- Department of Neurology, Memorial Sloan Kettering Cancer Center, New York, NY, United States
A common feature of advanced solid tumors is their ability to metastasize and colonize distant organs, including the Central Nervous System (CNS), which encompasses brain and leptomeningeal metastases (LM). While cerebrospinal fluid cytopathological analysis remains a gold standard diagnostic tool, it only provides limited insights into the biology of tumor cells; thus, it is urgent to develop minimally invasive biomarkers that enable a comprehensive quantitative and molecular characterization of disseminated cells, therapy response assessment, and disease monitoring. Liquid biopsy methods have been swiftly developed for some readily accessible bodily fluids such as plasma and urine; circulating tumor cells (CTCs) and circulating tumor DNA (ctDNA) from these sources have been rapidly implemented into clinical trial design, disease monitoring, and treatment assignment across different tumor types. However, the filter imposed by the brain blood barrier (BBB) hampers the release of tumor-derived cells and molecules from CNS metastases. Crucially, cerebrospinal fluid (CSF) liquid biopsy methods offer a unique and unparallel source to develop liquid biopsy methodologies in patients with CNS-disseminated disease, including the characterization of CTCs and ctDNA arising specifically from brain and leptomeningeal metastasis. These technologies have enabled a deeper understanding of tumor cell and molecular dynamics, including the reconstruction of clonal evolution in the brain microenvironment through longitudinal sapling. Here, we discuss the current challenges and opportunities that CSF liquid biopsy methods face for the implementation of these approaches into clinical settings.
Introduction
Central Nervous System (CNS) metastases, encompassing brain metastases (BrM) and leptomeningeal metastases (LM), represent some of the most serious and common neurological complications of solid tumors. Among all tumor types, breast, lung cancers and melanoma, have the highest likelihood of developing CNS metastases (1–3). across CNS malignancies, the incidence of CNS metastases is 3-10 times higher than the incidence of primary brain tumors (4). More than 25% of patients with stage IV lung cancer may have BrM initially and up to 54% of these patients will subsequently develop BM following the primary tumor diagnosis (5–7). In breast cancer 5-20% of patients and in melanoma 7-16% of patients, developed BrM (8–11). Another devastating neurologic complication is LM whose incidence is challenging to determine at earlier stages based on current diagnostic criteria (12). LM can occur as a standalone disease without BrM, be present at the time of BrM diagnosis in up to 30% of patients or develop much later in patients with BrM (13) (Figure 1). The timing of LM diagnosis holds specific prognostic significance related to its detection (14).
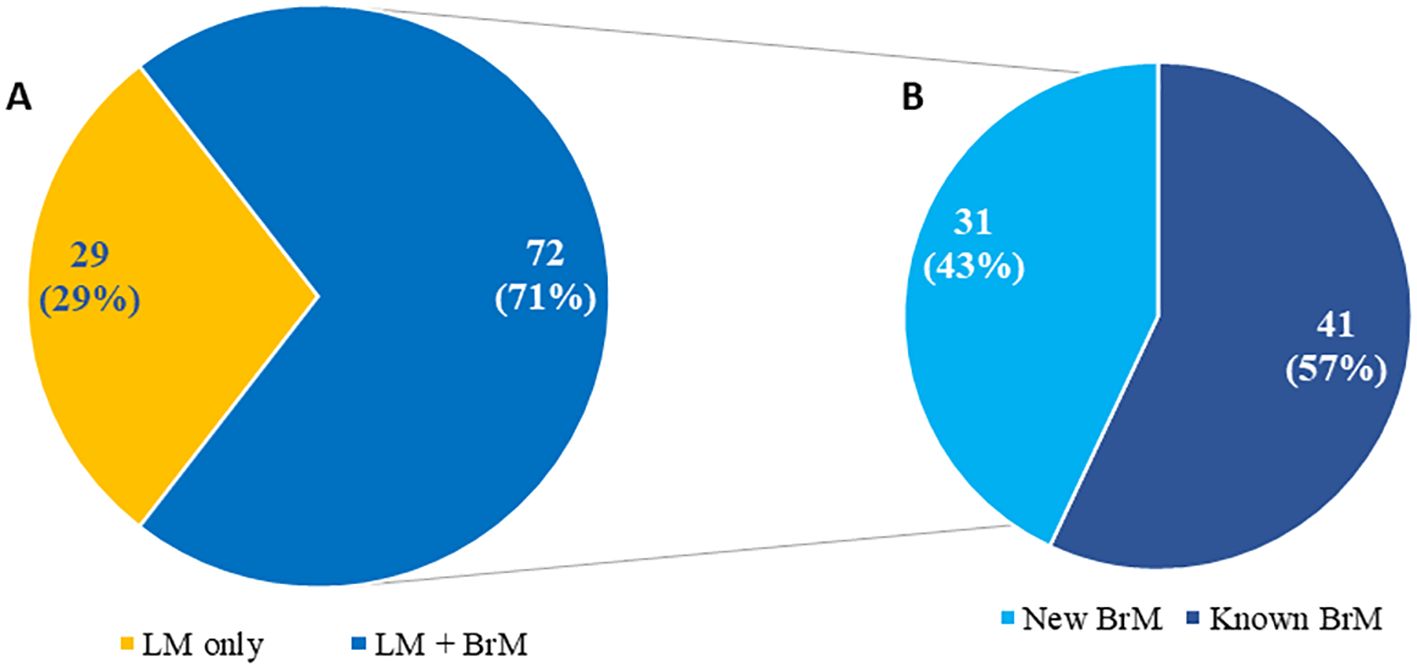
Figure 1. Distribution of patients (N = 101) with newly diagnosed leptomeningeal metastasis based on isolated leptomeningeal metastasis versus leptomeningeal metastasis and brain metastases (A) including time of brain metastases diagnosis (B) Adapted from Diaz’s paper “Quantitative assessment of circulating tumor cells in cerebrospinal fluid as a clinical tool to predict survival in leptomeningeal metastases” at Journal of Neuro-Oncology {Diaz, 2022 #256}.
