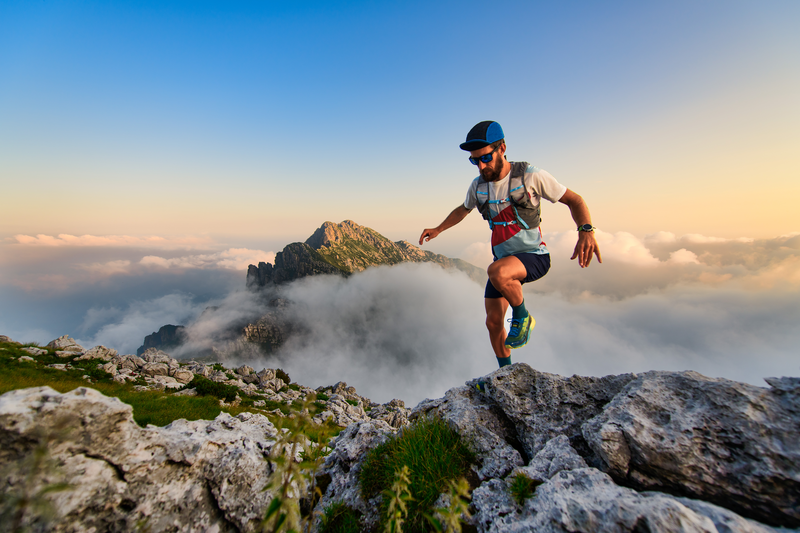
94% of researchers rate our articles as excellent or good
Learn more about the work of our research integrity team to safeguard the quality of each article we publish.
Find out more
PERSPECTIVE article
Front. Oncol. , 18 January 2024
Sec. Cancer Epidemiology and Prevention
Volume 13 - 2023 | https://doi.org/10.3389/fonc.2023.1304805
This article is part of the Research Topic Childhood Leukemias in Latin America: Epidemiology, Causality, Novel Predictive Profiles and Therapeutic Strategies View all 22 articles
Leukemias are the most common type of pediatric cancer around the world. Prognosis has improved during the last decades, and many patients are cured with conventional treatment as chemotherapy; however, many patients still present with a refractory disease requiring additional treatments, including hematopoietic stem cell transplantation. Immunotherapy with monoclonal antibodies or cellular therapy is a promising strategy for treating refractory or relapsed hematological malignancies. Particularly, CAR-T cells have shown clinical efficacy in clinical trials, and different products are now commercially approved by regulatory agencies in the USA and Europe. Many challenges still need to be solved to improve and optimize the potential of these therapies worldwide. Global access to cell therapy is a significant concern, and different strategies are being explored in the middle- and low-income countries. In Mexico, leukemias represent around 50% of total cancer diagnosed in pediatric patients, and the rate of relapsed or refractory disease is higher than reported in other countries, a multi-factorial problem. Although significant progress has been made during the last decades in leukemia diagnosis and treatment, making new therapies available to Mexican patients is a priority, and cell and gene therapies are on the horizon. Efforts are ongoing to make CAR-T cell therapy accessible for patients in Mexico. This article summarizes a general landscape of childhood leukemias in Mexico, and we give a perspective about the current strategies, advances, and challenges ahead to make gene and cell therapies for leukemia clinically available.
Leukemia is the most common cancer in children, its diagnosis and treatment have favorably changed in the last 50 years. Many factors contribute to a better prognosis, including improvements in diagnosis, biologic classification, and the development of new therapeutics, causing overall survival rates to improve from 30% in the 1960s to over 80% in this decade in developed countries. Low-risk pediatric leukemias currently have a survival of 95% in the ideal scenario, although refractory and relapsed leukemia still cause high mortality and disease-burden (1). Notable is the emerging field of cellular immunotherapy, where Chimeric Antigen Receptor (CAR) T cells show encouraging results in hematologic malignancies (2).
Access to new diagnostic and therapeutic options is not equally available worldwide. In low- and middle-income countries (LMIC) like Mexico many challenges need to be addressed in different areas, including clinical care, basic and clinical research, education, funding, and regulation to allow access to more cell and gene therapies.
In this paper, we describe some important aspects of the past and current healthcare status for pediatric leukemias in Mexico, review the basic and clinical aspects of CAR-T cell therapy, and discuss the challenges to make this therapy available.
Leukemias account for around 50% of newly diagnosed cancer in children (3, 4). Acute lymphoblastic leukemia (ALL) is the most common type of childhood leukemia (83%), followed by acute myeloid leukemias (13.1%), chronic myelocytic leukemia (2.8%), and myelodysplastic syndromes (1%) (5). Between 1998-2018, the proportion of leukemias as a cause of pediatric deaths increased from 0.3% to 1.3% (6). It represents a public health problem with socio-economic and psychological impact on patients and families. In general, the mortality rate is higher when compared with developed countries (3).
