- 1Department of Hematology, the First Affiliated Hospital of Kunming Medical University, Kunming, China
- 2Hematology Research Center of Yunnan Province, Kunming, China
- 3Department of Clinical Laboratory, First Affiliated Hospital of Kunming Medical University, Kunming, China
The occurrence and development of malignancies are closely related to abnormal cell cycle regulation. Myeloid leukemia factor 1 (MLF1) is a small nucleocytoplasmic shuttling protein associated with cell cycle exit, apoptosis, and certain immune functions. Therefore, it is pertinent to explore the role of MLF1 in health and diseases. Studies to date have suggested that MLF1 could act as a double-edged sword, regulating biochemical activities directly or indirectly. In hematopoietic cells, it serves as a protective factor for the development of lineages, and in malignancies, it serves as an oncogenesis factor. The diversity of its functions depends on the binding partners, including tumor inhibitors, scaffolding molecules, mitochondrial membrane proteins, and transcription factors. Emerging evidence indicates that MLF1 influences immune responses as well. This paper reviews the structure, biological function, and research progress on MLF1 in health and diseases to provide new insights for future research.
Introduction
Myeloid leukemia factor (MLF) is a poorly characterized family of conserved proteins which earliest member, myeloid leukemia factor 1 (MLF1), is associated with hemopoietic lineage commitment and malignancies. MLF1 has so far been shown to be a double-edged sword, acting as either a tumor suppressor or an oncogene, depending on the context of the cell. MLF1 has been initially described in the leukemic fusion protein NPM-MLF1, which is generated by a rare t(3;5)(q25.1;q34) chromosomal translocation in patients with acute myeloid leukemia (AML) (1), and implicated in the development of AML and myelodysplastic syndrome (MDS) (2). Although the role of NPM in the pathogenesis of leukemia has been well studied (3–6), the contribution of MLF1 to normal hematopoiesis and oncogenesis has not been adequately characterized. Several studies have demonstrated that MLF1 can regulate cell cycle exit and differentiation, promote apoptosis, inhibit proliferation in various cell types, enhance immune function, or impair the lymphocyte population. However, its biochemical activity remains largely unclear. Up to now, no systematic overview of MLF1 studies in pathology and physiology has been published. In this review, we summarized current knowledge of MLF1 and provided a valuable reference for future research.
MLF1 structure and function
MLF1 gene is located on human chromosome 3 and encodes MLF1 protein and its isoforms (7). MLF1 protein is a small nucleocytoplasmic shuttling protein (268 amino acids), which has a functional N-terminal nuclear export signal (NES) and two C-terminal nuclear localization signals (NLS), allowing MLF1 to shuttle between the nucleus and the cytoplasm (8, 9). MLF1 has a characterized central domain preserved within the MLF family (10, 11), comprising two identifiable motifs that bind to 14-3-3 protein and the COP9 signalosome by Ser34 and subunit 3 (CNS3), and a SAM domain, which is involved in many different biological processes and has RNA binding properties (12). Above features of MLF1 are summarized in Figure 1. MLF is highly conserved across species from Drosophila, murine, and shrimp to humans (13–15). The phenotypic defects associated with MLF loss in Drosophila can be rescued by human MLF1 (16). MLF overexpression reduces Drosophila wing and eye size (17), which is demonstrated by the fact that MLF activates the bsk-JNK pathway by interacting with DREF (18). Additionally, overexpressed MLF causes abnormal DNA synthesis in Drosophila (19). Enforced expression of murine MLF1 suppresses a rise in the cell cycle inhibitor p27Kip1 to disturb the development and the differentiation of erythrocytes (20, 21). Microarray analysis performed with MLF1-expressing cells has concluded that MLF1, when expressed in the nucleus, inhibited calcium cycle proteins and CR6 (cytokine response protein) associated with differentiation and growth arrest (8). Immune function is also associated with MLF1. It has been identified in kuruma shrimp and characterized as MjMLF, which plays a critical role both in antiviral and antibacterial immunity. MjMLF could inhibit the lethal white spot syndrome virus (WSSV) replication in vivo and accelerate Vibrio anguillarum, a gram-negative bacteria, clearance in shrimp (22, 23). In contrast, a study about lymphoma has shown that the overexpression of MLF1 increases lymphocyte apoptosis in vitro (13). Furthermore, MLF1 absence is consistently associated with the expansion of B- and T-cell numbers in the spleen (24). These findings imply that MLF1 might function as a context-dependent factor involved in the regulation of normal physiological processes and that its absence or overexpression leads to disease.
