (2017) 70:832–4. doi: 10.1111/his.13121
44. Arai K, Iwasaki T, Tsuchiya C, Sonoda A. Involvement of annexin A2 expression and apoptosis in reverse polarization of invasive micropapillary carcinoma of the breast. Case Rep Pathol. (2020) 2020:9242305. doi: 10.1155/2020/9242305
45. Pettinato G, Manivel CJ, Panico L, Sparano L, Petrella G. Invasive micropapillary carcinoma of the breast: clinicopathologic study of 62 cases of a poorly recognized variant with highly aggressive behavior. Am J Clin Pathol. (2004) 121:857–66. doi: 10.1309/xtj7-vhb4-9ud7-8x60
46. Dolezal D, Zhang X, Harigopal M. Increased expression of lef1 and β-catenin in invasive micropapillary carcinoma of the breast is associated with lymphovascular invasion and lymph node metastasis. Appl Immunohistochem Mol Morphol. (2022) 30:557–65. doi: 10.1097/pai.0000000000001052
47. Li Y, Liu J, Xu Z, Shang J, Wu S, Zhang M, et al. Construction and validation of a nomogram for predicting the prognosis of patients with lymph node-positive invasive micropapillary carcinoma of the breast: based on seer database and external validation cohort. Front Oncol. (2023) 13:1231302. doi: 10.3389/fonc.2023.1231302
48. Wolff AC, Hammond MEH, Allison KH, Harvey BE, Mangu PB, Bartlett JMS, et al. Human epidermal growth factor receptor 2 testing in breast cancer: American society of clinical oncology/college of American pathologists clinical practice guideline focused update. J Clin Oncol. (2018) 36:2105–22. doi: 10.1200/jco.2018.77.8738
49. Stewart RL, Caron JE, Gulbahce EH, Factor RE, Geiersbach KB, Downs-Kelly E. Her2 immunohistochemical and fluorescence in situ hybridization discordances in invasive breast carcinoma with micropapillary features. Modern Pathol. (2017) 30:1561–6. doi: 10.1038/modpathol.2017.65
50. Akrida I, Mulita F. The clinical significance of her2 expression in dcis. Med Oncol. (2022) 40:16. doi: 10.1007/s12032-022-01876-9
51. Siziopikou KP, Anderson SJ, Cobleigh MA, Julian TB, Arthur DW, Zheng P, et al. Preliminary results of centralized her2 testing in ductal carcinoma in situ (Dcis): nsabp B-43. Breast Cancer Res Treat. (2013) 142:415–21. doi: 10.1007/s10549-013-2755-z
52. Yun SU, Choi BB, Shu KS, Kim SM, Seo YD, Lee JS, et al. Imaging findings of invasive micropapillary carcinoma of the breast. J Breast Cancer. (2012) 15:57–64. doi: 10.4048/jbc.2012.15.1.57
53. Lim HS, Kuzmiak CM, Jeong SI, Choi YR, Kim JW, Lee JS, et al. Invasive micropapillary carcinoma of the breast: mr imaging findings. Korean J Radiol. (2013) 14:551–8. doi: 10.3348/kjr.2013.14.4.551
54. Jones KN, Guimaraes LS, Reynolds CA, Ghosh K, Degnim AC, Glazebrook KN. Invasive micropapillary carcinoma of the breast: imaging features with clinical and pathologic correlation. AJR Am J Roentgenol. (2013) 200:689–95. doi: 10.2214/ajr.12.8512
55. Alsharif S, Daghistani R, Kamberoğlu EA, Omeroglu A, Meterissian S, Mesurolle B. Mammographic, sonographic and mr imaging features of invasive micropapillary breast cancer. Eur J Radiol. (2014) 83:1375–80. doi: 10.1016/j.ejrad.2014.05.003
56. Kubota K, Ogawa Y, Nishioka A, Murata Y, Itoh S, Hamada N, et al. Radiological imaging features of invasive micropapillary carcinoma of the breast and axillary lymph nodes. Oncol Rep. (2008) 20:1143–7.
