- 1Department of Oncology, The Third Affiliated Hospital of Soochow University, Changzhou, China
- 2Respiratory Division, The Second Hospital of Hebei Medical University, Shijiazhuang, China
- 3Department of Dermatology, First People’s Hospital, Huzhou, China
- 4Department of Surgery, Huzhou Central Hospital, Huzhou, China
- 5Department of Tumor Biological Treatment, The Third Affiliated Hospital of Soochow University, Changzhou, China
Nowadays, breast cancer has become the most common cancer worldwide with a high mortality rate. Immune checkpoint blockade holds great promise in tumor‐targeted therapy, and CD47 blockade as one immune therapy is undergoing various preclinical studies and clinical trials to demonstrate its safety and efficacy in breast cancer. In this review, we summarized different therapeutic mechanisms targeting CD47 and its prognostic role and therapeutic value in breast cancer.
Introduction
Breast cancer has become the most common cancer worldwide, with nearly 2.3 million new cases in 2020 (1). Despite significant advances in diagnostic techniques and treatment modalities, breast cancer mortality remains high with more than 600 000 patients dying each year (2). Therefore, novel and more effective therapies are still urgently in need.
Since the functional change of immune system plays an important role in the occurrence and progression of breast cancer, immunotherapy especially the blockade of immune checkpoints leads to new breakthroughs (3–5). The development of immune checkpoint inhibitors (ICIs) targeting the adaptive immune system, such as programmed cell death protein-1 (PD-1) and its ligand PD-L1, and cytotoxic T-lymphocyte-associated antigen 4 (CTLA-4), has improved outcomes in patients with advanced metastatic breast cancer and triple-negative breast cancer (TNBC) (6, 7). Although ICIs monotherapy can enhance T cell-mediated immunity, the overall response rate (ORR) is generally less than 30% (7–11). The inhibition of immune checkpoints targeting the innate immune system offers a new solution. Increasing evidence indicates that CD47 acts as a dominant “don’t eat me” signal, enabling tumor cells to escape from macrophage-mediated phagocytosis (12–14). Currently, CD47 is an attractive target for the development of new anti-cancer therapeutics, including options against breast cancer.
Structure and Biological Function of CD47
CD47, originally found to be expressed on red blood cells (RBCs), is a 50 kDa transmembrane protein known as integrin-associated protein (IAP) (15). Structurally, CD47 consists of an extracellular N-terminal IgV domain, five highly hydrophobic transmembrane segments, and a short cytoplasmic tail (16).
It was not until 1999 that CD47 was identified as a ligand of signal regulatory protein-alpha (SIRPα) expressed on myeloid cells, including macrophages (17). The extracellular IgV domain of CD47 binds to SIRPα and initiates the phosphorylation of two tyrosine residues from immunoreceptor tyrosine-based inhibitory motif (ITIM) in the intracellular domain of SIRPα (18) (19–21). The phosphorylation of ITIM subsequently recruits and activates phosphatases SHP1 and SHP2 (22–24). This signaling cascade results in the dephosphorylation of myosin IIA, thereby inhibiting cytoskeleton rearrangement, which is a necessary step for macrophage phagocytosis of target cells (25) (Figure 1).
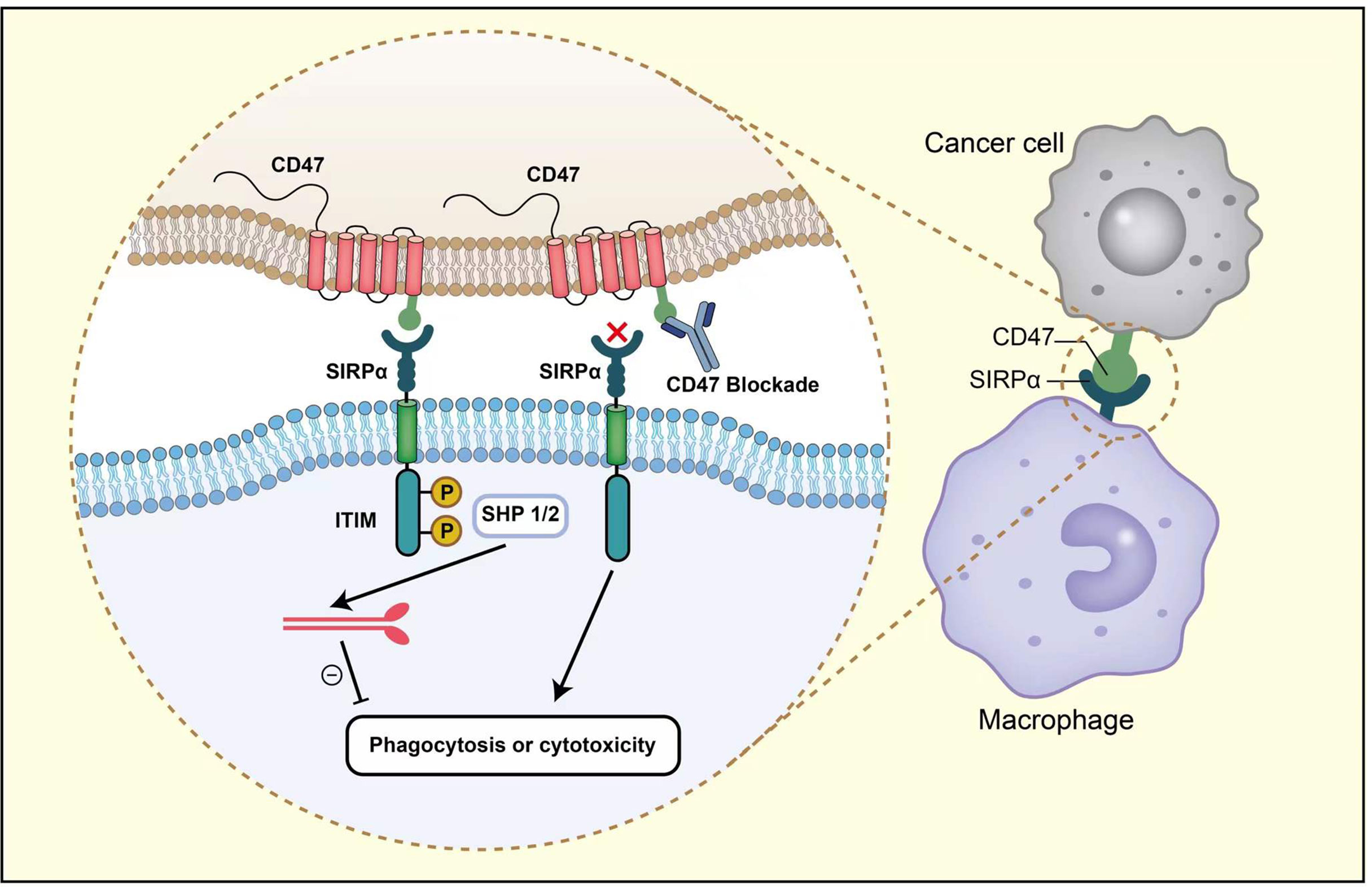
Figure 1 After the interaction between CD47 and SIRPα, two tyrosine residues from ITIM in the intracellular domain of SIRPα become phosphorylated. The phosphorylation activates SHP1 and SHP2, leading to the dephosphorylation of myosin IIA, thereby preventing macrophage phagocytosis. Anti-CD47 antibodies could block CD47-SIRPα axis and promote phagocytosis.