Mexico has a population of 130 million, the healthcare services are diverse. Health insurance is limited, and costly procedures like cancer treatment and HSCT are challenging to fund. The IMSS (Instituto Mexicano del Seguro Social) provides healthcare to the formally employed citizens, the ISSSTE (Instituto de Seguridad y Servicio Sociales de los Trabajadores del Estado) to the bureaucrats, and the SSA (Secretaría de Salud) covers population without a formal employment. Less than 7% of Mexicans have Private healthcare (7). Currently, there are certified pediatric oncologists and certified centers in every State of the Country. However, disparities in access to diagnostic and therapeutic procedures exist among centers and public and private hospitals (3). Nine centers are accredited to perform HSCT in pediatric patients distributed in Mexico City, Puebla, Guadalajara, and Monterrey (8).
In Mexico, the poor prognosis of children with ALL is multifactorial including biological and sociocultural factors. Pivotal studies in Mexico identified nutritional problems as a significant adverse prognostic factor for patients with leukemia (9). It has been reported that these two factors were not significant for early mortality, but they condition a higher risk of infections during treatment, leading to increased overall mortality (10). Currently, nutritional interventions must be part of the clinical care for all children with leukemia.
Before 2005, pediatric cancer was not part of Mexico’s National Health Program. The introduction of a National Health Program in 2005 provided specific financial coverage for leukemia treatment and national treatment protocols based on risk stratification, leading to improved prognosis. It also allowed broader access to hematopoietic stem cell transplantation (HSCT) for relapsed and refractory leukemias, increasing survival from 16% to 50% (11, 12). A retrospective analysis for pediatric ALL treated between 2005 and 2015 showed an estimated overall 5-year survival rate of 61.8%; the annual number of treated children doubled from 535 in 2005 to 1,070 in 2015 (13). Several factors contribute to the low survival rates for pediatric ALL in Mexico, including specific molecular epidemiology, late diagnosis, low precision diagnostic methods, limited access to treatment, therapy-related toxicity, exposure to environmental risk factors, high frequency of early relapse, lack of collaborative work, insufficient involvement in clinical trials, and lack of continuous medical education (14–16).
Significant effort has taken place to improve the diagnosis and treatment of pediatric leukemias. In 2016, a collaborative program with St. Jude Hospital (USA) started, seeking to develop multi-site protocols for clinical care. An initial retrospective evaluation showed that 82% of patients were classified as high-risk leukemia at diagnosis and that tests for minimal residual disease or molecular classification were unavailable on 28% of patients, highlighting challenges for evaluation and classification at diagnosis (17). More than 57 centers are now enrolled in this initiative, and a National Program for Pediatric Leukemia Treatment (MAS-ALL18 AMG) exists for the first-line treatment protocols (18). Other initiatives, such as ONCOCREAN, are taking place to improve regional clinical care and access to more precise diagnosis and classification methods. HSCT programs are available in many parts of the country, and now is considered a standard of care in refractory/relapsed ALL. Although access to new therapies and clinical trials improves slowly, there are positive developments. In a recent study that included 41 Mexican patients with CD19+ ALL treated with blinatumomab, 78% showed a complete response (Unpublished). Additionally, 63% of them received haploidentical HSCT after blinatumomab, with a significant improvement in survival compared to conventional chemotherapy (19).
The National Council for Humanity, Science and Technology (CONAHCYT) launched an initiative in 2020 to fund basic and clinical research to improve the prognosis of pediatric patients with leukemia (PRONACES SALUD) and reduce the gap between different regions across the country. We highlight three research areas from this initiative: 1) The national harmonization of flow cytometry tests for diagnosis and follow-up, 2) Improving knowledge about clinical and molecular epidemiology of Mexican patients with leukemia, 3) The development of a clinical program for CAR-T cell therapy (20).
Pediatric leukemia outcomes are sub-optimal in Mexico, especially for relapsed and refractory leukemias. The field is evolving rapidly worldwide and immune effector cell therapy, particularly CAR-T cells, is an area of great interest and opportunity for the upcoming years.
CAR-T cells are genetically engineered T cells expressing a CAR directed against a specific target. In oncology, CAR-T cells are directed against tumoral antigens, enabling the destruction of cancer cells without needing an interaction between T and antigen-presenting cells (21). The CAR design is an essential part of the success of this therapy. CARs combine a monoclonal antibody’s specificity with T cells’ effector functions. CAR-T cells express engineered receptors composed of four main domains: 1) an antigen-binding extracellular domain, which is typically a single-chain variable fragment (scFv) of monoclonal antibody (mAb), 2) a spacer domain that provides flexibility and helps to position the CAR toward the target, 3) a transmembrane domain that contributes to the stability and promotes cytokine release, and 4) an intracellular domain which provides T cell activation and expansion signals (22, 23) (Figure 1A). In addition, to enhance the activity, persistence, and safety of CAR-T cell therapy, different intracellular scaffolds have been designed and currently classified into five generations (Figure 1B) (24–27).