Diagnostic methods for CNS metastases include neuroimaging, brain tissue diagnosis if surgical resection is feasible and cerebrospinal fluid (CSF) studies for LM diagnosis. However, resection of CNS metastases can be challenging to perform due to the eloquent location of these tumors and may not be indicated when multiple metastases are present. Interestingly, a recent study of resected BrM suggested that sequencing of primary tumors alone might miss molecular aberrations present in 53% of CNS metastases, and various drug resistance mutations could be identified in CSF in about 50% of patients with CNS relapse during various kinase inhibitor therapy. This indicates that a primary tumor biopsy alone might not be sufficient to achieve optimal molecular guidance to treat CNS metastases in advance tumors (15, 16). As systemic therapies improve, patients with cancer have longer survival prospects and higher chances of developing CNS metastases as tumors acquire treatment resistance and relapse/recur over time. Our understanding of the molecular landscape of CNS metastases remains limited at the time of BrM and LM diagnosis, throughout the disease course, and during treatment. Hence, there is a substantial clinical need to discover cerebrospinal fluid (CSF) biomarkers for the diagnosis, prognosis and assessment for treatment response in patients with CNS metastases.
Leptomeningeal metastasis
LM is one of the underdiagnosed complications of cancer despite now being a treatable disease (12, 17–21). Establishing the diagnosis of LM based on standard cerebrospinal fluid (CSF) cytologic analysis or MRI findings is often difficult, particularly at the early stages of leptomeningeal dissemination when treatment interventions could be more effective in limiting spread and triggering additional symptoms. Brain and spine MRIs have the advantage of being non-invasive, but their findings may be nonspecific and equivocal for LM (22). CSF cytology examination is a “gold standard” minimally invasive diagnostic test that provides confirmation of LM but has low diagnostic sensitivity, often requiring multiple lumbar punctures to establish a diagnosis of LM (23–25). The interpretation of CSF cytology results in a dichotomous qualitative variable, positive or negative for malignant cells, and can be challenging if reported as suspicious or atypical. The current EANO/ESMO LM group consensus interprets those results as equivocal (26) and thus, negative results could be false negative as a result of low disease burden in the CNS compartment.
Recent development of new technologies and studies showing the feasibility to identifying tumor- derived cell-free circulating tumor DNA (ctDNA) in CSF and capturing and quantifying CSF circulating tumor cells (CTC) using rare cell capture technologies. This provides a unique opportunity to improve LM diagnosis and to characterize the molecular landscape of the CNS metastases, with the hope that “liquid biopsy” of CSF will be diagnostic, prognostic and therapeutic biomarkers (Figure 2).
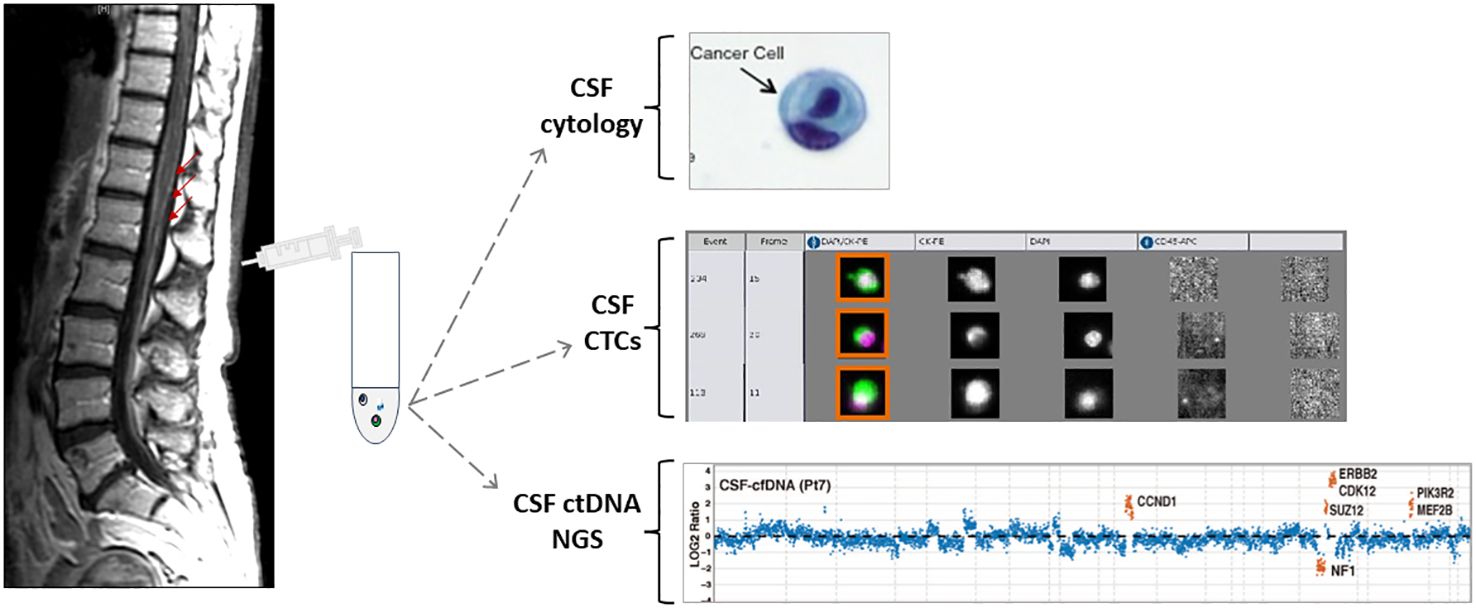
Figure 2. Schematic presentation of MRI of the spine with leptomeningeal metastasis and CSF collection followed by different CSF applications. Adapted from {Pentsova, 2016 #170} {Lin, 2017 #220}.
Quantitative assessment of disease burden in CSF: CSF CTC analysis by rare cell capture technologies
The validated CellSearch system (Menarini Silicon BioSystems), utilizing an immunomagnetic CTC selection method based on an rare cell capture technology (RCCT) and anti-epithelial cell adhesion molecule (EPCAM) antibody-conjugated ferroparticles, is an FDA-approved methodology for enumerating CTC from blood in patients with breast, prostate and colon cancers (27–31). The CellSearch system has been deployed to evaluate CSF-CTCs in patients with LM and has demonstrated potential as a diagnostic marker (Figure 3). A recent prospective study enrolled 95 patients with solid tumors (36 patients with breast cancer, 31 with lung cancer, 28 patients with other solid tumors) presenting with neurologic symptoms suspicious for LM or with MRI findings suspicious for LM, and referred them for clinical CSF examination (32). All patients underwent MRI of the brain and/or spine, and CSF cytopathology. Additionally, 3 ml of CSF from the same lumbar puncture were submitted for CSF-CTC analysis. Based on ROC analysis, presence of ≥ 3 CSF-CTC/per 3 ml was defined as the optimal cut-off for diagnosis of LM achieving a sensitivity of 93%, specificity 95%, positive predictive value 90%, negative predictive value 97%. Other studies reported similar findings of sensitivity above 80% and specificity above 95% (33–38). Additionally, several retrospective studies have demonstrated that LM patients with higher CSF-CTC counts at the time of diagnosis would have worse survival rate compared to those with lower counts, indicating a prognostic role for CSF-CTCs (39, 40).
