- 1Department of Obstetrics and Gynecology, Beijing Hospital, National Center of Gerontology; Institute of Geriatric Medicine, Chinese Academy of Medical Sciences, Beijing, China
- 2The Key Laboratory of Geriatrics, Beijing Institute of Geriatrics, Institute of Geriatric Medicine, Chinese Academy of Medical Sciences, Beijing Hospital, National Center of Gerontology of National Health Commission, Beijing, China
Metabolic reprogramming is a phenomenon in which cancer cells alter their metabolic pathways to support their uncontrolled growth and survival. Platinum-based chemotherapy resistance is associated with changes in glucose metabolism, amino acid metabolism, fatty acid metabolism, and tricarboxylic acid cycle. These changes lead to the creation of metabolic intermediates that can provide precursors for the biosynthesis of cellular components and help maintain cellular energy homeostasis. This article reviews the research progress of the metabolic reprogramming mechanism of platinumbased chemotherapy resistance caused by three major nutrients in ovarian cancer.
1 Introduction
In gynecologic cancer, OC is ranked 2nd in mortality (1). Among ovarian malignancies, epithelial ovarian cancer (EOC) accounts for 90% (2). Since most patients are diagnosed at an advanced stage (3), the first-line primary treatment regimen recommended by the NCCN guidelines is surgery followed by platinum-based chemotherapy (4). Nevertheless, 25% of patients occurs platinum resistance at first recurrence (5). Patients who recrudesce within 6 months after the end of platinum-based first-line therapy are classified as being platinum-resistant, with a poor response to subsequent chemotherapy and median overall survival of 9-12 months (6). Therefore, the mechanism of platinum resistance and its influencing factors have been actively studied and explored, and new treatment options have been proposed to improve the prognosis of patients by finding key targets.
Cisplatin utilizes DNA as its most critical target of action to exert its cytotoxic effects by forming DNA adducts (7). The mechanisms of platinum-based chemotherapy resistance in ovarian cancer are complex and multifactorial, involving genetic and epigenetic changes, alterations in drug transport and metabolism, and activation of cellular survival pathways (8–15). Recent studies have shown that metabolic reprogramming plays a crucial role in the development of platinum-based chemotherapy resistance in ovarian cancer (16).
Metabolic reprogramming is a phenomenon in which cancer cells alter their metabolic pathways to support their uncontrolled growth and survival (17, 18). Platinum-based chemotherapy resistance is associated with changes in glucose metabolism, amino acid metabolism, fatty acid metabolism, and tricarboxylic acid cycle. These changes lead to the creation of metabolic intermediates that can provide precursors for the biosynthesis of cellular components and help maintain cellular energy homeostasis (19). This article reviews the research progress of the metabolic reprogramming mechanism of platinum-based chemotherapy resistance caused by three major nutrients in ovarian cancer.
2 Glucose metabolism
In the 2020s, Warburg’s findings revealed that even under aerobic conditions, tumor cells preferentially utilize glycolysis, known as aerobic glycolysis, instead of oxidative phosphorylation (20, 21). This metabolic reprogramming in tumor cells is not aimed at increasing energy production, but rather at generating raw materials for the synthesis of biological macromolecules, which support cell proliferation under limited resources. Recent studies have demonstrated that oncogenes and various tumor regulators can modulate key proteins and rate-limiting enzymes involved in glucose metabolism in tumor cells, promoting their growth and survival (22–24).
Key substances in the glucose metabolism process of ovarian cancer cells, including glucose transporter 1, hexokinase 1 (HK1), hexokinase II (HK2), etc., promote the proliferation and metastasis of tumor cells through a variety of downstream target genes and signaling pathways (25–32). In platinum-resistant ovarian cancer, the dysregulation of glucose metabolism intermediates, key enzymes and abnormally active metabolic activities play a significant role in promoting drug resistance in tumor cells.
2.1 Serine/threonine kinase Aurora-A
In a study, the researchers utilized the organoid model of ovarian cancer to validate the hypothesis that abnormally overexpressed serine/threonine kinase Aurora-A directly phosphorylates sex-determining region Y-box 8 (SOX8) at Ser327 or indirectly enhances SOX8 transcription via c-Myc (33). Subsequently, SOX8 targets HK2 and lactate dehydrogenase (LDHA), which affect the glycolysis process via the SOX8/FOXK1 axis, ultimately leading to drug resistance (Table 1)
2.2 Hexokinase II
Zhang XY et al. (34) shows that HK2 presents resistance to cisplatin in the ovarian cancer cell, promotes the resistance by enhancing cisplatin-induced autophagy, and boosts the cisplatin-induced autophagy by activating the ERK1/2 pathway. The upregulation of HK2 expression and subsequent drug resistance can be attributed to the effects of lysophosphatidic acid (LPA) (35). HK2 is expressed on mitochondria in cancer cells and interacts with voltage-dependent anion channel 1 (VDAC1) to impede apoptosis (Figure 1). Notably, studies have demonstrated that peroxisome proliferator-activated receptor coactivator 1 (PGC1α) (36), which is highly expressed in drug-resistant ovarian cancer cells, promotes the transcription of α heat shock protein (HSP70). Consequently, the upregulation of HSP70 leads to increased expression of HK2 on mitochondria, and its binding to VDAC1. Therefore, the HSP70/HK2/VDAC1 signaling pathway may serve as a potential target for therapies.
