- Henan Provincial Key Laboratory of Pediatric Hematology, Children's Hospital Affiliated to Zhengzhou University, Zhengzhou University, Zhengzhou, China
Malignant blastomas develop relentlessly in all functional body organs inflicting severe health ailments in younger children. Malignant blastomas exhibit diverse clinical characteristics in compliance with their emergence in functional body organs. Surprisingly, neither of these preferred treatment types (surgery, radiotherapy, and chemotherapy) showed promise or were effective in treating malignant blastomas among child patients. N ew, innovative immunotherapeutic procedures including monoclonal antibodies and chimeric-antigen based receptor (CAR) cell therapy, coupled with the clinical study of reliable therapeutic targets and immune regulatory pathways targeting malignant blastomas, have attracted the attention of clinicians recently.
Introduction
Malignant blastomas, based on their emergence of localization in vital human organs, have been classified into various types, including neuroblastoma, retinoblastoma, hepatoblastoma, pleuropulmonary blastoma, and pancreatic blastoma. Malignant blastomas predominantly diagnosed in young children inflict severe health ailments on them. Blastomas observed in young children show diverse clinical characteristics with a profound heterogenicity (1). Immunotherapy has been unequivocally proclaimed as an effective treatment strategy to manage malignant blastomas akin to surgical therapy, chemotherapy, and radiotherapy. Nevertheless, among other proposed therapies shown above, immunotherapy has been found pertinent, efficient, and commendable with minimal side effects towards managing malignant blastomas. Moreover, unraveling novel immunotherapeutic procedures concordant with the exploration of crucial cell signaling pathways linked to malignant blastomas could benefit clinicians with an effective way of tackling severe health ailments of malignant blastomas. Children with malignant blastomas, who were exposed to immunotherapy, especially to monoclonal antibodies and chimeric-antigen based receptor (CAR) cell therapies, showed a significant improvement in their health condition with an improved lifestyle and a higher survival percentage. Also, patients showed a noticeable progress associated with a diminished recurrence of blastomas and an improved prognosis with fewer side effects than their counterparts subjected to other recommended anti-tumor therapies. This review elaborately discusses the emergence of advanced novel immunotherapies and their clinical merits in attenuating the progression of malignant blastomas and their associated detrimental health ailments on child patients.
Neuroblastoma
Neuroblastoma principally emerges from the immature embryonic neural crest and develops into an extracranial solid tumor in the early stages of growth in younger children (2, 3). Recently, the anti-GD2-monoclonal antibody that showed higher therapeutic efficacy against neuroblastoma has been proposed in the standard regulatory treatment guidelines accepted for neuroblastoma (2, 4, 5). In contrast, this treatment remains ineffective on child patients with severe neuroblastoma wherein the survival rate was <50% even with combinatorial immunotherapy. Dinutuximab, an anti-tumor drug, showed deleterious side effects such as chronic body pain, catheter-associated infection, fever, cough, edema, and impaired liver enzyme secretions on child patients (6). Henceforth, the drug has been referred to patients with more precautions, challenging all clinicians to treat severe neuroblastoma child patients. Nevertheless, dinutuximab exposure with the chimeric anti-GD2 antibody (CH14.18) treatment showed a positive clinical outcome with a better survival rate and a significant improvement in GM-CSF, IL-2, and RA concentrations in the neuroblastoma child patients in the phase III trial (5). The study outcomes were promising and notable with positive clinical inferences compared to the clinical outcomes of 226 neuroblastoma child patients who have responded positively to induction therapy and stem cell transplantation.
In the present scenario, the anti-GD2 antibody has been clinically recommended with other treatment means to manage neuro blastoma. GD2 (CH14.18/CHO) antibody exposure as a supplementary therapy with haploidentical stem cell transplantation was effective on child patients diagnosed with stage IV recurrent neuroblastoma. Immunological inferences of this study affirmed that an exposure to anti-GD2 antibody considerably enhanced antigen-dependent cytotoxicity and complement-dependent cytotoxicity accompanied by the increased pro-inflammatory cytokines and natural killer cell responses. Moreover, the use of haploidentical stem cell transplantation in combinatorial therapy, particularly with immunotherapy, which resulted in a positive clinical outcome, can be clinically adopted to treat advanced neuroblastoma via spawning functional effector cells to facilitate natural killer cells or T-cell therapy (7). The mechanisms related to anti-GD2-induced tumor microenvironment and cytotoxic action of neuroblastoma are detailed in Figure 1.
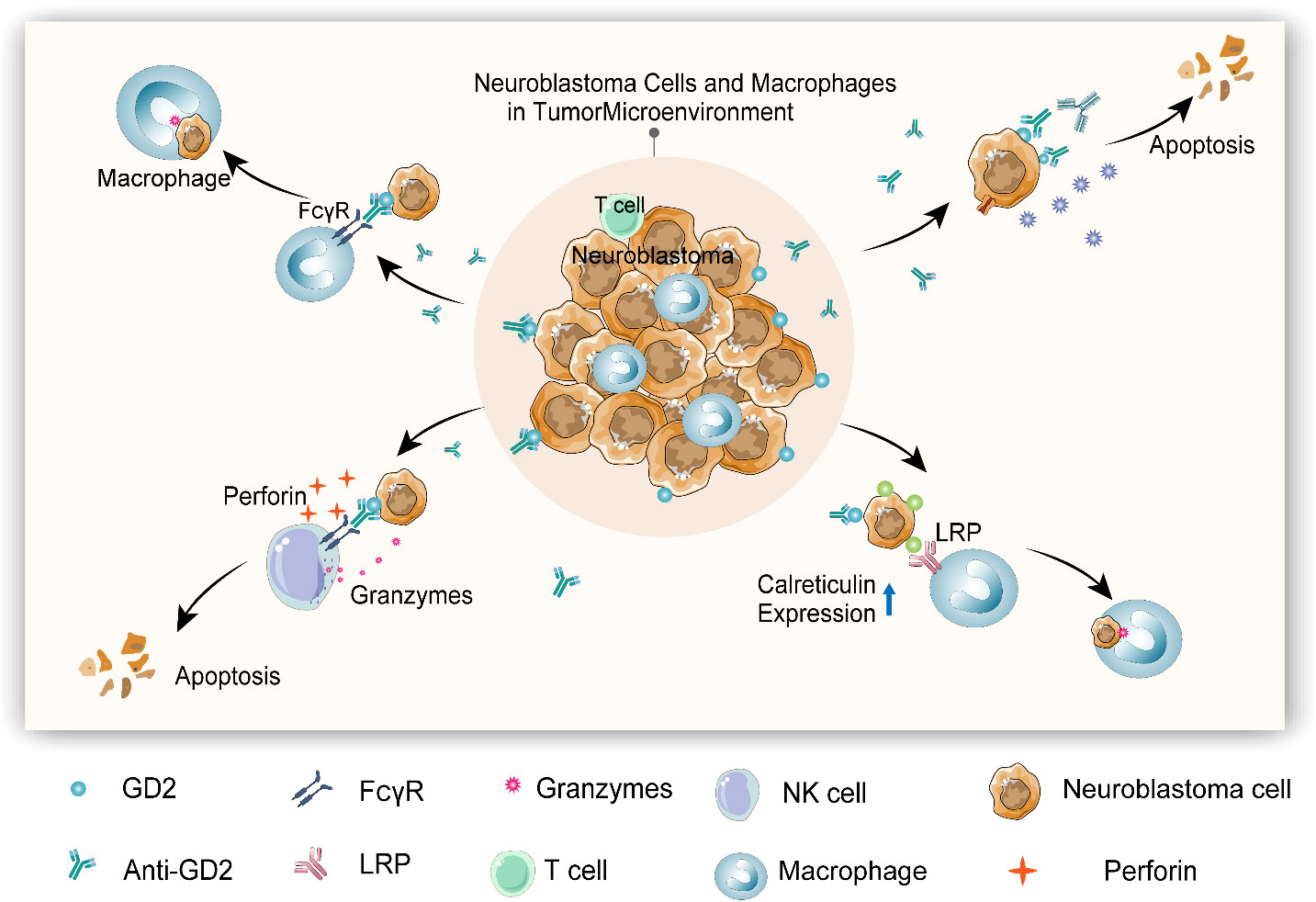
Figure 1 Anti-GD2-induced tumor microenvironment and cytotoxic action of neuroblastoma (1). Macrophage can be activated by anti-GD2 via FCγRI (CD64) and FcγRIIA (CD32), initiating phagocytosis to kill neuroblastoma cells (2). Anti-GD2 induces complement-dependent cytotoxicity (CDC) by triggering complementary activation through C1q antibody interactions to kill neuroblastoma cells (3). Anti-GD2 activates antibody-dependent cytotoxicity (ADCC) of natural killer (NK) cells via FcγRIIIA (CD16a), thereby releasing perforin and granulozyme to kill neuroblastoma cells (4). Anti-GD2 and other monoclonal antibodies can induce the expression of calreticulin (“eat me” molecule) on neuroblastoma cells and enhance the phagocytosis of macrophages.
