- 1Department of Oncology, Renmin Hospital of Wuhan University, Wuhan, Hubei, China
- 2Department of Hepatobiliary Surgery, Renmin Hospital of Wuhan University, Wuhan, Hubei, China
Pancreatic acinar cell carcinoma (PACC) is a rare pancreatic malignancy with unique clinical, molecular, and morphologic features. The long-term survival of patients with PACC is substantially better than that of patients with ductal adenocarcinoma of the pancreas. Surgical resection is considered the first choice for treatment; however, there is no standard treatment option for patients with inoperable disease. The patient with metastatic PACC reported herein survived for more than 5 years with various treatments including chemotherapy, radiotherapy, antiangiogenic therapy and combined immunotherapy.
Introduction
PACC is a rare malignancy, accounting for only 1-2% of all pancreatic malignancies. The main component is morphologically similar to alveolar cells and has the ability to synthesize exocrine enzymes. The primary site of PACC can be almost any part of the pancreas, but the head of the pancreas is the most common (1–3), with masses usually 10-11 cm in diameter (4–6).
Patients with PACC often come to the hospital with nonspecific symptoms, such as abdominal pain (60%), back pain (50%), weight loss (45%), nausea and vomiting (20%), black stools (12%), weakness, anorexia and diarrhea (8%) (7, 8). Unlike ductal adenocarcinoma, PACC rarely obstructs the bile ducts (9). Some patients may also present with lipase hypersecretion syndrome, which manifests as elevated lipase levels of more than 10,000 U or more than 10,000 U/dL (10, 11). Their levels of serum tumor markers, such as carbohydrate antigen 19-9 (CA 199) and carcinoembryonic antigen (CEA), are not consistently elevated. However, the blood levels of alpha-fetoprotein (AFP) can be elevated in younger patients (1).
The prognosis of PACC is better than that of ductal carcinoma (12, 13). Previous studies have shown that the mean overall survival time is approximately 47 months for limited disease and 14 months for metastatic disease, with 5-year survival rates ranging from 36.2% to 72.8% for surgically resected individuals (12–14).
Masses are usually detected by computed tomography (CT) and magnetic resonance imaging (MRI) and are then confirmed by fine needle aspiration (FNA) biopsy. However, MRI is superior to CT in identifying tumor margins, intratumor hemorrhage, tissue infiltration, and ductal expansion (15).
There is no clear treatment option for PACC. Some studies have shown that surgical resection significantly improves the long-term survival of patients (12). However, surgery is for only limited disease. After surgical resection, there are no standard treatment guidelines, and adjuvant therapy is individualized for most patients, with individual differences. In fact, approximately 50% of patients have metastases at the time of diagnosis (16). Metastatic sites usually include the regional lymph nodes and liver, with lung, cervical lymph node and ovarian metastases being uncommon (17). Surgery is not possible for locally advanced and metastatic disease.
A growing number of studies have demonstrated the diversity of mutated genes in PACC, with APC mutations to inactivate WNT signaling and CTNNB1 mutations to activate WNT signaling found in approximately 20% of patients with PACC (18). Even mutations in genes involved in DNA repair, such as ATM, BRCA1, BRCA2, PALB2 and MSH2, have been found in a subset of patients (19, 20), mainly manifesting as genomic instability with microvolatility of 7%-14% (18, 20). In addition, there are studies reporting significant chromosomal gains and losses in PACC patients (18, 21, 22). Performing extensive molecular analysis to identify specific genetic alterations may help to improve new therapeutic ideas.
The patients with metastatic PACC reported herein survived for more than 5 years with multiple treatment modalities applied successively, suggesting that combination therapy may be a relatively promising strategy to control tumor progression.
Case presentation
A 44-year-old woman experienced intermittent back pain in October 2017. Positron emission tomography/computed tomography (PET/CT) demonstrated a primary tumor approximately 88*63 mm in size in the pancreatic corpus and tail; multiple lymph node metastases in the greater omentum, mesentery, hepato-renal space, and hepato-stomach space; and a metastase in the left lobe of the liver. The blood level of alpha-fetoprotein (AFP) was significantly increased, but those of carcinoembryonic antigen (CEA) and carbohydrate antigen (CA) 19-9 in this patient were normal. Ultrasound-guided biopsy of the pancreas was performed. The pathology diagnosis was pancreatic acinar cell carcinoma (PACC) (stage IV) (Figure 1). The results of pancreas biopsy and pathological diagnosis (November 9th, 2017) were PCK (+), EMA (partial +), CK19 (partial +), CK7 (scattered +), Syn (scattered +), and CgA (scattered +).