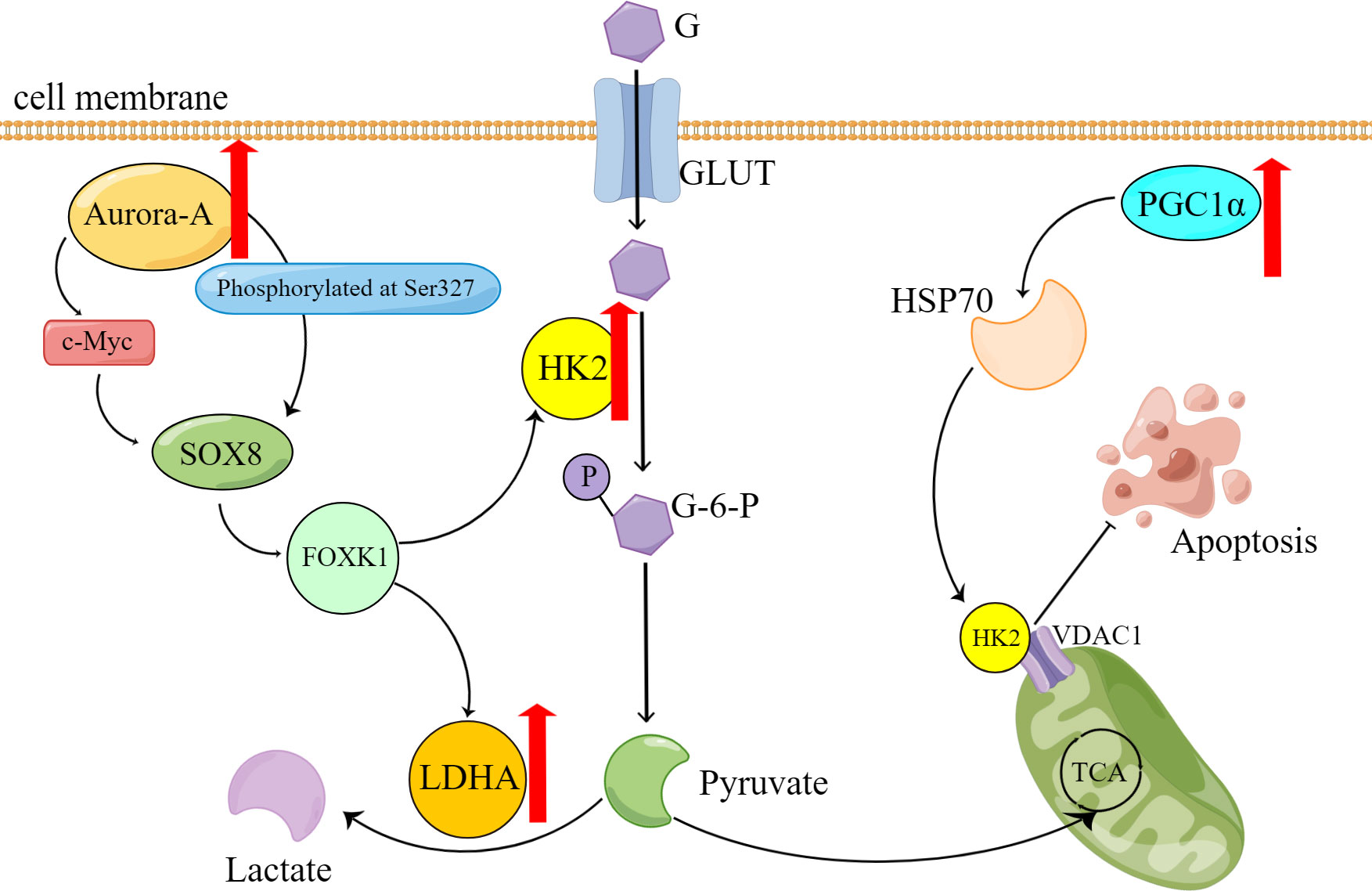
Figure 1 Overexpression of Aurora-A and PGC1α causes reprogramming of glucose metabolism and contributes to the onset of platinum resistance in ovarian cancer cells. G, glucose; GLUT, Glucose transporter; G-6-P, Glucose-6-phosphate; P, phosphate; TCA, tricarboxylic acid cycle.
2.3 Pyruvate dehydrogenase kinase 1
Platinum-resistant ovarian cancer cells exhibit increased levels of pyruvate dehydrogenase kinase 1 (PDK1) in comparison to platinum-sensitive cells. The study by Zhang M et al. revealed that upregulated PDK1 activates epidermal growth factor receptor (EGFR) to facilitate the development of chemotherapy resistance (37). Notably, downregulating PDK1 in drug-resistant cells enhances the sensitivity to cisplatin-induced cell death. Despite these findings, the precise mechanism of action remains unclear.
2.4 Phosphoglycerate dehydrogenase
Van Nyen T, et al. (38) explored the relevance of phosphoglycerate dehydrogenase (PHGDH) expression and serine biosynthesis activity in tumors with acquired platinum resistance after platinum chemotherapy. They recognized a subset of ovarian cancer patients who relapsed after platinum-based chemotherapy with decreasing in PHGDH expression. Then, through in vivo, in vitro, and metabolic pathways tests, they investigated that this subgroup had undergone metabolic changes of decreased serine biosynthesis, which caused tumor cells to need exogenous serine for metabolic activities. This metabolic shift is accompanied by a regenerative phenotype of NAD+. Although tumor cells have no significant change in NAD+ levels, NAD+ regeneration is active and benefits from maintaining PARP’s activity to resistant platinum.
2.5 Mitochondrial oxidative phosphorylation
While the role of aerobic glycolysis in tumors has been extensively studied, recent research suggests that mitochondrial oxidative phosphorylation (OXPHOS) may also contribute to platinum resistance in ovarian cancer.
Platinum-based chemotherapy agents may induce an increase in NAD+-dependent deacetylase SIRT1 (39), which in turn promotes OXPHOS and enriches chemoresistant aldehyde dehydrogenase (ALDH) + ovarian cancer stem cells (OCSC), ultimately leading to drug resistance. However, the precise mechanisms underlying this phenomenon require further investigation. Furthermore, as a critical modulator of the mitochondrial respiratory chain (40), downregulation of the TRAP1 chaperone protein inhibits glycolysis in tumor cells and shifts metabolism towards OXPHOS, which in turn promotes the release of various inflammatory factors, including IL-6, to stimulate an inflammatory response that reduces platinum sensitivity in ovarian cancer cells (41).
2.6 Potential of metformin
As a classic treatment for diabetes, metformin may play a therapeutic role by exploiting the metabolic changes of platinum-resistant ovarian cancer, which is conducive to the cytotoxic effect of platinum drugs and prevents the progression of tumor cells. Metformin has been identified as a promising drug to overcome resistance to platinum-based therapy in ovarian cancer. The mechanism of action involves targeting mitochondrial complex I and ATP synthases, leading to a partial reversal of resistance (42). Metformin also regulates apoptosis by downregulating Bcl-2 and Bcl-xL expression and upregulating Bax and Cytochrome expression (43). Moreover, metformin has been shown to reduce inflammation and improve drug sensitivity by inhibiting the NFκB signaling pathway and IL-6 secretion (44, 45). Furthermore, metformin can reverse resistance via the p53-PDK1-HKII pathway and by activating the AKT signaling pathway while inhibiting the IGF2R signaling pathway (46, 47). Notably, metformin can also modulate the metabolic profile of resistant cells by upregulating taurine and histidine levels and downregulating tyrosine kinase levels, thus preventing chemoresistance (48, 49). In summary, the combination of metformin and cisplatin presents a promising therapeutic strategy for preventing drug resistance in ovarian cancer cells with metabolic alterations.