Antigen-dependent cytotoxicity is an immunological response that attenuates tumor cells’ growth and development via enhanced antibody production. Dinutuximab’s antineoplastic effect has been markedly regulated by the antigen-dependent cytotoxicity stimulating antibody production. Also, dinutuximab exposure by inhibiting the crucial CD47-SIRPα marker ameliorated the neutrophil action degrading neuroblastoma development (8). This noticeable anti-neuroblastoma effect of dinutuximab is of the adrenergic phenotype, reinforcing its therapeutic proficiency against neuroblastoma.
Similarly, children with neuroblastoma exposed to anti-GD2 antibody showed a better recovery with the increased granulocyte-colony stimulating factor secretions. Research studies have assured the beneficial effect of elevated granulocyte-colony stimulating factor secretions with anti-GD2 antibody exposure providing a better clinical outcome and recovery on children with neuroblastoma (8, 9). Concomitantly, unveiling novel therapeutic targets linked to antigen-dependent cytotoxicity is crucial to ameliorate its therapeutic efficacy in targeting neuroblastoma.
However, studies have shown that subcutaneous administration of interleukin-2 (10, 11) while treating with intravenous dinutuximab triggered adverse symptoms of neuroblastoma with unprecedented inflammatory responses (9). Clinical trial validation of this combinatorial therapy affirming its appropriate dosage level is crucial before experimenting with this on children with neuroblastoma. An early review showed that anti-GD2 antibody (CH14.18) is ineffective in reversing the adverse health ailments and associated mortality in child patients (12). Yu et al.’s (13) clinical follow-up showed dinutuximab ameliorating the survival rate but eventually turned out to be inefficient in the later stages of development in children with neuroblastoma. Naxitamab, a newly developed anti-GD2 antibody, has been accredited by the US Food and Drug Administration (FDA) to treat refractory and/or recurrent neuroblastoma augmented with GM-CSF (14). Naxitamab treatment was found to be effective, with positive clinical outcomes on neuroblastoma child patients identified only with bone or bone marrow ailments in severe refractory/relapsed conditions. This clinical phase II trial was conducted in the recent international center that constitutes 48 normal control subjects and 36 children with neuroblastoma under the above clinical conditions. Nearly 58% of children with neuroblastoma (21 of 36) responded well to naxitamab therapy, 44% (16 of 36) of whom showed a complete recovery. Another 16% showed only a partial recovery with naxitamab therapy. The patients’ clinical responses were examined in a time interval of 25 weeks. Common side effects associated with infusion, including pain, were unanimously witnessed among all study patients. Simultaneously, 13 patients showed detrimental side effects with naxitamab treatment, of whom 4 patients were hypotensive, 4 patients had anaphylaxis, and the remaining 5 patients were each diagnosed with fatigue, fever, laryngeal edema, respiratory depression, and urticaria. Furthermore, four patients were expelled from the clinical trial after they developed severe adverse reactions to naxitamab. Surprisingly, there were no mortality recorded at any level of the clinical trials. Also, in detail, clinical trials have to be conducted meticulously to affirm the therapeutic efficacy of naxitamab against neuroblastoma (3). Clinical trials in progress deployed Naxitamab treatment for neuroblastoma are detailed in Table 1.
We believe that any T cell has an extremely sensitive and specific T-cell receptor (TCR) that can detect abnormal signals of the body and trigger a cascade of immune responses when the abnormal peptide chain is identified as the pathogen. Chimeric antigen receptor (CAR) T -cell therapy has become the most popular therapeutic method in cancer individualized immunotherapy. CAR T-cell therapy principally employs gene editing that facilitates T cells to target tumor cell-specific antigens through gene modification intended to kill target-specific tumor cells. For the CAR T-cell therapy, the T cells from patients’ site-specific tumor tissues will be derived and then injected back into the patients’ bodies after in vitro gene editing and amplification to produce pronounced anti-tumor effects (15). CAR T-cell therapy has shown promising clinical outcomes in patients diagnosed with acute lymphoblastic leukemia (16). However, due to side effects, antigen escape, and immune suppressive tumor microenvironment (TME), the CAR T-cell therapeutic effect on solid tumors is not satisfactory. Although CAR T-cell therapy has found many alternative targets in the treatment of solid tumors, most of them show limited anti-tumor effects in clinical trials (15, 17, 18). The application of CAR T-cell therapy in attenuating solid tumors demands more clinical studies. The clinical challenges in CAR T-cell therapy are illustrated in Figure 2.
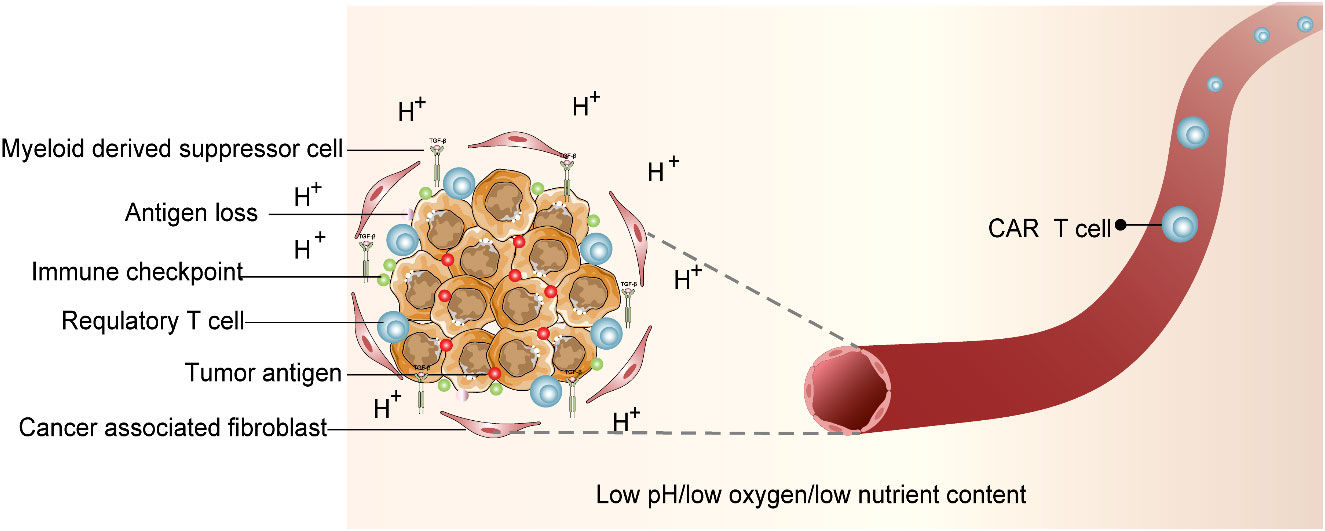
Figure 2 Immunosuppressive tumor microenvironment (TME). CAR T-cell therapy is entangled with difficulties. TME that deteriorates CAR T-cell survival is characterized by acidic pH, oxidative stress, nutritional depletion, and hypoxia. In addition, associated antigen loss, inhibitory cytokines and regulatory T cells (Tregs), myeloid-derived suppressor cells (MDSCs), tumor-associated macrophages (TAMs), and cancer-associated fibroblasts (CAFs) were factors that influence CAR T-cell activity.