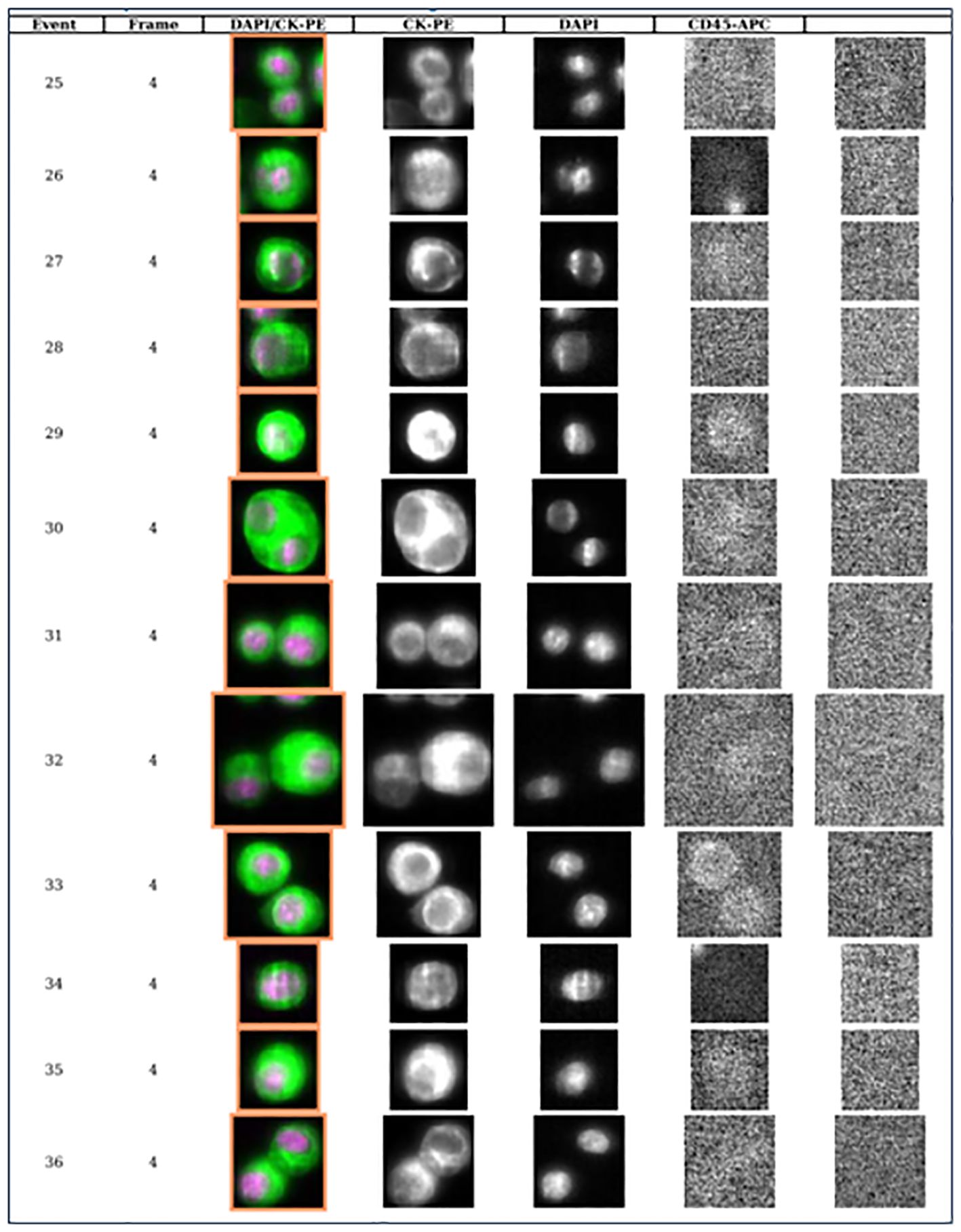
Figure 3. Library of CSF circulating tumor cells from patient with breast cancer and leptomeningeal metastases demonstrating 12 circulating tumor cells per 3 ml of CSF (each row represents one event or one CTC).
The role of CSF CTC analysis in response assessment
Advances in CSF biomarkers analysis allowed to further incorporate the CSF-CTC analysis as an exploratory endpoint in multiple IRB -approved clinical trials (19, 41, 42). For instance, in a phase I/II trial of intrathecal trastuzumab for LM in human epidermal growth factor receptor 2 positive (HER2+) breast carcinoma (42) aimed at characterizing changes in CSF CTCs over time as a potential biomarker of treatment response. Of the 15 patients, 9 had greater than 1 cycle of treatment and were evaluated: a decrease in CSF-CTC count with treatment, and a later increase in CTCs predating radiographic LM progression, were seen in several patients, which led to hypothesis that CSF-CTC analysis may serve as a platform to assess quantitative treatment response and should be further investigated.
The largest prospectively collected case series of 58 patients (41) treated with proton cranio-spinal irradiation (pCSI) reported statistical significance association (HR: 1.05 per 10 cells (95%CI: 1.01–1.10, P = .01) of pre-pCSI CSF-CTCs with CNS progression free survival (PFS) but not associated with overall survival. Moreover, in 31/58 patients with both a pre- and post-pCSI, no increase in CSF-CTCs was observed immediately post-pCSI. It is probably too early to conclude whether CSF-CTC results should influence the decision to discontinue treatment as the relationship between number of CSF-CTCs and radiographic disease CNS burden needs to be investigated further.
Currently the RCCT immunomagnetic platform for enumerating CTC in CSF could be available in a few but not all highly specialized cancer centers, complicating the widespread implementation of this approach, and the validation of its clinical utility across other institutions in the US and worldwide. Other limitations of the CellSearch platform are that it only detects cells from tumors of epithelial origin and have limited ability to provide comprehensive genomic characteristics of the cells. Thus far genomic sequencing of isolated CSF-CTCs has been achieved in patients with LM from breast cancer expressing human epidermal growth factor receptor 2 (HER2) and demonstrated similarities between CSF-CTCs and tumor cells from primary site in addition to several genetic alterations private to CSF-CTC (38, 42–44).