57. Fakhry S, Nada Y, Mohamed MM, Kamal RM, Eltohamy MI, Taha SNM, et al. Radiological characteristics of invasive micropapillary carcinoma of the breast. Clin Radiol. (2024) 79:e34–40. doi: 10.1016/j.crad.2023.09.010
58. Akgün E, Alçın G, Kelten Talu EC, Çermik TF, Söylemez Akkurt T, Şen E, et al. Investigation of clinical histopathologic features and metabolic parameters of (18)F-fdg pet/ct in invasive breast carcinoma with a micropapillary component. Mol Imaging Radionucl Ther. (2023) 32:221–5. doi: 10.4274/mirt.galenos.2023.06078
59. Akrida I, Michalopoulos NV, Lagadinou M, Papadoliopoulou M, Maroulis I, Mulita F. An updated review on the emerging role of indocyanine green (Icg) as a sentinel lymph node tracer in breast cancer. Cancers (Basel). (2023) 15:5755. doi: 10.3390/cancers15245755
60. Gokce H, Durak MG, Akin MM, Canda T, Balci P, Ellidokuz H, et al. Invasive micropapillary carcinoma of the breast: A clinicopathologic study of 103 cases of an unusual and highly aggressive variant of breast carcinoma. Breast J. (2013) 19:374–81. doi: 10.1111/tbj.12128
61. Chen H, Wu K, Wang M, Wang F, Zhang M, Zhang P. Invasive micropapillary carcinoma of the breast has a better long-term survival than invasive ductal carcinoma of the breast in spite of its aggressive clinical presentations: A comparison based on large population database and case-control analysis. Cancer Med. (2017) 6:2775–86. doi: 10.1002/cam4.1227
62. Rechsteiner A, Dietrich D, Varga Z. Prognostic relevance of mixed histological subtypes in invasive breast carcinoma: A retrospective analysis. J Cancer Res Clin Oncol. (2023) 149:4967–78. doi: 10.1007/s00432-022-04443-x
63. Liu H, Wang J, Liu Z, Wang L, Liu S, Zhang Q. Jagged1 modulated tumor-associated macrophage differentiation predicts poor prognosis in patients with invasive micropapillary carcinoma of the breast. Medicine. (2017) 96:e6663. doi: 10.1097/md.0000000000006663
64. Liu Y, Huang X, Bi R, Yang W, Shao Z. Similar prognoses for invasive micropapillary breast carcinoma and pure invasive ductal carcinoma: A retrospectively matched cohort study in China. PloS One. (2014) 9:e106564. doi: 10.1371/journal.pone.0106564
65. Mercogliano MF, Inurrigarro G, De Martino M, Venturutti L, Rivas MA, Cordo-Russo R, et al. Invasive micropapillary carcinoma of the breast overexpresses muc4 and is associated with poor outcome to adjuvant trastuzumab in her2-positive breast cancer. BMC Cancer. (2017) 17:895. doi: 10.1186/s12885-017-3897-x
66. Yu JI, Choi DH, Huh SJ, Cho EY, Kim K, Chie EK, et al. Differences in prognostic factors and failure patterns between invasive micropapillary carcinoma and carcinoma with micropapillary component versus invasive ductal carcinoma of the breast: retrospective multicenter case-control study (Krog 13-06). Clin Breast Cancer. (2015) 15:353–61.e1-2. doi: 10.1016/j.clbc.2015.01.008
67. Aker FV, Ekren E, Dogan M, Gurleyik G, Tanrikulu E, Oven BB. Clinicopathological features and prognosis of invasive micropapillary carcinoma compared to invasive ductal carcinoma-nos: worse or better? J Coll Physicians Surg Pak. (2022) 32:1196–201. doi: 10.29271/jcpsp.2022.09.1196
68. Wang R, Li N, Wang XJ, Chen T, Zhang H, Cheng Y, et al. Differences in the clinicopathological characteristics of pure and mixed invasive micropapillary breast carcinomas from eastern China. Ann Trans Med. (2021) 9:412. doi: 10.21037/atm-20-8045
69. Kaya C, Uçak R, Bozkurt E, Ömeroğlu S, Kartal K, Yazıcı P, et al. The impact of micropapillary component ratio on the prognosis of patients with invasive micropapillary breast carcinoma. J Invest Surg. (2020) 33:31–9. doi: 10.1080/08941939.2018.1474302
70. Chen AC, Paulino AC, Schwartz MR, Rodriguez AA, Bass BL, Chang JC, et al. Population-based comparison of prognostic factors in invasive micropapillary and invasive ductal carcinoma of the breast. Br J Cancer. (2014) 111:619–22. doi: 10.1038/bjc.2014.301