Studies have revealed that CAR T-cell therapy did not show any noticeable therapeutic effect on neuroblastoma patients (19). Research studies evaluating the therapeutic proficiency of CAR T-cell therapy against neuroblastoma in young children have been widely performed. SynNotch-based GD2-B7H3 CAR T-cell therapy is a distinct immunotherapy developed with higher specificity and therapeutic proficiency (to target neuroblastoma) that employs gated CAR T cells, which are more promising than conventional CAR T cells in terms of anti-tumor effect. This inference is made upon its supreme metabolic adaptation and minimal depletion levels. To ameliorate the therapeutic effect of CAR T cells on neuroblastoma, indoleamine-pyrrole 2, 3-dioxygenase 1 (IDO1), which attenuates the anti-tumor responses of anti-GD2 CAR T cells and natural killer cells, has been subjected to clinical phase I/II trials, offering a better clinical outcome in treating neuroblastoma (20). Clinical trials in progress employed CAR-T treatment for neuroblastoma are detailed in Table 2. Collectively, CAR T-cell therapy was effective in treating neuroblastoma.
Retinoblastoma
Retinoblastoma (RB) is a commonly diagnosed systemic intraocular tumor in child patients and is found to be detrimental and aggressive, mainly infiltrating retinal orbit and intracranial tissues. RB is inflicted by the bi-allelic loss regulating RB gene function. Surgical resection, radiotherapy, and chemotherapy are the available treatment options recommended to manage RB in children. Among these treatment choices, chemotherapy was perceived to be effective compared to the other recommended options, greatly attenuating tumor metastasis and its progression but still considered ineffective on refractory/severely ill children with RB (21, 22).
Recently, immunotherapy as a mode of treatment for children with RB diagnosed with severe retinal ailments has attracted the attention of clinicians.
Dinutuximab exposure to CD16-expressing NK-92MI (NK-92MIhCD16-GFP) cells and RB cells markedly triggered apoptosis with elevated lactic dehydrogenase (LDH) levels. Concomitantly, further exposure of RB cells in the same experimental setup involving dinutuximab with NK-92MIhCD16-GFP cells enhanced perforin granase B release and CD107a expression (23). Overall, dinutuximab administration with NK-92MIhCD16-GFP cells exhibited its notable anti-tumor effect, especially RB via anti- bot-dependent cell-mediated cytotoxicity. The mechanism by which the NK-92MIhCD16-GFP cells exhibits their anti-tumor effect against RB is illustrated in two levels in Figure 3.
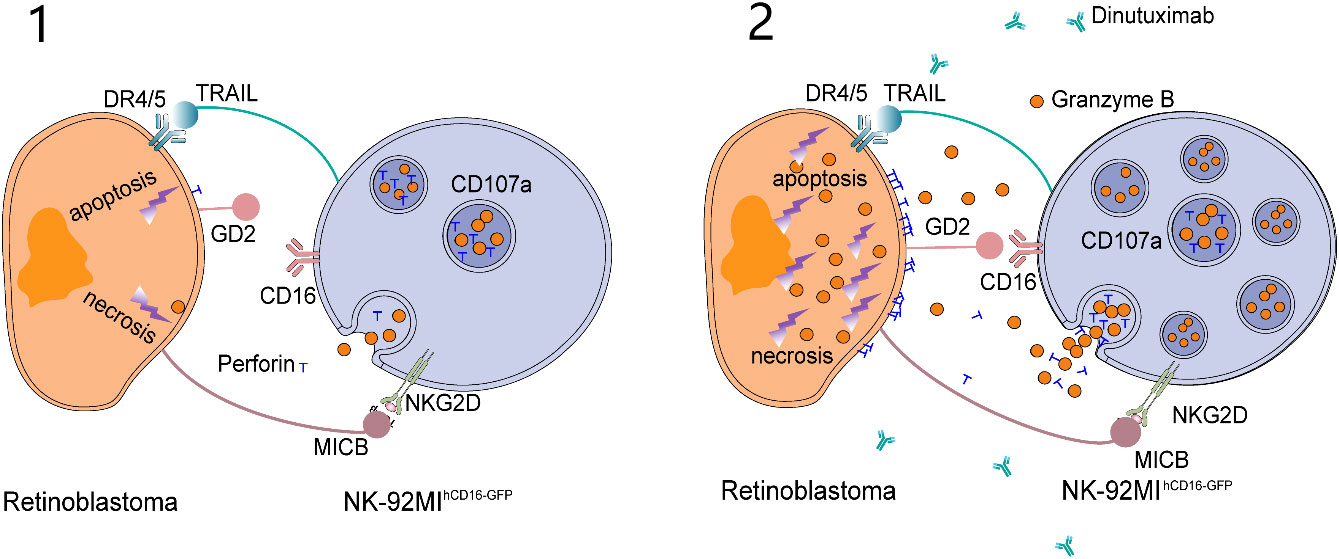
Figure 3 Wang et al. proposed the killing model of NK-92MIhCD16-GFP cells and dinutuximab to retinoblastoma cells. (1) NK-92MIhCD16-GFP cells can induce weak apoptosis and necrosis of retinoblastoma cells through intrinsic active receptors on NK-92MIhCD16-GFP cells and minimum perforin and granzyme B released by NK-92MIhCD16-GFP cells. (2) As a bridge between GD2 on retinoblastoma cells and CD16 on NK-92MIhCD16-GFP cells, dinutuximab triggers a strong antibody-dependent cytotoxicity (ADCC) effect and leads to massive death of retinoblastoma cells. At the same time, CD107a protects NK-92MIhCD16-GFP cells from cytotoxic granules.
An enhanced B7H3 expression positively correlated with tumor metastasis and prognostic malignancy can be a noticeable therapeutic target in managing RB and its associated retinal ailments. Also, clinical findings affirmed increased B7H3 expression in RB cells compared to normal retinal cells, emphasizing it as a promising target of RB management (21).
Earlier clinical studies have shown CAR T-cell therapy to be effective against RB. U pon co- culturing with GD2-antigen and GD2-CAR T cells, nearly 80% of RB cells died in 24 h, and approximately 100% cell mortality was achieved in 3 days (24).
In an in vivo mouse model, exposure of GD2-CAR T cells to IL-15, which specifically releases IL-15 in chitosan-PEG injectable hydrogel accompanied by RB tumor cell implantation, controlled tumor cell proliferation in approximately 60% of mice in 70 days (25). Alternatively, mice administered with GD2-CAR T cells in chitosan-PEG injectable hydrogel showed RB development. An in vitro study showed that RB induced CD-171- and GD2-specific CAR T-cell activation, undermining tumor development, irrespective of the length of the extracellular spacer and costimulatory domains. Also, the study revealed CD-171 and GD2 as notable targets of CAR T cells, warranting an in- depth clinical validation study using an in vivo model (26).
Glioma
Glioma, a tumor predominantly seen in the central nervous system (CNS) of adults, can also affect younger children causing similar health ailments. Glioblastoma is a notable glioma tumor exhibiting a higher degree of malignancy with a diverse heterogenicity in its clinical prevalence. With its notable heterogenicity, glioblastoma remains immune to chemoradiotherapy. Even with surgical therapy, glioblastoma attenuation is not abundantly feasible due to its higher malignancy. Above all, children with glioblastoma show a poor prognosis augmented with a diminished mean survival rate. Compared with these recommended treatments, immunotherapy was identified to be unique and promising, concentrating on the regulation of human host–immune system against glioblastoma emergence on the affected children. Compared with other treatments, immunotherapy may be precise, appropriate, and sustainable (27). Clinicians have focused on immunotherapy recent ly to improve the prognosis of glioblastoma in child patients. However, the use of checkpoint inhibitor monotherapy against glioma was discouraging to date, warranting further clinical trials.