The role of CD47 in immune recognition and phagocytosis was first described by Oldenborg et al. that red blood cells (RBCs) from CD47-/- mice were rapidly cleared when infused into wild-type recipient mice, and this effect was reversed when macrophages were depleted with clodronate liposome (26). Another study found that when RBCs senesce, CD47 expression decreased, and senescent erythrocytes lacking CD47 were considered ‘foreign’ and were rapidly cleared by macrophages in the spleen (27). These results showed that erythrocyte survival was highly dependent on CD47.
Accumulating data suggest that the CD47-SIRPα axis plays an important role in suppressing tumor phagocytosis by regulating the innate immune response. Knauf S et al. first identified the expression of CD47 on ovarian tumors as early as 1986 (28), and a series of studies subsequently confirmed that CD47 was highly expressed in both hematological and solid malignancies, including non-Hodgkin’s lymphoma (NHL) (29), chronic myeloid leukemia (CML) (17), myeloma (30), osteosarcoma (31), breast cancer (32), and other solid tumors. Overexpressed CD47 interacts with SIRPα on myeloid cells to help multiple malignant tumors escape immunosurveillance (33). The disruption of CD47-SIRPα axis leads to the failure of SIRPα phosphorylation, thereby promoting phagocytosis by macrophages. During this process, antigen-presenting cells (APCs) also initiate cross-priming, activating the adaptive immune system (34). Numerous studies have shown that anti-CD47 antibody significantly enhanced the function of macrophage phagocytosis (35, 36), dendritic cell (DC) antigen presentation (37, 38), and NK cell-mediated killing (39). Overall, CD47-SIRPα axis may mediate the link between innate and adaptive immunity.
Regulation of CD47 Expression in Breast Cancer
At the transcriptional level, more understanding about the regulation of CD47 expression in breast cancer has been described. The stimulation of tumor necrosis factor (TNF) inflammatory pathway activates nuclear factor-κB (NF-κB), which directly binds to a super enhancer (SE) site near the CD47 gene, promoting CD47 gene transcription. Contrarily, the blockade of TNF-α signaling has been shown to reduce CD47 expression and induce macrophage phagocytosis (40). Hypoxia-inducible factor 1 (HIF-1) binds to the CD47 promoter, activating gene transcription and increasing CD47 expression in breast cancer cells. Moreover, when cocultured with HIF-1-deficient breast cancer cells, the phagocytosis of macrophages was significantly enhanced (41). Using human and mouse models of leukemia and lymphoma, Casey et al. observed that MYC induced the transcription of both CD47 and PD-L1 (42). Notably, the regulatory effect of MYC on CD47 in breast cancer requires further studies. In conclusion, more understanding of CD47 expression regulation is very meaningful for optimizing CD47-related tumor targeted therapeutics.
CD47 Expression in Breast Cancer and its Correlation With Clinical Outcome
Although CD47 expression is shown to be associated with the development of numerous tumors (43–45), its role in breast cancer is less-well characterized. Next, we summarized the currently available data in breast cancer.
In 2010, Nagahara et al. first reported CD47 as a prognosis biomarker of breast cancer; compared with controls, breast cancer patients have higher CD47 mRNA, and the high CD47 expression in bone marrow were correlated with poor survival. They believed that determining CD47 expression levels in bone marrow or peripheral blood contributed to predict the number of circulating tumor cells that escaped from the immune system, which is indicative of the presence of micrometastases (46). Yuan et al. evaluated CD47 expression using immunohistochemistry and observed that CD47 expression in breast cancer samples was significantly associated with advanced tumor node metastasis (TNM) stage, histological grade, estrogen receptor (ER) status, progesterone receptor (PR) status, and recurrence. However, high CD47 expression had a limited correlation with reduced 5-year disease-free survival (47). An analysis of 353 breast cancer patients and a public data set showed that the high CD47 mRNA levels were correlated with poor-prognosis molecular subtypes (basal, Her2/Neu+) and adverse clinicopathological parameters (high-grade, ER-, PR-). Moreover, in Her2/neu+ breast cancer patients treated with trastuzumab plus vinorelbine, the expression level of CD47 was negatively correlated with the pathological response to treatment, and CD47 was significantly reduced in the complete responders (48). By analyzing two independent datasets of 1954 breast cancer patients, Zhang et al. demonstrated that an increase in CD47 mRNA was associated with a significant decrease in overall survival (OS). The authors also reported that HIF-1 raised CD47 expression to promote breast cancer cells escape from macrophage phagocytosis (41). In a study by Baccelli et al., there was a 7.4-year difference in mean OS between CD47 positive and negative patients. Moreover, CD47 was strongly associated with lymph node metastasis (49).
In triple-negative breast cancer, CD47 expression showed 2.3-fold higher in cancer stem cells (CSCs) than the normal counterparts by Gene Set Enrichment Analysis, and this upregulation was closely related to tumor growth (50). One study revealed that CSCs increased CD47 expression to avoid immune-mediated elimination during conventional anti-tumor therapy (51). When CD47 declined, CSCs were significantly reduced in a dose-dependent manner (41, 52). Yuan et al. examined CD47 expression in 97 breast cancer tissues, and they reported that the positive rate of CD47 in TNBC tissue was significantly higher than that in benign breast lesions, and CD47 overexpression positively correlated with TNBC metastasis and recurrence (53). Many other studies have also shown that CD47 was highly expressed in breast tumors, especially in TNBCs (54). Baccelli et al. demonstrated that overexpressed biomarkers including CD47, EpCAM, CD44, and MET in breast CSCs were strongly associated with decreased OS and increased number of metastatic sites in metastatic breast cancer (55).
Mechanism of Action and Implications of Targeting CD47-SIRPα Axis in the Breast Cancer Microenvironment
The occurrence and metastasis of tumors are closely related to the internal and external environment of tumor cells, which refers to tumor microenvironment. Tumor cells can maintain the survival condition through autocrine and paracrine. Additionally, by changing the microenvironment through immunity, the body can restrict and affect tumor development. Tumor microenvironment is now recognized as a potential therapeutic target. The inhibition of CD47-SIRPα axis in the tumor microenvironment facilitates the elimination of cancer cells mainly through the following four pathways (Figure 2).