71. Verras GI, Tchabashvili L, Mulita F, Grypari IM, Sourouni S, Panagodimou E, et al. Micropapillary breast carcinoma: from molecular pathogenesis to prognosis. Breast Cancer (Dove Med Press). (2022) 14:41–61. doi: 10.2147/bctt.S346301
72. Wu Y, Zhang N, Yang Q. The prognosis of invasive micropapillary carcinoma compared with invasive ductal carcinoma in the breast: A meta-analysis. BMC Cancer. (2017) 17:839. doi: 10.1186/s12885-017-3855-7
73. Hashmi AA, Aijaz S, Mahboob R, Khan SM, Irfan M, Iftikhar N, et al. Clinicopathologic features of invasive metaplastic and micropapillary breast carcinoma: comparison with invasive ductal carcinoma of breast. BMC Res Notes. (2018) 11:531. doi: 10.1186/s13104-018-3623-z
74. Kim J, Kim JY, Lee HB, Lee YJ, Seong MK, Paik N, et al. Characteristics and prognosis of 17 special histologic subtypes of invasive breast cancers according to world health organization classification: comparative analysis to invasive carcinoma of no special type. Breast Cancer Res Treat. (2020) 184:527–42. doi: 10.1007/s10549-020-05861-6
75. Nassar H, Pansare V, Zhang H, Che M, Sakr W, Ali-Fehmi R, et al. Pathogenesis of invasive micropapillary carcinoma: role of muc1 glycoprotein. Modern Pathol. (2004) 17:1045–50. doi: 10.1038/modpathol.3800166
76. Apostolopoulos V, Stojanovska L, Gargosky SE. Muc1 (Cd227): A multi-tasked molecule. Cell Mol Life sciences: CMLS. (2015) 72:4475–500. doi: 10.1007/s00018-015-2014-z
77. Sozzani P, Arisio R, Porpiglia M, Benedetto C. Is sialyl lewis X antigen expression a prognostic factor in patients with breast cancer? Int J Surg Pathol. (2008) 16:365–74. doi: 10.1177/1066896908324668
78. Liu B, Zheng X, Meng F, Han Y, Song Y, Liu F, et al. Overexpression of β1 integrin contributes to polarity reversal and a poor prognosis of breast invasive micropapillary carcinoma. Oncotarget. (2018) 9:4338–53. doi: 10.18632/oncotarget.22774
79. Umeda T, Ishida M, Murata S, Mori T, Kawai Y, Itoi N, et al. Immunohistochemical analyses of cd44 variant isoforms in invasive micropapillary carcinoma of the breast: comparison with a concurrent conventional invasive carcinoma of no special type component. Breast Cancer. (2016) 23:869–75. doi: 10.1007/s12282-015-0653-4
80. Badyal RK, Bal A, Das A, Singh G. Invasive micropapillary carcinoma of the breast: immunophenotypic analysis and role of cell adhesion molecules (Cd44 and E-cadherin) in nodal metastasis. Appl Immunohistochem Mol Morphol. (2016) 24:151–8. doi: 10.1097/pai.0000000000000167
81. Meng F, Liu B, Xie G, Song Y, Zheng X, Qian X, et al. Amplification and overexpression of psca at 8q24 in invasive micropapillary carcinoma of breast. Breast Cancer Res Treat. (2017) 166:383–92. doi: 10.1007/s10549-017-4407-1
82. Onder S, Fayda M, Karanlık H, Bayram A, Şen F, Cabioglu N, et al. Loss of arid1a expression is associated with poor prognosis in invasive micropapillary carcinomas of the breast: A clinicopathologic and immunohistochemical study with long-term survival analysis. Breast J. (2017) 23:638–46. doi: 10.1111/tbj.12823
83. Xu J, Ma H, Wang Q, Zhang H. Expression of autocrine motility factor receptor (Amfr) in human breast and lung invasive micropapillary carcinomas. Int J Exp Pathol. (2023) 104:43–51. doi: 10.1111/iep.12462
84. Vieira TC, Oliveira EA, Dos Santos BJ, Souza FR, Veloso ES, Nunes CB, et al. Cox-2 expression in mammary invasive micropapillary carcinoma is associated with prognostic factors and acts as a potential therapeutic target in comparative oncology. Front Vet Sci. (2022) 9:983110. doi: 10.3389/fvets.2022.983110
85. Çakır Y, Kelten Talu C, Mermut Ö, Can Trabulus D, Arslan E. The expression of galectin-3 in tumor and cancer-associated fibroblasts in invasive micropapillary breast carcinomas: relationship with clinicopathologic parameters. Eur J Breast Health. (2021) 17:341–51. doi: 10.4274/ejbh.galenos.2021.2021-2-8
86. Cui LF, Guo XJ, Wei J, Liu FF, Gu F, Fan Y, et al. Significance of interleukin-1beta expression and microvascular density in invasive micropapillary carcinoma of breast. Zhonghua Bing Li Xue Za Zhi. (2008) 37:599–603.