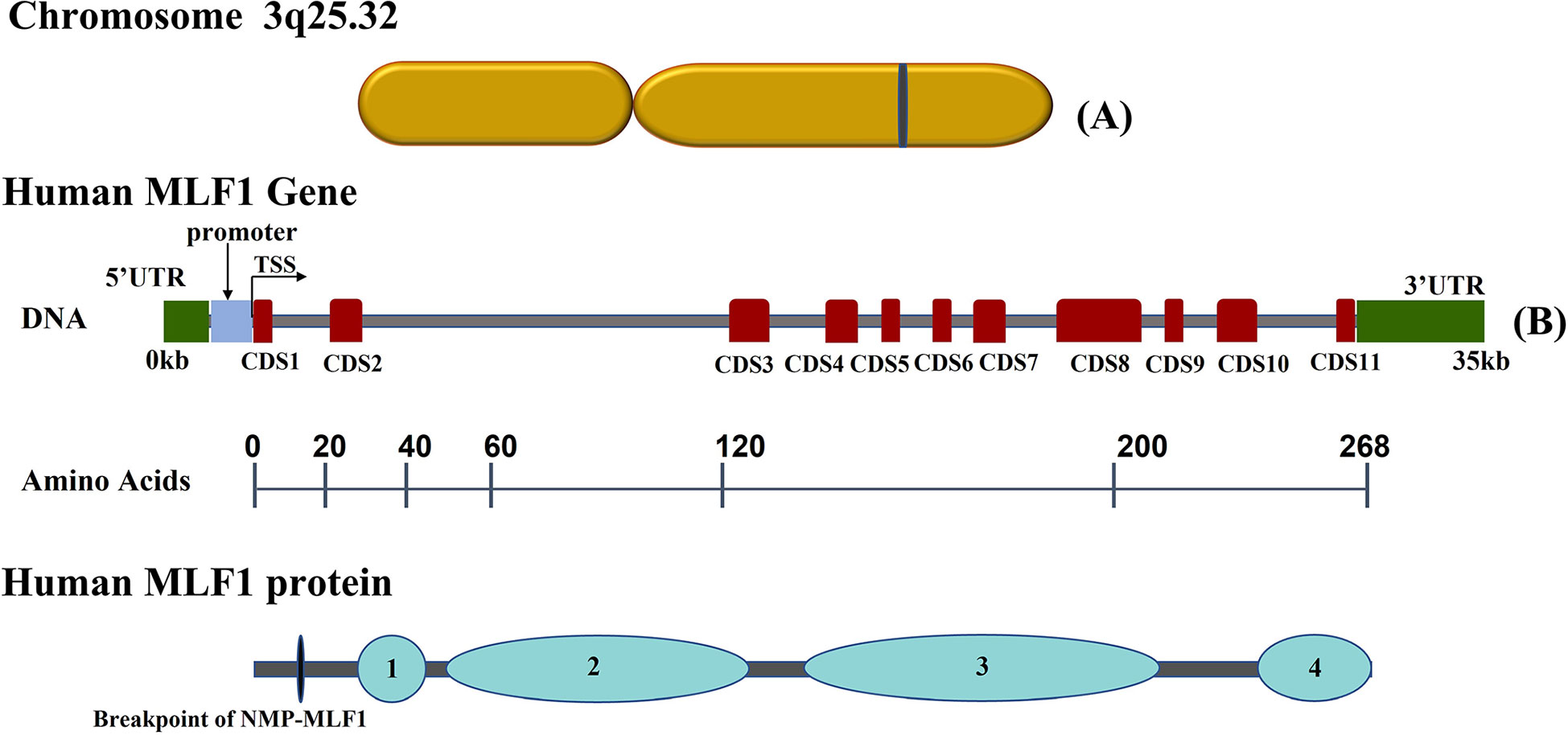
Figure 1 Schematic representation of human Myeloid Leukemia Factor 1 (MLF1) chromosome, gene and corresponding protein domains: (A) Diagram of MLF1 gene chromosome location. (B) The grey horizontal line represents the DNA sequence, red boxes on the sequence represent coding sequences (CDS) in MLF1, and two green boxes at the ends indicate UTRs. Underneath, the numbers over the black lines indicate the amino acid positions, which correspond to human MLF1 protein domains ([1] 14-3-3 protein binding domain (Ser34); [2] the COP9 signalosome subunit 3(CNS3) binding domain; [3] MLF family characteristic domain; [4] a SAM domain). All sequences were obtained from the NCBI database.
MLF1 and its distribution
MLF1 is widely expressed in different tissues. It is highly presented in the testis, heart, lung, brain, thyroid gland, gall bladder, kidney, and digestive system and is expressed to some extent in human bone marrow, spleen, and lymph nodes (25). At the cellular level, MLF1 transcripts are dominantly expressed in CD34+ cells but only slightly in GlyA+, CD3+, CD19+, or CD14+ cells and granulocytes (2). These facts indicate that the expression of MLF1 in CD34+ progenitor cells decreases during differentiation to each lineage, especially toward the myeloid and erythroid lineage (15). Cells at an early stage seem to need MLF1. At the subcellular level, MLF1 is mostly found in the cytoplasm. The apoptosis-inducing domain contained in MLF1 is unique because it requires dimerization and nuclear transportation to induce cell death, whereas most of the well-known ‘death domains’ function in the cytoplasm (26). However, the relationship between the increased accumulation of MLF1 in the cytoplasm and diseases is still unclear. Notably, a functional NES sequence is important for both MLF1 protein and NMP-MLF1 fusion protein to exert proleukemic effects. Additionally, studies have demonstrated that an MLF1 mutant containing only NES sequence inhibited proliferation more strongly than WT protein (9, 27). The contribution of NPM to NPM-MLF1-induced leukemogenesis is debatable (28), whereas NPM-MLF1 fusion protein without NES sequence loses oncogenic transformation ability (9). However, the regulatory mechanisms of the abnormal localization of MLF1 in the nucleus remain unknown.
MLF1 networks
MLF1 plays an essential role in cell development by interacting with multiple factors, which are summarized in Figure 2.
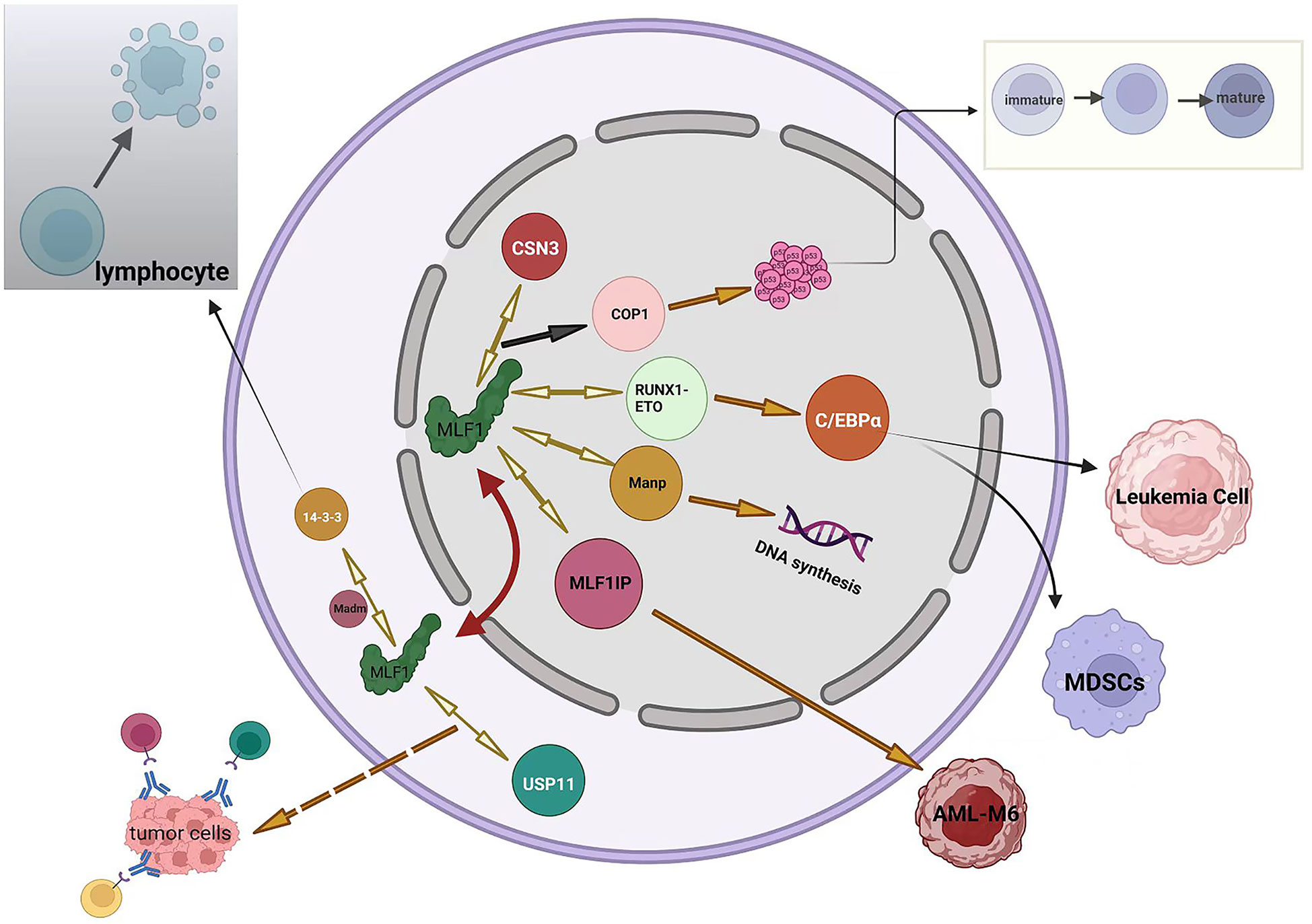
Figure 2 Summary of MLF1 networks. Black arrows represent negative regulation (including inhibition or downregulation), orange arrows represent positive regulation (including promotion or upregulation), orange dotted arrow represents speculation, and bidirectional yellow arrows indicate proteins interacting with MLF1 protein. The red two-way arrow illustrates the shuttling of MLF1 between the nucleus and the cytoplasm.