3 Lipid metabolism
Adipocytes and the tumor microenvironment formed with the participation of adipocytes have a very important impact on the occurrence, development, and prognosis of malignant tumors (50). Ovarian cancer is no exception (51). Aberrant expression of key enzymes, transport proteins, cellular receptors, and various adipocyte secretagogues (including cytokines, adipokines, and pro-inflammatory factors) in ovarian cancer promotes the formation of tumor cell microenvironment and increases the aggressiveness of ovarian cancer cells. For example, CD36 (52), one of the fatty acid translocases (FAT), contributes to the growth and distant metastasis of ovarian cancer cells; fatty acid binding protein 4(FABP4) contributes to the metastasis and implantation of ovarian cancer into the omentum (53). In turn, these key substances may serve as indicators for monitoring the process of platinum-based chemotherapy in ovarian cancer and as key targets for treatment after the development of platinum resistance.
3.1 β-oxidation
Fatty acid β-oxidation has been linked to platinum resistance (54), with genetic ablation of the homology box-containing developmental regulator NKX2-8 promoting fatty acid metabolism reprogramming and subsequent drug resistance in epithelial ovarian cancer cells in the adipose microenvironment (55). Additionally, a novel biomarker, Collagen type XI alpha 1 (COL11A1) (56), has been found to induce platinum resistance by upregulating fatty acid metabolism in ovarian cancer through its binding to discoid domain receptor 2 and activating Src-Akt-AMPK signaling. Furthermore, activation of the transcription factor c-JUN α diacylglycerol kinase (DGKA) leads to the recruitment of c-JUN N-terminal kinase to c-JUN and enhances the expression of the cell cycle regulator WEE1 under cisplatin stimulation, ultimately leading to platinum resistance in ovarian cancer (57).
3.2 Fatty acid binding protein 4
In the context of ovarian cancer, FABP4 plays a central role in regulating adipocyte-induced lipid metabolism in cancer cells (58). Its overexpression promotes tumor proliferation and metastasis and mediates drug resistance to carboplatin. However, the downstream factors and pathways that specifically mediate drug resistance of FABP4 remain unclear. Adipocytes can also inhibit cisplatin-induced apoptosis by secreting arachidonic acid, which activates Akt (59). Moreover, the SP1-12LOX axis can upregulate the expression of multidrug resistance-associated protein (MRP) via signal transduction, increase the production of arachidonic acid-derived metabolites, and promote drug resistance (60).
3.3 Cholesterol
Cholesterol metabolism dysregulation can be a contributing factor to platinum resistance in ovarian cancer, as cholesterol is a vital component of cell membranes and plasma lipoproteins and a precursor for important bioactive molecules (61). The decrease in TRAP1 expression, as mentioned earlier, also impacts cholesterol metabolism. In drug-resistant ovarian cancer cells, exogenous uptake becomes the primary source of cholesterol, with decreased expression of farnesyl bisphosphate synthase (FDPS) and oxidized squalene cyclase (OCS) involved in endogenous cholesterol synthesis, and increased expression of low-density lipoprotein receptor (LDLR) promoting exogenous cholesterol uptake (62). Furthermore, carboplatin-resistant ovarian cancer cells exhibit upregulation of ADP ribosylation factor 4C (ARL4C), a member of the ADP ribosylation factor subfamily, and Notch-RBP-Jκ-H5K3Me4, a member of the oxysterol-binding protein family (OSBP), resulting in the upregulation of OSBPL5, a member of the OSBP family, promoting cholesterol accumulation and autophagy, leading to carboplatin resistance (63). Malignant ascites exhibited elevated cholesterol levels, with upregulated drug efflux proteins ABCG2 and MDR1, and cholesterol receptor LXRα expression, contributing to multidrug resistance (64).
4 Amino acid metabolism
Assays of serum-free amino acids have shown that the levels of many amino acids change significantly during the development of ovarian cancer and that this change in turn promotes tumor development (65, 66). Amino acids that changed significantly compared to healthy women included histidine, alanine, asparagine, citrulline, cystine, ethanolamine, lysine, methionine, ornithine, threonine, and tryptophan (67). There are many mechanisms of tumorigenesis caused by changes in amino acid metabolism, such as regulating the invasion of ovarian cancer cells through transcription factor ETS1 in the case of glutamine deprivation (68); Six enzymes of folic acid metabolism pathway were overexpressed, among which serine hydroxymethyltransferase 1 promoted the expression of cancer inflammatory factors by regulating sialic acid N-acetylneuraminic acid, and promoted the growth and metastasis of ovarian cancer tumor cells (69); Upregulation of non-tyrosine kinase (FER) in ovarian cancer promotes tumorigenesis by activating the PI3K-AKT pathway through the kinase-substrate mode of action between FER and insulin receptor substrate 4 (IRS4) (70). Changes in amino acid metabolism may also contribute to the development of platinum resistance in ovarian cancer.
4.1 Glutamine
Glutamine, the most abundant non-essential amino acid in the human body, serves a multitude of purposes, including its function as a key substrate or molecule in the biosynthesis of various compounds, and its essential role in maintaining normal cellular function and integrity (71). Aside from relying on aerobic glycolysis, drug-resistant ovarian cancer cells exhibit an addiction to glutamine (72). DNA methylation has been found to regulate the expression of the critical enzyme glutamine synthetase, resulting in the metabolic reprogramming of glutamine in tumor cells (73). Preferentially directing glutamine towards glutathione (GSH) synthesis instead of the TCA cycle ultimately leads to increased levels of glutamine, glutamic acid, and glutathione. Despite the sensitivity of drug-resistant tumor cells to nutrient deficiencies, glutamine can combat starvation-induced cell death by increasing nucleotide concentrations (74).