Clinical significance of CSF ctDNA as a diagnostic, prognostic and treatment response biomarkers
Detection of ctDNA in plasma has showed promising potential as a source of “liquid biopsy” to reflect extracranial treatment response and outcomes in a variety of cancers (45–47). However recent studies have shown that plasma ctDNA may not accurately reflect intracranial disease burden in patients with BM (48, 49). Less than a decade ago several studies showed the feasibility of CSF cfDNA sequencing (16, 48–50). Several retrospective studies have demonstrated a positivity rate of CSF ctDNA consistently above 50% for patients with primary or metastatic brain tumors, including detection of tumor-associated mutations in the CSF of 63% of patients with brain metastases (16); in patients with glioma, CSF ctDNA can be found at a much higher positivity rate than plasma and correlates with prognosis (51, 52). CSF is relatively acellular and has direct anatomical contact with BM, making it easier to detect ctDNA in this fluid, potentially with a better correlation with prognosis than plasma ctDNA. Since than many groups reported results which suggested that CSF sequencing may offer a window into the identification of drug resistance mechanisms in patients whose primary tumor responded to genotype-directed targeted cancer therapy but then developed tumor progression in the CNS (Table 1). Additional work is needed to understand to what extent CSF cfDNA reflects the genetic alterations of the primary tumor and/or CNS metastases as a small study showed that some paired BM specimens had unique alterations in TP53 and KRAS, but there were notably very few unique mutations in the CSF specimens (65). Based on current data many questions need to be answered in a clinical setting: how to use CSF ctDNA to monitoring genetic evolution of the tumor in response to treatment, what the role of clonal and subclonal mutation in the CSF is, and whether CSF ctDNA presence or absence, or the percentage of variant allele frequency (VAL) in the sample are the marker of response and correlation with survival and might thus be clinically useful as a “surrogate” source of tumor-derived DNA.
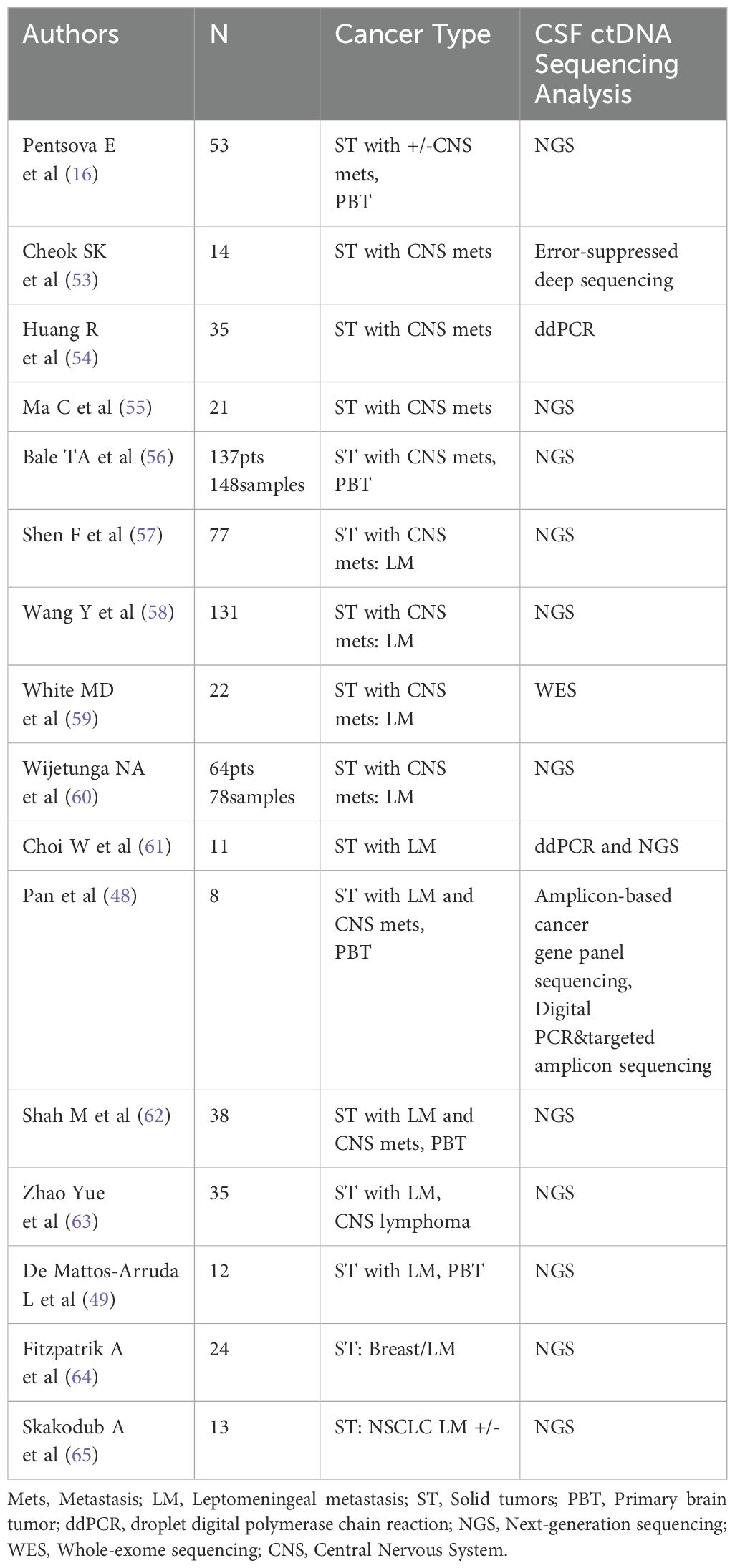
Table 1. Studies analyzing CSF ctDNA in patients with solid tumors with and without brain and leptomeningeal metastases (Updated: July 2024).