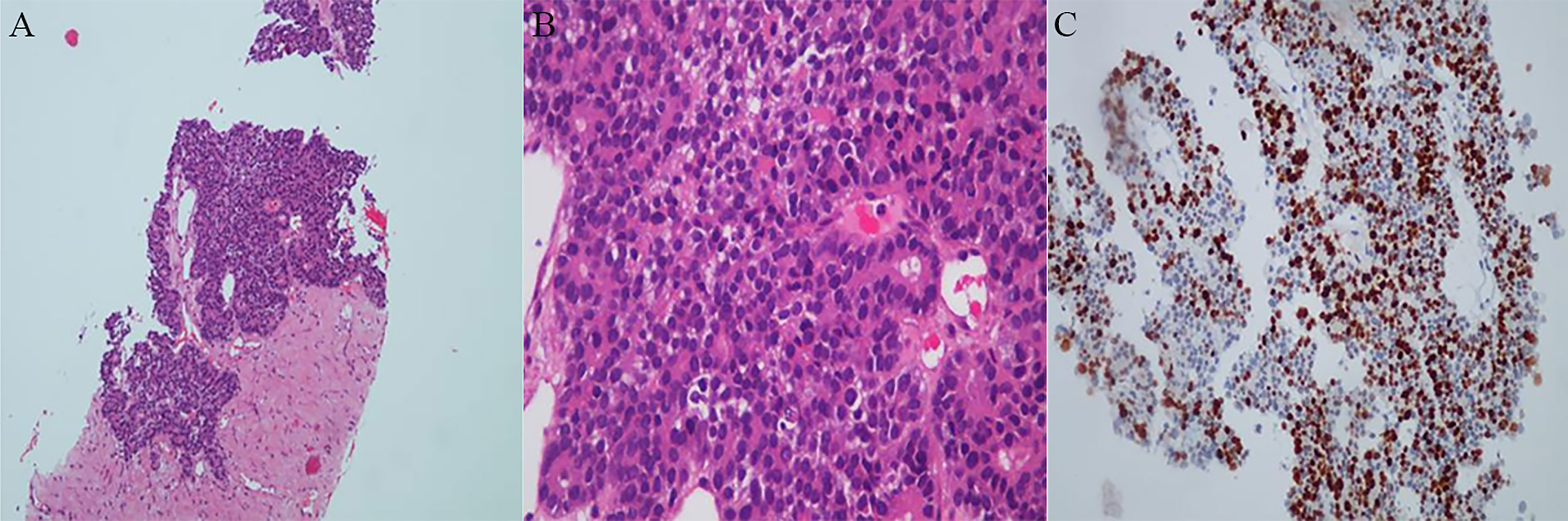
Figure 1 Histopathologic examinations of primary tumor. (A) Low magnification field of view. (B) High magnification field of view. A dense, nested or lamellar arrangement of tumor cells can be observed, some of which are in the form of vesicles or small glandular lumen structures. (C) Immunohistochemistry reaction. The results showed PCK (+), EMA (partial +), CK19 (partial +), CK7 (scattered +) Syn (scattered little +) and CgA (scattered little +).
An adjuvant GS regimen (gemcitabine, 1000 mg/m2, Day 1 and Day 8; S-1, 40 mg/d 1–14, bid, Q21d) was initiated in December 2017. The GS regimen was stopped after two cycles because of progressive disease (PD). A chemotherapy regimen consisting of oxaliplatin plus irinotecan was used for 15 cycles from January 6th, 2018, to February 20th, 2019. The patient was reviewed periodically during treatment, and she achieved a partial response (PR) based on CT scan results until an abdominal CT scan in February showed that the numbers and sizes of primary tumor and local necrosis had increased. Therefore, treatment with single-agent albumin-bound paclitaxel was initiated. Chemotherapy was stopped after 2 cycles because of further progression of the primary tumor. Subsequently, the patient was treated with an antiangiogenic therapy, oral anlotinib (10 mg, once daily from Day 1 to 14, every 3 weeks). During anlotinib treatment, the patient underwent regular re-examination, which indicated that the patient’s condition was stable and that the primary tumor gradually decreased in size. The progression-free survival (PFS) time was 23 months.
In March 2021, the patient presented with pelvic pain without an obvious cause. The serum AFP level was increased to 109.3 mmol/L. PET/CT scan showed increased number of tumors at the primary site and detected a new pelvic metastase (Figure 2). Metastatic PACC was confirmed by biopsy of the pelvic metastase.
Immunohistochemical results were Ki-67 (+20%), CD10 (−), CD56 (−), CgA (−), P53(+), PCK (+), SYN (+), CK7(+), CK20(−), COX-2(+), Villin (+), CDX-2(+), P40(−), Pax-8(−), SSTR2(+), and PD-1 (−) (Figure 3). Then, the patient was treated with radiotherapy for the pelvic metastase (24 Gy in 3 fractions) and primary tumors (4 Gy in 2 fractions). Radiotherapy began on May 25th, 2021. Meanwhile, she was treated with a PD-1 inhibitor (sintilimab, 200 mg), and recombinant human granulocyte-macrophage (rhuGM-CSF) was injected subcutaneously at a dose of 200 mg per day for 2 weeks. However, the novel anticancer oral medication had a substantially high copay per month, despite insurance coverage, making the drug unaffordable for the patient. The drug anlotinib was made accessible to the patient at no charge through support provided by the CTTQ Patient Assistance Foundation. Subsequently, the patient received anti-PD-1 therapy and antiangiogenic therapy (oral anlotinib). There was a significant decrease in the AFP level and clinical improvement to a PR for the metastatic pelvic metastase. It is important to note that all the treatments were well tolerated, with only mild toxicities. However, in August 2022, CT examination indicated disease progression with multiple metastases to the liver and bone. Figure 4 showed the image changes of the same lesion site before and after treatment. Figure 5 showed the treatment flow chart.
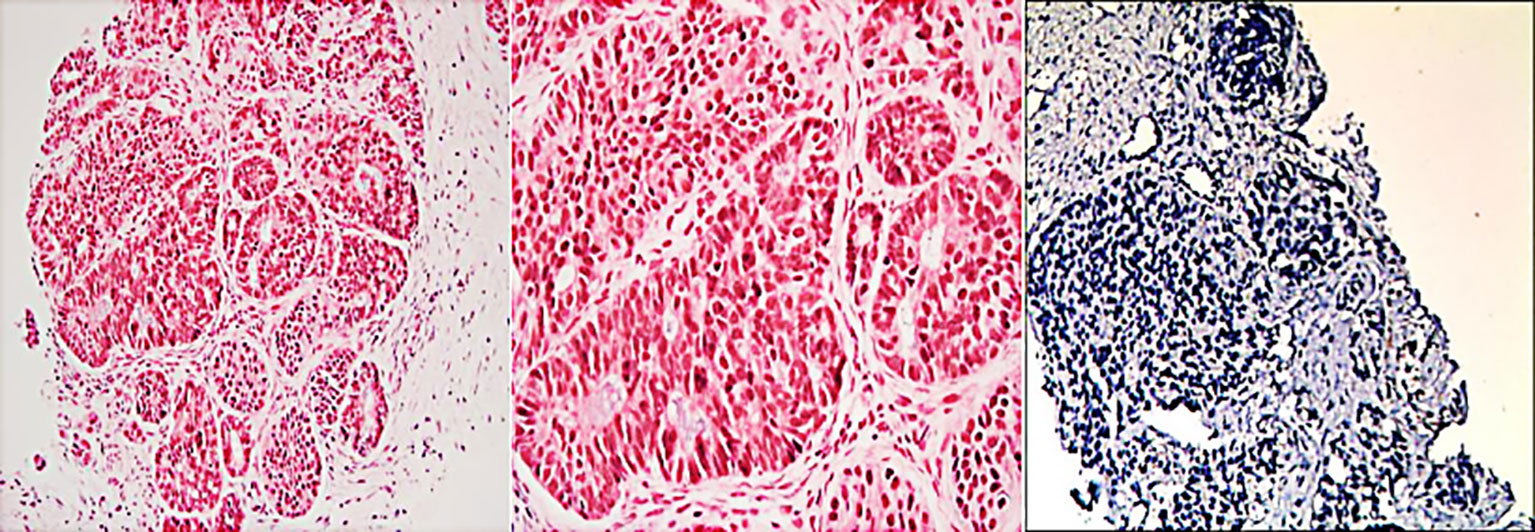
Figure 3 Histopathologic and immunohistochemical examinations of biopsy tissue from the pelvic metastase.