87. Liu F, Lang R, Wei J, Fan Y, Cui L, Gu F, et al. Increased expression of sdf-1/cxcr4 is associated with lymph node metastasis of invasive micropapillary carcinoma of the breast. Histopathology. (2009) 54:741–50. doi: 10.1111/j.1365-2559.2009.03289.x
88. Gruel N, Fuhrmann L, Lodillinsky C, Benhamo V, Mariani O, Cédenot A, et al. Lin7a is a major determinant of cell-polarity defects in breast carcinomas. Breast Cancer research: BCR. (2016) 18:23. doi: 10.1186/s13058-016-0680-x
89. Pan Y, Yu Y, Wang X, Zhang T. Tumor-associated macrophages in tumor immunity. Front Immunol. (2020) 11:583084. doi: 10.3389/fimmu.2020.583084
90. Yamaguchi Y, Gibson J, Ou K, Lopez LS, Ng RH, Leggett N, et al. Pd-L1 blockade restores car T cell activity through ifn-Γ-regulation of cd163+ M2 macrophages. J Immunother Cancer. (2022) 10:e004400. doi: 10.1136/jitc-2021-004400
91. Franklin RA, Liao W, Sarkar A, Kim MV, Bivona MR, Liu K, et al. The cellular and molecular origin of tumor-associated macrophages. Sci (New York NY). (2014) 344:921–5. doi: 10.1126/science.1252510
92. Liu L, Zhao WY, Zheng XY. Znf746 promotes M2 macrophage polarisation and favours tumour progression in breast cancer via the jagged1/notch pathway. Cell signalling. (2023) 112:110892. doi: 10.1016/j.cellsig.2023.110892
93. Lignitto L, Carlucci A, Sepe M, Stefan E, Cuomo O, Nisticò R, et al. Control of pka stability and signalling by the ring ligase praja2. Nat Cell Biol. (2011) 13:412–22. doi: 10.1038/ncb2209
94. Zhong J, Wang H, Chen W, Sun Z, Chen J, Xu Y, et al. Ubiquitylation of mfhas1 by the ubiquitin ligase praja2 promotes M1 macrophage polarization by activating jnk and P38 pathways. Cell Death Dis. (2017) 8:e2763. doi: 10.1038/cddis.2017.102
95. Kanomata N, Kurebayashi J, Koike Y, Yamaguchi R, Moriya T. Cd1d- and pja2-related immune microenvironment differs between invasive breast carcinomas with and without a micropapillary feature. BMC Cancer. (2019) 19:76. doi: 10.1186/s12885-018-5221-9
96. Kawano T, Cui J, Koezuka Y, Toura I, Kaneko Y, Motoki K, et al. Cd1d-restricted and tcr-mediated activation of valpha14 nkt cells by glycosylceramides. Sci (New York NY). (1997) 278:1626–9. doi: 10.1126/science.278.5343.1626
97. Hix LM, Shi YH, Brutkiewicz RR, Stein PL, Wang CR, Zhang M. Cd1d-expressing breast cancer cells modulate nkt cell-mediated antitumor immunity in a murine model of breast cancer metastasis. PloS One. (2011) 6:e20702. doi: 10.1371/journal.pone.0020702
98. Lv J, Shi Q, Han Y, Li W, Liu H, Zhang J, et al. Spatial transcriptomics reveals gene expression characteristics in invasive micropapillary carcinoma of the breast. Cell Death Dis. (2021) 12:1095. doi: 10.1038/s41419-021-04380-6
99. Menendez JA, Decker JP, Lupu R. In support of fatty acid synthase (Fas) as a metabolic oncogene: extracellular acidosis acts in an epigenetic fashion activating fas gene expression in cancer cells. J Cell Biochem. (2005) 94:1–4. doi: 10.1002/jcb.20310
100. Li W, Li F, Zhang X, Lin HK, Xu C. Insights into the post-translational modification and its emerging role in shaping the tumor microenvironment. Signal Transduct Target Ther. (2021) 6:422. doi: 10.1038/s41392-021-00825-8