4.2 Glutathione
GSH is synthesized from glutamic acid, cysteine, and glycine and is one of the important members of cellular antioxidants (75). GSH depletion in tumor cells can increase the attack of chemotherapy drugs on tumor cells through reactive oxygen species (ROS) accumulation, detoxification reduction, and iron death to achieve therapeutic purposes (76). Non-targeted metabolomics analyses showed that platinum-resistant ovarian cancer cells have significantly reduced levels of GSH, which contributes to chemoresistance by forming adducts with cisplatin, leading to reduced intracellular concentrations of the active drug, and cisplatin-GSH adducts also reduce intracellular GSH levels. And these resistant cells can activate alternative antioxidant defenses independent of GSH (77). Cysteine stored in large quantities in GSH has a protective effect on ovarian cancer cells in a hypoxic environment and can also make tumor cells resistant to carboplatin (78, 79) (Figure 2).
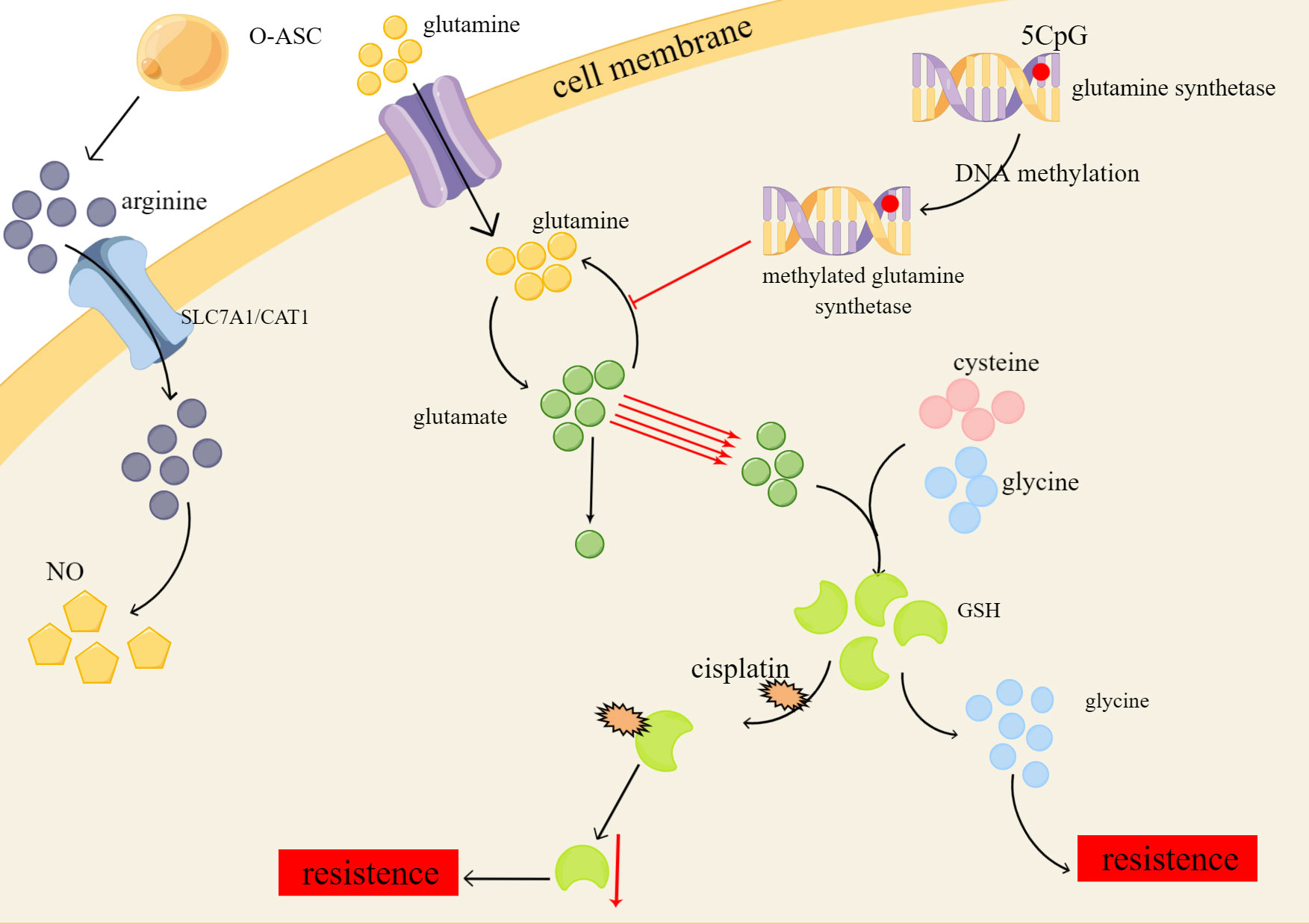
Figure 2 Platinum resistance caused by changes of glutamine, GSH and ariginine in amino acid metabolism of ovarian cancer. O-ASC, omental adipose stromal cells; SLC7A1/CAT1, cationic amino acid transporters; NO, nitric oxide.
4.3 Other amino acids
In the context of arginine deficiency, Omental adipose stromal cells (O-ASC) have been shown to stimulate tumor cell growth and NO synthesis by secreting arginine (80). The uptake and transport of arginine across cell membranes are mediated by cationic amino acid transporters, specifically SLC7A1/CAT1, which are highly expressed in ovarian cancer and are involved in the metabolic reprogramming of arginine, ultimately promoting platinum resistance (81). Furthermore, studies have shown that ovarian cancer cells with elevated levels of indoleamine 2,3-dioxygenase 1 (IDO1), an enzyme that catalyzes the conversion of tryptophan to l-kynurenine (Kyn), exhibit platinum resistance by downregulating the ROS/p53 pathway (82). Hence, it is important to further investigate the role of arginine transport and IDO1 in the development of platinum resistance in ovarian cancer cells.
5 Summary
The metabolic processes of the three major nutrients are interrelated and influence each other. For example, glucose can be converted into fat, and the intermediate products of sugar metabolism can generate non-essential amino acids. Glycerol can be converted into sugar in the body, and most amino acids can be converted into glucose. Proteins can be converted into fats, amino acids can be used as raw material for phospholipid synthesis, and glycerol can be converted into non-essential amino acids. The metabolism between the three is linked by the tricarboxylic acid cycle. The changes in key enzymes, intermediates, upstream genes, and downstream targets in the process of being stimulated by cytotoxic drugs can cause changes in a variety of metabolic processes, resulting in changes in ovarian cancer cells and their tumor microenvironment. These changes can help tumor cells cope with the need for nutrients during growth. In addition to the three major nutrients, the metabolic changes of vitamins, metals, and trace elements may also lead to platinum resistance in tumors. At present, the clinical treatment of patients with platinum-resistant recurrent ovarian cancer is still a difficult problem, and the key substances in the process of growth and metabolism and their molecular pathways may be used as therapeutic targets to improve the prognosis and survival time of patients. In general, metabolic reprogramming is of great significance in finding the key points for the treatment of platinum-resistant relapse.