Figure 1 Main structure and evolution of CARs design. (A), The core structure of a CAR highlighting the major components, 1) extracellular domain: antigen-recognition domain, a single-chain fragment variant (scFV), 2) space domain, 3) transmembrane domain, and 4) an Intracellular domain. (B), the CAR evolution is classified into five generations: the first one, only contain the CD3ζ, the second one includes additional costimulatory signaling domains (CD28 or 4–1BB), while, the third-generation combine multiple co-stimulatory domains, such as CD28–41BB or CD28-OX40 and the fifth generation contain an extra intracellular domain. This CAR comprise truncated intracellular domains of cytokine receptors (IL-2Rβ) with a motif for binding transcription factors such as STAT-3/5.
The CAR-T cells have emerged as an effective therapy for treating relapsed and refractory (R/R) B-ALL in children and adults (27). CD19/CAR-T cell infusion treatment induced high complete remission (CR) rates in patients with poor prognosis and few therapeutic options in clinical trials and real-life treatment reports (27, 28). However, this therapy still has significant limitations, such as long manufacturing times, high costs, its autologous nature, and resistance mechanisms that could reduce its effectiveness (25). Despite encouraging responses, more than half of patients will experience a relapse in the long term (29). Consolidation with allogeneic HSCT could improve long-term outcomes (30).
Some approaches to enhance efficacy and avoid resistance to CAR-T cells involve alternative targets, such as CD20 and CD22, and the design of bi- and tri-specific CAR-T cells (31). Additionally, new platforms are developing to shorten the CAR-T cell manufacturing process (32). Another approach to increase the availability of CAR-T therapy involves knocking out the native human leukocyte antigen (HLA) and T-cell receptor (TCR) genes in T cells obtained from healthy donors to avoid compatibility issues, thus enabling the development of universal, off-the-shelf CAR-T cell therapies, which could expand accessibility to a more significant number of patients (25).
By April 2022, there were 2,756 active cell therapy agents in immune-oncology research worldwide, a 36% increase compared to 2021. Remarkably, these therapies have yet to be geographically distributed significantly in recent years, with most clinical trials in the USA and a few European countries (33). Barriers to the wider clinical use of CAR-T cells include costs, patient health status, a limited number of treatment and manufacturing centers, and geographic distance (34).
India recently initiated a phase III clinical trial at a Mumbai Hospital (35, 36) with a strategy to manufacture CAR-T cells on-site, aiming to reduce ten-fold costs compared to commercial products. Brazil has authorized more than 18 clinical trials with Advanced Therapy Products since 2018, and in July 2022, the first CAR-T cell therapy clinical trial was authorized (37, 38). Initiatives for global access to CAR-T cell therapies are ongoing in different parts of the world (39). A Latin American Consortium initiated in collaboration with Caring Cross in 2022, where specialists from Brazil, Argentina, Chile, Mexico, and Colombia regularly meet and discuss advances and challenges for national and regional implementation (40).
CAR-T cell research and clinical use must join a regulatory framework. 2017, the first CAR-T cell therapy received FDA approval (41). Currently, six CAR-T cell products are approved by the FDA (USA)/EMA (Europe) for the treatment of hematologic malignancies (Table 1). Heterogeneity in regulation worldwide adds significant challenges to advancing gene and cell therapy. The FDA considers gene therapy products to be biologics, and the regulatory framework is issued by the Center of Biologics Evaluation and Research (CBER). EMA considers CAR-T cells as cellular products and regulates them under the Advanced Therapy Medicinal Products (ATMP) framework. Both agencies published regulatory guidelines that agree on the importance of controlling the quality of each element involved in CAR-T cell production (42, 43).
World Health Organization (WHO) also published a document recognizing that this type of product requires specific regulation and classifies CAR-T cells as ATMP´s (44).
Regulatory frameworks from the FDA, EMA, and WHO may serve as a basis for establishing regulations worldwide. Regulation must be dynamic to facilitate accessibility while maintaining high-quality standards. Some of the difficulties in Mexico and Latin America in establishing a clear regulatory framework include a) the lack of specific laws or regulatory documents regarding gene and cell therapies, b) the absence of expert committees to evaluate these products and, c) the lack of financial support for the research and development of regulation.
Interest in CAR-T cell therapy has increased in Mexico in the last few years. Currently, there is only one clinical trial, led by the Autonomous University of Nuevo León, approved by the Mexican Regulatory Agency COFEPRIS for treating adults with CD19-positive leukemia or lymphoma. Notably, in early 2023, their group successfully imported a clinical-grade lentiviral vector for the first time in Mexico (45). Our group is developing a clinical trial for treating pediatric leukemia with CD19 CAR-T cells using an on-site semi-automated manufacturing process in a closed system. This manufacturing process has shown previously effective and reproducible cellular and clinical results (46).
The cost of CAR-T cell therapy is currently estimated between US$373,000 and $475,000 per infusion, not including patient care expenses (47). There is a need for cost-effective strategies and efforts to optimize patient access (48).