1. MLF1 in cell development and apoptosis. MLF1, shuttling from the cytoplasm to the nucleus, binds COP9 subunit 3 (CSN3), which leads to the downregulation of COP1; therefore, the cell cycle of hematopoietic cells becomes arrested because the bonding accelerates the accumulation of wild-type p53 in the nucleus (29). The above-mentioned process has also been demonstrated in Drosophila (17). Tumor suppressor p53 remains a vital mechanism of inhibiting tumor escape from apoptosis, and emerging evidence suggests that mutant p53 also promotes inflammation and supports tumor immune evasion (30, 31). Yoneda-Kato et al. have demonstrated that the MLF1-induced growth arrest depended on the integrity of the p53 allele (29). This raises the question of whether MLF1 still acts as a protective mechanism when p53 is mutated or if it enhances the oncogenicity of mutated p53. Overexpression of MLF1 promotes apoptotic death of the cells but is negatively regulated by 14-3-3 protein blocking its Bcl-XL homology domain 3 (BH3), which prevents the cell from apoptosis (32, 33). 14-3-3 (RSXSXP) motifs are involved in important cell processes, such as death, differentiation, and division (34–36). Bcl-XL, a Bcl-2 family member, maintains a fully functional immune system that ensures an efficient clearance of senescent cells (37). The above-presented conclusion has been obtained in lymphocytes, suggesting that MLF1 is required for lymphocytes to respond to apoptotic stimulations. Additionally, the nuclear content of MLF1 is also regulated by 14-3-3 protein, which sequesters MLF1 in the murine cytoplasm (32), However, another study has suggested the opposite conclusion that the distribution of full-length human MLF1 is 14-3-3 protein-independent (38). Therefore, the subcellular localization of MLF1 is probably regulated by other unknown proteins. A yeast two-hybrid screen has identified that MLF1 binds with an adaptor, which contains a 220-bp cDNA fragment and several potential phosphorylation sites in the vicinity of both the NLS and NES. At the time of its isolation, it had no homology to sequences in the database and was named Madm for MLF1-adaptor molecule. Madm mediates phosphorylation of 14-3-3 binding site of MLF1, which then immunoprecipitates and localizes to the cytoplasm. Thus, Madm might regulate the localization of MLF1 in the cytoplasm. In contrast to MLF1, which promotes the maturation, ectopic expression of Madm suppresses differentiation in myeloid cells (32). Louise N et al. have reported that MLF1 interacts with Manp, also known as scaffold attachment factor-A (SAF-A), which is a member of the heterogeneous nuclear ribonucleoprotein (hnRNP) family and homologous to hnRNP-U. Manp localizes exclusively in the nucleus and redirects MLF1 into the nucleus (8). Recent studies have suggested that hnRNP-U regulates DNA replication, organizes large-scale chromosome structures, and protects the genome from instability (39–41). The effects of MLF on DNA synthesis have been previously discussed (19). However, the relationship between MLF1 and hn-RNP in DNA synthesis remains unclear.