Author contributions
All authors contributed to the article and approved the submitted version.
Conflict of interest
The authors declare that they have no known competing financial interests or personal relationships that could have appeared to influence the work reported in this paper.
Publisher’s note
All claims expressed in this article are solely those of the authors and do not necessarily represent those of their affiliated organizations, or those of the publisher, the editors and the reviewers. Any product that may be evaluated in this article, or claim that may be made by its manufacturer, is not guaranteed or endorsed by the publisher.
References
1. Lheureux S, Braunstein M, Oza AM. Epithelial ovarian cancer: Evolution of management in the era of precision medicine. CA Cancer J Clin (2019), 280–304. doi: 10.3322/caac.21559
2. Armstrong DK, Alvarez RD, Backes FJ, Bakkum-Gamez JN, Barroilhet L, Behbakht K, et al. NCCN guidelines(R) insights: ovarian cancer, version 3.2022. J Natl Compr Canc Netw (2022), 972–80. doi: 10.6004/jnccn.2022.0047
3. Torre LA, Trabert B, DeSantis CE, Miller KD, Samimi G, Runowicz CD, et al. Ovarian cancer statistics, 2018. CA Cancer J Clin (2018), 284–96. doi: 10.3322/caac.21456
4. Armstrong DK, Alvarez RD, Backes FJ, Bakkum-Gamez JN, Barroilhet L, Behbakht K, et al. NCCN guidelines® Insights: ovarian cancer, version 3.2022. J Natl Compr Cancer Network: JNCCN (2022) 20(9):972–80. doi: 10.6004/jnccn.2022.0047
5. Shoji T, Enomoto T, Abe M, Okamoto A, Nagasawa T, Oishi T, et al. Efficacy and safety of standard of care with/without bevacizumab for platinum-resistant ovarian/fallopian tube/peritoneal cancer previously treated with bevacizumab: The Japanese Gynecologic Oncology Group study JGOG3023. Cancer Sci (2021) 113(1):240–50. doi: 10.1111/cas.15185
6. Davis A, Tinker AV, Friedlander M. Platinum resistant” ovarian cancer: what is it, who to treat and how to measure benefit? Gynecol Oncol (2014) 133(3):624–31. doi: 10.1016/j.ygyno.2014.02.038
7. Kanno Y, Chen C-Y, Lee H-L, Chiou J-F, Chen Y-J. Molecular mechanisms of chemotherapy resistance in head and neck cancers. Front Oncol (2021) 11:640392. doi: 10.3389/fonc.2021.640392
8. Galluzzi L, Senovilla L, Vitale I, Michels J, Martins I, Kepp O, et al. Molecular mechanisms of cisplatin resistance. Oncogene (2011) 31(15):1869–83. doi: 10.1038/onc.2011.384
9. Dasari S, Tchounwou PB. Cisplatin in cancer therapy: molecular mechanisms of action. Eur J Pharmacol (2014) 740:364–78. doi: 10.1016/j.ejphar.2014.07.025
10. Tian L, Shao M, Gong Y, Wei T, Zhu Y, Chao Y, et al. Epigenetic platinum complexes breaking the “Eat me/don’t eat me” Balance for enhanced cancer chemoimmunotherapy. Bioconjug Chem (2022), 343–52. doi: 10.1021/acs.bioconjchem.1c00576
11. Pejovic T, Fitch K, Mills G. Ovarian cancer recurrence: “is the definition of platinum resistance modified by PARP inhibitors and other intervening treatments”. Cancer Drug res. (Alhambra Calif) (2022) 5(2):451–8. doi: 10.20517/cdr.2021.138
12. Nicholson HA, Sawers L, Clarke RG, Hiom KJ, Ferguson MJ, Smith G. Fibroblast growth factor signaling influences homologous recombination-mediated DNA damage repair to promote drug resistance in ovarian cancer. Br J cancer (2022) 127(7):1340–51. doi: 10.1038/s41416-022-01899-z
13. Ziebarth AJ, Nowsheen S, Steg AD, Shah MM, Katre AA, Dobbin ZC, et al. Endoglin (CD105) contributes to platinum resistance and is a target for tumor-specific therapy in epithelial ovarian cancer. Clin Cancer Res (2012) 19(1):170–82. doi: 10.1158/1078-0432.CCR-12-1045
14. Ali R, Aouida M, Alhaj Sulaiman A, MadhuSudan S, Ramotar D. Can cisplatin therapy be improved? Pathways that can be targeted. Int J Mol Sci (2022) 23(13). doi: 10.3390/ijms23137241
15. Rinne N, Christie EL, Ardasheva A, Kwok CH, Demchenko N, Low C, et al. Targeting the PI3K/AKT/mTOR pathway in epithelial ovarian cancer, therapeutic treatment options for platinum-resistant ovarian cancer. Cancer Drug res (Alhambra Calif) (2021) 4(3):573–95. doi: 10.20517/cdr.2021.05
16. Cocetta V, Ragazzi E, Montopoli M. Links between cancer metabolism and cisplatin resistance. Int Rev Cell Mol Biol (2020) 354:107–64. doi: 10.1016/bs.ircmb.2020.01.005
17. Hanahan D, Weinberg RA. Hallmarks of cancer: the next generation. Cell (2011) 144(5):646–74. doi: 10.1016/j.cell.2011.02.013
18. Pavlova NN, Thompson CB. The emerging hallmarks of cancer metabolism. Cell Metab (2016) 23(1):27–47. doi: 10.1016/j.cmet.2015.12.006
19. Wang L, Zhao X, Fu J, Xu W, Yuan J. The role of tumour metabolism in cisplatin resistance. Front Mol Biosci (2021) 8:691795. doi: 10.3389/fmolb.2021.691795
20. Kubicka A, Matczak K, Łabieniec-Watała M. More than meets the eye regarding cancer metabolism. Int J Mol Sci (2021) 22(17). doi: 10.3390/ijms22179507
21. Vander Heiden MG, Cantley LC, Thompson CB. Understanding the warburg effect: the metabolic requirements of cell proliferation. Sci (New York NY) (2009) 324(5930):1029–33. doi: 10.1126/science.1160809