Glioblastoma without tumor -specific antigen gene-protein expression and, thus, resistant to immunotherapy is labeled “cold tumor” (28). Alternatively, a tumor that is susceptible to immunotherapy is labeled “hot tumor”. Also, glioblastoma can undermine the host–immune responses in retaliation by permitting lower tumor -infiltrating lymphocytes compared with the other malignant blastomas (29). At present, immunotherapy for glioblastoma includes tumor vaccine, CAR T-cell therapy, tumor site-specific therapy using tumor-infiltrating lymphocytes, T-cell receptor therapy, and other immunosuppressants.
The well-studied therapeutic targets for CAR T-cell therapy in glioma include IL13Rα2, EGFRvIII, HER2, and GD2. Clinical trials conducted on these biomarkers (as tumor targets) for CAR T-cell therapy showed promising results in treating glioblastoma despite the small number of studies (15). Evolution from T-cell receptor mimetics to the fourth-generation chimeric antigen receptor are detailed in Figure 4. Moreover, patients subjected to therapeutic treatment, particularly single therapeutic targets, become vulnerable to relapse/drug resistance. Therefore, the use of a variety of antigens or immunosuppressive cytokines is recommended to manage tumor development. Afterwards, it is mandatory to widely explore the target antigen library to manage glioma. EphA2, B7H3, and NKG2DL have been unveiled as the new therapeutic targets for CAR T-cell therapy against glioma, and relevant clinical trials are ongoing (15). Clinical trials in progress employing CAR-T treatment for tackling glioma are detailed in Table 3.
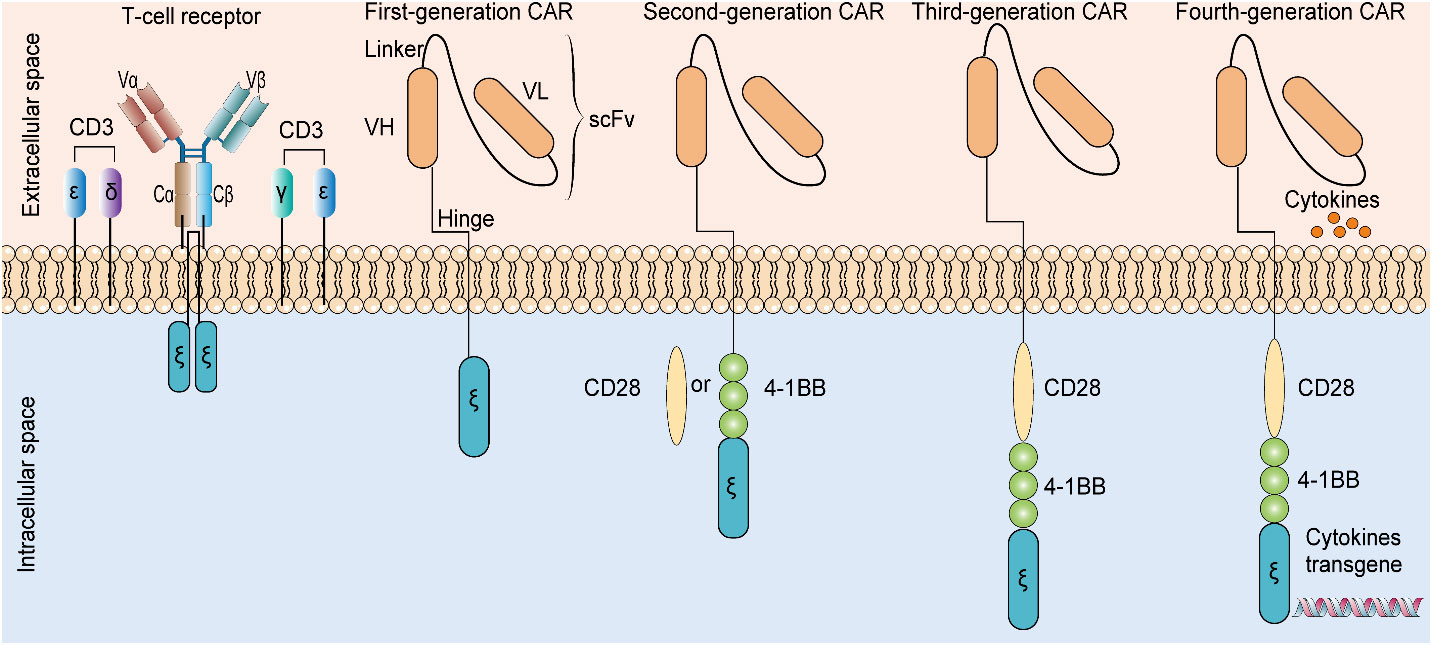
Figure 4 Evolution from T-cell receptor mimetics to the fourth-generation chimeric antigen receptor. The presence of additional cytokine transgenes, costimulatory domains, and T-cell signal domains in four generations of CAR are different. CAR: chimeric antigen receptor. scFV: single-chain variable fragment. VH: variable heavy chain. VL: variable light chain.
Vaccines against glioblastoma are classified into five types: (1) dendritic cell vaccines, (2) polypeptide vaccines, (3) autologous vaccine, (4) heat-shock protein vaccines, and (5) viral protein-based vaccine (30). Immune checkpoints play a significant role in regulating immune response; thus, researchers have developed a series of immune checkpoint inhibitors (ICIs). ICIs can rejuvenate the immune system of children, preventing tumor growth and metastasis. The discovery of ICIs has been acknowledged as a major breakthrough in cancer treatment (31). The key ICIs targeted for glioma include the following: cytotoxic T- lymphocyte-associated protein type 4 (CTLA-4) and programmed cell death-1 (PD-1)/programmed cell death ligand-1 (PD-L1). These ICI s have been principally targeted to treat severe neoplastic diseases (32) and have achieved satisfactory results in some cancers. However, at present, ICI therapy still has serious side effects (e.g., drug resistance). Such side effects are usually related to the increase in immune system activity, which can be manifested as fatigue, anemia, neutropenia, hypothyroidism, rash, colitis, pneumonia and even myocarditis, hepatitis, and other serious adverse events. In terms of drug resistance, most patients show primary drug resistance, and some patients show acquired drug resistance after treatment reaction (31). Therefore, for the successful application of ICI treatment, one needs a comprehensive understanding of its toxicity and the development of new immune checkpoint molecules and combination with other treatments to overcome drug resistance.
PD-L1 expression in glioblastoma remains contradictory. Nonetheless, glioblastoma patients with a higher PD-L1 expression showed an impaired prognosis likely governed by immunosuppressive mechanisms. Nivolumab, which targets PD-1, did not improve longevity in patients diagnosed with recurrent glioblastoma. Alternatively, bevacizumab showed promising results in its phase III trial against glioma development (33). Collectively, immunotherapy for glioma demands further advanced clinical trials using a higher combination therapy that engages immunotherapies targeting the immune system and cells for the effective checkpoint suppression of cancer cells. We firmly believe that combined immunotherapy could have a major breakthrough in managing glioma.
Hepatoblastoma
Hepatoblastoma is a highly prevalent liver malignancy among children accounting for 50%–60% of cases. In comparison, solid malignant tumor incidence accounts for 0.8%–2.0% in children. Hepatoblastoma akin to neuroblastoma and nephroblastoma has been identified as an intraperitoneal malignancy (34) that inflicts severe health ailments in early childhood. Hepatoblastoma, also classified as an embryonic tumor with its detrimental effects, remains unexplored. Clinicians presume that the onset of its adverse symptoms could occur during the advanced fetal stage or later during adulthood, as well as during the infant stage and early childhood. Children in the age group <3 years/<15 years are highly susceptible to the detrimental effects of hepatoblastoma compared to adults (35).