2. MLF1 in immune function and leukemia. In a drosophila model of leukemia, MLF has been demonstrated to control the development of hematopoietic stem cells by stabilizing the RUNX transcription factor Lozenge (LZ). MLF controls LZ activity and prevents its degradation, which is critical to control crystal cell number in the fly (42). Further study has shown that MLF and DnaJ-1 interact through conserved domains to form a chaperone complex that directly regulates LZ activity. Importantly, the interaction controls RUNX transcription factor activity and Notch signaling during blood cell development in vivo (43). RUNX members are key regulators of hematopoiesis; particularly, RUNX1 functions as a positive regulator for definitive hematopoietic stem cell emergence and megakaryocyte and lymphocyte differentiation (44). RUNX1-ETO, the mutant and infusion form of the RUNX1 protein, has been identified in cancer. MLF1 stabilizes the human oncogenic fusion protein RUNX1-ETO. Further study has indicated that MLF1 impairs RUNX1-ETO accumulation and reduces RUNX1-ETO-dependent leukemia cell proliferation (42). It is reasonable to conclude that MLF1 functions as a tumor suppressor gene in leukemia. However, the expression level of MLF1 in healthy adults’ bone marrow is not as high as expected. Moreover, high expression of MLF1 is associated with poor prognosis for AML and MDS (2). To some extent, MLF1 is required to inhibit the development of leukemia. However, it does not always appear to be a protective factor, and when leukemia is developed, MLF1 is positively correlated with leukemia (2). Reasonably, it can be inferred that MLF1 is a context-dependent gene, with its elevated expression being associated with leukemia promotion and suppression in different settings. CCAAT/enhancer-binding protein-α (C/EBPα) is a key transcription factor regulating myeloid differentiation in normal hematopoiesis and is frequently dysregulated in AML (45). Studies have shown that Trib1 and RUNX1-ETO downregulate C/EBPa and induce AML in mouse models (46, 47). MLF1 treatment upregulates the level of C/EBPα by suppressing Trib1 or RUNX1-ETO, which causes the inactivation of myeloid-derived suppressor cells (MDSCs) with potent antitumor responses across different tumor models and cancer patients (48). In mouse or leukemia cell models, the distribution of C/EBPa is paralleled with MLF1 (49). MLF1-interacting protein (MLF1IP), also known as PB1P1, KLIP1, KLP1, CENP-U, and CENP-50, specifically binds with MLF1, as shown by yeast two-hybrid analysis and pulldown assays (50). MLF1IP differs from MLF1 without other known protein homology. MLF1IP is a centromere-binding protein (51) that shows 25% identity to the SMC family of proteins and some homology to myosin, which is involved in actin cytoskeletal organization (52). MLF1IP may be an erythroid lineage-specific gene, as it is expressed exclusively in CFU-E erythroid precursor cells but not in mature erythrocytes (50, 53). MLF1 drives the occurrence of erythroleukemia as well (20, 21). A study has found that NMP-MLF1 infusion protein is more likely to occur in M6 (according to FAB classification) patients than in other leukemia types (54). Therefore, the interaction between MLF1IP and MLF1 will most likely play a role in the occurrence of M6. High expression of MLF1IP is associated with poor prognosis in several cancers, such as breast cancer, glioma, and diffuse large B-cell lymphoma (DLBC) (55). Furthermore, MLF1IP also plays a role in the development of the immune system (56). However, the functional consequences of MLF1 and MLF1IP interaction remain largely unknown. HAX-1, a 35-kDa inner mitochondrial membrane protein, functions as an anti-apoptosis protein (57), and its deficiency and overexpression result in the loss of lymphocytes and tumorigenesis, respectively (58, 59). This expression balance of MLF1 is also critical for its function. MLF1 has been recently revealed to directly associate with HAX-1 by co-immunoprecipitation assay. Animal experiments have confirmed that the two have interaction, and severe splenocyte and thymocyte lymphopenia in Hax1−/− mice can be reversed by MLF1 deficiency (13). However, it is unclear whether their effects on lymphocytes are synergistic or antagonistic. As of now, despite conflicting evidence, the relationship between MLF1 and immune function has not been adequately investigated. Further research is required to clarify this issue.
3. MLF1 in antitumor protection. MLF1 protein is directly associated with the deubiquitinase ubiquitin-specific peptidase 11 (USP11), which is a promising therapeutic target. Additionally, USP11 has promoted the accumulation of MLF2 in all tested cells (60), whereas MLF1 and MLF2 are approximately 40% similar (11). USP11 plays a dual role in the development of tumors (61, 62). Based on the studies conducted so far, MLF1 may act as both a tumor suppressor and tumor oncogene, depending on the context of the cell. It is worth mentioning that MLF1 is a positive factor in various biological processes, such as progenitor cell development and tumor regression. Whether MLF1, together with upregulated USP11 protein, enhances antitumor ability still needs further research.
MLF1 and disease
MLF1 functions as a double-edged sword in various diseases. An early clinical study has found that t(3;5) is more likely to occur in M6 patients than in patients with other leukemia types (54). A preclinical study has confirmed that MLF1 expression drives the occurrence of erythroleukemia (20, 21). A significantly higher level of MLF1 expression is detected in over 25% of patients with immature AML subtypes and higher malignant MDS (2). MLF1 is also upregulated in lung squamous cell carcinoma and esophageal carcinomas (63, 64). MLF1 overexpression results in aggregate formation; however, there is still controversy over the cause and effect of protein aggregate in neurodegenerative diseases (65). The whole-exome sequencing of small intestine neuroendocrine tumors has revealed that MLF1 is therapeutically relevant (66). The presence of MLF1 protein inhibits apoptosis caused by neurotoxicity induced by Huntingtin (HTT) aggregates (67). An extensive genome-wide association study (GWAS) has indicated that MLF1 expression is high in neuroblastoma and that silenced MLF1 significantly suppresses tumor proliferation (68). Recent research in the heart has shown that the increased expression of MLF1 leads to accelerated apoptosis and reduced cardiac cell proliferation (69). However, an aberrant downregulation of MLF1 is also related to tumorigenesis. Aberrant DNA methylation plays a significant role and is extensive (70), as indicated by the higher incidence of aberrant DNA methylation of known tumor-suppressor genes than that of mutations (71). MLF1 is methylation-silenced in the gastric cancer cell line and is upregulated 27-fold after 5-AZA-dC treatment. There is a possibility that MLF1 silencing is causally related to the development and progression of gastric cancer (72, 73). A comparative study has identified that MLF1 is also a methylation marker for the detection of early gastric neoplasia and field cancerization (74). Shuang Zhao et al. have found that the expression levels of MLF1 were downregulated in tumor tissues compared to normal tissues, which suggested that MLF1 influences tumor initiation and progression in nasopharyngeal carcinoma (75). Defects in the centrosome and cilium are associated with a set of human diseases. Ramona A. Hoh et al. have found that MLF1 was associated with diseases, was upregulated during ciliogenesis, and localized to centrioles and cilia (76). Hypermethylated MLF1 gene in mantle cell lymphoma (MCL) has been confirmed by genome-wide DNA methylation analysis, and aberrant methylation is associated with inverse changes in mRNA levels (77). Marcela B. Mansur et al. have identified a recurrent somatic deletion on chromosome 3. This loss results in the complete deletion of MLF1 and has not been previously described in infant T-cell acute lymphoblastic leukemia (78).