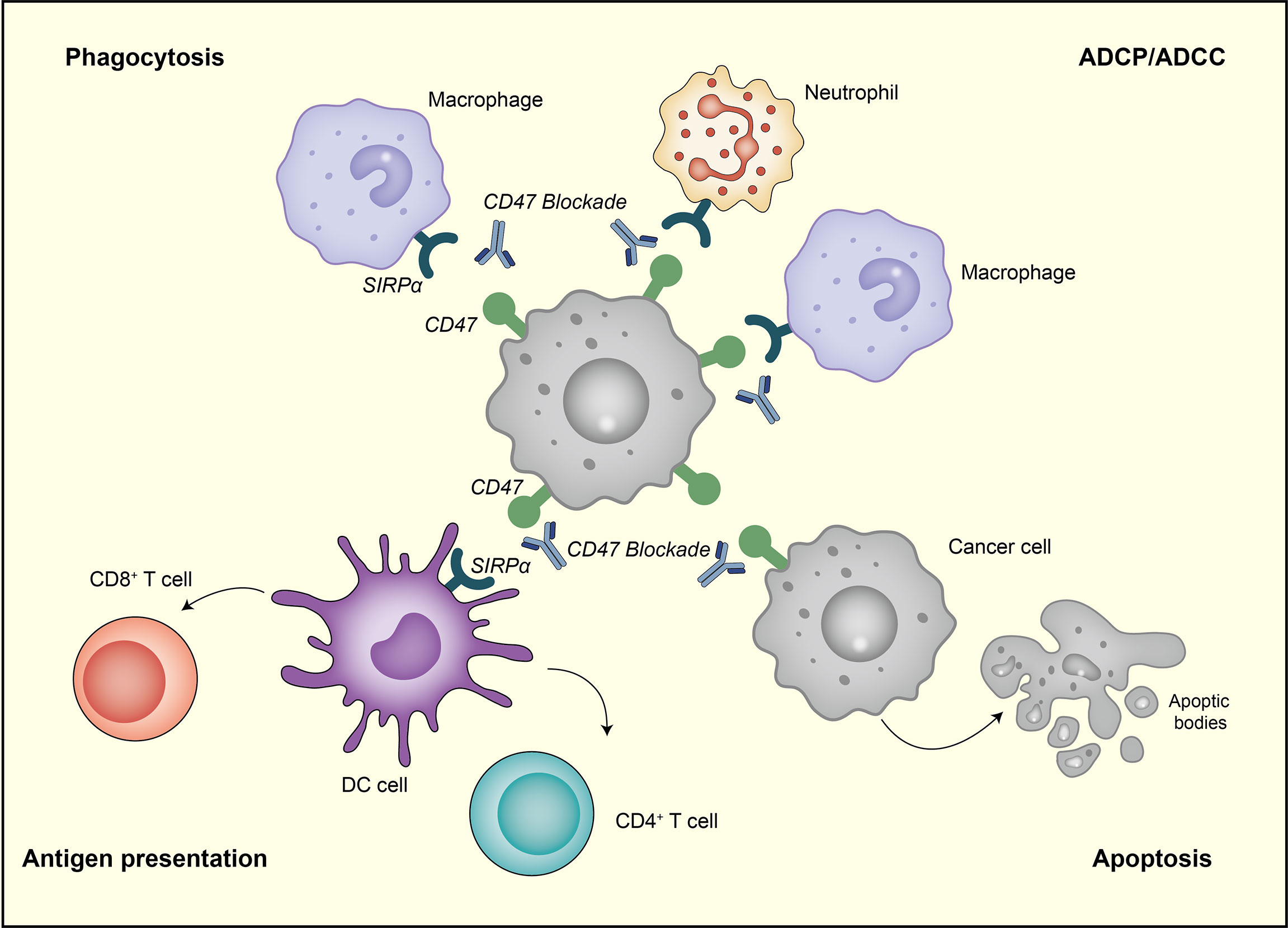
Figure 2 The therapeutic targeting of CD47-SIRPα pathway can cause the elimination of breast cancer cells through the following four pathways. First, the inhibition of CD47-SIRPα could enhance tumor cell phagocytosis by macrophage. Second, anti-CD47 antibody enables the phagocytic uptake of tumor cells by dendritic cells and subsequent antigen presentation to CD4 + and CD8 + T cells, thereby stimulating anti-tumor adaptive immune response. Third, anti-CD47 antibody eliminates tumor cells via traditional Fc-dependent mechanisms, including ADCC and ADCP. Fourth, anti-CD47 antibody stimulates tumor cell apoptosis through a caspase-independent mechanism.
In the first pathway, anti-CD47 antibody disrupts anti−engulfment signal, promoting M1/M2 macrophages-mediated phagocytosis and shifting the immunosuppressive phenotype of tumor-associated macrophages (TAMs) toward M1 subtype in vivo (32, 56). Feliz-Mosquea et al. reported that CD47 blockade significantly increased macrophage infiltration and phagocytosis on breast cancer cells (57). Zhang et al. came to a similar conclusion that the knockdown of CD47 expression increased macrophage-mediated cytotoxicity toward breast cancer cells, and the level of CD47 was negatively correlated with the degree of phagocytosis (41).
In the second pathway, the inhibition of CD47-SIRPα axis enhances the antigen presentation ability of DC and antigen is subsequently presented to CD4+ and CD8+ T cells, leading to the activation of adaptive immune response (58, 59). Recently, Kosaka et al. suggested that the combination treatment of cGAMP and anti-CD47 mAb induced effective anti-tumor immune responses through the activation of monocyte/macrophage phagocytosis and adaptive immune response, which relied on STING and type I IFN signaling. This combination therapy also leads to immune memory and systemic anti-tumor immune responses (60).
In the third pathway, anti-CD47 antibody could eliminate breast cancer cells via traditional Fc-dependent mechanisms, including neutrophil-mediated antibody-dependent cellular cytotoxicity (ADCC) and macrophage-mediated antibody-dependent cellular phagocytosis (ADCP) (12, 61). A study by Matlung et al. demonstrated that targeting CD47-SIRPα could further improve ADCC by a cytotoxicity mechanism identified as trogoptosis (62). Zhao et al. proved that B6H12, a murine antibody against human CD47, can enhance ADCC activity (48). In preclinical models of HER2+ breast cancer, CD47 blockade significantly increased ADCP and enhanced trastuzumab therapeutic outcomes (63).
In the fourth pathway, the inhibition of CD47 or SIRPα can induce tumor cell apoptosis (64, 65), which could be attributed to the direct ligation of CD47 rather than the caspase-dependent pathway (66, 67). In breast cancer, anti-CD47 antibody mediates direct apoptosis of tumor cells, involving the regulation of cAMP levels via heterotrimeric Gi with subsequent effects mediated by PKA. Notably, this effect can be effectively blocked by any drug that maintains intracellular cAMP levels and PKA activity (68).
Targeting CD47 in Breast Cancer Treatments
While SIRPα-CD47 signaling cascade remains incompletely understood, the value of targeting CD47 in tumor treatment has been increasingly confirmed, and the clinical studies on CD47 mAbs have made rapid progress (Table 1). Here, we reviewed and summarized recent advances in CD47 antibodies in breast cancer treatment (Table 2).