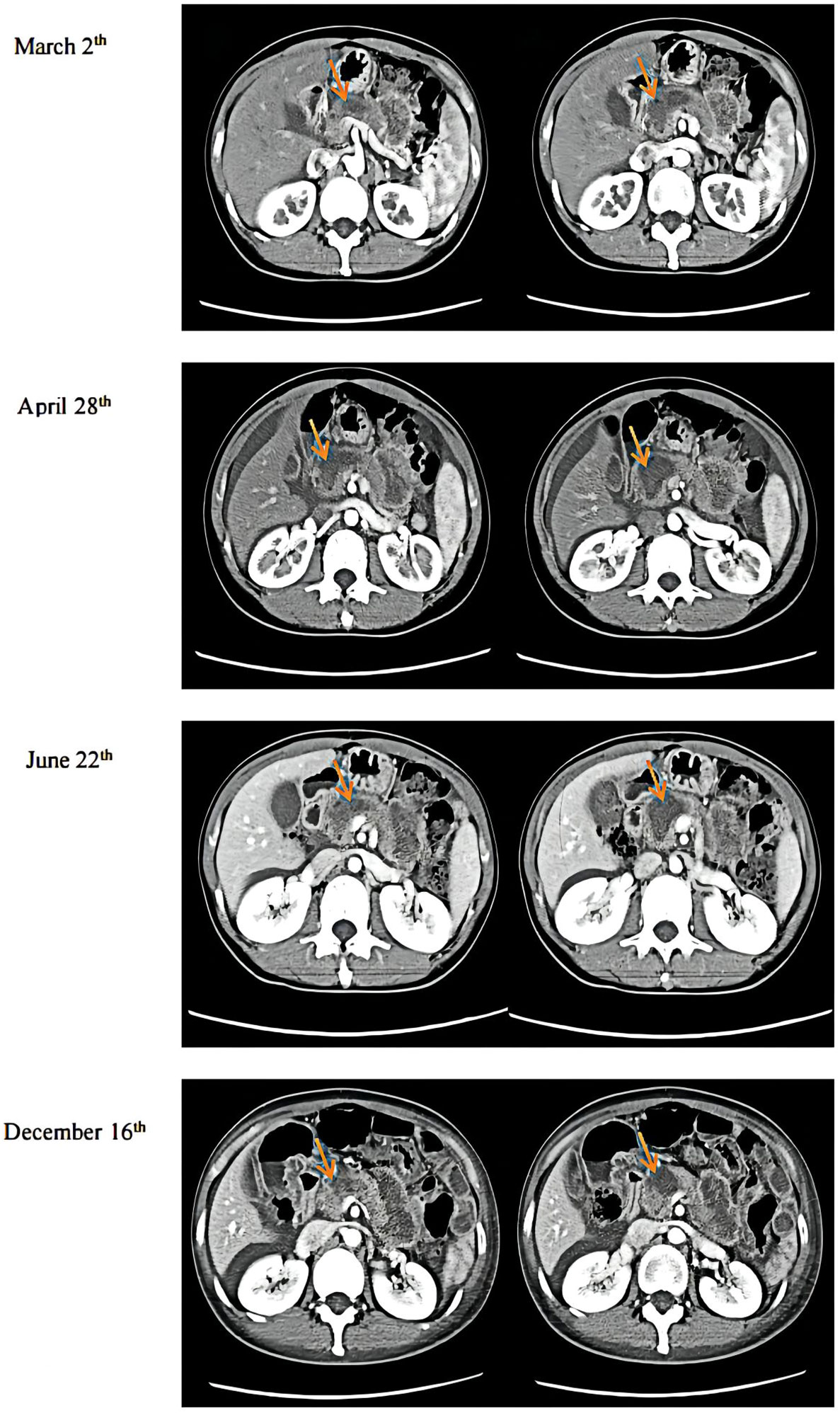
Figure 4 CT examination results. March 2nd was during anlotinib treatment. April 28th was before radiotherapy. June 22nd was after immunotherapy plus radiotherapy, and December 16th was during maintenance immunotherapy.
Genetic tests were performed on samples from the pelvic metastase, and the heterozygous mutation c.182A > G (p. Gln61Arg) was detected in the NRAS gene, while no mutations were detected in the BRCA gene, BRAF gene, NTRK gene, EGFR gene or TP53 gene (wild-type).
Discussion
The prevalence of PACC has been estimated to be below 1% among all pancreatic malignancies. More than 50% of patients with PACC have metastatic disease at diagnosis. Although the reported prognosis of PACC is better than that of pancreatic ductal adenocarcinoma (23), the prognosis remains dismal. The OS time of patients with metastatic PACC is 19.6 months (24). Many studies point out surgical resection as the most effective therapeutic strategy. The survival benefit of systemic therapy is more controversial (12, 25, 26). The patient with metastatic PACC reported herein survived over 5 years through treatment with multiple therapies including radiation therapy, chemotherapy, antiangiogenic therapy, and combined immunotherapy.