Conclusion and future perspectives
In conclusion, MLF1 is a small shuttling protein playing a critical role in biological and pathological processes. Currently, research regarding MLF1 has mainly focused on cancer development, which is still an obscure and disputed topic. In general, although there is more evidence supporting the point that MLF1 contributes to tumor suppression, a few studies have confirmed the tumorigenesis of MLF1 in solid and hematologic tumors, which cannot be neglected. Additionally, the functions of MLF1 in immune response still need further investigations despite some already reported studies. Given the complexity and variety of involved proteins, we may draw a conclusion that MLF1 might be a double-edged factor in the regulation of cell cycle, immunity, stem cell development, and cancer. However, studies about MLF1 are still inadequate; therefore, expanding the research on MLF1 is significant and may enrich the knowledge of MLF1 in the above-mentioned conditions. On the other hand, exploring regulations of MLF1 shuttling will provide a better understanding of MLF1, which helps develop novel specific MLF1-aimed drugs that might provide a promising strategy for cancer treatment, as well as other pathologies, such as neurological diseases. Therefore, analysis of the partner protein, localization, and shuttling mechanism might provide new insights for future research.
Author contributions
All authors contributed to the article and approved the submitted version.
Funding
The present study was supported by the National Natural Science Foundation of China (No. 81760035).
Conflict of interest
The authors declare that the research was conducted in the absence of any commercial or financial relationships that could be construed as a potential conflict of interest.
Publisher’s note
All claims expressed in this article are solely those of the authors and do not necessarily represent those of their affiliated organizations, or those of the publisher, the editors and the reviewers. Any product that may be evaluated in this article, or claim that may be made by its manufacturer, is not guaranteed or endorsed by the publisher.
References
1. Yoneda-Kato N, Look AT, Kirstein MN, Valentine MB, Raimondi SC, Cohen KJ, et al. The T (3,5)(Q25.1;Q34) of myelodysplastic syndrome and acute myeloid leukemia produces a novel fusion gene, npm-Mlf1. Oncogene (1996) 12(2):265–75.
2. Matsumoto N, Yoneda-Kato N, Iguchi T, Kishimoto Y, Kyo T, Sawada H, et al. Elevated Mlf1 expression correlates with malignant progression from myelodysplastic syndrome. Leukemia (2000) 14(10):1757–65. doi: 10.1038/sj.leu.2401897
3. Falini B, Mecucci C, Tiacci E, Alcalay M, Rosati R, Pasqualucci L, et al. Cytoplasmic nucleophosmin in acute myelogenous leukemia with a normal karyotype. New Engl J Med (2005) 352(3):254–66. doi: 10.1056/NEJMoa041974
4. Grisendi S, Pandolfi PP. Npm mutations in acute myelogenous leukemia. New Engl J Med (2005) 352(3):291–2. doi: 10.1056/NEJMe048337
5. Nakagawa M, Kameoka Y, Suzuki R. Nucleophosmin in acute myelogenous leukemia. New Engl J Med (2005) 352(17):1819–20. doi: 10.1056/NEJM200504283521719
6. Wang R, Xu P, Chang L-L, Zhang S-Z, Zhu H-H. Targeted therapy in Npm1-mutated aml: Knowns and unknowns. Front In Oncol (2022) 12:972606. doi: 10.3389/fonc.2022.972606
7. Muzny DM, Scherer SE, Kaul R, Wang J, Yu J, Sudbrak R, et al. The DNA sequence, annotation and analysis of human chromosome 3. Nature (2006) 440(7088):1194–8. doi: 10.1038/nature04728
8. Winteringham LN, Endersby R, Kobelke S, McCulloch RK, Williams JH, Stillitano J, et al. Myeloid leukemia factor 1 associates with a novel heterogeneous nuclear ribonucleoprotein U-like molecule. J Biol Chem (2006) 281(50):38791–800. doi: 10.1074/jbc.M605401200
9. Yoneda-Kato N, Kato J-Y. Shuttling imbalance of Mlf1 results in P53 instability and increases susceptibility to oncogenic transformation. Mol Cell Biol (2008) 28(1):422–34. doi: 10.1128/MCB.02335-06
10. Gobert V, Haenlin M, Waltzer L. Myeloid leukemia factor: A return ticket from human leukemia to fly hematopoiesis. Transcription (2012) 3(5):250–4. doi: 10.4161/trns.21490
11. Kuefer MU, Look AT, Williams DC, Valentine V, Naeve CW, Behm FG, et al. Cdna cloning, tissue distribution, and chromosomal localization of Myelodysplasia/Myeloid leukemia factor 2 (Mlf2). Genomics (1996) 35(2):392–6. doi: 10.1006/geno.1996.0376
12. Kim CA, Bowie JU. Sam Domains: Uniform structure, diversity of function. Trends In Biochem Sci (2003) 28(12):625–8. doi: 10.1016/j.tibs.2003.11.001
13. Sun Y, Chao J-R, Xu W, Pourpak A, Boyd K, Moshiach S, et al. Mlf1 is a proapoptotic antagonist of hop complex-mediated survival. Biochim Et Biophys Acta Mol Cell Res (2017) 1864(4):719–27. doi: 10.1016/j.bbamcr.2017.01.016