In resource-limited settings, where many other healthcare problems need to be solved, the cost of CAR-T cell therapies is a significant barrier. Commercial CAR-T cells with centralized manufacturing have very high costs (49). Point-of-care manufacturing reduces costs significantly while maintaining good quality standards. The use of automated CAR-T cell manufacturing equipment has been demonstrated to be feasible in HIC and LMIC (35, 46). A recent study from India demonstrates the feasibility of a decentralized automated process of anti-CD19 CAR-T cells with fulfillment of all standard criteria assays for clinical application at a significantly reduced manufacturing cost of US$35,107 (excluding the cost of the lentiviral vector, which may vary from $5,000 to $28,889 per patient) (35). Real-world data on manufacturing costs in Mexico is lacking, but our estimates are around $30,000 per product. Costs of clinical care, apheresis, management of adverse reactions, and monitoring will add to the final treatment costs.
This high financial burden will need to be analyzed within the healthcare systems and the real impact these therapies will have on the general population (49). It is necessary to use different economic approaches to evaluate these therapies and see the potential long-term benefits (50, 51). For every child who does not survive cancer, there is a loss of 70 productive years for the Society (6). We performed a cost-benefit analysis using the social return on investment method, and we estimate that if 21 pediatric patients with refractory/relapsed leukemia are successfully treated with CAR-T cells, there is a social return of $5.43 for each $1 invested in the funding (unpublished). CAR-T cells may represent a cost-effective option for pediatric R/R B-ALL if a one-year survival above 70% is achieved (52).
In Mexico, a robust regulatory framework is needed. There are issues that require our attention: a) general regulation for the clinical use of genetically modified cells and tissues, b) the regulation for the import/export, use, and manufacture of viral and non-viral vectors for clinical use, c) regulation of the manufacturing process, d) guidelines and regulation of specialized centers for clinical use of cell and gene therapies. In 2023, the Mexican Health Regulatory Agency (COFEPRIS) established for the first time a group of experts in advanced therapies from different fields, including academic, medical, scientific, pharmaceutical, and regulatory areas. The aim is to develop an initial regulatory framework.
Another limiting factor for CAR-T cell therapy is the accessibility clinical-grade viral vectors due to their high cost. In the future, local or regional manufacturing of GMP clinical-grade viral vectors may help to improve accessibility and reduce costs. Our group, in collaboration with Triovance, designed and produced a research-grade humanized lentiviral vector encoding for anti-CD19 CAR. Ongoing studies at the Faculty of Pharmacy at the Universidad Autónoma del Estado de Morelos are evaluating the CAR-T cell production processes using this vector (transduction, cell culture, cell expansion, and modified-T cells functionality) in a pre-clinical cellular model.
While cellular product manufacturing is a significant component of CAR-T cell therapy, specialized clinical centers and multi-disciplinary personnel are also needed to treat patients (53, 54). Starting to develop local and national clinical guidelines for CAR-T cell therapy will be beneficial to this field, as well as looking for accreditations from International standards (i.e. FACT) which strengthen our ability to manufacture cells and treat patients according to international quality standards, broadening also international collaborations (55).
HSCT is formally available in Mexico since 1995, and currently, around 15 centers have experienced and well-established programs in public and private hospitals, with variable capacity to perform autologous and allogeneic procedures from compatible related and unrelated, cord blood, or haploidentical donors (8, 56). There are several reports of the Mexican HSCT experience, which can be comparable with the success in other developing and developed countries (57). Mexican reports have shown how specific transplantation models adapted to our reality can maintain good clinical results at reduced costs (58). This infrastructure and experience may serve as a basis for developing local and National immune effector cell programs to broaden the impact and reach more patients. There is a need of establishing thoughtful, focused, and efficient care models to achieve maximum benefits in resource-limited areas.
The historical journey of pediatric leukemia diagnosis and treatment in Mexico shows remarkable improvements during the last decades and the capacity for change within healthcare systems.
CAR-T cell therapies have demonstrated efficacy and safety in recent clinical trials, leading to exponential progress in research in this field. In the upcoming years, these therapies will likely become part of conventional treatments for refractory or relapsed leukemias.
Currently, CAR-T cell therapy is not an essential or cost-effective treatment for emerging economies. There are also opinions on the need for more rationale for providing this therapy at the public expense of patients (50). However, with the early clinical successes and current advancements, much funding has been pouring into the field, and rapid improvements in equipment, reagents, and methods will eventually start to push down the costs. Research, non-profitable models, and academic collaborations between public and private institutions may help generate real-world data in the coming years.
Several key issues must be addressed to improve global access in Mexico, including infrastructure and material resources, training of human resources, reducing supplies and manufacturing costs, gaining clinical experience through clinical trials, and establishing an appropriate regulatory framework. Support from health authorities, collaborative efforts of academic, scientific, and private institutions, and increased investment in health and research are all indispensable. Specific research on the Mexican population is also required to determine whether specific characteristics might impact the outcomes of these treatments.
Implementing cellular therapies in Mexico and Latin America is feasible and necessary. With the potential growth of these therapies in the upcoming years, we need to start working and tackling challenges. There is infrastructure and previous experience in cancer diagnosis and treatment, with high-quality transplant programs and blood banks at the national level, which will serve as a basis for implementing CAR-T cell therapy programs.