Molecular profiling of CSF in patients with brain tumors to identify potential micro RNAs (miR) associated to brain or leptomeningeal dissemination is of great interest, as it could provide a tool to predict and anticipate metastatic disease. Due to technical limitations in discerning tumor and immune cell miR profiles due to the rarity of CTCs, results are heterogeneous and require cautious interpretation. Despite heterogeneous findings, some studies report associations of specific miRs found in the CSF with the presence of brain tumors. An early study in patients with primary and metastatic brain tumors found, using a candidate approach, that miR10b, miR-21 and miR-200 were overexpressed in samples from patients with primary and metastatic brain tumors compared to controls{Teplyuk, 2012 #299}. Follow-up studies conducting miR profiling found differential expression of miR-30e, miR-140, let-7b, mR-10a and miR-21-3p in patients with brain tumors in a cohort of 175 patients that included 13 patients with metastasis; these miRs were validated in an external cohort of 105 patients {Kopkova, 2019 #298}. More recent approaches study miR signatures, which could be more informative as they aggregate global miR profile changes; in a cohort of 65 patients (including 11 patients with LM and 6 patients with BM), miR-335-5p and miR-34b-3p were linked to brain metastasis{Im, 2021 #297}. Although provocative, these studies are limited by the small number of patients, and most of them are not tested in prospective settings; besides these low numbers, these cohorts are heterogeneous as metastases from different types of primary tumors are often grouped to compensate the small cohort samples in granular analyses. Thus, future studies in this line should focus on larger, homogeneous cohorts and incorporate to the extent possible prospective testing of miR profiles.
Paralleling the advances in plasma and other non-CNS bodily fluids, considerable progress has been achieved after almost a decade of CSF-based liquid biopsy: from its conceptual inception and feasibility to its clinical validation, including the potential to influence clinical trial design, several roadblocks have been cleared out regarding the utility of CSF liquid biopsy for CNS disease. There are a few additional questions that must be answered to better understand the prognostic implications of detectable CTCs and ctDNA in CSF, the role of the molecular profile of CSF cfDNA from patients with CNS metastases and its correlation with the molecular profile of blood cfDNA/primary tumors or other sites of metastases to identify mutations or clonal populations that may predispose to CNS metastases. Most prominently, randomized prospective studies are sorely needed to provide sufficient evidence of feasibility in clinical pipelines, and a thorough benchmarking of molecular profiling methods (including cell detection and capture methods) has to be conducted to circumvent technical limitations of these approaches.
Discussion
The advent of increasingly effective diagnostic procedure and treatments across cancer types has resulted in overall improved clinical outcomes for primary tumors, but CNS metastases are still a common feature for most advanced solid tumors, for which scanty treatment options are currently available. The treatments for CNS metastases often are assigned based on the molecular profiles of the primary tumor, and monitoring tumor responses remains a challenge beyond imaging, given that repeated biopsy is not always feasible or safe for these patients. This particularity of CNS metastasis severely limits the development of experimental approaches, for which response assessments are challenging. Besides, patients with CNS metastases are often excluded from trials because of severe neurological deficits.
In contrast to most other solid tumors, traditional liquid biopsy approaches using plasma have been largely ineffective for CNS-disseminated disease, due to the limited release of cellular and molecular tumor components across the brain-blood barrier towards the main circulatory system. Thus, CSF-based liquid biopsies have profoundly revolutionized the ways in which we can access to these types of clinically relevant information in a minimally invasive manner.
The development of CSF biomarkers may allow us to diagnose CNS metastases earlier and find why CNS disease does not respond to treatment at the time of recurrence, thus increasing the chance patients can participate in clinical trials.
As these methods continue to develop with increasing resolution, sensitivity, and specificity, data integration with orthogonal methods (such as electronic health records, advanced imaging, patient-reported outcomes, and computational methods) will increase the robustness of CSF liquid biopsy as a useful biomarker that provide unique clinical and molecular information.
Author contributions
EP: Writing – original draft, Writing – review & editing.
Funding
The author(s) declare financial support was received for the research, authorship, and/or publication of this article. This research was supported by grants from the Memorial Sloan Kettering Brain Tumor Center and the National Institutes of Health (P30-CA008748).
Acknowledgments
I thank Miguel Foronda Alvaro for his editorial assistance.
Conflict of interest
The authors declares that the research was conducted in the absence of any commercial or financial relationships that could be construed as a potential conflict of interest.
Publisher’s note
All claims expressed in this article are solely those of the authors and do not necessarily represent those of their affiliated organizations, or those of the publisher, the editors and the reviewers. Any product that may be evaluated in this article, or claim that may be made by its manufacturer, is not guaranteed or endorsed by the publisher.
References
1. Tabouret E, Chinot O, Metellus P, Tallet A, Viens P, Gonçalves A. Recent trends in epidemiology of brain metastases: an overview. Anticancer Res. (2012) 32:4655–62.