PACC has unique characteristics in terms of biological behavior, imaging and prognosis relative to PDAC, such as the AFP level in some patients (27). In our case, the change in the AFP level during therapy was useful for evaluating the benefit of treatment. However, the levels of CA 19-9 and CEA, which are predictive markers for pancreatic cancer, remained normal in our case.
Surgical resection is the mainstay of treatment for PACC. It has been shown that the median survival of patients who underwent surgery was significantly better than those who did not (36 months vs 14 months) (7). Whether adjuvant therapy is recommended for patients after surgery is controversial, and most adjuvant therapy is individualised with variable and non-representative response rates. Due to the presence of genetic variants in the APC gene/β-catenin pathway in patients with PACC, chemotherapy regimens known to be active in PDAC or colorectal cancer are often used clinically (28). Combination chemotherapy regimens based on gemcitabine or fluoropyrimidine are commonly used (10, 29). No standard chemotherapy regimen has been established for patients with unresectable or recurrent PACC because PACC is a rare cancer of the pancreas, and no large-scale randomized controlled trials have yet been conducted for this disease. This group of patients usually receives neoadjuvant or palliative 5-FU chemotherapy (30, 31). Fluoropyrimidine-based combination chemotherapy can improve disease control rates (7, 10, 24, 29, 32, 33). Patients with good fitness status are treated with folinic acid/fluorouracil/oxaliplatin or folinic acid/fluorouracil/irinotecan. In contrast, patients in poor physical condition are usually treated with gemcitabine/protein-bound paclitaxel (34). Irinotecan-containing regimens are potentially beneficial regimens for unresectable or recurrent PACC (24, 26). Yoo et al. (35) confirmed that oxaliplatin-based chemotherapy had improved activity against pancreatic ACC compared to gemcitabine. Likewise, we found that the combination of oxaliplatin plus irinotecan was associated with a better response than the gemcitabine combination in our case. Compared with the benefit of gemcitabine in PDAC, the greater clinical benefit of systemic therapy observed in PACC may be explained by the greater use of combination chemotherapy regimens incorporating oxaliplatin and irinotecan over the last decade.
Myriad mutations known to have a role in tumorigenesis have been described in several PACC series (19, 20, 24, 36, 37). PACC has a genomic profile distinct from that of PDAC, with only rare mutations in TP53, KRAS, and p16, despite mutations in these genes being common in PDAC. Approximately 20% of patients with PACC have APC or CTNNB1 which could affect WNT signalling (18). Mutations in BRCA2, PALB2, ATM, BAP1, BRAF and JAK1 are likely to occur in more than a third of PACC patients (20). Genes that are involved in DNA repair, such as ATM, BRCA1, BRCA2, PALB2 and MSH2, can result in genomic instability when mutations occur (19, 20). Microsatellite instability ranges from 7% to 14% in patients with PACC (18, 20, 38). Recurrent rearrangements of BRAF and RAF1 are also present in approximately 23% of patients (37). Of these, RAF genomic alterations are observed to be mutually exclusive with altered inactivation of DNA repair genes in 45% of PACC patients (37). In addition, it has been reported that ALK mutations occur in PACC patient (39), which is quite rare, and in only 0.16% of PDAC patients (40).
There is currently no indication for tumor multigene testing for patients with advanced PACC. However, once a druggable molecular target is detected, it may improve patient survival and quality of life. Thus, a comprehensive molecular analysis was performed and the results indicated that only NRAS mutations were found, which was quite rare. The fact is that NRAS mutations occur predominantly in melanoma and the prognosis is dismal (41). Mutations in NRAS constitutively activate intracellular signaling through multiple pathways, most notably the Ras-Raf-MAPK and PI3K-Akt pathways. Activated signaling pathways can induce cell cycle dysregulation, prosurvival pathway activation, and cell proliferation (42). However, there are currently no drugs that target NRAS, but studying the signalling pathways downstream of NRAS and thus finding druggable targets has become a potential therapeutic approach (41).
Besides, inhibition of angiogenesis is an established therapeutic strategy for many solid tumors. The results of several preclinical and clinical trials have shown that antiangiogenic therapies do not improve the efficacy of pancreatic cancer treatment (43), but these trials did not involve PAAC. Anlotinib, a novel oral multitarget tyrosine kinase inhibitor, could inhibit VEGFR, PDGFR, FGFR, C-Kit, other kinases, and tumor angiogenesis- and proliferation-related signaling pathways (44). Although it has been reported in the treatment of PDAC (45), this is the first report on anlotinib treatment in advanced metastatic PACC achieving a long-term PFS time of 23 months after failure of multiline chemotherapy.
Radiotherapy (RT) is often used to “down-stage” or convert a tumor from borderline resectable to resectable and, in general, is provided as both conventional fractionation and hypofractionated stereotactic body radiotherapy (SBRT) (29). The goal of palliative RT is often to relieve pain and bleeding and/or ameliorate local obstructive symptoms in patients with nonmetastatic or metastatic disease. Besides, RT could improve survival in the metastatic disease context, which has been well established in SCLC. A systematic review revealed a modest response rate to radiotherapy in patients with localized acinar cell carcinoma of the pancreas. In several studies, a “major response” rate was observed in 25% to 35% of these patients (7, 10). However, if stable disease was included in the definition of response (i.e., the disease control rate), significantly higher response rates were seen in highly selected studies when radiotherapy was added (7, 10).