It is necessary to increase awareness and knowledge about cell and gene therapy and to encourage investment in research and healthcare. Initial steps are ongoing, and the goal is clear: making these therapies a reality for children around the globe. Different groups are now looking for options to implement CAR-T cell and other immune effector therapies in Mexico, and we believe this is a time of opportunity to establish national and international collaborations and share ideas to achieve this goal and benefit more patients in the future.
The original contributions presented in the study are included in the article/supplementary material. Further inquiries can be directed to the corresponding author.
JB-O: Conceptualization, Writing – original draft, Writing – review & editing. AH-L: Conceptualization, Writing – review & editing. CG-D: Writing – review & editing. RR-L: Writing – review & editing. HF-B: Writing – review & editing. AM-A: Writing – review & editing, Conceptualization. AO-V: Conceptualization, Writing – original draft, Writing – review & editing.
The author(s) declare financial support was received for the research, authorship, and/or publication of this article. The financial support for this work was by I0031-2019-03MACROPRONAIISALUD-2019 Programa Nacional Estratégico para la Leucemia Infantil, CONAHCYT (Project number 303060). A post-doctoral fellowship from CONACHYT is given to Hernández-López A (CVU: 253979). We aknowledge Fundación Gonzalo Río Arronte I.A.P. for their support.
The authors declare that the research was conducted in the absence of any commercial or financial relationships that could be construed as a potential conflict of interest.
All claims expressed in this article are solely those of the authors and do not necessarily represent those of their affiliated organizations, or those of the publisher, the editors and the reviewers. Any product that may be evaluated in this article, or claim that may be made by its manufacturer, is not guaranteed or endorsed by the publisher.
1. Atun R, Bhakta N, Denburg A, Frazier AL, Friedrich P, Gupta S, et al. Sustainable care for children with cancer: a Lancet Oncology Commission. Lancet Oncol (2020) 21(4):e185–224. doi: 10.1016/S1470-2045(20)30022-X
2. Haslauer T, Greil R, Zaborsky N, Geisberger R. CAR T-cell therapy in hematological Malignancies. Int J Mol Sci (2021) 22(16):8996. doi: 10.3390/ijms22168996
4. Fajardo-Gutierrez A, González-Miranda G, Pachuca-Vázquez A, Allende-López A, Fajardo-Yamamoto LM, Rendón-Macías ME. Cancer incidence and mortality in Children in the Mexican Social Security Institute (1966-2013). Salud publica Mex (2016) 58:162–70. doi: 10.21149/spm.v58i2.7785
5. Rivera-Luna R, Shalkow-Klincovstein J, Velasco-Hidalgo L, Cárdenas-Cardós R, Zapata-Tarrés M, Aguilar-Ortiz M, et al. Descriptive Epidemiology in Mexican children with cancer under an open national public health insurance program. BMC Cancer (2014) 14:790. doi: 10.1186/1471-2407-14-790
7. Leon-Rodriguez E, Rivera Franco M, Ruiz González MC. Associtation of outcomes and socioeconomic status in mexican patients undergoing allogeneic stem cell transplantation. Biol Blood Marrow Transplant (2019) 25:2098–12. doi: 10.1016/j.bbmt.2019.06.010
8. Rodriguez-Romo L, González-Llano O, Mancias-Guerra C, Jaime-Pérez JC, Gómez-Peña A, Ruiz-Arguelles G, et al. Pediatric hematopoietic SCT in Mexico: recent activity and main problems. Bone Marrow Transplant (2011) 46:607–9. doi: 10.1038/bmt.2010.151
9. Gómez-Almaguer D, Ruiz-Argûelles GJ, Ponce-de-León S. Nutricional status and socio-economic conditions as prognostic factors in the outcome of therapy in childhood acute lymphoblastic leukemia. Int J Cancer Supply (1998) 11:52–5. doi: 10.1002/(sici)1097-0215(1998)78:11+<52::aid-ijc15>3.0.co;2-3
10. Rivera-Luna R, Olaya-Vargas A, Velásquez-Aviña M, Frenk S, Cárdenas-Cardós R, Leal-Leal C, et al. Early death in children with acute lymphoblastic leukemia: does malnutrition play a role? Pediatr Hematol Oncol (2008) 25(1):17–26. doi: 10.1080/08880010701774132
11. Olaya-Vargas A, Pérez-García M, Ramírez-Uribe N, Campo-Martinez MAD, Lopez-Hernández G, Hernández-García M, et al. Low dose total body irradiation (600 cGy) conditioning regimen in allogenic hematopoietic stem cell transplant in children with acute lymphoblastic leukemia. J Cancer Ther (2016) 7:586–92. doi: 10.4236/jct.2016.78061
12. Shalkow-Klinkovstein J, Sofia N-M, Marcelino. E-A. Comportamiento Eidemiologico del Cancer en Menores de 18 años, México 2008-2014, Secretaría de Salud, Subsecretaria de Prevención y Promoción de la Salud Centro Nacional para la Salud de la Infancia y la Adolescencia. In: Dirección de Prevención y Tratamiento del Cáncer en la Infancia y la Adolescencia, 1th Ed. (2015) México: Secretaría de Salud. p. 1–244. Available at: https://www.yumpu.com/es/document/view/56091667/20160601-boletin-2014-sedp12sep16-4.