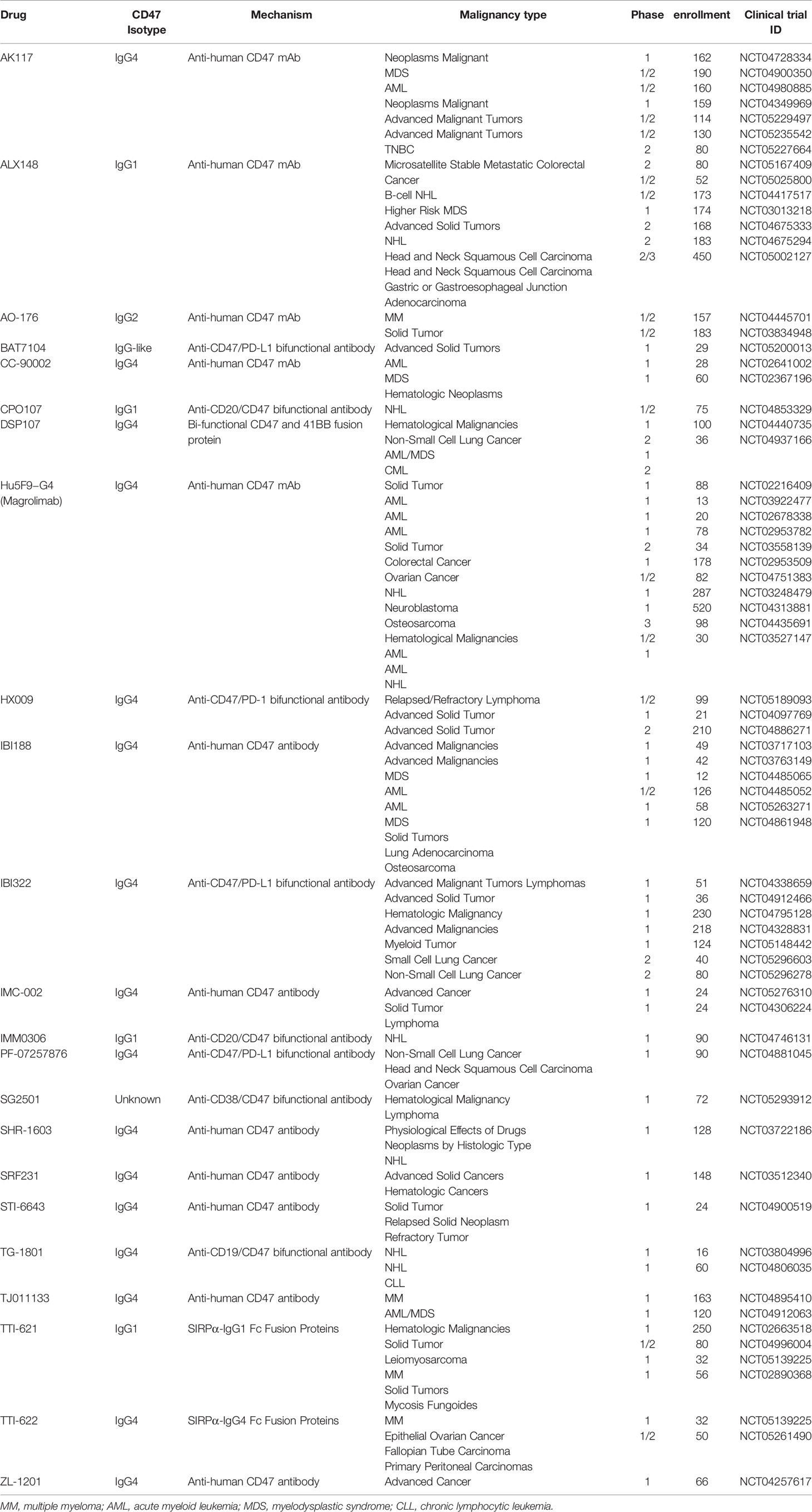
Table 1 Clinical trials targeting CD47 registered with the National Clinical Trials Registry (NCT) system.
Anti-CD47 Antibody Monotherapy
In 2004, Manna et al. found that anti-CD47 mAb 1F7 could cause the death of four different breast cancer cell lines (68). Kaur et al. showed that CD47 blockade inhibited breast CSCs proliferation and asymmetric cell division (50). It is well known that CSCs play an important role in tumor survival, proliferation, metastasis, and recurrence.
Iribarren et al. demonstrated that the monotherapy of CD47 antibody could effectively reduce tumor growth and increase overall survival in AT3 breast cancer model. Regulatory T cells (Tregs) are involved in tumor development and progression by inhibiting antitumor immunity. Of note, this treatment results in a partial reduction of M2 macrophages and almost complete elimination of immunosuppressive Tregs, suggesting that CD47 blockade remodels the tumor microenvironment (69). This elimination might be attributed to CD47 expression on Tregs, and anti-CD47 antibody would increase ADCP of the targeted Tregs (75).
In orthotopic mouse breast cancer model, anti-CD47 antibody inhibited tumor growth and prevented metastasis on larger tumors, and may be curative on smaller tumors; Importantly, anti-CD47 mAbs produced no unacceptable toxicity in immune competent mice, albeit with a temporary anemia, indicating the safety of targeting CD47 (32).
Anti-CD47 Antibody in Combination With Chemotherapy
It is important to point out that targeting CD47 can immensely enhance the anti-tumor effect of other therapeutic strategies.
Calreticulin (CRT) is the dominant pro-phagocytic signal on multiple human cancers, which facilitates cell clearance by engaging its counter receptor LDL-receptor-related protein (LRP) on phagocytes. The balance between antiphagocytic signal (i.e., CD47) and pro-phagocytic signal (i.e., CRT) ultimately determines if cancer cells will be phagocyted or not (76, 77). Anthracyclines induce the rapid translocation of CRT to the cell surface, thereby increasing the immunogenicity of tumors (78). The blockade of CD47 in combination with anthracyclines results in the activation of immunogenic cell death pathway and enhances tumor ablation in vivo (57). Iribarren et al. observed that anti-CD47 antibodies and anthracycline mitoxantrone (MTX) could be favorably combined against carcinogen-induced breast cancers, and this synergistic effect inhibited tumor growth more significantly than either treatment alone (69). Feliz-Mosquea et al. used a 2-dimensional high-throughput cell proliferation assay in mouse 4T1 breast cancer model, and they concluded that targeting CD47 could reduce breast cancer growth and metastasis by activating anti-tumor innate immune response, thereby enhancing the efficacy of doxorubicin chemotherapy in vivo. In addition, anti-CD47 antibodies prevent anthracycline-mediated cardiotoxicity and tissue toxicity (57).
Recently, Cao et al. demonstrated that the combination of CD47 blockade and cabazitaxel, an FDA-approved chemotherapeutic agent (79), produced a potent anticancer effect in TNBC preclinical models, promoting Programmed Cell Removal (PrCR) of cancer cells, and inhibiting tumor development and metastasis; while the efficacy of CD47 antibody alone in inducing PrCR was not satisfactory. More importantly, they discovered that the anti-cancer effect of cabazitaxel in TNBC was due to macrophage activation rather than drug cytotoxicity toward cancer cells. Hence, the combination of CD47 blockade and cabazitaxel may be an effective strategy for TNBC treatment (54).
Numerous studies found that CD47 expression was upregulated in chemotherapy-treated TNBC cells (80, 81). Si et al. developed an innovative antibody-drug conjugates (ADCs) constructed from a specific anti-CD47 mAb and the potent cytotoxic drug-mertansine for the treatment of TNBCs following the standard cytotoxic chemotherapies. Compared with free drug (single drug not conjugated to antibody), ADC showed higher tumor suppressor potency with reduced IC50, and significantly inhibited tumor growth after chemotherapy in TNBC mouse models. Moreover, the whole blood analysis indicated that the new anti-CD47 mAb had no general immune toxicity (70).
Anti-CD47 Antibody in Combination With Tumor-Targeting Antibodies
Anti-CD47 antibody can also be used with biologics in addition to the combination with regular chemotherapy.
In the study of Weiskopf K et al., using the Her2/neu+ breast cancer cell line for phagocytosis determination, the combination of trastuzumab (an anti-HER2 antibody) and high-affinity recombinant SIRPα protein FD6 or CV1 resulted in the highest level of phagocytosis, which was significantly higher than the additive effect of either agent administered alone. Furthermore, CV1-monomer combined with trastuzumab completely eliminated tumors in breast cancer xenograft model using the humanized NOD/SCID/IL-2 receptor gamma-chain(null) (NSG) mice (71).
During radiotherapy, tumors can gradually adapt to changes in the physical and chemical environment and develop radioresistance, which is the main reason for the failure of clinical radiotherapy. Candas-Green et al. found that the aggressive behavior of radioresistant breast cancer was caused by CD47-mediated anti-phagocytosis conjugated with HER2-prompted proliferation. In vivo experiments, the dual inhibition of CD47 and HER2 can effectively increase the radiosensitivity of radiotherapy-resistant tumors and enhance the phagocytosis of tumor cells by macrophages (72).