101. Huang H, Sabari BR, Garcia BA, Allis CD, Zhao Y. Snapshot: histone modifications. Cell. (2014) 159:458–.e1. doi: 10.1016/j.cell.2014.09.037
102. Liu F, Ma F, Wang Y, Hao L, Zeng H, Jia C, et al. Pkm2 methylation by carm1 activates aerobic glycolysis to promote tumorigenesis. Nat Cell Biol. (2017) 19:1358–70. doi: 10.1038/ncb3630
103. Zhong XY, Yuan XM, Xu YY, Yin M, Yan WW, Zou SW, et al. Carm1 methylates gapdh to regulate glucose metabolism and is suppressed in liver cancer. Cell Rep. (2018) 24:3207–23. doi: 10.1016/j.celrep.2018.08.066
104. Zhi R, Wu K, Zhang J, Liu H, Niu C, Li S, et al. Prmt3 regulates the progression of invasive micropapillary carcinoma of the breast. Cancer Sci. (2023) 114:1912–28. doi: 10.1111/cas.15724
105. Bilbrough T, Piemontese E, Seitz O. Dissecting the role of protein phosphorylation: A chemical biology toolbox. Chem Soc Rev. (2022) 51:5691–730. doi: 10.1039/d1cs00991e
106. Revathidevi S, Munirajan AK. Akt in cancer: mediator and more. Semin Cancer Biol. (2019) 59:80–91. doi: 10.1016/j.semcancer.2019.06.002
107. Chen X, Lin Y, Jin X, Zhang W, Guo W, Chen L, et al. Integrative proteomic and phosphoproteomic profiling of invasive micropapillary breast carcinoma. J Proteomics. (2022) 257:104511. doi: 10.1016/j.jprot.2022.104511
108. Krug K, Jaehnig EJ, Satpathy S, Blumenberg L, Karpova A, Anurag M, et al. Proteogenomic landscape of breast cancer tumorigenesis and targeted therapy. Cell. (2020) 183:1436–56.e31. doi: 10.1016/j.cell.2020.10.036
109. Ding Y, Chen X, Liu C, Ge W, Wang Q, Hao X, et al. Identification of a small molecule as inducer of ferroptosis and apoptosis through ubiquitination of gpx4 in triple Negative Breast Cancer cells. J Hematol Oncol. (2021) 14:19. doi: 10.1186/s13045-020-01016-8
110. Pandkar MR, Sinha S, Samaiya A, Shukla S. Oncometabolite lactate enhances breast cancer progression by orchestrating histone lactylation-dependent C-myc expression. Trans Oncol. (2023) 37:101758. doi: 10.1016/j.tranon.2023.101758
111. Martincorena I, Campbell PJ. Somatic mutation in cancer and normal cells. Sci (New York NY). (2015) 349:1483–9. doi: 10.1126/science.aab4082
112. Erickson A, He M, Berglund E, Marklund M, Mirzazadeh R, Schultz N, et al. Spatially resolved clonal copy number alterations in benign and Malignant tissue. Nature. (2022) 608:360–7. doi: 10.1038/s41586-022-05023-2
113. Shi Q, Shao K, Jia H, Cao B, Li W, Dong S, et al. Genomic alterations and evolution of cell clusters in metastatic invasive micropapillary carcinoma of the breast. Nat Commun. (2022) 13:111. doi: 10.1038/s41467-021-27794-4
114. Küçükköse E, Laoukili J, Gorelick AN, Degner S, Laclé MM, van den Bent L, et al. Lymphatic invasion of plakoglobin-dependent tumor cell clusters drives formation of polyclonal lung metastases in colon cancer. Gastroenterology. (2023) 165:429–44.e15. doi: 10.1053/j.gastro.2023.02.047
115. Aceto N, Bardia A, Miyamoto DT, Donaldson MC, Wittner BS, Spencer JA, et al. Circulating tumor cell clusters are oligoclonal precursors of breast cancer metastasis. Cell. (2014) 158:1110–22. doi: 10.1016/j.cell.2014.07.013