The improvement in condition of the patient after radiotherapy according to our developed protocol may be due to the following reasons. Firstly, radiotherapy can activate the immune system and trigger an antitumor immune response after cytotoxic death and immunostimulatory signal release, increasing T-cell transport to the tumor (46, 47). Although radiotherapy significantly upregulates PD-L1 expression (48), combined anti-PD-1/PD-L1 therapy counterbalances this negative effect. SBRT is more likely to induce immunogenic death in tumor cells, promote the release and presentation of tumor-associated antigens, and generate more potent systemic antitumor effects (49). Moreover, SBRT avoids lymphocytopenia, suggesting that SBRT is a better choice for combination therapy than is conventional stepwise radiotherapy.
Low-dose radiation cannot kill the tumor itself, but it is beneficial to T-cell recruitment, stromal microenvironmental regulation, and immune function promotion (50). Low-dose radiation can also improve the systemic response rates of metastatic disease treated with high-dose radiation and immunotherapy (51). In a study of a bilateral subcutaneous tumor mouse model, more than half of the mice recovered after triple treatment. This suggests that high/low-dose radiotherapy combined with anti-PD1 antibody therapy can produce a synergistic effect, and the additional low-dose radiotherapy is well tolerated by patients (52).
Immunotherapy is still at the experimental stage in the treatment of pancreatic cancer. Pembrolizumab was first included in the NCCN guidelines as a second-line treatment only for advanced solid tumors with high microsatellite instability (MSI-H) or DNA mismatch repair deficiency (dMMR). A total of 83% of pancreatic cancer patients responded to pembrolizumab treatment, with response durations ranging from 2.6 to 9.2 months (53). Tislelizumab is a humanized monoclonal antibody with high affinity and specificity for PD-1 that was specifically designed to minimize FcγR macrophage binding to eliminate antibody-dependent phagocytosis (54).
GM-CSF can enhance the effect of immunotherapy, which is closely related to its mechanism of promoting the proliferation of dendritic cells and M1-type macrophages and enhancing antigen presentation (55). Animal studies have shown that GM-CSF combined with immune checkpoint inhibitors can improve the effectiveness of PD-1/PD-L1 inhibitors by improving antigen presentation and attracting T cells to infiltrate the tumor microenvironment (56, 57). Similarly, combination therapies have been safe and effective in advanced metastatic melanoma clinical trials. In addition, some cytokines, including GM-CSF, synergize with radiotherapy (58). Abscopal responses are defined as systemic antitumor responses outside the primary radiation field. Interestingly, we found that GM-CSF combined with RT could improve the abscopal effect in preclinical data (59). A prospective study also showed that local radiotherapy combined with GM-CSF enhanced the abscopal effect (60). A phase II trial demonstrated the safety of the combination therapies. Additionally, similar clinical trials are ongoing, such as NCT04892498.
After combining high-dose radiotherapy with GM-CSF, this patient was treated with immunotherapy and achieved good disease control. Immunotherapy may benefit from combination with radiotherapy and GM-CSF due to systemic immune response activation. We should consider whether this triple therapy could provide a more diversified treatment strategy for advanced PACC and improve the poor prognosis of this disease.
Recent studies have shown that anlotinib can downregulate the expression of PD-L1 on vascular endothelial cells to alter the tumor immune microenvironment (61). NaZhou et al. (62) designed a phase IB trial to analyze the efficacy and safety of anlotinib in combination with a PD-1 inhibitor in advanced non-small cell lung cancer. The combination therapy showed favorable efficacy and manageable toxicity, especially in the 12 mg anlotinib cohort. However, the reason why anlotinib plays a vital role in treating PACC is still unclear.
Conclusion
The rarity of PACC has led to limited recognition of the disease. Surgical resection is considered the first choice of treatment; however, there is no standard regimen for inoperable individuals. Breakthroughs in precision medicine may assist clinicians in formulating tailor-made therapies for their patients. In addition, both subtype-specific therapy and combination therapy might represent relatively promising strategies to control tumor progression.
Author contributions
JH and JG collected data. FZ and TX wrote the manuscript. XL: Conception, organization, execution and review of manuscript. All authors contributed to the article and approved the submitted version.
Funding
This research was supported by Renmin Hospital of Wuhan University Cross-Innovation Talent Project (JCRCWL-2022-003).
Conflict of interest
The authors declare that the research was conducted in the absence of any commercial or financial relationships that could be construed as a potential conflict of interest.
Publisher’s note
All claims expressed in this article are solely those of the authors and do not necessarily represent those of their affiliated organizations, or those of the publisher, the editors and the reviewers. Any product that may be evaluated in this article, or claim that may be made by its manufacturer, is not guaranteed or endorsed by the publisher.