2. Nieder C, Spanne O, Mehta MP, Grosu AL, Geinitz H. Presentation, patterns of care, and survival in patients with brain metastases: what has changed in the last 20 years? Cancer. (2011) 117:2505–12. doi: 10.1002/cncr.v117.11
3. Cagney DN, Martin AM, Catalano PJ, Redig AJ, Lin NU, Lee EQ, et al. Incidence and prognosis of patients with brain metastases at diagnosis of systemic Malignancy: a population-based study. Neuro Oncol. (2017) 19:1511–21. doi: 10.1093/neuonc/nox077
4. Davis FG, Dolecek TA, McCarthy BJ, Villano JL. Toward determining the lifetime occurrence of metastatic brain tumors estimated from 2007 United States cancer incidence data. Neuro Oncol. (2012) 14:1171–7. doi: 10.1093/neuonc/nos152
5. Schouten LJ, Rutten J, Huveneers HA. Twijnstra A: Incidence of brain metastases in a cohort of patients with carcinoma of the breast, colon, kidney, and lung and melanoma. Cancer. (2002) 94:2698–705. doi: 10.1002/cncr.v94:10
6. Li BT, Lou E, Hsu M, Yu HA, Naidoo J, Zauderer MG, et al. Serum biomarkers associated with clinical outcomes fail to predict brain metastases in patients with stage IV non-small cell lung cancers. PloS One. (2016) 11:e0146063. doi: 10.1371/journal.pone.0146063
7. Villano JL, Durbin EB, Normandeau C, Thakkar JP, Moirangthem V, Davis FG. Incidence of brain metastasis at initial presentation of lung cancer. Neuro Oncol. (2015) 17:122–8. doi: 10.1093/neuonc/nou099
8. Aizer AA, Lamba N, Ahluwalia MS, Aldape K, Boire A, Brastianos PK, et al. Brain metastases: A Society for Neuro-Oncology (SNO) consensus review on current management and future directions. Neuro Oncol. (2022) 24:1613–46. doi: 10.1093/neuonc/noac118
9. Barnholtz-Sloan JS, Sloan AE, Davis FG, Vigneau FD, Lai P, Sawaya RE. Incidence proportions of brain metastases in patients diagnosed (1973 to 2001) in the Metropolitan Detroit Cancer Surveillance System. J Clin Oncol. (2004) 22:2865–72. doi: 10.1200/JCO.2004.12.149
10. Sperduto PW, Chao ST, Sneed PK, Luo X, Suh J, Roberge D, et al. Diagnosis-specific prognostic factors, indexes, and treatment outcomes for patients with newly diagnosed brain metastases: a multi-institutional analysis of 4,259 patients. Int J Radiat Oncol Biol Phys. (2010) 77:655–61. doi: 10.1016/j.ijrobp.2009.08.025
11. Berghoff AS, Schur S, Fureder LM, Gatterbauer B, Dieckmann K, Widhalm G, et al. Descriptive statistical analysis of a real life cohort of 2419 patients with brain metastases of solid cancers. ESMO Open. (2016) 1:e000024. doi: 10.1136/esmoopen-2015-000024
12. Wilcox JA, Chukwueke UN, Ahn MJ, Aizer AA, Bale TA, Brandsma D, et al. Leptomeningeal metastases from solid tumors: A SNO and ASCO consensus review on clinical management and future directions. Neuro Oncol. (2024). doi: 10.1093/neuonc/noae103
13. Diaz M, Singh P, Kotchetkov IS, Skakodub A, Meng A, Tamer C, et al. Quantitative assessment of circulating tumor cells in cerebrospinal fluid as a clinical tool to predict survival in leptomeningeal metastases. J Neurooncol. (2022) 157:81–90. doi: 10.1007/s11060-022-03949-1
14. Morikawa A, Jordan L, Rozner R, Patil S, Boire A, Pentsova E, et al. Characteristics and outcomes of patients with breast cancer with leptomeningeal metastasis. Clin Breast Cancer. (2016). doi: 10.1016/j.clbc.2016.07.002
15. Brastianos PK, Carter SL, Santagata S, Cahill DP, Taylor-Weiner A, Jones RT, et al. Genomic characterization of brain metastases reveals branched evolution and potential therapeutic targets. Cancer Discov. (2015). doi: 10.1158/2159-8290.CD-15-0369
16. Pentsova EI, Shah RH, Tang J, Boire A, You D, Briggs S, et al. Evaluating cancer of the central nervous system through next-generation sequencing of cerebrospinal fluid. J Clin Oncol. (2016) 34:2404–15. doi: 10.1200/JCO.2016.66.6487
17. Nanjo S, Hata A, Okuda C, Kaji R, Okada H, Tamura D, et al. Standard-dose osimertinib for refractory leptomeningeal metastases in T790M-positive EGFR-mutant non-small cell lung cancer. Br J Cancer. (2018) 118:32–7. doi: 10.1038/bjc.2017.394
18. Park S, Lee MH, Seong M, Kim ST, Kang JH, Cho BC, et al. A phase II, multicenter, two cohort study of 160 mg osimertinib in EGFR T790M-positive non-small-cell lung cancer patients with brain metastases or leptomeningeal disease who progressed on prior EGFR TKI therapy. Ann Oncol. (2020) 31:1397–404. doi: 10.1016/j.annonc.2020.06.017
19. Morikawa A, de StanChina E, Pentsova E, Kemeny MM, Li BT, Tang K, et al. Phase I study of intermittent high-dose lapatinib alternating with capecitabine for HER2-positive breast cancer patients with central nervous system metastases. Clin Cancer Res. (2019) 25:3784–92. doi: 10.1158/1078-0432.CCR-18-3502
20. Bauer TM, Shaw AT, Johnson ML, Navarro A, Gainor JF, Thurm H, et al. Brain penetration of lorlatinib: cumulative incidences of CNS and non-CNS progression with lorlatinib in patients with previously treated ALK-positive non-small-cell lung cancer. Target Oncol. (2020) 15:55–65. doi: 10.1007/s11523-020-00702-4
21. Yang TJ, Wijetunga NA, Yamada J, Wolden S, Mehallow M, Goldman DA, et al. Clinical trial of proton craniospinal irradiation for leptomeningeal metastases. Neuro Oncol. (2021) 23:134–43. doi: 10.1093/neuonc/noaa152
22. Clarke JL, Perez HR, Jacks LM, Panageas KS, Deangelis LM. Leptomeningeal metastases in the MRI era. Neurology. (2010) 74:1449–54. doi: 10.1212/WNL.0b013e3181dc1a69
23. Pauls S, Fischer AC, Brambs HJ, Fetscher S, Höche W, Bommer M. Use of magnetic resonance imaging to detect neoplastic meningitis: limited use in leukemia and lymphoma but convincing results in solid tumors. Eur J Radiol. (2012) 81:974–8. doi: 10.1016/j.ejrad.2011.02.020
24. Wasserstrom WR, Glass JP, Posner JB. Diagnosis and treatment of leptomeningeal metastases from solid tumors: experience with 90 patients. Cancer. (1982) 49:759–72. doi: 10.1002/1097-0142(19820215)49:4<759::AID-CNCR2820490427>3.0.CO;2-7
25. Glantz MJ, Cole BF, Glantz LK, Cobb J, Mills P, Lekos A, et al. Cerebrospinal fluid cytology in patients with cancer: minimizing false-negative results. Cancer. (1998) 82:733–9. doi: 10.1002/(SICI)1097-0142(19980215)82:4<733::AID-CNCR17>3.0.CO;2-Z
26. Le Rhun E, Weller M, van den Bent M, Brandsma D, Furtner J, Rudà R, et al. Leptomeningeal metastasis from solid tumours: EANO-ESMO Clinical Practice Guideline for diagnosis, treatment and follow-up. ESMO Open. (2023) 8:101624. doi: 10.1016/j.esmoop.2023.101624