Impairments in trastuzumab-mediated ADCC may lead to relative resistance to trastuzumab in advanced-stage HER2+ breast cancer patients (82, 83). Trastuzumab could engage Fc-γ receptors (FcγR) on macrophages and promote ADCP, which can be enhanced by anti-CD47 antibody (84). The combination of anti-CD47 antibody and trastuzumab significantly suppressed the growth of ADCC-tolerant HER2+ breast cancers, which could represent a potential new treatment option for HER2+ breast cancer patients (73).
Similarly, in a study by Tsao et al., anti-CD47 antibody significantly enhanced trastuzumab-mediated ADCP and promoted TAM expansion and activation. In addition, CD47 expression was inversely associated with the survival of HER2+ breast cancer patients, and the tumors in human HER2+ breast cancer xenografts models treated with trastuzumab plus CD47 inhibition showed complete regression (63).
The highly immunosuppressive microenvironment after surgery is critical for the recurrence and metastasis of breast cancer. Recently, Huang et al. designed an injectable Double-Layer-Gel (DLG) matrix for postsurgical treatment of breast cancer. The outer layer of DLG could release sorafenib first, which reeducates TAMs and promotes an immunogenic tumor microenvironment. The inner layer, loaded with anti-CD47 antibody, enabled the sustained release of anti-CD47 antibody. They demonstrated that in breast cancer mouse model, the DLG-based strategy efficiently prevented tumor recurrence and metastasis by locally reversing immunosuppression and synergistically blocking CD47-dependent immune escape (74).
Biosafety Problems and Future Perspectives
Due to the ubiquitous expression in normal cells (85), anti-CD47 antibodies could cause possible off-target effects, such as anemia, thrombocytopenia, and leukopenia (76). One study suggested that Hu5F9-G4, an anti-CD47 antibody, alone or in combination with other antibodies may accidentally kill normal erythrocytes, leading to anemia (86). To alleviate this adverse effect, Advani et al., proposed to give short priming low-dose of Hu5F9-G4 in combination with rituximab to selectively eliminate the aged RBCs, thereby inducing compensatory hematopoiesis (87). The wide expression of CD47 also creates an “antigen sink”, which means that larger initiation doses and/or frequenter administrations may be required to achieve effective blockade. Thus, there is an ongoing need to exploit safer solutions to overcome toxicities, and several strategies have been developed to address these issues by selectively binding to CD47 on tumor cells, including the identification of tumor-specific CD47 epitopes and the designs of bispecific antibody.
Although single CD47-targeted agents may have significant efficacy in breast cancer, data from immunocompetent mice and breast cancer xenograft models suggest that combination therapy is still required. Presently, this synergy has been shown to be effective in preclinical models, such as anti-CD47 therapy combined with chemotherapy or immune checkpoint inhibition agents. Future advances in cancer screening and precision medicine would help define which type and stage of breast cancer is most amenable to be treated with one or more specific types of anti-CD47 therapies.
Conclusions
In conclusion, CD47 is a novel attractive target for the treatment of breast cancer, which functions as ‘don’t eat me’ signal to assist cancer cells to escape immunosurveillance. Strategies targeting the CD47-SIRPα axis demonstrate promising results for breast cancer treatment. However, there are a series of biosafety problems with such treatments, and further clinical trials are needed to determine the clinical efficacy of these strategies.
Author Contributions
All authors listed have made a substantial, direct, and intellectual contribution to the work and approved it for publication.
Funding
This work was supported by the National Key R&D Program [grant number 2018YFC1313400]; the Joint Research Fund for Overseas Chinese, Hong Kong and Macao Scholars [grant number 31729001]; the National Natural Science Foundation of China [grant numbers 81972869, 81902386].
Conflict of Interest
The authors declare that the research was conducted in the absence of any commercial or financial relationships that could be construed as a potential conflict of interest.
Publisher’s Note
All claims expressed in this article are solely those of the authors and do not necessarily represent those of their affiliated organizations, or those of the publisher, the editors and the reviewers. Any product that may be evaluated in this article, or claim that may be made by its manufacturer, is not guaranteed or endorsed by the publisher.
References
1. Sung H, Ferlay J, Siegel RL, Laversanne M, Soerjomataram I, Jemal A, et al. Global Cancer Statistics 2020: Globocan Estimates of Incidence and Mortality Worldwide for 36 Cancers in 185 Countries. CA: Cancer J Clin (2021) 71(3):209–49. doi: 10.3322/caac.21660
2. Noguchi E, Shien T, Iwata H. Current Status of Pd-1/Pd-L1 Blockade Immunotherapy in Breast Cancer. Japanese J Clin Oncol (2021) 51(3):321–32. doi: 10.1093/jjco/hyaa230
3. Couzin-Frankel J. Breakthrough of the Year 2013. Cancer Immunother Sci (New York NY) (2013) 342(6165):1432–3. doi: 10.1126/science.342.6165.1432
4. Adams S, Gatti-Mays ME, Kalinsky K, Korde LA, Sharon E, Amiri-Kordestani L, et al. Current Landscape of Immunotherapy in Breast Cancer: A Review. JAMA Oncol (2019) 5(8):1205–14. doi: 10.1001/jamaoncol.2018.7147
5. Vonderheide RH, Domchek SM, Clark AS. Immunotherapy for Breast Cancer: What Are We Missing? Clin Cancer Res an Off J Am Assoc Cancer Res (2017) 23(11):2640–6. doi: 10.1158/1078-0432.Ccr-16-2569
6. Kwapisz D. Pembrolizumab and Atezolizumab in Triple-Negative Breast Cancer. Cancer immunol immunother CII (2021) 70(3):607–17. doi: 10.1007/s00262-020-02736-z