13. Muñoz-Aguirre P, Huerta-Gutierrez R, Zamora S, Mohar A, Vega-Vega L, Hernández-Ávila JE, et al. Acute lymphoblastic leukaemia survival in children covered by seguro popular in Mexico: A national comprehensive analysis 2005-2017. Health Syst Reform (2021) 7(1):e1914897. doi: 10.1080/23288604.2021.1914897
14. Zapata-Tarrés M, Velasco-Hidalgo L, González-Garay A, Cárdenas-Cardos R, Rivera-Luna R. Does the Human Development Index relate with acute lymphoblastic leukemia incidence? Bol Med Hosp Infant Mex. (2021) 78(4):301–5. doi: 10.24875/BMHIM.20000043
15. Rivera-Luna R, Perez-Vera P, Galvan-Diaz C, Velasco-Hidalgo L, Olaya-Vargas A, Cardenas-Cardos R, et al. Triple-hit explanation for the worse prognosis of pediatric acute lymphoblastic leukemia among Mexican and Hispanic children. Front Oncol (2022) 12:1072811. doi: 10.3389/fonc.2022.1072811
16. Keever MAV, Gómez JA, Núñez AE, Rojas JB. Meta-analysis of prognostic factors related to mortality in children with acute lymphoblastic leukemia. Boletin Infantil del Hosp Infantil Mexico (2012) 69(3):175–89.
17. Gonzalez-Montalvo P, Romo H, Vega-Vega L, Sanchez-Zubieta F, Altamirano-Alvarez E, Rivera-Gomez R, et al. Collaborative risk-adapted treatment for pediatric acute lymphoblastic leukemia in Mexico. Blood (2017) 130(Supplement 1):2116. doi: 10.1182/blood.V130.Suppl_1.2116.2116
18. Romo H, Gonzalez-Montalvo P, Arce D, Aguilar-Escobar D, Mercado N, Echeandia N, et al. Evaluation of early treatment response utilizing the MAS-ALL18 adapted management guideline in four "Mexico in alliance with st. Jude" (MAS) member hospitals. Blood (2021) 138(Supplement 1):1210. doi: 10.1182/blood-2021-154325
19. Locatelli F, Zugmaier G, Rizzari C, Morris JD, Gruhn B, Klingebiel T, et al. Effect of blinatumomab vs chemotherapy on event-free survival among children with high-risk first-relapse B-cell acute lymphoblastic leukemia: A randomized clinical trial. JAMA (2021) 325(9):843–54. doi: 10.1001/jama.2021.0987
20. Roces. MaÁ. Pronaces Salud: Desarrollo científico, tecnológico e innovación para garantizar la salud como derecho universal. In: Revista Ciencias y Humanidades, volumen 4 (2022) Mexico: CONAHCYT. p. 3–5.
21. Labanieh L, Mackall C. CAR immune cells: design principles, resistance and the next generation. Nature (2023) 614:635–48. doi: 10.1038/s41586-023-05707-3
22. De Marco RC, Monzo HJ, Ojala PM. CAR T cell therapy: A versatile living drug. Int J Mol Sci (2023) 24(7):6300. doi: 10.3390/ijms24076300
23. Alnefaie A, Albogami S, Asiri Y, Ahmad T, Alotaibi SS, Al-Sanea MM, et al. Chimeric antigen receptor T-cells: an overview of concepts, applications, limitations, and proposed solutions. Front Bioeng. Biotechnol (2022) 10:797440. doi: 10.3389/fbioe.2022.797440
24. Dwivedi A, Karulkar A, Ghosh S, Rafiq A, Purwar R. Lymphocytes in cellular therapy: functional regulation of CAR T cells. Front Immunol (2019) 18:9:3180. doi: 10.3389/fimmu.2018.03180
25. Hernández-López A, Téllez-González MA, Mondragón-Terán P, Meneses-Acosta A. Chimeric antigen receptor-T cells: A pharmaceutical scope. Front Pharmacol (2021) 12:720692. doi: 10.3389/fphar.2021.720692
26. Tokarew N, Ogonek J, Endres S, von Bergwelt-Baildon M, Kobold S. Teaching an old dog new tricks: next-generation CAR T cells. Br J Cancer. (2019) 120(1):26–37. doi: 10.1038/s41416-018-0325-1
27. Mehrabadi AZ, Ranjbar R, Farzanehpour M, Shahriary A, Dorostkar R, Hamidinejad MA, et al. Therapeutic potential of CAR T cell in Malignancies: A scoping review. BioMed Pharmacother. (2022) 146:112512. doi: 10.1016/j.biopha.2021.112512
28. Sobia A, Muhammad YA, Farhan K, Sana IK, Muhammad AA, Zoia EK. A systematic review and meta-analysis of CD19-specific CAR-T cell therapy in relapsed/refractory acute lymphoblastic leukaemia in paediatrics and young adults: Safety and efficacy outcomes. Clin Lymphoma Myeloma Leukemia (2021) 21(4):e334–e347. doi: 10.1016/j.clml.2020.12.010