14. Ohno K, Takahashi Y, Hirose F, Inoue YH, Taguchi O, Nishida Y, et al. Characterization of a drosophila homologue of the human Myelodysplasia/Myeloid leukemia factor (Mlf). Gene (2000) 260(1-2):133–43. doi: 10.1016/S0378-1119(00)00447-9
15. Hitzler JK, Witte DP, Jenkins NA, Copeland NG, Gilbert DJ, Naeve CW, et al. Cdna cloning, expression pattern, and chromosomal localization of Mlf1, murine homologue of a gene involved in myelodysplasia and acute myeloid leukemia. Am J Pathol (1999) 155(1):53–9. doi: 10.1016/S0002-9440(10)65098-5
16. Martin-Lannerée S, Lasbleiz C, Sanial M, Fouix S, Besse F, Tricoire H, et al. Characterization of the drosophila myeloid leukemia factor. Genes To Cells: Devoted To Mol Cell Mech (2006) 11(12):1317–35. doi: 10.1111/j.1365-2443.2006.01023.x
17. Sugano W, Ohno K, Yoneda-Kato N, Kato J-Y, Yamaguchi M. The myeloid leukemia factor interacts with Cop9 signalosome subunit 3 in drosophila melanogaster. FEBS J (2008) 275(3):588–600. doi: 10.1111/j.1742-4658.2007.06229.x
18. Yanai H, Yoshioka Y, Yoshida H, Nakao Y, Plessis A, Yamaguchi M. Drosophila myeloid leukemia factor acts with dref to activate the jnk signaling pathway. Oncogenesis (2014) 3:e98. doi: 10.1038/oncsis.2014.13
19. Fouix S, Martin-Lannerée S, Sanial M, Morla L, Lamour-Isnard C, Plessis A. Over-expression of a novel nuclear interactor of suppressor of fused, the drosophila Myelodysplasia/Myeloid leukaemia factor, induces abnormal morphogenesis associated with increased apoptosis and DNA synthesis. Genes To Cells (2003) 8(11):897–911. doi: 10.1046/j.1365-2443.2003.00685.x
20. Williams JH, Daly LN, Ingley E, Beaumont JG, Tilbrook PA, Lalonde JP, et al. Hls7, a hemopoietic lineage switch gene homologous to the leukemia-inducing gene Mlf1. EMBO J (1999) 18(20):5559–66. doi: 10.1093/emboj/18.20.5559
21. Winteringham LN, Kobelke S, Williams JH, Ingley E, Klinken SP. Myeloid leukemia factor 1 inhibits erythropoietin-induced differentiation, cell cycle exit and P27kip1 accumulation. Oncogene (2004) 23(29):5105–9. doi: 10.1038/sj.onc.1207661
22. Feng X-W, Huo L-J, Sun J-J, Xu J-D, Niu G-J, Wang J-X, et al. Myeloid leukemia factor functions in anti-wssv immune reaction of kuruma shrimp, marsupenaeus japonicus. Fish Shellfish Immunol (2017) 70:416–25. doi: 10.1016/j.fsi.2017.09.028
23. Feng XW, Huo LJ, Shi XZ. Myeloid leukemia factor participates in the antibacterial immune reaction of kuruma shrimp marsupenaeus japonicus. Invertebrate Survival J (2018) 15:203–9. doi: 10.25431/1824-307X/isj.v15i1.203-209
24. Sun Y, Fu A, Xu W, Chao J-R, Moshiach S, Morris SW. Myeloid leukemia factor 1 interfered with bcl-xl to promote apoptosis and its function was regulated by 14-3-3. J Physiol Biochem (2015) 71(4):807–21. doi: 10.1007/s13105-015-0445-5
25. Fagerberg L, Hallström BM, Oksvold P, Kampf C, Djureinovic D, Odeberg J, et al. Analysis of the human tissue-specific expression by genome-wide integration of transcriptomics and antibody-based proteomics. Mol Cell Proteomics (2014) 13(2):397–406. doi: 10.1074/mcp.M113.035600
26. Ashkenazi A, Dixit VM. Death receptors: Signaling and modulation. Science (1998) 281(5381):1305–8. doi: 10.1126/science.281.5381.1305
27. Yoneda-Kato N, Fukuhara S, Kato J. Apoptosis induced by the myelodysplastic syndrome-associated npm-Mlf1 chimeric protein. Oncogene (1999) 18(25):3716–24. doi: 10.1038/sj.onc.1202711
28. Falini B, Nicoletti I, Bolli N, Martelli MP, Liso A, Gorello P, et al. Translocations and mutations involving the nucleophosmin (Npm1) gene in lymphomas and leukemias. Haematologica (2007) 92(4):519–32. doi: 10.3324/haematol.11007
29. Yoneda-Kato N, Tomoda K, Umehara M, Arata Y, Kato J-Y. Myeloid leukemia factor 1 regulates P53 by suppressing Cop1 Via Cop9 signalosome subunit 3. EMBO J (2005) 24(9):1739–49. doi: 10.1038/sj.emboj.7600656
30. Cui Y, Guo G. Immunomodulatory function of the tumor suppressor P53 in host immune response and the tumor microenvironment. Int J Mol Sci (2016) 17(11):1942. doi: 10.3390/ijms17111942
31. Daver NG, Maiti A, Kadia TM, Vyas P, Majeti R, Wei AH, et al. Tp53-mutated myelodysplastic syndrome and acute myeloid leukemia: Biology, current therapy, and future directions. Cancer Discovery (2022) 12(11):2516–29. doi: 10.1158/2159-8290.CD-22-0332
32. Lim R, Winteringham LN, Williams JH, McCulloch RK, Ingley E, Tiao JYH, et al. Madm, a novel adaptor protein that mediates phosphorylation of the 14-3-3 binding site of myeloid leukemia factor 1. J Biol Chem (2002) 277(43):40997–1008. doi: 10.1074/jbc.M206041200
33. Molzan M, Weyand M, Rose R, Ottmann C. Structural insights of the Mlf1/14-3-3 interaction. FEBS J (2012) 279(4):563–71. doi: 10.1111/j.1742-4658.2011.08445.x