7. Heeke AL, Tan AR. Checkpoint Inhibitor Therapy for Metastatic Triple-Negative Breast Cancer. Cancer metastasis Rev (2021) 40(2):537–47. doi: 10.1007/s10555-021-09972-4
8. Yu WB, Ye ZH, Chen X, Shi JJ, Lu JJ. The Development of Small-Molecule Inhibitors Targeting Cd47. Drug Discovery Today (2021) 26(2):561–8. doi: 10.1016/j.drudis.2020.11.003
9. Masoumi E, Tahaghoghi-Hajghorbani S, Jafarzadeh L, Sanaei MJ, Pourbagheri-Sigaroodi A, Bashash D. The Application of Immune Checkpoint Blockade in Breast Cancer and the Emerging Role of Nanoparticle. J Controlled release Off J Controlled Release Soc (2021) 340:168–87. doi: 10.1016/j.jconrel.2021.10.018
10. Brahmer JR, Tykodi SS, Chow LQ, Hwu WJ, Topalian SL, Hwu P, et al. Safety and Activity of Anti-Pd-L1 Antibody in Patients With Advanced Cancer. New Engl J Med (2012) 366(26):2455–65. doi: 10.1056/NEJMoa1200694
11. Topalian SL, Hodi FS, Brahmer JR, Gettinger SN, Smith DC, McDermott DF, et al. Safety, Activity, and Immune Correlates of Anti-Pd-1 Antibody in Cancer. New Engl J Med (2012) 366(26):2443–54. doi: 10.1056/NEJMoa1200690
12. McCracken MN, Cha AC, Weissman IL. Molecular Pathways: Activating T Cells After Cancer Cell Phagocytosis From Blockade of Cd47 “Don't Eat Me” Signals. Clin Cancer Res an Off J Am Assoc Cancer Res (2015) 21(16):3597–601. doi: 10.1158/1078-0432.Ccr-14-2520
13. Vonderheide RH. Cd47 Blockade as Another Immune Checkpoint Therapy for Cancer. Nat Med (2015) 21(10):1122–3. doi: 10.1038/nm.3965
14. Melo Garcia L, Barabé F. Harnessing Macrophages Through the Blockage of Cd47: Implications for Acute Myeloid Leukemia. Cancers (2021) 13(24). doi: 10.3390/cancers13246258
15. Brown E, Hooper L, Ho T, Gresham H. Integrin-Associated Protein: A 50-Kd Plasma Membrane Antigen Physically and Functionally Associated With Integrins. J Cell Biol (1990) 111(6 Pt 1):2785–94. doi: 10.1083/jcb.111.6.2785
16. Reinhold MI, Lindberg FP, Plas D, Reynolds S, Peters MG, Brown EJ. In Vivo Expression of Alternatively Spliced Forms of Integrin-Associated Protein (Cd47). J Cell Sci (1995) 108(Pt 11):3419–25. doi: 10.1242/jcs.108.11.3419
17. Seiffert M, Cant C, Chen Z, Rappold I, Brugger W, Kanz L, et al. Human Signal-Regulatory Protein Is Expressed on Normal, But Not on Subsets of Leukemic Myeloid Cells and Mediates Cellular Adhesion Involving Its Counterreceptor Cd47. Blood (1999) 94(11):3633–43. doi: 10.1182/blood.V94.11.3633
18. Barclay AN, Brown MH. The Sirp Family of Receptors and Immune Regulation. Nat Rev Immunol (2006) 6(6):457–64. doi: 10.1038/nri1859
19. Kharitonenkov A, Chen Z, Sures I, Wang H, Schilling J, Ullrich A. A Family of Proteins That Inhibit Signalling Through Tyrosine Kinase Receptors. Nature (1997) 386(6621):181–6. doi: 10.1038/386181a0
20. Han X, Sterling H, Chen Y, Saginario C, Brown EJ, Frazier WA, et al. Cd47, a Ligand for the Macrophage Fusion Receptor, Participates in Macrophage Multinucleation. J Biol Chem (2000) 275(48):37984–92. doi: 10.1074/jbc.M002334200
21. Vernon-Wilson EF, Kee WJ, Willis AC, Barclay AN, Simmons DL, Brown MH. Cd47 Is a Ligand for Rat Macrophage Membrane Signal Regulatory Protein Sirp (Ox41) and Human Sirpalpha 1. Eur J Immunol (2000) 30(8):2130–7. doi: 10.1002/1521-4141(2000)30:8<2130::Aid-immu2130>3.0.Co;2-8
22. Fujioka Y, Matozaki T, Noguchi T, Iwamatsu A, Yamao T, Takahashi N, et al. A Novel Membrane Glycoprotein, Shps-1, That Binds the Sh2-Domain-Containing Protein Tyrosine Phosphatase Shp-2 in Response to Mitogens and Cell Adhesion. Mol Cell Biol (1996) 16(12):6887–99. doi: 10.1128/mcb.16.12.6887
23. Noguchi T, Matozaki T, Fujioka Y, Yamao T, Tsuda M, Takada T, et al. Characterization of a 115-Kda Protein That Binds to Sh-Ptp2, a Protein-Tyrosine Phosphatase With Src Homology 2 Domains, in Chinese Hamster Ovary Cells. J Biol Chem (1996) 271(44):27652–8. doi: 10.1074/jbc.271.44.27652
24. Neel BG, Gu H, Pao L. The 'Shp'ing News: Sh2 Domain-Containing Tyrosine Phosphatases in Cell Signaling. Trends Biochem Sci (2003) 28(6):284–93. doi: 10.1016/s0968-0004(03)00091-4
25. Tsai RK, Discher DE. Inhibition of "Self" Engulfment Through Deactivation of Myosin-Ii at the Phagocytic Synapse Between Human Cells. J Cell Biol (2008) 180(5):989–1003. doi: 10.1083/jcb.200708043
26. Oldenborg PA, Zheleznyak A, Fang YF, Lagenaur CF, Gresham HD, Lindberg FP. Role of Cd47 as a Marker of Self on Red Blood Cells. Science (2000) 288(5473):2051–4. doi: 10.1126/science.288.5473.2051
27. Burger P, Hilarius-Stokman P, de Korte D, van den Berg TK, van Bruggen R. Cd47 Functions as a Molecular Switch for Erythrocyte Phagocytosis. Blood (2012) 119(23):5512–21. doi: 10.1182/blood-2011-10-386805
28. Catalán R, Orozco-Morales M, Hernández-Pedro NY, Guijosa A, Colín-González AL, Ávila-Moreno F, et al. Cd47-Sirpα Axis as a Biomarker and Therapeutic Target in Cancer: Current Perspectives and Future Challenges in Nonsmall Cell Lung Cancer. J Immunol Res (2020) 2020:9435030. doi: 10.1155/2020/9435030
29. Chao MP, Tang C, Pachynski RK, Chin R, Majeti R, Weissman IL. Extranodal Dissemination of Non-Hodgkin Lymphoma Requires Cd47 and Is Inhibited by Anti-Cd47 Antibody Therapy. Blood (2011) 118(18):4890–901. doi: 10.1182/blood-2011-02-338020
30. Kim D, Wang J, Willingham SB, Martin R, Wernig G, Weissman IL. Anti-Cd47 Antibodies Promote Phagocytosis and Inhibit the Growth of Human Myeloma Cells. Leukemia (2012) 26(12):2538–45. doi: 10.1038/leu.2012.141
31. Mohanty S, Yerneni K, Theruvath JL, Graef CM, Nejadnik H, Lenkov O, et al. Nanoparticle Enhanced Mri Can Monitor Macrophage Response to Cd47 Mab Immunotherapy in Osteosarcoma. Cell Death Dis (2019) 10(2):36. doi: 10.1038/s41419-018-1285-3
32. Willingham SB, Volkmer JP, Gentles AJ, Sahoo D, Dalerba P, Mitra SS, et al. The Cd47-Signal Regulatory Protein Alpha (Sirpa) Interaction Is a Therapeutic Target for Human Solid Tumors. Proc Natl Acad Sci USA (2012) 109(17):6662–7. doi: 10.1073/pnas.1121623109