Children with hepatoblastoma showed adverse symptoms such as asymptomatic abdominal mass. Furthermore, other symptoms like fever, decreased body mass, anorexia, and obstructive jaundice are seldom observed among children with hepatoblastoma. Clinical therapies recommended for treating hepatoblastoma include surgical procedures, radiotherapy, liver transplantation, and chemotherapy (36). A mong these, immunotherapy, which principally engages active immune responses, is exceptional in managing hepatoblastoma. Immunotherapy possessed explicit clinical merits on children with hepatoblastoma demanded prolonged radiotherapy and chemotherapy. Clinical pathways and peptides linked to hepatoblastoma have been explored meticulously. Immunosuppressants related to these therapeutic targets were effective in dealing with refractory hepatoblastoma on child patients found immune to surgical procedures, radiotherapy, and chemotherapy.
Common inhibitory protein targets of hepatoblastoma are associated with classical tumor pathways and peptides, particularly glypican-3 (GPC3). GPC3, which is a 65- kDa membranous receptor protein with 580 amino acids, is identified as one of the heparan sulfate proteoglycans (37). GPC3 is ubiquitously expressed on tumor cell membranes, including hepatocellular carcinoma, ovarian clear cell carcinoma, melanoma, lung squamous cell carcinoma, hepatoblastoma, Wilms tumor, and yolk sac tumor. Moreover, GPC3 critically regulates the classical Wnt/β-catenin pathway activation, which is a notable therapeutic means of managing the above deleterious tumor conditions, especially hepatoblastoma (38). GPC3 is also a notable therapeutic target in immunotherapy used for attenuating solid tumor growth in younger children. Immunosuppressants coupled with other immunotherapies, such as tumor vaccine, monoclonal antibody, antibody–drug conjugate, bispecific antibody, CTL, and CAR T-cell therapy specific to GPC3, could attenuate solid embryonic tumor in children (39). Peptide vaccines raised against tumor antigens have proven to be moderately effective in controlling solid refractory tumors in children (40). The GPC3 peptide vaccine exclusive to GPC3-+ve hepatoblastoma has remarkably controlled its emergence at the second level in affected children. Concordantly, a monoclonal antibody raised against GPC3 tumor antigen attenuated hepatocellular carcinoma in patients enrolled in a clinical phase I trial study (41). It is more likely for this GPC3-specific monoclonal antibody to exhibit a similar anti-tumor effect in children with hepatoblastoma. Noticeably, children diagnosed with hepatoblastoma aged >1 year responded well to the GPC3 peptide vaccine than the lower age group children (42). The study defines the age limit for children with hepatoblastoma who intend to undertake immunotherapy, especially the GPC3 peptide vaccine. The mechanism of cancer immunotherapy targeting GPC3 is shown in Figure 5. There are only two clinical trials in progress of GCP3 for hepatoblastoma now, as detailed in Table 4.
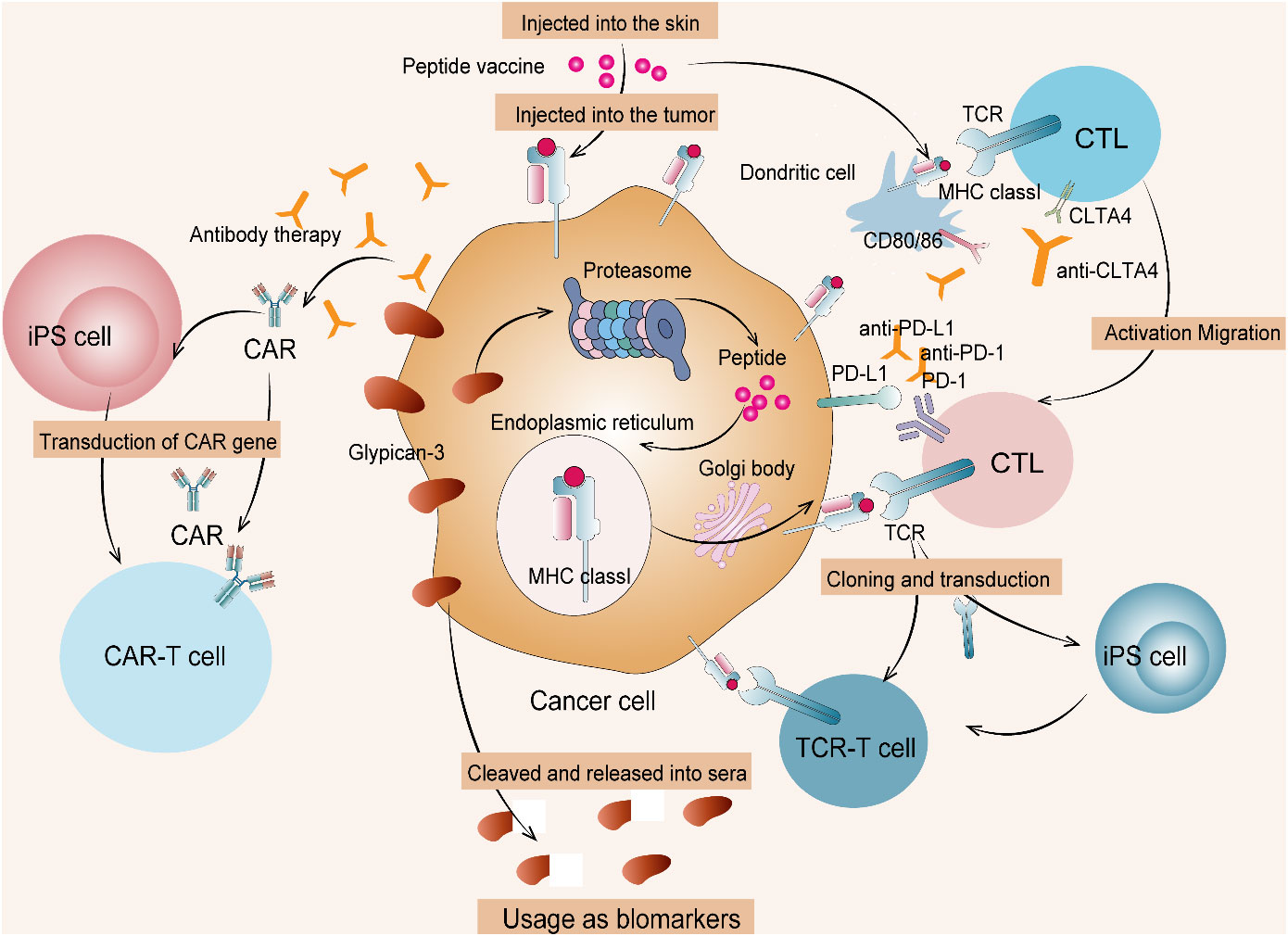
Figure 5 Cancer immunotherapy targeting GPC3. (1) Therapies targeting intracellular GPC3 mainly include GPC3 peptide vaccine and adaptive immunotherapy based on T cells transduced with a suitable TCR. (2) Therapies targeting membrane-bound GPC3 mainly include antibody therapy and anti-GPC3-CAR T-cell therapy. (3) Such T cells and CAR T cells from induced pluripotent stem (iPS) cells are currently being developed. CTL, cytotoxic T lymphocyte; TCR, T-cell receptor.
Pulmonary blastoma
Pulmonary blastoma, defined as a sarcomatoid carcinoma of the lung, is rarely seen among children and accounts for <0.5% of cases. According to the article of Koss, pulmonary blastoma has been bifurcated into three major subtypes: (1) classic biphasic pulmonary blastoma (CBPB), (2) pleuropneumoblastoma, and (3) adequately differentiated fetal adenocarcinoma (43). Pleuropulmonary blastoma is a highly prevalent preliminary lung malignant tumor diagnosed in children (44).
The histopathological examination of pleuropulmonary blastoma tissue morphology showed epithelium and stroma with unevenly branched glandular structures. Additionally, hemorrhage and necrosis were seen on the tumor tissue cells. It is likely that children diagnosed with pleuropulmonary blastoma in their early stages were merely subjected to surgical therapy (45).