34. Peng CY, Graves PR, Thoma RS, Wu Z, Shaw AS, Piwnica-Worms H. Mitotic and G2 checkpoint control: Regulation of 14-3-3 protein binding by phosphorylation of Cdc25c on serine-216. Sci (New York NY) (1997) 277(5331):1501–5. doi: 10.1126/science.277.5331.1501
35. Zha J, Harada H, Yang E, Jockel J, Korsmeyer SJ. Serine phosphorylation of death agonist bad in response to survival factor results in binding to 14-3-3 not bcl-X(L). Cell (1996) 87(4):619–28. doi: 10.1016/S0092-8674(00)81382-3
36. Sluchanko NN. Recent advances in structural studies of 14-3-3 protein complexes. Adv In Protein Chem Struct Biol (2022) 130:289–324. doi: 10.1016/bs.apcsb.2021.12.004
37. Mas-Bargues C, Borrás C, Viña J. Bcl-xl as a modulator of senescence and aging. Int J Mol Sci (2021) 22(4):1527. doi: 10.3390/ijms22041527
38. Molzan M, Ottmann C. Subcellular localization of full-length human myeloid leukemia factor 1 (Mlf1) is independent of 14-3-3 proteins. Cell Mol Biol Lett (2013) 18(1):137–48. doi: 10.2478/s11658-012-0044-1
39. Marenda M, Lazarova E, Gilbert N. The role of saf-a/Hnrnp U in regulating chromatin structure. Curr Opin In Genet Dev (2022) 72:38–44. doi: 10.1016/j.gde.2021.10.008
40. Nozawa R-S, Boteva L, Soares DC, Naughton C, Dun AR, Buckle A, et al. Saf-a regulates interphase chromosome structure through oligomerization with chromatin-associated rnas. Cell (2017) 169(7):1214–1227.e18. doi: 10.1016/j.cell.2017.05.029
41. Connolly C, Takahashi S, Miura H, Hiratani I, Gilbert N, Donaldson AD, et al. Saf-a promotes origin licensing and replication fork progression to ensure robust DNA replication. J Cell Sci (2022) 135(2):jcs258991. doi: 10.1242/jcs.258991
42. Bras S, Martin-Lannerée S, Gobert V, Augé B, Breig O, Sanial M, et al. Myeloid leukemia factor is a conserved regulator of runx transcription factor activity involved in hematopoiesis. Proc Natl Acad Sci United States America (2012) 109(13):4986–91. doi: 10.1073/pnas.1117317109
43. Miller M, Chen A, Gobert V, Augé B, Beau M, Burlet-Schiltz O, et al. Control of runx-induced repression of notch signaling by mlf and its partner dnaj-1 during drosophila hematopoiesis. PloS Genet (2017) 13(7):e1006932. doi: 10.1371/journal.pgen.1006932
44. Lin T-C. Runx1 and cancer. Biochim Et Biophys Acta Rev On Cancer (2022) 1877(3):188715. doi: 10.1016/j.bbcan.2022.188715
45. Nakamae I, Kato J-Y, Yokoyama T, Ito H, Yoneda-Kato N. Myeloid leukemia factor 1 stabilizes tumor suppressor C/Ebpα to prevent Trib1-driven acute myeloid leukemia. Blood Adv (2017) 1(20):1682–93. doi: 10.1182/bloodadvances.2017007054
46. Pabst T, Mueller BU, Harakawa N, Schoch C, Haferlach T, Behre G, et al. Aml1-eto downregulates the granulocytic differentiation factor C/Ebpalpha in T (8,21) myeloid leukemia. Nat Med (2001) 7(4):444–51. doi: 10.1038/86515
47. Dedhia PH, Keeshan K, Uljon S, Xu L, Vega ME, Shestova O, et al. Differential ability of tribbles family members to promote degradation of C/Ebpalpha and induce acute myelogenous leukemia. Blood (2010) 116(8):1321–8. doi: 10.1182/blood-2009-07-229450
48. Hashimoto A, Sarker D, Reebye V, Jarvis S, Sodergren MH, Kossenkov A, et al. Upregulation of C/Ebpα inhibits suppressive activity of myeloid cells and potentiates antitumor response in mice and patients with cancer. Clin Cancer Res (2021) 27(21):5961–78. doi: 10.1158/1078-0432.CCR-21-0986
49. Nakamae I, Kato JY, Yokoyama T, Ito H, Yoneda-Kato N. Myeloid leukemia factor 1 stabilizes tumor suppressor C/Ebpalpha to prevent Trib1-driven acute myeloid leukemia. Blood Adv (2017) 1(20):1682–93. doi: 10.1182/bloodadvances.2017007054
50. Hanissian SH, Akbar U, Teng B, Janjetovic Z, Hoffmann A, Hitzler JK, et al. Cdna cloning and characterization of a novel gene encoding the Mlf1-interacting protein Mlf1ip. Oncogene (2004) 23(20):3700–7. doi: 10.1038/sj.onc.1207448
51. Minoshima Y, Hori T, Okada M, Kimura H, Haraguchi T, Hiraoka Y, et al. The constitutive centromere component cenp-50 is required for recovery from spindle damage. Mol Cell Biol (2005) 25(23):10315–28. doi: 10.1128/MCB.25.23.10315-10328.2005
52. Cope MJ, Whisstock J, Rayment I, Kendrick-Jones J. Conservation within the myosin motor domain: Implications for structure and function. Structure (1996) 4(8):969–87. doi: 10.1016/S0969-2126(96)00103-7
53. Feng G, Zhang T, Liu J, Ma X, Li B, Yang L, et al. Mlf1ip promotes normal erythroid proliferation and is involved in the pathogenesis of polycythemia Vera. FEBS Lett (2017) 591(5):760–73. doi: 10.1002/1873-3468.12587
54. Raimondi SC, Dubé ID, Valentine MB, Mirro J, Watt HJ, Larson RA, et al. Clinicopathologic manifestations and breakpoints of the T (3,5) in patients with acute nonlymphocytic leukemia. Leukemia (1989) 3(1):42–7.