33. Matlung HL, Szilagyi K, Barclay NA, van den Berg TK. The Cd47-Sirpα Signaling Axis as an Innate Immune Checkpoint in Cancer. Immunol Rev (2017) 276(1):145–64. doi: 10.1111/imr.12527
34. Feng M, Jiang W, Kim BYS, Zhang CC, Fu YX, Weissman IL. Phagocytosis Checkpoints as New Targets for Cancer Immunotherapy. Nat Rev Cancer (2019) 19(10):568–86. doi: 10.1038/s41568-019-0183-z
35. Weiskopf K, Jahchan NS, Schnorr PJ, Cristea S, Ring AM, Maute RL, et al. Cd47-Blocking Immunotherapies Stimulate Macrophage-Mediated Destruction of Small-Cell Lung Cancer. J Clin Invest (2016) 126(7):2610–20. doi: 10.1172/jci81603
36. Chao MP, Alizadeh AA, Tang C, Myklebust JH, Varghese B, Gill S, et al. Anti-Cd47 Antibody Synergizes With Rituximab to Promote Phagocytosis and Eradicate Non-Hodgkin Lymphoma. Cell (2010) 142(5):699–713. doi: 10.1016/j.cell.2010.07.044
37. Liu X, Pu Y, Cron K, Deng L, Kline J, Frazier WA, et al. Cd47 Blockade Triggers T Cell-Mediated Destruction of Immunogenic Tumors. Nat Med (2015) 21(10):1209–15. doi: 10.1038/nm.3931
38. Soto-Pantoja DR, Terabe M, Ghosh A, Ridnour LA, DeGraff WG, Wink DA, et al. Cd47 in the Tumor Microenvironment Limits Cooperation Between Antitumor T-Cell Immunity and Radiotherapy. Cancer Res (2014) 74(23):6771–83. doi: 10.1158/0008-5472.Can-14-0037-t
39. Kim MJ, Lee JC, Lee JJ, Kim S, Lee SG, Park SW, et al. Association of Cd47 With Natural Killer Cell-Mediated Cytotoxicity of Head-And-Neck Squamous Cell Carcinoma Lines. Tumour Biol J Int Soc Oncodevelopmental Biol Med (2008) 29(1):28–34. doi: 10.1159/000132568
40. Betancur PA, Abraham BJ, Yiu YY, Willingham SB, Khameneh F, Zarnegar M, et al. A Cd47-Associated Super-Enhancer Links Pro-Inflammatory Signalling to Cd47 Upregulation in Breast Cancer. Nat Commun (2017) 8:14802. doi: 10.1038/ncomms14802
41. Zhang H, Lu H, Xiang L, Bullen JW, Zhang C, Samanta D, et al. Hif-1 Regulates Cd47 Expression in Breast Cancer Cells to Promote Evasion of Phagocytosis and Maintenance of Cancer Stem Cells. Proc Natl Acad Sci USA (2015) 112(45):E6215–23. doi: 10.1073/pnas.1520032112
42. Casey SC, Tong L, Li Y, Do R, Walz S, Fitzgerald KN, et al. Myc Regulates the Antitumor Immune Response Through Cd47 and Pd-L1. Sci (New York NY) (2016) 352(6282):227–31. doi: 10.1126/science.aaf7984
43. Majeti R, Chao MP, Alizadeh AA, Pang WW, Jaiswal S, Gibbs KD Jr., et al. Cd47 Is an Adverse Prognostic Factor and Therapeutic Antibody Target on Human Acute Myeloid Leukemia Stem Cells. Cell (2009) 138(2):286–99. doi: 10.1016/j.cell.2009.05.045
44. Li Y, Lu S, Xu Y, Qiu C, Jin C, Wang Y, et al. Overexpression of Cd47 Predicts Poor Prognosis and Promotes Cancer Cell Invasion in High-Grade Serous Ovarian Carcinoma. Am J Trans Res (2017) 9(6):2901–10.
45. Lee TK, Cheung VC, Lu P, Lau EY, Ma S, Tang KH, et al. Blockade of Cd47-Mediated Cathepsin S/Protease-Activated Receptor 2 Signaling Provides a Therapeutic Target for Hepatocellular Carcinoma. Hepatol (Baltimore Md) (2014) 60(1):179–91. doi: 10.1002/hep.27070
46. Nagahara M, Mimori K, Kataoka A, Ishii H, Tanaka F, Nakagawa T, et al. Correlated Expression of Cd47 and Sirpa in Bone Marrow and in Peripheral Blood Predicts Recurrence in Breast Cancer Patients. Clin Cancer Res an Off J Am Assoc Cancer Res (2010) 16(18):4625–35. doi: 10.1158/1078-0432.Ccr-10-0349
47. Yuan J, He H, Chen C, Wu J, Rao J, Yan H. Combined High Expression of Cd47 and Cd68 Is a Novel Prognostic Factor for Breast Cancer Patients. Cancer Cell Int (2019) 19:238. doi: 10.1186/s12935-019-0957-0
48. Zhao XW, van Beek EM, Schornagel K, van der Maaden H, Van Houdt M, Otten MA, et al. Cd47-Signal Regulatory Protein-A (Sirpα) Interactions Form a Barrier for Antibody-Mediated Tumor Cell Destruction. Proc Natl Acad Sci USA (2011) 108(45):18342–7. doi: 10.1073/pnas.1106550108
49. Baccelli I, Stenzinger A, Vogel V, Pfitzner BM, Klein C, Wallwiener M, et al. Co-Expression of Met and Cd47 Is a Novel Prognosticator for Survival of Luminal Breast Cancer Patients. Oncotarget (2014) 5(18):8147–60. doi: 10.18632/oncotarget.2385
50. Kaur S, Elkahloun AG, Singh SP, Chen QR, Meerzaman DM, Song T, et al. A Function-Blocking Cd47 Antibody Suppresses Stem Cell and Egf Signaling in Triple-Negative Breast Cancer. Oncotarget (2016) 7(9):10133–52. doi: 10.18632/oncotarget.7100
51. Soltanian S, Matin MM. Cancer Stem Cells and Cancer Therapy. Tumour Biol J Int Soc Oncodevelopmental Biol Med (2011) 32(3):425–40. doi: 10.1007/s13277-011-0155-8
52. Smigiel JM, Parameswaran N, Jackson MW. Potent Emt and Csc Phenotypes Are Induced by Oncostatin-M in Pancreatic Cancer. Mol Cancer Res MCR (2017) 15(4):478–88. doi: 10.1158/1541-7786.Mcr-16-0337
53. Yuan J, Shi X, Chen C, He H, Liu L, Wu J, et al. High Expression of Cd47 in Triple Negative Breast Cancer Is Associated With Epithelial-Mesenchymal Transition and Poor Prognosis. Oncol Lett (2019) 18(3):3249–55. doi: 10.3892/ol.2019.10618
54. Cao X, Li B, Chen J, Dang J, Chen S, Gunes EG, et al. Effect of Cabazitaxel on Macrophages Improves Cd47-Targeted Immunotherapy for Triple-Negative Breast Cancer. J immunother Cancer (2021) 9(3). doi: 10.1136/jitc-2020-002022
55. Baccelli I, Schneeweiss A, Riethdorf S, Stenzinger A, Schillert A, Vogel V, et al. Identification of a Population of Blood Circulating Tumor Cells From Breast Cancer Patients That Initiates Metastasis in a Xenograft Assay. Nat Biotechnol (2013) 31(6):539–44. doi: 10.1038/nbt.2576
56. Zhang M, Hutter G, Kahn SA, Azad TD, Gholamin S, Xu CY, et al. Anti-Cd47 Treatment Stimulates Phagocytosis of Glioblastoma by M1 and M2 Polarized Macrophages and Promotes M1 Polarized Macrophages in Vivo. PLoS One (2016) 11(4):e0153550. doi: 10.1371/journal.pone.0153550
57. Feliz-Mosquea YR, Christensen AA, Wilson AS, Westwood B, Varagic J, Meléndez GC, et al. Combination of Anthracyclines and Anti-Cd47 Therapy Inhibit Invasive Breast Cancer Growth While Preventing Cardiac Toxicity by Regulation of Autophagy. Breast Cancer Res Treat (2018) 172(1):69–82. doi: 10.1007/s10549-018-4884-x