Lobectomy is the widely used surgical methodology to treat pleuropulmonary blastoma (46). Chemotherapy and radiotherapy are other mainstream treatment methods recommended for pleuropulmonary blastoma management. There is a lack of research studies that illustrate the merits of target-based therapy of pleuropulmonary blastoma. A case report on a pleuropulmonary blastoma child with CD74-ROS1 gene mutation showed that the child positively responded to the chemo drug crizotinib (47). The case study warranted further in- depth clinical studies to confirm the correlation between this gene mutation and the pleuropulmonary blastoma incidence. Moreover, the case study also emphasized exploring more detrimental gene mutations linked to pleuropulmonary blastoma.
Pancreatic blastoma
Pancreatic blastoma is a malignant tumor of indigenous cell origin seldom diagnosed in children. In compliance with the detrimental stages of pancreatic blastoma emergence, treatment procedures such as surgical therapy, chemotherapy, and radiotherapy would be chosen. Surgical therapy remains the highly preferred therapeutic procedure for managing pancreatic hepatoblastoma among children wherein nearly 200 patients underwent surgical therapy worldwide (48). Further studies should be conducted to understand the therapeutic efficacy of immunotherapy against pancreatic hepatoblastoma.
Nephroblastoma
Nephroblastoma is another common abdominal solid tumor and kidney tumor in children akin to neuroblastoma with a higher incidence (49). Surgical procedures augmented with radiotherapy and chemotherapy have proven to be effective, with a recovery rate of 90%. Nevertheless, this treatment method showed noticeable side effects with adverse toxicity on treated children with nephroblastoma (50).
Children < 10 years of age are highly susceptible to nephroblastoma. The age group between 2 and 5 years was identified to be crucial with a higher incidence of nephroblastoma (51). Chemotherapy drugs of recent use inflict higher mortality within its short interval of exposure governing other detrimental ailments of heart, lung, and kidney, including infertility and secondary tumor development eventually on the patients (52). It is likely that immunotherapy can exhibit a noticeable therapeutic effect but with trivial side effects on nephroblastoma patients. Macrophage infiltration is ubiquitous in all cancerous tissues observed at the highest level in all tumor states, including nephroblastoma.
A literature survey of 230 research studies on nephroblastoma revealed 23 studies on immunotherapy conducted since 2003, of which 17 studies reported in the past decade focused on immunotherapy. The notable immunotherapy procedures reported in the literature review included target-based immunotherapeutic drugs, peptide vaccines, and other cancerous cell surface components of the target (53–56).
CD3+ T lymphocytes expressed at a negligible level by nephroblastoma are likely to trigger immune responses presided by T-regulatory/helper cells (55, 56). Moreover, nephroblastoma tissue cells with elevated interleukin-10 (IL-10) and indoleamine 2,3 dioxygenase expression can inhibit host–immune responses with enhanced immunosuppressant action. Tumor-specific cytotoxic T-cell recruitment preventing nephroblastoma tumor metastasis is feasible by administering T-cell vaccine or CAR T-cell generation (53). Clinical trials in progress of CAR-T treatment for nephroblastoma are detailed in Table 5.
The clinical phase I trial performed using tumor-centric antigen focusing on the recruitment of cytotoxic T cells inhibited the development of pediatric-solid tumors in relapsed/refractory states on the respective study patients (54). Biological components of tumor cells such as polysaccharides, glycoproteins, and cell surface receptors play vital roles in tumor cell metastasis governed by tumor cell- to-cell communication, proliferation, and infiltration. Target-based tumor therapy engages cell surface molecules such as GM3 and GPC3, which are promising and effective in treating nephroblastoma (40, 57, 58).
Collectively, research studies that focused on immunotherapy against nephroblastoma included cell surface components, cytokines, and tumor -suppressing genes. These proposed research studies recommend immunotherapy as a treatment to manage nephroblastoma (59).
Conclusion and future prospects
The prognosis of patients diagnosed with malignant blastoma was poor and disappointing, with recommended therapeutic treatment methods such as active surgery, chemotherapy, and radiotherapy. Patients subjected to chemotherapy and radiotherapy suffered from severe secondary health complications, eventually adversely affecting their lifestyle and livelihood. Recent ly, immunotherapy that employs monoclonal antibodies, cancer vaccines, and CAR cell therapy in managing malignant blastomas in affected patients shows promise and is effective (16, 60). Although a few novel therapeutic approaches excluding immunotherapy have been subjected to clinical trials, it is still time-consuming and challenging to implement strategies that are clinically based on study outcomes in treating malignant blastomas. We firmly believe that having immunotherapy as an adjunct therapy could benefit patients with malignant blastoma in terms of reduced mortality and increased life expectancy with sustainable clinical outcomes.
Data availability statement
The original contributions presented in the study are included in the article/supplementary material. Further inquiries can be directed to the corresponding authors.
Author contributions
BZ collected and analyzed data. LD and LL wrote the manuscript. SAK revised this manuscript. YD designed the study. WL and CZ collected and analyzed data and supervised the drafting of the manuscript. All authors contributed to the article and approved the submitted version.
Conflict of interest
The authors declare that the research was conducted in the absence of any commercial or financial relationships that could be construed as a potential conflict of interest.
Publisher’s note
All claims expressed in this article are solely those of the authors and do not necessarily represent those of their affiliated organizations, or those of the publisher, the editors and the reviewers. Any product that may be evaluated in this article, or claim that may be made by its manufacturer, is not guaranteed or endorsed by the publisher.
References
1. Willis RA. The borderland of embryology and pathology. Bull New York Acad Med (1950) 26(7):440–60.
2. Zirngibl F, Ivasko SM, Grunewald L, Klaus A, Schwiebert S, Ruf P, et al. Gd2-directed bispecific trifunctional antibody outperforms dinutuximab beta in a murine model for aggressive metastasized neuroblastoma. J Immunother Cancer (2021) 9(7):13. doi: 10.1136/jitc-2021-002923
3. Martinez Sanz P, van Rees DJ, van Zogchel LMJ, Klein B, Bouti P, Olsman H, et al. G-Csf as a suitable alternative to gm-csf to boost dinutuximab-mediated neutrophil cytotoxicity in neuroblastoma treatment. J Immunother Cancer (2021) 9(5):13. doi: 10.1136/jitc-2020-002259