55. Xu Y, Zhang L, Wang Q, Zheng M. Overexpression of Mlf1ip promotes colorectal cancer cell proliferation through Brca1/Akt/P27 signaling pathway. Cell Signal (2022) 92:110273. doi: 10.1016/j.cellsig.2022.110273
56. Wang X, Marcinkiewicz M, Gatain Y, Bouchard M, Mao J, Tremblay M, et al. Investigation of tissue-specific expression and functions of Mlf1-ip during development and in the immune system. PloS One (2013) 8(5):e63783. doi: 10.1371/journal.pone.0063783
57. Suzuki Y, Demoliere C, Kitamura D, Takeshita H, Deuschle U, Watanabe T. Hax-1, a novel intracellular protein, localized on mitochondria, directly associates with Hs1, a substrate of src family tyrosine kinases. J Immunol (1997) 158(6):2736–44. doi: 10.4049/jimmunol.158.6.2736
58. Trebinska A, Rembiszewska A, Ciosek K, Ptaszynski K, Rowinski S, Kupryjanczyk J, et al. Hax-1 overexpression, splicing and cellular localization in tumors. BMC Cancer (2010) 10:76. doi: 10.1186/1471-2407-10-76
59. Dong Q, Li D, Zhao H, Zhang X, Liu Y, Hu Y, et al. Anti-apoptotic hax-1 suppresses cell apoptosis by promoting c-abl kinase-involved ros clearance. Cell Death Dis (2022) 13(4):298. doi: 10.1038/s41419-022-04748-2
60. Huang D, Liu C, Sun X, Sun X, Qu Y, Tang Y, et al. Crl4 and Usp11 oppositely regulate the stability of myeloid leukemia factors (Mlfs). Biochem Biophys Res Commun (2020) 529(2):127–32. doi: 10.1016/j.bbrc.2020.05.186
61. Liao Y, Zhou D, Wang P, Yang M, Jiang N. Ubiquitin specific peptidase 11 as a novel therapeutic target for cancer management. Cell Death Discovery (2022) 8(1):292. doi: 10.1038/s41420-022-01083-5
62. Guo T, Tang H, Yuan Z, Zhang E, Wang X. The dual role of Usp11 in cancer. J Oncol (2022) 2022:9963905. doi: 10.1155/2022/9963905
63. Sun W, Zhang K, Zhang X, Lei W, Xiao T, Ma J, et al. Identification of differentially expressed genes in human lung squamous cell carcinoma using suppression subtractive hybridization. Cancer Lett (2004) 212(1):83–93. doi: 10.1016/j.canlet.2004.03.023
64. Chen J, Guo L, Peiffer DA, Zhou L, Chan OTM, Bibikova M, et al. Genomic profiling of 766 cancer-related genes in archived esophageal normal and carcinoma tissues. Int J Cancer (2008) 122(10):2249–54. doi: 10.1002/ijc.23397
65. Li Z-F, Wu X, Jiang Y, Liu J, Wu C, Inagaki M, et al. Non-pathogenic protein aggregates in skeletal muscle in Mlf1 transgenic mice. J Neurol Sci (2008) 264(1-2):77–86. doi: 10.1016/j.jns.2007.07.027
66. Banck MS, Kanwar R, Kulkarni AA, Boora GK, Metge F, Kipp BR, et al. The genomic landscape of small intestine neuroendocrine tumors. J Clin Invest (2013) 123(6):2502–8. doi: 10.1172/JCI67963
67. Banerjee M, Datta M, Bhattacharyya NP. Modulation of mutant huntingtin aggregates and toxicity by human myeloid leukemia factors. Int J Biochem Cell Biol (2017) 82:1–9. doi: 10.1016/j.biocel.2016.11.008
68. McDaniel LD, Conkrite KL, Chang X, Capasso M, Vaksman Z, Oldridge DA, et al. Common variants upstream of Mlf1 at 3q25 and within cpz at 4p16 associated with neuroblastoma. PloS Genet (2017) 13(5):e1006787. doi: 10.1371/journal.pgen.1006787
69. Rangrez AY, Pott J, Kluge A, Frauen R, Stiebeling K, Hoppe P, et al. Myeloid leukemia factor-1 is a novel modulator of neonatal rat cardiomyocyte proliferation. Biochim Et Biophys Acta Mol Cell Res (2017) 1864(4):634–44. doi: 10.1016/j.bbamcr.2017.01.004
70. Dawson MA, Kouzarides T. Cancer epigenetics: From mechanism to therapy. Cell (2012) 150(1):12–27. doi: 10.1016/j.cell.2012.06.013
71. Ushijima T, Sasako M. Focus on gastric cancer. Cancer Cell (2004) 5(2):121–5. doi: 10.1016/S1535-6108(04)00033-9
72. Yamashita S, Tsujino Y, Moriguchi K, Tatematsu M, Ushijima T. Chemical genomic screening for methylation-silenced genes in gastric cancer cell lines using 5-Aza-2’-Deoxycytidine treatment and oligonucleotide microarray. Cancer Sci (2006) 97(1):64–71. doi: 10.1111/j.1349-7006.2006.00136.x
73. Shi J, Zhang G, Yao D, Liu W, Wang N, Ji M, et al. Prognostic significance of aberrant gene methylation in gastric cancer. Am J Cancer Res (2012) 2(1):116–29.
74. Watanabe Y, Kim HS, Castoro RJ, Chung W, Estecio MRH, Kondo K, et al. Sensitive and specific detection of early gastric cancer with DNA methylation analysis of gastric washes. Gastroenterology (2009) 136(7):2149–58. doi: 10.1053/j.gastro.2009.02.085
75. Zhao S, Dong X, Ni X, Li L, Lu X, Zhang K, et al. Exploration of a novel prognostic risk signature and its effect on the immune response in nasopharyngeal carcinoma. Front In Oncol (2021) 11:709931. doi: 10.3389/fonc.2021.709931
76. Hoh RA, Stowe TR, Turk E, Stearns T. Transcriptional program of ciliated epithelial cells reveals new cilium and centrosome components and links to human disease. PloS One (2012) 7(12):e52166. doi: 10.1371/journal.pone.0052166
77. Leshchenko VV, Kuo P-Y, Shaknovich R, Yang DT, Gellen T, Petrich A, et al. Genomewide DNA methylation analysis reveals novel targets for drug development in mantle cell lymphoma. Blood (2010) 116(7):1025–34. doi: 10.1182/blood-2009-12-257485
Keywords: myeloid leukemia factor 1, nucleo-cytoplasmic shuttling protein, cell cycle regulation, immune function, cell development and differentiation, malignancy development
Citation: Li Z, Yang Y, Wu K, Li Y and Shi M (2023) Myeloid leukemia factor 1: A “double-edged sword” in health and disease. Front. Oncol. 13:1124978. doi: 10.3389/fonc.2023.1124978
Received: 15 December 2022; Accepted: 17 January 2023;
Published: 06 February 2023.
Edited by:
Michele Redell, Baylor College of Medicine, United StatesReviewed by:
Ju Huang, Wenzhou Medical University, ChinaCopyright © 2023 Li, Yang, Wu, Li and Shi. This is an open-access article distributed under the terms of the Creative Commons Attribution License (CC BY). The use, distribution or reproduction in other forums is permitted, provided the original author(s) and the copyright owner(s) are credited and that the original publication in this journal is cited, in accordance with accepted academic practice. No use, distribution or reproduction is permitted which does not comply with these terms.
*Correspondence: Mingxia Shi, c2hteGlhMjAwMkBzaW5hLmNvbQ==
†These authors have contributed equally to this work and share first authorship