29. Cappell K, Kochenderfer J. Long-term outcomes following CAR T cell therapy: what we know so far. Nat Rev Clin Oncol (2023) 20:359–71. doi: 10.1038/s41571-023-00754-1
30. Jacoby E. The role of allogeneic HSCT after CAR T cells for acute lymphoblastic leukemia. Bone Marrow Transplant (2019) 54:810–4. doi: 10.1038/s41409-019-0604-3
31. Fry TJ, Shah NN, Orentas R, Stetler-Stevenson M, Yuan C, Ramakrishna S, et al. CD22-targeted CAR T cells induce remission in B-ALL that is naive or resistant to CD19-targeted CAR immunotherapy. Nat Med (2018) 24(1):20. doi: 10.1038/nm.4441
32. Moutsatsou P, Ochs J, Schmitt RH, Hewitt CJ, Hanga MP. Automation in cell and gene therapy manufacturing: from past to future. Biotechnol Lett (2019) 41:2250. doi: 10.3389/fimmu.2019.02250
33. Saez-Ibañez AR, Upadhaya S, Partridge T, Shah M, Correa D, Campbell J. Landscape of cancer cell therapies: trends and real-world data. Nat Rev Drug Discovery (2022) 21:631–2. doi: 10.1038/d41573-022-00095-1
34. Kansagra A, Farnia S, Majhail N. Expanding access to chimeric antigen receptor T-cell therapies: challenges and opportunities. Am Soiety Clan Once Educ Book (2020) 40):e27–34. doi: 10.1200/EDBK_279151
35. Palani H, Arunachalam A, Yasar M, Venkatraman A, Kulkarni U, Lionel S, et al. Decentralized manufacturing of anti CD19 CAR-T cells using CliniMacs Prodigy: real-world experience and cost analysis in India. Bone Marrow Transplant (2023) 58:160–7. doi: 10.1038/s41409-022-01866-5
36. ClinicSpots. CAR-T cell therapy in India (2023). Available at: https://www.clinicspots.com/blog/car-t-cell-therapy-in-India (Accessed August 17th, 2023).
37. National research with CAR-T cells is authorized by Anvisa (2022). Available at: https://agenciabrasil.ebc.com.br/en/saude/noticia/2022-07/anvisa-authorizes-national-research-car-t-cells-treat-cancer (Accessed August 17th, 2023).
38. Hungria V, Pierola AA, Schmidt FJ, Crusoe E, Pessoa de Magalhanes FRJ, Maiolino A, et al. CAR-T cell therapy for multiple myeloma: a practical toolkit for treatment in Brazil. J transfussion hemathol Cell Ther (2023) 45(2):266–74. doi: 10.1016/j.htct.2022.08.002
39. Adair JE, Androski L, Bayigga L, Bazira D, Brando E, Dee L, et al. Towards access for all: 1st Working Group Report for the Global Gene Therapy Initiative (GGTI). Gene Ther (2023) 30:216–21. doi: 10.1038/s41434-021-00284-4
40. Available at: https://caringcross.org/.
41. U.S. Food & Drug Administration (FDA). FDA Approval Brings First Gene Therapy to the United States (2017). Available at: https://www.fda.gov/news-events/press-announcements/fda-approval-brings-first-gene-therapy-united-states (Accessed August 14th, 2023).
42. U.S. Food & Drug Administration (FDA). Considerations for the development of Chimeric Antigen Receptor (CAR) T Cell Products (2022). Available at: https://www.fda.gov/regulatory-information/search-fda-guidance-documents/considerations-development-chimeric-antigen-receptor-car-t-cell-products (Accessed August 14th, 2023).
43. European Medicines Agency (EMA. European comission DG health and food Safety and European Medicines Agency Action Plan on ATMPs . Available at: https://www.ema.europa.eu/en/documents/other/european-commission-dg-health-food-safety-european-medicines-agency-action-plan-advanced-therapy_en-0.pdf (Accessed August 14th, 2023).
44. World Health Organization (WHO). Considerations in developing a regulatory framework for human cells and tissues and for advanced therapy medicinal products (2023). Available at: https://www.who.int/publications/m/item/considerations-in-developing-a-regulatory-framework-for-human-cells-and-tissues-and-for-advanced-therapy-medicinal-products (Accessed August 14th, 2023).
45. COFEPRIS. COFEPRIS entrega primer permiso de importación de vector para tratamiento CAR-T para pacientes con cáncer de sangre (2023). Available at: https://www.gob.mx/cofepris/articulos/cofepris-entrega-primer-permiso-de-importacion-de-vector-para-tratamiento-car-t-para-pacientes-con-cancer-de-sangre?idiom=es (Accessed September 26th, 2023).