4. Simon T, Hero B, Faldum A, Handgretinger R, Schrappe M, Klingebiel T, et al. Long term outcome of high-risk neuroblastoma patients after immunotherapy with antibody Ch14.18 or oral metronomic chemotherapy. BMC Cancer (2011) 11:21. doi: 10.1186/1471-2407-11-21
5. Yu AL, Gilman AL, Ozkaynak MF, London WB, Kreissman SG, Chen HX, et al. Anti-Gd2 antibody with gm-csf, interleukin-2, and isotretinoin for neuroblastoma. New Engl J Med (2010) 363(14):1324–34. doi: 10.1056/NEJMoa0911123
6. Blom T, Lurvink R, Aleven L, Mensink M, Wolfs T, Dierselhuis M, et al. Treatment-related toxicities during anti-Gd2 immunotherapy in high-risk neuroblastoma patients. Front Oncol (2020) 10:601076. doi: 10.3389/fonc.2020.601076
7. Seitz CM, Flaadt T, Mezger M, Lang AM, Michaelis S, Katz M, et al. Immunomonitoring of stage iv relapsed neuroblastoma patients undergoing haploidentical hematopoietic stem cell transplantation and subsequent Gd2 (Ch14.18/Cho) antibody treatment. Front Immunol (2021) 12:690467. doi: 10.3389/fimmu.2021.690467
8. Ladenstein R, Pötschger U, Valteau-Couanet D, Luksch R, Castel V, Ash S, et al. Investigation of the role of dinutuximab beta-based immunotherapy in the siopen high-risk neuroblastoma 1 trial (Hr-Nbl1). Cancers (2020) 12(2):19. doi: 10.3390/cancers12020309
9. Ladenstein R, Pötschger U, Valteau-Couanet D, Luksch R, Castel V, Yaniv I, et al. Interleukin 2 with anti-Gd2 antibody Ch14.18/Cho (Dinutuximab beta) in patients with high-risk neuroblastoma (Hr-Nbl1/Siopen): A multicentre, randomised, phase 3 trial. Lancet Oncol (2018) 19(12):1617–29. doi: 10.1016/s1470-2045(18)30578-3
10. Mora J, Bear M, Chan G, Morgenstern DA, Nysom K, Tornoe K, et al. Naxitamab for the treatment of Refractory/Relapsed high-risk neuroblastoma (Hr nb): Updated efficacy and safety data from the international, multicenter phase ii trial 201. Ann Oncol (2021) 32:S833–S. doi: 10.1016/j.annonc.2021.08.1348
12. Simon T, Hero B, Faldum A, Handgretinger R, Schrappe M, Niethammer D, et al. Consolidation treatment with chimeric anti-Gd2-Antibody Ch14.18 in children older than 1 year with metastatic neuroblastoma. J Clin Onco: Off J Am Soc Clin Oncol (2004) 22(17):3549–57. doi: 10.1200/jco.2004.08.143
13. Yu AL, Gilman AL, Ozkaynak MF, Naranjo A, Diccianni MB, Gan J, et al. Long-term follow-up of a phase iii study of Ch14.18 (Dinutuximab) + cytokine immunotherapy in children with high-risk neuroblastoma: Cog study Anbl0032. Clin Cancer Res: an Off J Am Assoc Cancer Res (2021) 27(8):2179–89. doi: 10.1158/1078-0432.Ccr-20-3909
14. Martínez-Sanz P, Hoogendijk AJ, Verkuijlen P, Schornagel K, van Bruggen R, van den Berg TK, et al. Cd47-sirpα checkpoint inhibition enhances neutrophil-mediated killing of dinutuximab-opsonized neuroblastoma cells. Cancers (2021) 13(17):15. doi: 10.3390/cancers13174261
15. Qu C, Zhang H, Cao H, Tang L, Mo H, Liu F, et al. Tumor buster - where will the car-T cell therapy ‘Missile’ go? Mol Cancer (2022) 21(1):201. doi: 10.1186/s12943-022-01669-8
16. Maude SL, Frey N, Shaw PA, Aplenc R, Barrett DM, Bunin NJ, et al. Chimeric antigen receptor T cells for sustained remissions in leukemia. New Engl J Med (2014) 371(16):1507–17. doi: 10.1056/NEJMoa1407222
17. Zhylko A, Winiarska M, Graczyk-Jarzynka A. The great war of today: Modifications of car-T cells to effectively combat malignancies. Cancers (2020) 12(8):29. doi: 10.3390/cancers12082030
18. Ma S, Li X, Wang X, Cheng L, Li Z, Zhang C, et al. Current progress in car-T cell therapy for solid tumors. Int J Biol Sci (2019) 15(12):2548–60. doi: 10.7150/ijbs.34213
19. Moghimi B, Muthugounder S, Jambon S, Tibbetts R, Hung L, Bassiri H, et al. Preclinical assessment of the efficacy and specificity of Gd2-B7h3 synnotch car-T in metastatic neuroblastoma. Nat Commun (2021) 12(1):511. doi: 10.1038/s41467-020-20785-x
20. Caforio M, Sorino C, Caruana I, Weber G, Camera A, Cifaldi L, et al. Gd2 redirected car T and activated nk-Cell-Mediated secretion of ifnγ overcomes mycn-dependent Ido1 inhibition, contributing to neuroblastoma cell immune escape. J Immunother Cancer (2021) 9(3):11. doi: 10.1136/jitc-2020-001502
21. Ganesan B, Parameswaran S, Sharma A, Krishnakumar S. Clinical relevance of B7h3 expression in retinoblastoma. Sci Rep (2020) 10(1):10185. doi: 10.1038/s41598-020-67101-7
22. Chen X, Kunda PE, Lin J, Zhou M, Huang J, Zhang H, et al. Syk-targeted dendritic cell-mediated cytotoxic T lymphocytes enhance the effect of immunotherapy on retinoblastoma. J Cancer Res Clin Oncol (2018) 144(4):675–84. doi: 10.1007/s00432-018-2584-x
23. Wang H, Yang J, Pan H, Tai MC, Maher MH, Jia R, et al. Dinutuximab synergistically enhances the cytotoxicity of natural killer cells to retinoblastoma through the perforin-granzyme b pathway. OncoTargets Ther (2020) 13:3903–20. doi: 10.2147/ott.S228532
24. Sujjitjoon J, Sayour E, Tsao ST, Uiprasertkul M, Sanpakit K, Buaboonnam J, et al. Gd2-specific chimeric antigen receptor-modified T cells targeting retinoblastoma - assessing tumor and T cell interaction. Trans Oncol (2021) 14(2):100971. doi: 10.1016/j.tranon.2020.100971
25. Wang K, Chen Y, Ahn S, Zheng M, Landoni E, Dotti G, et al. Gd2-specific car T cells encapsulated in an injectable hydrogel control retinoblastoma and preserve vision. Nat Cancer (2020) 1(10):990–7. doi: 10.1038/s43018-020-00119-y
26. Andersch L, Radke J, Klaus A, Schwiebert S, Winkler A, Schumann E, et al. Cd171- and Gd2-specific car-T cells potently target retinoblastoma cells in preclinical in vitro testing. BMC Cancer (2019) 19(1):895. doi: 10.1186/s12885-019-6131-1
27. Zhang W, Qin T, Yang Z, Yin L, Zhao C, Feng L, et al. Telomerase-positive circulating tumor cells are associated with poor prognosis Via a neutrophil-mediated inflammatory immune environment in glioma. BMC Med (2021) 19(1):277. doi: 10.1186/s12916-021-02138-7
28. Yasmin IA, Mohana Sundaram S, Banerjee A, Varier L, Dharmarajan A, Warrier S. Netrin-like domain of Sfrp4, a wnt antagonist inhibits stemness, metastatic and invasive properties by specifically blocking mmp-2 in cancer stem cells from human glioma cell line U87mg. Exp Cell Res (2021) 409(2):112912. doi: 10.1016/j.yexcr.2021.112912
29. Mohammed S, Ravikumar V, Warner E, Patel SH, Bakas S, Rao A, et al. Quantifying T2-flair mismatch using geographically weighted regression and predicting molecular status in lower-grade gliomas. AJNR Am J Neuroradiol (2022) 43(1):33–9. doi: 10.3174/ajnr.A7341
30. Bianconi A, Prior A, Zona G, Fiaschi P. Anticoagulant therapy in high grade gliomas: A systematic review on state of the art and future perspectives. J Neurosurgical Sci (2021). doi: 10.23736/s0390-5616.21.05536-3
31. Wang Y, Zhang H, Liu C, Wang Z, Wu W, Zhang N, et al. Immune checkpoint modulators in cancer immunotherapy: Recent advances and emerging concepts. J Hematol Oncol (2022) 15(1):111. doi: 10.1186/s13045-022-01325-0
32. Li G, Lan Q. Exosome-mediated transfer of circ-Glis3 enhances temozolomide resistance in glioma cells through the mir-548m/Med31 axis. Cancer Biother Radiopharmaceuticals (2021) 12. doi: 10.1089/cbr.2021.0299
33. Wang X, Lu J, Guo G, Yu J. Immunotherapy for recurrent glioblastoma: Practical insights and challenging prospects. Cell Death Dis (2021) 12(4):299. doi: 10.1038/s41419-021-03568-0