References
1. Klimstra DS. Nonductal neoplasms of the pancreas. Mod Pathol (2007) 20 Suppl 1:S94–112. doi: 10.1038/modpathol.3800686
2. Bechade D, Desjardin M, Salmon E, Desolneux G, Becouarn Y, Evrard S, et al. Pancreatic acinar cell carcinoma. Case Rep Gastroenterol (2016) 10(1):174–80. doi: 10.1159/000445867
3. Fontenot J, Spieler B, Hudson C, Boulmay B. Pancreatic acinar cell carcinoma–literature review and case report of a 56-year-old man presenting with abdominal pain. Radiol Case Rep (2020) 15(1):39–43. doi: 10.1016/j.radcr.2019.10.009
4. Raman SP, Hruban RH, Cameron JL, Wolfgang CL, Kawamoto S, Fishman EK. Acinar cell carcinoma of the pancreas: computed tomography features–a study of 15 patients. Abdom Imaging (2013) 38(1):137–43. doi: 10.1007/s00261-012-9868-4
5. Chiou YY, Chiang JH, Hwang JI, Yen CH, Tsay SH, Chang CY. Acinar cell carcinoma of the pancreas: clinical and computed tomography manifestations. J Comput Assist Tomogr (2004) 28(2):180–6. doi: 10.1097/00004728-200403000-00005
6. Thompson ED, Wood LD. Pancreatic neoplasms with acinar differentiation: a review of pathologic and molecular features. Arch Pathol Lab Med (2020) 144(7):808–15. doi: 10.5858/arpa.2019-0472-RA
7. Holen KD, Klimstra DS, Hummer A, Gonen M, Conlon K, Brennan M, et al. Clinical characteristics and outcomes from an institutional series of acinar cell carcinoma of the pancreas and related tumors. J Clin Oncol (2002) 20(24):4673–8. doi: 10.1200/JCO.2002.02.005
8. Sridharan V, Mino-Kenudson M, Cleary JM, Rahma OE, Perez K, Clark JW, et al. Pancreatic acinar cell carcinoma: a multi-center series on clinical characteristics and treatment outcomes. Pancreatology (2021), S1424-3903(21)00162–9. doi: 10.1016/j.pan.2021.05.011
9. Klimstra DS, Heffess CS, Oertel JE, Rosai J. Acinar cell carcinoma of the pancreas. a clinicopathologic study of 28 cases. Am J Surg Pathol (1992) 16(9):815–37. doi: 10.1097/00000478-199209000-00001
10. Seth AK, Argani P, Campbell KA, Cameron JL, Pawlik TM, Schulick RD, et al. Acinar cell carcinoma of the pancreas: an institutional series of resected patients and review of the current literature. J Gastrointest Surg (2008) 12(6):1061–7. doi: 10.1007/s11605-007-0338-1
11. Burns WA, Matthews MJ, Hamosh M, Weide GV, Blum R, Johnson FB. Lipase-secreting acinar cell carcinoma of the pancreas with polyarthropathy. a light and electron microscopic, histochemical, and biochemical study. Cancer (1974) 33(4):1002–9. doi: 10.1002/1097-0142(197404)33:4<1002::aid-cncr2820330415>3.0.co;2-r
12. Schmidt CM, Matos JM, Bentrem DJ, Talamonti MS, Lillemoe KD, Bilimoria KY. Acinar cell carcinoma of the pancreas in the united states: prognostic factors and comparison to ductal adenocarcinoma. J Gastrointest Surg (2008) 12(12):2078–86. doi: 10.1007/s11605-008-0705-6
13. Wisnoski NC, Townsend CM Jr., Nealon WH, Freeman JL, Riall TS. 672 patients with acinar cell carcinoma of the pancreas: a population-based comparison to pancreatic adenocarcinoma. Surgery (2008) 144(2):141–8. doi: 10.1016/j.surg.2008.03.006
14. Kitagami H, Kondo S, Hirano S, Kawakami H, Egawa S, Tanaka M. Acinar cell carcinoma of the pancreas: clinical analysis of 115 patients from pancreatic cancer registry of Japan pancreas society. Pancreas (2007) 35(1):42–6. doi: 10.1097/mpa.0b013e31804bfbd3
15. Hsu MY, Pan KT, Chu SY, Hung CF, Wu RC, Tseng JH. CT and MRI features of acinar cell carcinoma of the pancreas with pathological correlations. Clin Radiol (2010) 65(3):223–9. doi: 10.1016/j.crad.2009.11.010
16. Kryklyva V, Haj Mohammad N, Morsink FHM, Ligtenberg MJL, Offerhaus GJA, Nagtegaal ID, et al. Pancreatic acinar cell carcinoma is associated with BRCA2 germline mutations: a case report and literature review. Cancer Biol Ther (2019) 20(7):949–55. doi: 10.1080/15384047.2019.1595274
17. Nasser F, Motta Leal Filho JM, Affonso BB, Galastri FL, Cavalcante RN, Martins DLN, et al. Liver metastases in pancreatic acinar cell carcinoma treated with selective internal radiation therapy with y-90 resin microspheres. Case Rep Hepatol (2017) 2017:1847428. doi: 10.1155/2017/1847428
18. Abraham SC, Wu TT, Hruban RH, Lee JH, Yeo CJ, Conlon K, et al. Genetic and immunohistochemical analysis of pancreatic acinar cell carcinoma: frequent allelic loss on chromosome 11p and alterations in the APC/beta-catenin pathway. Am J Pathol (2002) 160(3):953–62. doi: 10.1016/S0002-9440(10)64917-6
19. Furukawa T, Sakamoto H, Takeuchi S, Ameri M, Kuboki Y, Yamamoto T, et al. Whole exome sequencing reveals recurrent mutations in BRCA2 and FAT genes in acinar cell carcinomas of the pancreas. Sci Rep (2015) 5:8829. doi: 10.1038/srep08829
20. Jiao Y, Yonescu R, Offerhaus GJ, Klimstra DS, Maitra A, Eshleman JR, et al. Whole-exome sequencing of pancreatic neoplasms with acinar differentiation. J Pathol (2014) 232(4):428–35. doi: 10.1002/path.4310
21. Dewald GW, Smyrk TC, Thorland EC, McWilliams RR, Van Dyke DL, Keefe JG, et al. Fluorescence in situ hybridization to visualize genetic abnormalities in interphase cells of acinar cell carcinoma, ductal adenocarcinoma, and islet cell carcinoma of the pancreas. Mayo Clin Proc (2009) 84(9):801–10. doi: 10.4065/84.9.801