27. Scher HI, Jia X, de Bono JS, Fleisher M, Pienta KJ, Raghavan D, et al. Circulating tumour cells as prognostic markers in progressive, castration-resistant prostate cancer: a reanalysis of IMMC38 trial data. Lancet Oncol. (2009) 10:233–9. doi: 10.1016/S1470-2045(08)70340-1
28. Went PT, Lugli A, Meier S, Bundi M, Mirlacher M, Sauter G, et al. Frequent EpCam protein expression in human carcinomas. Hum Pathol. (2004) 35:122–8. doi: 10.1016/j.humpath.2003.08.026
29. Cristofanilli M, Budd GT, Ellis MJ, Stopeck A, Matera J, Miller MC, et al. Circulating tumor cells, disease progression, and survival in metastatic breast cancer. N Engl J Med. (2004) 351:781–91. doi: 10.1056/NEJMoa040766
30. Liu MC, Shields PG, Warren RD, Cohen P, Wilkinson M, Ottaviano YL, et al. Circulating tumor cells: a useful predictor of treatment efficacy in metastatic breast cancer. J Clin Oncol. (2009) 27:5153–9. doi: 10.1200/JCO.2008.20.6664
31. Allard WJ, Matera J, Miller MC, Repollet M, Connelly MC, Rao C, et al. Tumor cells circulate in the peripheral blood of all major carcinomas but not in healthy subjects or patients with nonmalignant diseases. Clin Cancer Res. (2004) 10:6897–904. doi: 10.1158/1078-0432.CCR-04-0378
32. Lin X, Fleisher M, Rosenblum M, Lin O, Boire A, Briggs S, et al. Cerebrospinal fluid circulating tumor cells: a novel tool to diagnose leptomeningeal metastases from epithelial tumors. Neuro Oncol. (2017) 19:1248–54. doi: 10.1093/neuonc/nox066
33. Nayak L, Fleisher M, Gonzalez-Espinoza R, Lin O, Panageas K, Reiner A, et al. Rare cell capture technology for the diagnosis of leptomeningeal metastasis in solid tumors. Neurology. (2013) 80:1598–605;discussion 1603. doi: 10.1212/WNL.0b013e31828f183f
34. Lee JS, Melisko ME, Magbanua MJ, Kablanian AT, Scott JH, Rugo HS, et al. Detection of cerebrospinal fluid tumor cells and its clinical relevance in leptomeningeal metastasis of breast cancer. Breast Cancer Res Treat. (2015) 154:339–49. doi: 10.1007/s10549-015-3610-1
35. Tu Q, Wu X, Le Rhun E, Blonski M, Wittwer B, Taillandier L, et al. CellSearch technology applied to the detection and quantification of tumor cells in CSF of patients with lung cancer leptomeningeal metastasis. Lung Cancer. (2015) 90:352–7. doi: 10.1016/j.lungcan.2015.09.008
36. Torre M, Lee EQ, Chukwueke UN, Nayak L, Cibas ES, Lowe AC. Integration of rare cell capture technology into cytologic evaluation of cerebrospinal fluid specimens from patients with solid tumors and suspected leptomeningeal metastasis. J Am Soc Cytopathol. (2020) 9:45–54. doi: 10.1016/j.jasc.2019.09.001
37. van Bussel MTJ, Pluim D, Milojkovic Kerklaan B, Bol M, Sikorska K, Linders DTC, et al. Circulating epithelial tumor cell analysis in CSF in patients with leptomeningeal metastases. Neurology. (2020) 94:e521–8. doi: 10.1212/WNL.0000000000008751
38. Darlix A, Cayrefourcq L, Pouderoux S, Menjot de Champfleur N, Bievelez A, Jacot W, et al. Detection of circulating tumor cells in cerebrospinal fluid of patients with suspected breast cancer leptomeningeal metastases: A prospective study. Clin Chem. (2022) 68:1311–22. doi: 10.1093/clinchem/hvac127
39. Nevel KS, DiStefano N, Lin X, Skakodub A, Ogilvie SQ, Reiner AS, et al. A retrospective, quantitative assessment of disease burden in patients with leptomeningeal metastases from non-small-cell lung cancer. Neuro Oncol. (2020) 22:675–83. doi: 10.1093/neuonc/noz208
40. Diaz M, Singh P, Kotchetkov I, Skakodub A, Reiner A, Panageas K, et al. Biom-05. Circulating tumor cells (ctc) in cerebrospinal fluid (csf) as a predictor of survival in cns metastases. Neuro-Oncology. (2020) 22:ii2–2.
41. Wijetunga NA, Boire A, Young RJ, Yamada Y, Wolden S, Yu H, et al. Quantitative cerebrospinal fluid circulating tumor cells are a potential biomarker of response for proton craniospinal irradiation for leptomeningeal metastasis. Neurooncol Adv. (2021) 3:vdab181. doi: 10.1093/noajnl/vdab181
42. Malani R, Fleisher M, Kumthekar P, Lin X, Omuro A, Groves MD, et al. Cerebrospinal fluid circulating tumor cells as a quantifiable measurement of leptomeningeal metastases in patients with HER2 positive cancer. J Neurooncol. (2020) 148:599–606. doi: 10.1007/s11060-020-03555-z
43. Magbanua MJ, Melisko M, Roy R, Sosa EV, Hauranieh L, Kablanian A, et al. Molecular profiling of tumor cells in cerebrospinal fluid and matched primary tumors from metastatic breast cancer patients with leptomeningeal carcinomatosis. Cancer Res. (2013) 73:7134–43. doi: 10.1158/0008-5472.CAN-13-2051
44. Li X, Zhang Y, Ding J, Wang M, Li N, Yang H, et al. Clinical significance of detecting CSF-derived tumor cells in breast cancer patients with leptomeningeal metastasis. Oncotarget. (2018) 9:2705–14. doi: 10.18632/oncotarget.23597
45. Yu HA, Arcila ME, Rekhtman N, Sima CS, Zakowski MF, Pao W, et al. Analysis of tumor specimens at the time of acquired resistance to EGFR-TKI therapy in 155 patients with EGFR-mutant lung cancers. Clin Cancer Res. (2013) 19:2240–7. doi: 10.1158/1078-0432.CCR-12-2246
46. Abbosh C, Frankell AM, Harrison T, Kisistok J, Garnett A, Johnson L, et al. Tracking early lung cancer metastatic dissemination in TRACERx using ctDNA. Nature. (2023) 616:553–62. doi: 10.1038/s41586-023-05776-4
47. Jamal-Hanjani M, Wilson GA, McGranahan N, Birkbak NJ, Watkins TBK, Veeriah S, et al. Tracking the evolution of non-small-cell lung cancer. N Engl J Med. (2017) 376:2109–21. doi: 10.1056/NEJMoa1616288
48. Pan W, Gu W, Nagpal S, Gephart MH, Quake SR. Brain tumor mutations detected in cerebral spinal fluid. Clin Chem. (2015) 61:514–22. doi: 10.1373/clinchem.2014.235457
49. De Mattos-Arruda L, Mayor R, Ng CK, Weigelt B, Martínez-Ricarte F, Torrejon D, et al. Cerebrospinal fluid-derived circulating tumour DNA better represents the genomic alterations of brain tumours than plasma. Nat Commun. (2015) 6:8839. doi: 10.1038/ncomms9839
50. Wang Y, Springer S, Zhang M, McMahon KW, Kinde I, Dobbyn L, et al. Detection of tumor-derived DNA in cerebrospinal fluid of patients with primary tumors of the brain and spinal cord. Proc Natl Acad Sci U.S.A. (2015) 112:9704–9.