22. Taefehshokr S, Taefehshokr N, Hemmat N, Hajazimian S, Isazadeh A, Dadebighlu P, et al. The pivotal role of MicroRNAs in glucose metabolism in cancer. Pathol Res practice (2021) 217:153314. doi: 10.1016/j.prp.2020.153314
23. Zhang Y, Meng Q, Sun Q, Xu ZX, Zhou H, Wang Y. LKB1 deficiency-induced metabolic reprogramming in tumorigenesis and non-neoplastic diseases. Mol Metab (2021) 44:101131. doi: 10.1016/j.molmet.2020.101131
24. Boroughs LK, DeBerardinis RJ. Metabolic pathways promoting cancer cell survival and growth. Nat Cell Biol (2015) 17(4):351–9. doi: 10.1038/ncb3124
25. Rudlowski C, Moser M, Becker AJ, Rath W, Buttner R, Schroder W, et al. GLUT1 mRNA and protein expression in ovarian borderline tumors and cancer. Oncology (2004) 66(5):404–10. doi: 10.1159/000079489
26. Ma Y, Wang W, Idowu MO, Oh U, Wang XY, Temkin SM, et al. Ovarian cancer relies on glucose transporter 1 to fuel glycolysis and growth: anti-tumor activity of BAY-876. Cancers (2018) 11(1). doi: 10.3390/cancers11010033
27. Wang X, Duan W, Li X, Liu J, Li D, Ye L, et al. PTTG regulates the metabolic switch of ovarian cancer cells via the c-myc pathway. Oncotarget (2015) 6(38):40959–69. doi: 10.18632/oncotarget.5726
28. Iida T, Yasuda M, Miyazawa M, Fujita M, Osamura RY, Hirasawa T, et al. Hypoxic status in ovarian serous and mucinous tumors: relationship between histological characteristics and HIF-1alpha/GLUT-1 expression. Arch Gynecol Obstet (2008) 277(6):539–46. doi: 10.1007/s00404-007-0500-8
29. Cantuaria G, Fagotti A, Ferrandina G, Magalhaes A, Nadji M, Angioli R, et al. GLUT-1 expression in ovarian carcinoma: association with survival and response to chemotherapy. Cancer (2001) 92(5):1144–50. doi: 10.1002/1097-0142(20010901)92:5<1144::aid-cncr1432>3.0.co;2-t
30. Li Y, Tian H, Luo H, Fu J, Jiao Y, Li Y. Prognostic significance and related mechanisms of hexokinase 1 in ovarian cancer. OncoTargets Ther (2020) 13:11583–94. doi: 10.2147/ott.S270688
31. Liu X, Zuo X, Sun X, Tian X, Teng Y. Hexokinase 2 promotes cell proliferation and tumor formation through the wnt/β-catenin pathway-mediated cyclin D1/c-myc upregulation in epithelial ovarian cancer. J Cancer (2022) 13(8):2559–69. doi: 10.7150/jca.71894
32. Siu MKY, Jiang YX, Wang JJ, Leung THY, Han CY, Tsang BK, et al. Hexokinase 2 regulates ovarian cancer cell migration, invasion and stemness via FAK/ERK1/2/MMP9/NANOG/SOX9 signaling cascades. Cancers (2019) 11(6). doi: 10.3390/cancers11060813
33. Sun H, Wang H, Wang X, Aoki Y, Wang X, Yang Y, et al. Aurora-A/SOX8/FOXK1 signaling axis promotes chemoresistance via suppression of cell senescence and induction of glucose metabolism in ovarian cancer organoids and cells. Theranostics (2020) 10(15):6928–45. doi: 10.7150/thno.43811
34. Zhang XY, Zhang M, Cong Q, Zhang MX, Zhang MY, Lu YY, et al. Hexokinase 2 confers resistance to cisplatin in ovarian cancer cells by enhancing cisplatin-induced autophagy. Int J Biochem Cell Biol (2018) 95:9–16. doi: 10.1016/j.biocel.2017.12.010
35. Mukherjee A, Ma Y, Yuan F, Gong Y, Fang Z, Mohamed EM, et al. Lysophosphatidic acid up-regulates hexokinase II and glycolysis to promote proliferation of ovarian cancer cells. Neoplasia (New York NY) (2015) 17(9):723–34. doi: 10.1016/j.neo.2015.09.003
36. Li Y, Kang J, Fu J, Luo H, Liu Y, Li Y, et al. PGC1α Promotes cisplatin resistance in ovarian cancer by regulating the HSP70/HK2/VDAC1 signaling pathway. Int J Mol Sci (2021) 22(5). doi: 10.3390/ijms22052537
37. Zhang M, Cong Q, Zhang XY, Zhang MX, Lu YY, Xu CJ. Pyruvate dehydrogenase kinase 1 contributes to cisplatin resistance of ovarian cancer through EGFR activation. J Cell Physiol (2019) 234(5):6361–70. doi: 10.1002/jcp.27369
38. Van Nyen T, Planque M, van Wagensveld L, Duarte JAG, Zaal EA, Talebi A, et al. Serine metabolism remodeling after platinum-based chemotherapy identifies vulnerabilities in a subgroup of resistant ovarian cancers. Nat Commun (2022) 13(1):4578. doi: 10.1038/s41467-022-32272-6
39. Sriramkumar S, Sood R, Huntington TD, Ghobashi AH, Vuong TT, Metcalfe TX, et al. Platinum-induced mitochondrial OXPHOS contributes to cancer stem cell enrichment in ovarian cancer. J Transl Med (2022) 246. doi: 10.1186/s12967-022-03447-y
40. Rasola A, Neckers L, Picard D. Mitochondrial oxidative phosphorylation TRAP(1)ped in tumor cells. Trends Cell Biol (2014) 24(8):455–63. doi: 10.1016/j.tcb.2014.03.005
41. Matassa DS, Amoroso MR, Lu H, Avolio R, Arzeni D, Procaccini C, et al. Oxidative metabolism drives inflammation-induced platinum resistance in human ovarian cancer. Cell Death Differ (2016) 23(9):1542–54. doi: 10.1038/cdd.2016.39
42. Ricci F, Brunelli L, Affatato R, Chilà R, Verza M, Indraccolo S, et al. Overcoming platinum-acquired resistance in ovarian cancer patient-derived xenografts. Ther Adv Med Oncol (2019) 11:1758835919839543. doi: 10.1177/1758835919839543
43. Patel S, Kumar L, Singh N. Metformin and epithelial ovarian cancer therapeutics. Cell Oncol (Dordrecht) (2015) 38(5):365–75. doi: 10.1007/s13402-015-0235-7