22. Taruscio D, Paradisi S, Zamboni G, Rigaud G, Falconi M, Scarpa A. Pancreatic acinar carcinoma shows a distinct pattern of chromosomal imbalances by comparative genomic hybridization. Genes Chromosomes Cancer (2000) 28(3):294–9. doi: 10.1002/1098-2264(200007)28:3<294::AID-GCC7>3.0.CO;2-N
23. Petrova E, Wellner J, Nording AK, Braun R, Honselmann KC, Bolm L, et al. Survival outcome and prognostic factors for pancreatic acinar cell carcinoma: retrospective analysis from the German cancer registry group. Cancers (Basel) (2021) 13(23):6121. doi: 10.3390/cancers13236121
24. Lowery MA, Klimstra DS, Shia J, Yu KH, Allen PJ, Brennan MF, et al. Acinar cell carcinoma of the pancreas: new genetic and treatment insights into a rare malignancy. Oncologist (2011) 16(12):1714–20. doi: 10.1634/theoncologist.2011-0231
25. Patel DJ, Lutfi W, Sweigert P, Eguia E, Abood G, Knab L, et al. Clinically resectable acinar cell carcinoma of the pancreas: is there a benefit to adjuvant systemic therapy? Am J Surg (2020) 219(3):522–6. doi: 10.1016/j.amjsurg.2019.10.013
26. Takahashi H, Ikeda M, Shiba S, Imaoka H, Todaka A, Shioji K, et al. Multicenter retrospective analysis of chemotherapy for advanced pancreatic acinar cell carcinoma: potential efficacy of platinum- and irinotecan-containing regimens. Pancreas (2021) 50(1):77–82. doi: 10.1097/MPA.0000000000001718
27. Nojima T, Kojima T, Kato H, Sato T, Koito K, Nagashima K. Alpha-fetoprotein-producing acinar cell carcinoma of the pancreas. Hum Pathol (1992) 23(7):828–30. doi: 10.1016/0046-8177(92)90354-6
28. Ordonez NG. Pancreatic acinar cell carcinoma. Adv Anat Pathol (2001) 8(3):144–59. doi: 10.1097/00125480-200105000-00003
29. Butturini G, Pisano M, Scarpa A, D’Onofrio M, Auriemma A, Bassi C. Aggressive approach to acinar cell carcinoma of the pancreas: a single-institution experience and a literature review. Langenbecks Arch Surg (2011) 396(3):363–9. doi: 10.1007/s00423-010-0706-2
30. Distler M, Ruckert F, Dittert DD, Stroszczynski C, Dobrowolski F, Kersting S, et al. Curative resection of a primarily unresectable acinar cell carcinoma of the pancreas after chemotherapy. World J Surg Oncol (2009) 7:22. doi: 10.1186/1477-7819-7-22
31. Antoine M, Khitrik-Palchuk M, Saif MW. Long-term survival in a patient with acinar cell carcinoma of pancreas. a case report and review of literature. JOP (2007) 8(6):783–9.
32. Matos JM, Schmidt CM, Turrini O, Agaram NP, Niedergethmann M, Saeger HD, et al. Pancreatic acinar cell carcinoma: a multi-institutional study. J Gastrointest Surg (2009) 13(8):1495–502. doi: 10.1007/s11605-009-0938-z
33. Seki Y, Okusaka T, Ikeda M, Morizane C, Ueno H. Four cases of pancreatic acinar cell carcinoma treated with gemcitabine or s-1 as a single agent. Japanese J Clin Oncol (2009) 39(11):751–5. doi: 10.1093/jjco/hyp085
34. Glazer ES, Neill KG, Frakes JM, Coppola D, Hodul PJ, Hoffe SE, et al. Systematic review and case series report of acinar cell carcinoma of the pancreas. Cancer Control (2016) 23(4):446–54. doi: 10.1177/107327481602300417
35. Yoo C, Kim BJ, Kim KP, Lee JL, Kim TW, Ryoo BY, et al. Efficacy of chemotherapy in patients with unresectable or metastatic pancreatic acinar cell carcinoma: potentially improved efficacy with oxaliplatin-containing regimen. Cancer Res Treat (2017) 49(3):759–65. doi: 10.4143/crt.2016.371
36. Bergmann F, Aulmann S, Sipos B, Kloor M, von Heydebreck A, Schweipert J, et al. Acinar cell carcinomas of the pancreas: a molecular analysis in a series of 57 cases. Virchows Arch (2014) 465(6):661–72. doi: 10.1007/s00428-014-1657-8
37. Chmielecki J, Hutchinson KE, Frampton GM, Chalmers ZR, Johnson A, Shi C, et al. Comprehensive genomic profiling of pancreatic acinar cell carcinomas identifies recurrent RAF fusions and frequent inactivation of DNA repair genes. Cancer Discovery (2014) 4(12):1398–405. doi: 10.1158/2159-8290.CD-14-0617
38. Liu K, Peng W, Zhou Z. The CT findings of pancreatic acinar cell carcinoma in five cases. Clin Imaging (2013) 37(2):302–7. doi: 10.1016/j.clinimag.2012.06.003
39. Gaule M, Pesoni C, Quinzii A, Zecchetto C, Casalino S, Merz V, et al. Exceptional clinical response to alectinib in pancreatic acinar cell carcinoma with a novel ALK-KANK4 gene fusion. JCO Precis Oncol (2022) 6:e2100400. doi: 10.1200/PO.21.00400
40. Singhi AD, Ali SM, Lacy J, Hendifar A, Nguyen K, Koo J, et al. Identification of targetable ALK rearrangements in pancreatic ductal adenocarcinoma. J Natl Compr Canc Netw (2017) 15(5):555–62. doi: 10.6004/jnccn.2017.0058
41. Randic T, Kozar I, Margue C, Utikal J, Kreis S. NRAS mutant melanoma: towards better therapies. Cancer Treat Rev (2021) 99:102238. doi: 10.1016/j.ctrv.2021.102238
42. Hodis E, Watson IR, Kryukov GV, Arold ST, Imielinski M, Theurillat JP, et al. A landscape of driver mutations in melanoma. Cell (2012) 150(2):251–63. doi: 10.1016/j.cell.2012.06.024
43. Annese T, Tamma R, Ruggieri S, Ribatti D. Angiogenesis in pancreatic cancer: pre-clinical and clinical studies. Cancers (Basel) (2019) 11(3):381. doi: 10.3390/cancers11030381
44. Lin B, Song X, Yang D, Bai D, Yao Y, Lu N. Anlotinib inhibits angiogenesis via suppressing the activation of VEGFR2, PDGFRbeta and FGFR1. Gene (2018) 654:77–86. doi: 10.1016/j.gene.2018.02.026