116. Huang L, Ji H, Yin L, Niu X, Wang Y, Liu Y, et al. High expression of plakoglobin promotes metastasis in invasive micropapillary carcinoma of the breast via tumor cluster formation. J Cancer. (2019) 10:2800–10. doi: 10.7150/jca.31411
117. Zhang Y, Wang X. Targeting the wnt/β-catenin signaling pathway in cancer. J Hematol Oncol. (2020) 13:165. doi: 10.1186/s13045-020-00990-3
118. Wu L, Zhao JC, Kim J, Jin HJ, Wang CY, Yu J. Erg is a critical regulator of wnt/lef1 signaling in prostate cancer. Cancer Res. (2013) 73:6068–79. doi: 10.1158/0008-5472.Can-13-0882
119. Simons JP, Schanzer A, Flahive JM, Osborne NH, Mills JL Sr., Bradbury AW, et al. Survival prediction in patients with chronic limb-threatening ischemia who undergo infrainguinal revascularization. Eur J Vasc endovascular Surg. (2019) 58:S120–S34. doi: 10.1016/j.ejvs.2019.04.009
120. Meng X, Ma H, Yin H, Yin H, Yu L, Liu L, et al. Nomogram predicting the risk of locoregional recurrence after mastectomy for invasive micropapillary carcinoma of the breast. Clin Breast Cancer. (2021) 21:e368–e76. doi: 10.1016/j.clbc.2020.12.003
121. Chen Y, Yu C, Chen D, Tang Y, Zhu K, Guo R, et al. A prognostic nomogram based on risk assessment for invasive micropapillary carcinoma of the breast after surgery. Cancer Med. (2023) 12:8050–62. doi: 10.1002/cam4.5595
122. Wang S, Zhang Y, Yin F, Wang X, Yang Z. Survival outcomes after breast-conserving therapy compared with mastectomy for patients with early-stage invasive micropapillary carcinoma of the breast: A seer population-based study. Front Oncol. (2021) 11:741737. doi: 10.3389/fonc.2021.741737
123. Lewis GD, Xing Y, Haque W, Patel T, Schwartz M, Chen A, et al. Prognosis of lymphotropic invasive micropapillary breast carcinoma analyzed by using data from the national cancer database. Cancer Commun (Lond). (2019) 39:60. doi: 10.1186/s40880-019-0406-4
Keywords: breast, invasive micropapillary carcinoma, poor prognosis, opportunities, challenges
Citation: Qiu P, Cui Q, Huang S, Zhang Y, Zhang H and Luo H (2024) An overview of invasive micropapillary carcinoma of the breast: past, present, and future. Front. Oncol. 14:1435421. doi: 10.3389/fonc.2024.1435421
Received: 20 May 2024; Accepted: 28 October 2024;
Published: 15 November 2024.
Edited by:
Georgios-Ioannis Verras, Southampton General Hospital, United KingdomReviewed by:
Ioannis Boutas, National and Kapodistrian University of Athens, GreeceFrancesk Mulita, General Hospital of Eastern Achaia- Unit of Aigio, Greece
Jianguo Lai, Guangdong Provincial People’s Hospital, China
Copyright © 2024 Qiu, Cui, Huang, Zhang, Zhang and Luo. This is an open-access article distributed under the terms of the Creative Commons Attribution License (CC BY). The use, distribution or reproduction in other forums is permitted, provided the original author(s) and the copyright owner(s) are credited and that the original publication in this journal is cited, in accordance with accepted academic practice. No use, distribution or reproduction is permitted which does not comply with these terms.
*Correspondence: Hui Luo, bHVvaHVpQGdkbXUuZWR1LmNu; Haitao Zhang, dGFvaGFpemhhbmczM0AxNjMuY29t; Yuanqi Zhang, emhhbmd5dWFucWlAZ2RtdS5lZHUuY24=