44. Xu S, Yang Z, Jin P, Yang X, Li X, Wei X, et al. Metformin suppresses tumor progression by inactivating stromal fibroblasts in ovarian cancer. Mol Cancer Ther (2018) 17(6):1291–302. doi: 10.1158/1535-7163.Mct-17-0927
45. Dos Santos Guimarães I, Ladislau-Magescky T, Tessarollo NG, Dos Santos DZ, Gimba ERP, Sternberg C, et al. Chemosensitizing effects of metformin on cisplatin- and paclitaxel-resistant ovarian cancer cell lines. Pharmacol reports: PR (2018) 70(3):409–17. doi: 10.1016/j.pharep.2017.11.007
46. Du J, Shi HR, Ren F, Wang JL, Wu QH, Li X, et al. Inhibition of the IGF signaling pathway reverses cisplatin resistance in ovarian cancer cells. BMC cancer (2017) 17(1):851. doi: 10.1186/s12885-017-3840-1
47. Han CY, Patten DA, Lee SG, Parks RJ, Chan DW, Harper ME, et al. p53 Promotes chemoresponsiveness by regulating hexokinase II gene transcription and metabolic reprogramming in epithelial ovarian cancer. Mol carcinogenesis (2019) 58(11):2161–74. doi: 10.1002/mc.23106
48. Bishnu A, Sakpal A, Ghosh N, Choudhury P, Chaudhury K, Ray P. Long term treatment of metformin impedes development of chemoresistance by regulating cancer stem cell differentiation through taurine generation in ovarian cancer cells. Int J Biochem Cell Biol (2019) 107:116–27. doi: 10.1016/j.biocel.2018.12.016
49. Kim NY, Lee HY, Lee C. Metformin targets Axl and Tyro3 receptor tyrosine kinases to inhibit cell proliferation and overcome chemoresistance in ovarian cancer cells. Int J Oncol (2015) 47(1):353–60. doi: 10.3892/ijo.2015.3004
50. Mukherjee A, Bilecz AJ, Lengyel E. The adipocyte microenvironment and cancer. Cancer metastasis Rev (2022) 41(3):575–87. doi: 10.1007/s10555-022-10059-x
51. Yoon H, Lee S. Fatty acid metabolism in ovarian cancer: therapeutic implications. Int J Mol Sci (2022) 23(4). doi: 10.3390/ijms23042170
52. Pascual G, Avgustinova A, Mejetta S, Martín M, Castellanos A, Attolini CS, et al. Targeting metastasis-initiating cells through the fatty acid receptor CD36. Nature (2017) 541(7635):41–5. doi: 10.1038/nature20791
53. Eckert MA, Coscia F, Chryplewicz A, Chang JW, Hernandez KM, Pan S, et al. Proteomics reveals NNMT as a master metabolic regulator of cancer-associated fibroblasts. Nature (2019) 569(7758):723–8. doi: 10.1038/s41586-019-1173-8
54. Huang D, Chowdhury S, Wang H, Savage SR, Ivey RG, Kennedy JJ, et al. Multiomic analysis identifies CPT1A as a potential therapeutic target in platinum-refractory, high-grade serous ovarian cancer. Cell Rep Med (2021) 2(12):100471. doi: 10.1016/j.xcrm.2021.100471
55. Zhu J, Wu G, Song L, Cao L, Tan Z, Tang M, et al. NKX2-8 deletion-induced reprogramming of fatty acid metabolism confers chemoresistance in epithelial ovarian cancer. EBioMedicine (2019) 43:238–52. doi: 10.1016/j.ebiom.2019.04.041
56. Nallanthighal S, Rada M, Heiserman JP, Cha J, Sage J, Zhou B, et al. Inhibition of collagen XI alpha 1-induced fatty acid oxidation triggers apoptotic cell death in cisplatin-resistant ovarian cancer. Cell Death Dis (2020) 11(4):258. doi: 10.1038/s41419-020-2442-z
57. Li J, Pan C, Boese AC, Kang J, Umano AD, Magliocca KR, et al. DGKA provides platinum resistance in ovarian cancer through activation of c-JUN-WEE1 signaling. Clin Cancer research: an Off J Am Assoc Cancer Res (2020) 26(14):3843–55. doi: 10.1158/1078-0432.Ccr-19-3790
58. Mukherjee A, Chiang CY, Daifotis HA, Nieman KM, Fahrmann JF, Lastra RR, et al. Adipocyte-induced FABP4 expression in ovarian cancer cells promotes metastasis and mediates carboplatin resistance. Cancer Res (2020) 80(8):1748–61. doi: 10.1158/0008-5472.Can-19-1999
59. Yang J, Zaman MM, Vlasakov I, Roy R, Huang L, Martin CR, et al. Adipocytes promote ovarian cancer chemoresistance. Sci Rep (2019) 9(1):13316. doi: 10.1038/s41598-019-49649-1
60. Zhang Q, Yan G, Lei J, Chen Y, Wang T, Gong J, et al. The SP1-12LOX axis promotes chemoresistance and metastasis of ovarian cancer. Mol Med (Cambridge Mass) (2020) 26(1):39. doi: 10.1186/s10020-020-00174-2
61. Huang X, Wei X, Qiao S, Zhang X, Li R, Hu S, et al. Low density lipoprotein receptor (LDLR) and 3-hydroxy-3-methylglutaryl coenzyme a reductase (HMGCR) expression are associated with platinum-resistance and prognosis in ovarian carcinoma patients. Cancer Manage Res (2021) 13:9015–24. doi: 10.2147/cmar.S337873
62. Criscuolo D, Avolio R, Calice G, Laezza C, Paladino S, Navarra G, et al. Cholesterol homeostasis modulates platinum sensitivity in human ovarian cancer. Cells (2020) 9(4). doi: 10.3390/cells9040828
63. Yang J, Peng S, Zhang K. ARL4C depletion suppresses the resistance of ovarian cancer to carboplatin by disrupting cholesterol transport and autophagy via notch-RBP-Jκ-H3K4Me3-OSBPL5. Hum Exp Toxicol (2022) 41:9603271221135064. doi: 10.1177/09603271221135064
64. Kim S, Lee M, Dhanasekaran DN, Song YS. Activation of LXRɑ/β by cholesterol in Malignant ascites promotes chemoresistance in ovarian cancer. BMC cancer (2018) 18(1):1232. doi: 10.1186/s12885-018-5152-5