45. Li S, Niu M, Deng W, Li N, Wei C, Luo S. Anlotinib is effective in the treatment of advanced pancreatic cancer: a case report. Anticancer Drugs (2022) 33(1):e558–e61. doi: 10.1097/CAD.0000000000001173
46. Burnette B, Weichselbaum RR. Radiation as an immune modulator. Semin Radiat Oncol (2013) 23(4):273–80. doi: 10.1016/j.semradonc.2013.05.009
47. Formenti SC, Demaria S. Combining radiotherapy and cancer immunotherapy: a paradigm shift. J Natl Cancer Inst (2013) 105(4):256–65. doi: 10.1093/jnci/djs629
48. Oweida A, Lennon S, Calame D, Korpela S, Bhatia S, Sharma J, et al. Ionizing radiation sensitizes tumors to PD-L1 immune checkpoint blockade in orthotopic murine head and neck squamous cell carcinoma. Oncoimmunology (2017) 6(10):e1356153. doi: 10.1080/2162402X.2017.1356153
49. Palma DA, Louie AV, Rodrigues GB. New strategies in stereotactic radiotherapy for oligometastases. Clin Cancer Res (2015) 21(23):5198–204. doi: 10.1158/1078-0432.CCR-15-0822
50. Barsoumian HB, Ramapriyan R, Younes AI, Caetano MS, Menon H, Comeaux NI, et al. Low-dose radiation treatment enhances systemic antitumor immune responses by overcoming the inhibitory stroma. J Immunother Cancer (2020) 8(2):e000537. doi: 10.1136/jitc-2020-000537
51. Menon H, Chen D, Ramapriyan R, Verma V, Barsoumian HB, Cushman TR, et al. Influence of low-dose radiation on abscopal responses in patients receiving high-dose radiation and immunotherapy. J Immunother Cancer (2019) 7(1):237. doi: 10.1186/s40425-019-0718-6
52. Yin L, Xue J, Li R, Zhou L, Deng L, Chen L, et al. Effect of low-dose radiation therapy on abscopal responses to hypofractionated radiation therapy and anti-PD1 in mice and patients with non-small cell lung cancer. Int J Radiat Oncol Biol Phys (2020) 108(1):212–24. doi: 10.1016/j.ijrobp.2020.05.002
53. Lemery S, Keegan P, Pazdur R. First FDA approval agnostic of cancer site - when a biomarker defines the indication. N Engl J Med (2017) 377(15):1409–12. doi: 10.1056/NEJMp1709968
54. Dahan R, Sega E, Engelhardt J, Selby M, Korman AJ, Ravetch JV. FcgammaRs modulate the anti-tumor activity of antibodies targeting the PD-1/PD-L1 axis. Cancer Cell (2015) 28(3):285–95. doi: 10.1016/j.ccell.2015.08.004
55. van de Laar L, Coffer PJ, Woltman AM. Regulation of dendritic cell development by GM-CSF: molecular control and implications for immune homeostasis and therapy. Blood (2012) 119(15):3383–93. doi: 10.1182/blood-2011-11-370130
56. Everly JJ, Lonial S. Immunomodulatory effects of human recombinant granulocyte-macrophage colony-stimulating factor (rhuGM-CSF): evidence of antitumour activity. Expert Opin Biol Ther (2005) 5(3):293–311. doi: 10.1517/14712598.5.3.293
57. Gurbatri CR, Lia I, Vincent R, Coker C, Castro S, Treuting PM, et al. Engineered probiotics for local tumor delivery of checkpoint blockade nanobodies. Sci Transl Med (2020) 12(530):eaax0876. doi: 10.1126/scitranslmed.aax0876
58. Palata O, Hradilova Podzimkova N, Nedvedova E, Umprecht A, Sadilkova L, Palova Jelinkova L, et al. Radiotherapy in combination with cytokine treatment. Front Oncol (2019) 9:367. doi: 10.3389/fonc.2019.00367
59. Demaria S, Ng B, Devitt ML, Babb JS, Kawashima N, Liebes L, et al. Ionizing radiation inhibition of distant untreated tumors (abscopal effect) is immune mediated. Int J Radiat Oncol Biol Phys (2004) 58(3):862–70. doi: 10.1016/j.ijrobp.2003.09.012
60. Golden EB, Chhabra A, Chachoua A, Adams S, Donach M, Fenton-Kerimian M, et al. Local radiotherapy and granulocyte-macrophage colony-stimulating factor to generate abscopal responses in patients with metastatic solid tumours: a proof-of-principle trial. Lancet Oncol (2015) 16(7):795–803. doi: 10.1016/S1470-2045(15)00054-6
61. Liu S, Qin T, Liu Z, Wang J, Jia Y, Feng Y, et al. Anlotinib alters tumor immune microenvironment by downregulating PD-L1 expression on vascular endothelial cells. Cell Death Dis (2020) 11(5):309. doi: 10.1038/s41419-020-2511-3
Keywords: pancreatic acinar cell carcinoma, chemotherapy, radiotherapy, antiangiogenic therapy, immunotherapy
Citation: Zhao F, Yang D, Xu T, He J, Guo J and Li X (2023) New treatment insights into pancreatic acinar cell carcinoma: case report and literature review. Front. Oncol. 13:1210064. doi: 10.3389/fonc.2023.1210064
Received: 21 April 2023; Accepted: 20 June 2023;
Published: 03 July 2023.
Edited by:
Samir Pathak, Bristol Royal Infirmary, United KingdomReviewed by:
Valeria Merz, University of Verona, ItalySatvinder Singh Mudan, The London Clinic, United Kingdom
Copyright © 2023 Zhao, Yang, Xu, He, Guo and Li. This is an open-access article distributed under the terms of the Creative Commons Attribution License (CC BY). The use, distribution or reproduction in other forums is permitted, provided the original author(s) and the copyright owner(s) are credited and that the original publication in this journal is cited, in accordance with accepted academic practice. No use, distribution or reproduction is permitted which does not comply with these terms.
*Correspondence: Xiangpan Li, cm0wMDEyMjdAd2h1LmVkdS5jbg==
†These authors have contributed equally to this work