34. Hu H, Zhang W, Zhi T, Li J, Wen Y, Li F, et al. Genotypic characteristics of hepatoblastoma as detected by next generation sequencing and their correlation with clinical efficacy. Front Oncol (2021) 11:628531. doi: 10.3389/fonc.2021.628531
35. Nagae G, Yamamoto S, Fujita M, Fujita T, Nonaka A, Umeda T, et al. Genetic and epigenetic basis of hepatoblastoma diversity. Nat Commun (2021) 12(1):5423. doi: 10.1038/s41467-021-25430-9
36. Moosburner S, Schmelzle M, Schöning W, Kästner A, Seika P, Globke B, et al. Liver transplantation is highly effective in children with irresectable hepatoblastoma. Medicina (2021) 57(8):9. doi: 10.3390/medicina57080819
37. Li W, Guo L, Rathi P, Marinova E, Gao X, Wu MF, et al. Redirecting T cells to glypican-3 with 4-1bb zeta chimeric antigen receptors results in Th1 polarization and potent antitumor activity. Hum Gene Ther (2017) 28(5):437–48. doi: 10.1089/hum.2016.025
38. Ortiz MV, Roberts SS, Glade Bender J, Shukla N, Wexler LH. Immunotherapeutic targeting of Gpc3 in pediatric solid embryonal tumors. Front Oncol (2019) 9:108. doi: 10.3389/fonc.2019.00108
39. Koh KN, Namgoong JM, Yoon HM, Cho YA, Choi SH, Shin J, et al. Recent improvement in survival outcomes and reappraisal of prognostic factors in hepatoblastoma. Cancer Med (2021) 10(10):3261–73. doi: 10.1002/cam4.3897
40. Tsuchiya N, Hosono A, Yoshikawa T, Shoda K, Nosaka K, Shimomura M, et al. Phase I study of glypican-3-Derived peptide vaccine therapy for patients with refractory pediatric solid tumors. Oncoimmunology (2017) 7(1):e1377872. doi: 10.1080/2162402x.2017.1377872
41. Shimizu Y, Suzuki T, Yoshikawa T, Endo I, Nakatsura T. Next-generation cancer immunotherapy targeting glypican-3. Front Oncol (2019) 9:248. doi: 10.3389/fonc.2019.00248
42. Kinoshita Y, Tanaka S, Souzaki R, Miyoshi K, Kohashi K, Oda Y, et al. Glypican 3 expression in pediatric malignant solid tumors. Eur J Pediatr Surgery: Off J Austrian Assoc Pediatr Surg [et al] = Z fur Kinderchirurgie (2015) 25(1):138–44. doi: 10.1055/s-0034-1393961
43. Koss MN. Pulmonary blastomas. Cancer Treat Res (1995) 72:349–62. doi: 10.1007/978-1-4615-2630-8_16
44. Knight S, Knight T, Khan A, Murphy AJ. Current management of pleuropulmonary blastoma: A surgical perspective. Children (2019) 6(8):9. doi: 10.3390/children6080086
45. Van Loo S, Boeykens E, Stappaerts I, Rutsaert R. Classic biphasic pulmonary blastoma: A case report and review of the literature. Lung Cancer (2011) 73(2):127–32. doi: 10.1016/j.lungcan.2011.03.018
46. Kim K, Gupta S, Gupta S, Mittar P, Minimo C, Tester W. Incidental early diagnosis of biphasic pulmonary blastoma in a patient with history of stage iv lung adenocarcinoma. Thorac Cancer (2020) 11(10):3029–33. doi: 10.1111/1759-7714.13629
47. Zaidi A, Zamvar V, Macbeth F, Gibbs AR, Kulatilake N, Butchart EG. Pulmonary blastoma: Medium-term results from a regional center. Ann Thorac Surg (2002) 73(5):1572–5. doi: 10.1016/s0003-4975(02)03494-x
48. Ibuka S, Uehara S, Ueno T, Oue T, Miyamura T, Hashii Y, et al. Complete resection of pancreatoblastoma with portal vein obstruction after high-dose chemotherapy: A case report. J Pediatr Hematol/Oncol (2017) 39(5):e275–e8. doi: 10.1097/mph.0000000000000842
50. Wayne AS, Capitini CM, Mackall CL. Immunotherapy of childhood cancer: From biologic understanding to clinical application. Curr Opin Pediatr (2010) 22(1):2–11. doi: 10.1097/MOP.0b013e3283350d3e
51. Pearson ADJ, Rossig C, Lesa G, Diede SJ, Weiner S, Anderson J, et al. Accelerate and European medicines agency paediatric strategy forum for medicinal product development of checkpoint inhibitors for use in combination therapy in paediatric patients. Eur J Cancer (2020) 127:52–66. doi: 10.1016/j.ejca.2019.12.029
52. van der Pal HJ, van Dalen EC, van Delden E, van Dijk IW, Kok WE, Geskus RB, et al. High risk of symptomatic cardiac events in childhood cancer survivors. J Clin Onco: Off J Am Soc Clin Oncol (2012) 30(13):1429–37. doi: 10.1200/jco.2010.33.4730
53. Mardanpour K, Rahbar M, Mardanpour S, Mardanpour N, Rezaei M. Cd8+ T-cell lymphocytes infiltration predict clinical outcomes in wilms’ tumor. Tumour Biol: J Int Soc Oncodevelopmental Biol Med (2020) 42(12):1010428320975976. doi: 10.1177/1010428320975976
54. Hont AB, Cruz CR, Ulrey R, O’Brien B, Stanojevic M, Datar A, et al. Immunotherapy of relapsed and refractory solid tumors with ex vivo expanded multi-tumor associated antigen specific cytotoxic T lymphocytes: A phase I study. J Clin Onco: Off J Am Soc Clin Oncol (2019) 37(26):2349–59. doi: 10.1200/jco.19.00177
55. Maturu P, Jones D, Ruteshouser EC, Hu Q, Reynolds JM, Hicks J, et al. Role of cyclooxygenase-2 pathway in creating an immunosuppressive microenvironment and in initiation and progression of wilms’ tumor. Neoplasia (2017) 19(3):237–49. doi: 10.1016/j.neo.2016.07.009
56. Maturu P, Overwijk WW, Hicks J, Ekmekcioglu S, Grimm EA, Huff V. Characterization of the inflammatory microenvironment and identification of potential therapeutic targets in wilms tumors. Trans Oncol (2014) 7(4):484–92. doi: 10.1016/j.tranon.2014.05.008
57. Cacciavillano W, Sampor C, Venier C, Gabri MR, de Dávila MT, Galluzzo ML, et al. A phase I study of the anti-idiotype vaccine racotumomab in neuroblastoma and other pediatric refractory malignancies. Pediatr Blood Cancer (2015) 62(12):2120–4. doi: 10.1002/pbc.25631
58. Mulens V, de la Torre A, Marinello P, Rodríguez R, Cardoso J, Díaz R, et al. Immunogenicity and safety of a Neugcgm3 based cancer vaccine: Results from a controlled study in metastatic breast cancer patients. Hum Vaccines (2010) 6(9):736–44. doi: 10.4161/hv.6.9.12571
59. Palmisani F, Kovar H, Kager L, Amann G, Metzelder M, Bergmann M. Systematic review of the immunological landscape of wilms tumors. Mol Ther Oncolytics (2021) 22:454–67. doi: 10.1016/j.omto.2021.06.016
Keywords: malignant blastomas, advanced immunotherapy, monoclonal antibodies, chimeric-antigen based receptor cell therapy, childhood
Citation: Zang B, Ding L, Liu L, Arun Kumar S, Liu W, Zhou C and Duan Y (2023) The immunotherapy advancement targeting malignant blastomas in early childhood. Front. Oncol. 13:1015115. doi: 10.3389/fonc.2023.1015115
Received: 09 August 2022; Accepted: 12 January 2023;
Published: 16 February 2023.
Edited by:
Peter Brossart, University of Bonn, GermanyReviewed by:
Michael C Burger, Goethe University Frankfurt, GermanyQuan Cheng, Xiangya Hospital, Central South University, China
Copyright © 2023 Zang, Ding, Liu, Arun Kumar, Liu, Zhou and Duan. This is an open-access article distributed under the terms of the Creative Commons Attribution License (CC BY). The use, distribution or reproduction in other forums is permitted, provided the original author(s) and the copyright owner(s) are credited and that the original publication in this journal is cited, in accordance with accepted academic practice. No use, distribution or reproduction is permitted which does not comply with these terms.
*Correspondence: Yongtao Duan, ZHVhbnlvbmd0YW84NjA0MDlAMTYzLmNvbQ==; Chongchen Zhou, emhvdWNob25nY2hlbkAxNjMuY29t
†These authors have contributed equally to this work and share first authorship