65. Horala A, Plewa S, Derezinski P, Klupczynska A, Matysiak J, Nowak-Markwitz E, et al. Serum free amino acid profiling in differential diagnosis of ovarian tumors-A comparative study with review of the literature. Int J Environ Res Public Health (2021) 18(4). doi: 10.3390/ijerph18042167
66. Bachmayr-Heyda A, Aust S, Auer K, Meier SM, Schmetterer KG, Dekan S, et al. Integrative systemic and local metabolomics with impact on survival in high-grade serous ovarian cancer. Clin Cancer research: an Off J Am Assoc Cancer Res (2017) 23(8):2081–92. doi: 10.1158/1078-0432.Ccr-16-1647
67. Plewa S, Horała A, Dereziński P, Klupczynska A, Nowak-Markwitz E, Matysiak J, et al. Usefulness of amino acid profiling in ovarian cancer screening with special emphasis on their role in cancerogenesis. Int J Mol Sci (2017) 18(12). doi: 10.3390/ijms18122727
68. Prasad P, Roy SS. Glutamine regulates ovarian cancer cell migration and invasion through ETS1. Heliyon (2021) 7(5):e07064. doi: 10.1016/j.heliyon.2021.e07064
69. Gupta R, Yang Q, Dogra SK, Wajapeyee N. Serine hydroxymethyl transferase 1 stimulates pro-oncogenic cytokine expression through sialic acid to promote ovarian cancer tumor growth and progression. Oncogene (2017) 36(28):4014–24. doi: 10.1038/onc.2017.37
70. Zhang Y, Xiong X, Zhu Q, Zhang J, Chen S, Wang Y, et al. FER-mediated phosphorylation and PIK3R2 recruitment on IRS4 promotes AKT activation and tumorigenesis in ovarian cancer cells. eLife (2022) 11. doi: 10.7554/eLife.76183
71. Cruzat V, Macedo Rogero M, Noel Keane K, Curi R, Newsholme P. Glutamine: metabolism and immune function, supplementation and clinical translation. Nutrients (2018) 10(11). doi: 10.3390/nu10111564
72. Masamha CP, LaFontaine P. Molecular targeting of glutaminase sensitizes ovarian cancer cells to chemotherapy. J Cell Biochem (2018) 119(7):6136–45. doi: 10.1002/jcb.26814
73. Guo J, Satoh K, Mori M, Tomita M, Soga T. Reprogramming of glutamine metabolism via glutamine synthetase silencing induces cisplatin resistance in A2780 ovarian cancer cells. BMC cancer (2021) 21(1):174. doi: 10.1186/s12885-021-07879-5
74. Obrist F, Michels J, Durand S, Chery A, Pol J, Levesque S, et al. Metabolic vulnerability of cisplatin-resistant cancers. EMBO J (2018) 37(14). doi: 10.15252/embj.201798597
75. Liu T, Sun L, Zhang Y, Wang Y, Zheng J. Imbalanced GSH/ROS and sequential cell death. J Biochem Mol Toxicol (2022) 36(1):e22942. doi: 10.1002/jbt.22942
76. Niu B, Liao K, Zhou Y, Wen T, Quan G, Pan X, et al. Application of glutathione depletion in cancer therapy: Enhanced ROS-based therapy, ferroptosis, and chemotherapy. Biomaterials (2021) 277:121110. doi: 10.1016/j.biomaterials.2021.121110
77. Criscuolo D, Avolio R, Parri M, ROmano S, Chiarugi P, Matassa DS, et al. Decreased levels of GSH are associated with platinum resistance in high-grade serous ovarian cancer. Antioxidants (Basel Switzerland) (2022) 11(8). doi: 10.3390/antiox11081544
78. Nunes SC, Ramos C, Lopes-Coelho F, Sequeira CO, Silva F, Gouveia-Fernandes S, et al. Cysteine allows ovarian cancer cells to adapt to hypoxia and to escape from carboplatin cytotoxicity. Sci Rep (2018) 8(1):9513. doi: 10.1038/s41598-018-27753-y
79. Nunes SC, Serpa J. Glutathione in ovarian cancer: A double-edged sword. Int J Mol Sci (2018) 19(7). doi: 10.3390/ijms19071882
80. Salimian Rizi B, Caneba C, Nowicka A, Nabiyar AW, Liu X, Chen K, et al. Nitric oxide mediates metabolic coupling of omentum-derived adipose stroma to ovarian and endometrial cancer cells. Cancer Res (2015) 75(2):456–71. doi: 10.1158/0008-5472.Can-14-1337
81. You S, Zhu X, Yang Y, Du X, Song K, Zheng Q, et al. SLC7A1 overexpression is involved in energy metabolism reprogramming to induce tumor progression in epithelial ovarian cancer and is associated with immune-infiltrating cells. J Oncol (2022) 2022:5864826. doi: 10.1155/2022/5864826
Keywords: ovarian cancer, platinum resistance, metabolic reprogramming, glucose metabolism, lipid metabolism, amino acid metabolism
Citation: Yan J, Xu F, Zhou D, Zhang S, Zhang B, Meng Q and Lv Q (2023) Metabolic reprogramming of three major nutrients in platinum-resistant ovarian cancer. Front. Oncol. 13:1231460. doi: 10.3389/fonc.2023.1231460
Received: 30 May 2023; Accepted: 08 August 2023;
Published: 22 August 2023.
Edited by:
Jian-Jun Wei, Northwestern University, United StatesReviewed by:
Zhi-Bin Wang, Xiangya School of Medicine, Central South University, ChinaStephanie M. McGregor, University of Wisconsin-Madison, United States
Copyright © 2023 Yan, Xu, Zhou, Zhang, Zhang, Meng and Lv. This is an open-access article distributed under the terms of the Creative Commons Attribution License (CC BY). The use, distribution or reproduction in other forums is permitted, provided the original author(s) and the copyright owner(s) are credited and that the original publication in this journal is cited, in accordance with accepted academic practice. No use, distribution or reproduction is permitted which does not comply with these terms.
*Correspondence: Dan Zhou, emhvdWRhbl83OTExMjRAMTYzLmNvbQ==