46. Maschan M, Caimi P, Reese-Koc J, Pacheco-Sánchez G, Sharma A, Molostova O, et al. Multiple site place-of-care manufactured anti-CD19 CAR-T cells induce high remission rates in B-cell Malignancy patients. Nat Commun (2021) 12:7200. doi: 10.1038/s41467-021-27312-6
47. Buie LW. Balancing the CAR T: perspectives on efficacy and safety of CAR T-cell therapy in hematologic Malignancies. Am J Manag Care (2021) 27:S243–52. doi: 10.37765/ajmc.2021.88736
48. Sterner R, Sterner R. CAR-T cell therapy: current limitations and potential strategies. Blood Cancer J (2021) 11(4):69. doi: 10.1038/s41408-021-00459-7
49. Orentas R, Dropulic B, De Lima M. Place of care manufacturing of chimeric antigen receptor cells: Opportunities and challenges. Semin Hematol (2023) 60(1):20–4. doi: 10.1053/j.seminhematol.2023.01.001
50. Burki TK. CAR T-cell therapy roll-out in low-income and middle-income countries. In: Focus, The Lancet Haematology (2021) The Lancet Haematology (Elsevier). doi: 10.1016/S2352-3026(21)00068-5
51. Kin J, Lerman B, Barnes J, Bourisquot B, Jian Tan Y, Robinson A, et al. Cost effectiveness of chimeric antigen receptor T-cell therapy in relapsed or refractory pediatric B-cell acute lymphoblastic leukemia. J Clin Oncol (2018) 36(32):3192–202. doi: 10.1200/JCO.2018.79.0642
52. Sarkar R, Glowed N, Schiff D, Murphy J. Cost-effectiveness of chimeric antigen receptor T-cell therapy in pediatric relapsed/refractory B-cell acute lymphoblastic leukemia. JNCI J Natl Cancer Int (2019) 111(7):djy193. doi: 10.1093/jnci/djy193
53. Dulan S, Viers K, Wagner J, Clark M, Chang B, Gorospe G, et al. Developing and Monitoring a Standard-of-Care Chimeric antigen Receptor (CAR) T cell Clinical Quality and Regulatory Program. Bill Blood Marrow Transplant (2020) 26(8):1386–93. doi: 10.1016/j.bbmt.2020.03.021
54. Ravindranath A, Dubey A, Suresh S, Chaudhuri G, Chirmule N. CAR-T cell therapy in India requires a paradigm shift in training, education and health care processes. Cytotherapy (2022) 24(2):101–9. doi: 10.1016/j.jcyt.2021.09.007
55. Available at: https://www.factglobal.org/standards/immune-effector-standards.
56. Correa C, Gonzalez-Ramella O, Baldomero H, Basquiera AL, Baena R, Arcuri L, et al. Increasing access to hematopoietic cell transplantation in Latin America: results of the 2018 LABMT activity survey and trends since 2012. Bone Marrow Transplant (2022) 57(6):881–8. doi: 10.1038/s41409-022-01630-9
57. Leon-Rodriguez E, Rivera-Franco M. Outcomes of Hematopoietic Stem Cell Transplantation at a Limited-Resource Center in Mexico are comparable to those in developed countries. Biol Blood Marrow Transplant (2017) 23:1998–2003. doi: 10.1016/j.bbmt.2017.07.010
Keywords: childhood leukemia, Mexico, immunotherapy, cellular therapy, CAR-T cells, global access, gene therapy, cell therapy
Citation: Bustamante-Ogando JC, Hernández-López A, Galván-Díaz C, Rivera-Luna R, Fuentes-Bustos HE, Meneses-Acosta A and Olaya-Vargas A (2024) Childhood leukemias in Mexico: towards implementing CAR-T cell therapy programs. Front. Oncol. 13:1304805. doi: 10.3389/fonc.2023.1304805
Received: 30 September 2023; Accepted: 15 December 2023;
Published: 18 January 2024.
Edited by:
Rosana Pelayo, Delegación Puebla, MexicoReviewed by:
Hector Mayani, Mexican Social Security Institute (IMSS), MexicoCopyright © 2024 Bustamante-Ogando, Hernández-López, Galván-Díaz, Rivera-Luna, Fuentes-Bustos, Meneses-Acosta and Olaya-Vargas. This is an open-access article distributed under the terms of the Creative Commons Attribution License (CC BY). The use, distribution or reproduction in other forums is permitted, provided the original author(s) and the copyright owner(s) are credited and that the original publication in this journal is cited, in accordance with accepted academic practice. No use, distribution or reproduction is permitted which does not comply with these terms.
*Correspondence: Juan Carlos Bustamante-Ogando, ZHJidXN0YW1hbnRlX2lucEBob3RtYWlsLmNvbQ==
Disclaimer: All claims expressed in this article are solely those of the authors and do not necessarily represent those of their affiliated organizations, or those of the publisher, the editors and the reviewers. Any product that may be evaluated in this article or claim that may be made by its manufacturer is not guaranteed or endorsed by the publisher.
Research integrity at Frontiers
Learn more about the work of our research integrity team to safeguard the quality of each article we publish.