51. Miller AM, Shah RH, Pentsova EI, Pourmaleki M, Briggs S, Distefano N, et al. Tracking tumour evolution in glioma through liquid biopsies of cerebrospinal fluid. Nature. (2019) 565:654–8. doi: 10.1038/s41586-019-0882-3
52. Miller AM, Szalontay L, Bouvier N, Hill K, Ahmad H, Rafailov J, et al. Next-generation sequencing of cerebrospinal fluid for clinical molecular diagnostics in pediatric, adolescent and young adult brain tumor patients. Neuro Oncol. (2022) 24:1763–72. doi: 10.1093/neuonc/noac035
53. Cheok SK, Narayan A, Arnal-Estape A, Gettinger S, Goldberg SB, Kluger HM, et al. Tumor DNA mutations from intraparenchymal brain metastases are detectable in CSF. JCO Precis Oncol. (2021) 5. doi: 10.1200/PO.20.00292
54. Huang R, Xu X, Li D, Chen K, Zhan Q, Ge M, et al. Digital PCR-based detection of EGFR mutations in paired plasma and CSF samples of lung adenocarcinoma patients with central nervous system metastases. Target Oncol. (2019) 14:343–50. doi: 10.1007/s11523-019-00645-5
55. Ma C, Yang X, Xing W, Yu H, Si T, Guo Z. Detection of circulating tumor DNA from non-small cell lung cancer brain metastasis in cerebrospinal fluid samples. Thorac Cancer. (2020) 11:588–93. doi: 10.1111/1759-7714.13300
56. Bale TA, Yang SR, Solomon JP, Nafa K, Middha S, Casanova J, et al. Clinical experience of cerebrospinal fluid-based liquid biopsy demonstrates superiority of cell-free DNA over cell pellet genomic DNA for molecular profiling. J Mol Diagn. (2021) 23:742–52. doi: 10.1016/j.jmoldx.2021.03.001
57. Shen F, Liang N, Fan Z, Zhao M, Kang J, Wang X, et al. Genomic alterations identification and resistance mechanisms exploration of NSCLC with central nervous system metastases using liquid biopsy of cerebrospinal fluid: A real-world study. Front Oncol. (2022) 12:889591. doi: 10.3389/fonc.2022.889591
58. Wang Y, Jiang F, Xia R, Li M, Yao C, Li Y, et al. Unique genomic alterations of cerebrospinal fluid cell-free DNA are critical for targeted therapy of non-small cell lung cancer with leptomeningeal metastasis. Front Oncol. (2021) 11:701171. doi: 10.3389/fonc.2021.701171
59. White MD, Klein RH, Shaw B, Kim A, Subramanian M, Mora JL, et al. Detection of leptomeningeal disease using cell-free DNA from cerebrospinal fluid. JAMA Netw Open. (2021) 4:e2120040. doi: 10.1001/jamanetworkopen.2021.20040
60. Wijetunga NA, Goglia AG, Weinhold N, Berger MF, Cislo M, Higginson DS, et al. Dynamic mutational landscape of cerebrospinal fluid circulating tumor DNA and predictors of survival after proton craniospinal irradiation for leptomeningeal metastases. Clin Cancer Res. (2023) 29:775–83. doi: 10.1158/1078-0432.CCR-22-2434
61. Choi W, Cho Y, Park SY, Hwang KH, Han JY, Lee Y. A nanowire-based liquid biopsy method using cerebrospinal fluid cell-free DNA for targeted management of leptomeningeal carcinomatosis. J Cancer Res Clin Oncol. (2021) 147:213–22. doi: 10.1007/s00432-020-03324-5
62. Shah M, Takayasu T, Zorofchian Moghadamtousi S, Arevalo O, Chen M. Evaluation of the oncomine pan-cancer cell-free assay for analyzing circulating tumor DNA in the cerebrospinal fluid in patients with central nervous system Malignancies. J Mol Diagn. (2021) 23:171–80. doi: 10.1016/j.jmoldx.2020.10.013
63. Zhao Y, He JY, Zou YL, Guo XS, Cui JZ, Guo L, et al. Evaluating the cerebrospinal fluid ctDNA detection by next-generation sequencing in the diagnosis of meningeal Carcinomatosis. BMC Neurol. (2019) 19:331. doi: 10.1186/s12883-019-1554-5
64. Fitzpatrick A, Iravani M, Mills A, Childs L, Alaguthurai T, Clifford A, et al. Assessing CSF ctDNA to improve diagnostic accuracy and therapeutic monitoring in breast cancer leptomeningeal metastasis. Clin Cancer Res. (2022) 28:1180–91. doi: 10.1158/1078-0432.CCR-21-3017
Keywords: brain metastasis, leptomeningeal metastasis, liquid biopsy, cerebrospinal fluid, biomarkers
Citation: Pentsova E (2024) Applications of cerebrospinal fluid circulating tumor cells and circulating tumor-derived DNA in diagnosis, prognosis, and personalized treatment of CNS metastases. Front. Oncol. 14:1409383. doi: 10.3389/fonc.2024.1409383
Received: 29 March 2024; Accepted: 05 September 2024;
Published: 30 September 2024.
Edited by:
Takeshi Kondoh, Kobe University, JapanReviewed by:
Serena Bonin, University of Trieste, ItalySunny Malhotra, Vall d’Hebron University Hospital, Spain
Copyright © 2024 Pentsova. This is an open-access article distributed under the terms of the Creative Commons Attribution License (CC BY). The use, distribution or reproduction in other forums is permitted, provided the original author(s) and the copyright owner(s) are credited and that the original publication in this journal is cited, in accordance with accepted academic practice. No use, distribution or reproduction is permitted which does not comply with these terms.
*Correspondence: Elena Pentsova, cGVudHNvdmVAbXNrY2Mub3Jn