58. Matozaki T, Murata Y, Okazawa H, Ohnishi H. Functions and Molecular Mechanisms of the Cd47-Sirpalpha Signalling Pathway. Trends Cell Biol (2009) 19(2):72–80. doi: 10.1016/j.tcb.2008.12.001
59. Latour S, Tanaka H, Demeure C, Mateo V, Rubio M, Brown EJ, et al. Bidirectional Negative Regulation of Human T and Dendritic Cells by Cd47 and Its Cognate Receptor Signal-Regulator Protein-Alpha: Down-Regulation of Il-12 Responsiveness and Inhibition of Dendritic Cell Activation. J Immunol (Baltimore Md 1950) (2001) 167(5):2547–54. doi: 10.4049/jimmunol.167.5.2547
60. Kosaka A, Ishibashi K, Nagato T, Kitamura H, Fujiwara Y, Yasuda S, et al. Cd47 Blockade Enhances the Efficacy of Intratumoral Sting-Targeting Therapy by Activating Phagocytes. J Exp Med (2021) 218(11). doi: 10.1084/jem.20200792
61. Sockolosky JT, Dougan M, Ingram JR, Ho CC, Kauke MJ, Almo SC, et al. Durable Antitumor Responses to Cd47 Blockade Require Adaptive Immune Stimulation. Proc Natl Acad Sci USA (2016) 113(19):E2646–54. doi: 10.1073/pnas.1604268113
62. Matlung HL, Babes L, Zhao XW, van Houdt M, Treffers LW, van Rees DJ, et al. Neutrophils Kill Antibody-Opsonized Cancer Cells by Trogoptosis. Cell Rep (2018) 23(13):3946–59.e6. doi: 10.1016/j.celrep.2018.05.082
63. Tsao LC, Crosby EJ, Trotter TN, Agarwal P, Hwang BJ, Acharya C, et al. Cd47 Blockade Augmentation of Trastuzumab Antitumor Efficacy Dependent on Antibody-Dependent Cellular Phagocytosis. JCI Insight (2019) 4(24). doi: 10.1172/jci.insight.131882
64. Chao MP, Weissman IL, Majeti R. The Cd47-Sirpα Pathway in Cancer Immune Evasion and Potential Therapeutic Implications. Curr Opin Immunol (2012) 24(2):225–32. doi: 10.1016/j.coi.2012.01.010
65. Mateo V, Lagneaux L, Bron D, Biron G, Armant M, Delespesse G, et al. Cd47 Ligation Induces Caspase-Independent Cell Death in Chronic Lymphocytic Leukemia. Nat Med (1999) 5(11):1277–84. doi: 10.1038/15233
66. Kikuchi Y, Uno S, Kinoshita Y, Yoshimura Y, Iida S, Wakahara Y, et al. Apoptosis Inducing Bivalent Single-Chain Antibody Fragments Against Cd47 Showed Antitumor Potency for Multiple Myeloma. Leukemia Res (2005) 29(4):445–50. doi: 10.1016/j.leukres.2004.09.005
67. Kikuchi Y, Uno S, Yoshimura Y, Otabe K, Iida S, Oheda M, et al. A Bivalent Single-Chain Fv Fragment Against Cd47 Induces Apoptosis for Leukemic Cells. Biochem Biophys Res Commun (2004) 315(4):912–8. doi: 10.1016/j.bbrc.2004.01.128
68. Manna PP, Frazier WA. Cd47 Mediates Killing of Breast Tumor Cells Via Gi-Dependent Inhibition of Protein Kinase a. Cancer Res (2004) 64(3):1026–36. doi: 10.1158/0008-5472.can-03-1708
69. Iribarren K, Buque A, Mondragon L, Xie W, Lévesque S, Pol J, et al. Anticancer Effects of Anti-Cd47 Immunotherapy in Vivo. Oncoimmunology (2019) 8(3):1550619. doi: 10.1080/2162402x.2018.1550619
70. Si Y, Zhang Y, Guan JS, Ngo HG, Totoro A, Singh AP, et al. Anti-Cd47 Monoclonal Antibody-Drug Conjugate: A Targeted Therapy to Treat Triple-Negative Breast Cancers. Vaccines (2021) 9(8). doi: 10.3390/vaccines9080882
71. Weiskopf K, Ring AM, Ho CC, Volkmer JP, Levin AM, Volkmer AK, et al. Engineered Sirpα Variants as Immunotherapeutic Adjuvants to Anticancer Antibodies. Sci (New York NY) (2013) 341(6141):88–91. doi: 10.1126/science.1238856
72. Candas-Green D, Xie B, Huang J, Fan M, Wang A, Menaa C, et al. Dual Blockade of Cd47 and Her2 Eliminates Radioresistant Breast Cancer Cells. Nat Commun (2020) 11(1):4591. doi: 10.1038/s41467-020-18245-7
73. Upton R, Banuelos A, Feng D, Biswas T, Kao K, McKenna K, et al. Combining Cd47 Blockade With Trastuzumab Eliminates Her2-Positive Breast Cancer Cells and Overcomes Trastuzumab Tolerance. Proc Natl Acad Sci USA (2021) 118(29). doi: 10.1073/pnas.2026849118
74. Huang L, Zhang Y, Li Y, Meng F, Li H, Zhang H, et al. Time-Programmed Delivery of Sorafenib and Anti-Cd47 Antibody Via a Double-Layer-Gel Matrix for Postsurgical Treatment of Breast Cancer. Nano-micro Lett (2021) 13(1):141. doi: 10.1007/s40820-021-00647-x
75. Zhang A, Ren Z, Tseng KF, Liu X, Li H, Lu C, et al. Dual Targeting of Ctla-4 and Cd47 on T(Reg) Cells Promotes Immunity Against Solid Tumors. Sci Trans Med (2021) 13(605). doi: 10.1126/scitranslmed.abg8693
76. Chao MP, Jaiswal S, Weissman-Tsukamoto R, Alizadeh AA, Gentles AJ, Volkmer J, et al. Calreticulin Is the Dominant Pro-Phagocytic Signal on Multiple Human Cancers and Is Counterbalanced by Cd47. Sci Trans Med (2010) 2(63):63ra94. doi: 10.1126/scitranslmed.3001375
77. Feng M, Marjon KD, Zhu F, Weissman-Tsukamoto R, Levett A, Sullivan K, et al. Programmed Cell Removal by Calreticulin in Tissue Homeostasis and Cancer. Nat Commun (2018) 9(1):3194. doi: 10.1038/s41467-018-05211-7
78. Clarke C, Smyth MJ. Calreticulin Exposure Increases Cancer Immunogenicity. Nat Biotechnol (2007) 25(2):192–3. doi: 10.1038/nbt0207-192
79. Tsao CK, Cutting E, Martin J, Oh WK. The Role of Cabazitaxel in the Treatment of Metastatic Castration-Resistant Prostate Cancer. Ther Adv Urol (2014) 6(3):97–104. doi: 10.1177/1756287214528557
80. Nigro A, Ricciardi L, Salvato I, Sabbatino F, Vitale M, Crescenzi MA, et al. Enhanced Expression of Cd47 Is Associated With Off-Target Resistance to Tyrosine Kinase Inhibitor Gefitinib in Nsclc. Front Immunol (2019) 10:3135. doi: 10.3389/fimmu.2019.03135
81. Samanta D, Park Y, Ni X, Li H, Zahnow CA, Gabrielson E, et al. Chemotherapy Induces Enrichment of Cd47(+)/Cd73(+)/Pdl1(+) Immune Evasive Triple-Negative Breast Cancer Cells. Proc Natl Acad Sci USA (2018) 115(6):E1239–e48. doi: 10.1073/pnas.1718197115
82. Li H, Shao B, Yan Y, Song G, Liu X, Wang J, et al. Efficacy and Safety of Trastuzumab Combined With Chemotherapy for First-Line Treatment and Beyond Progression of Her2-Overexpressing Advanced Breast Cancer. Chin J Cancer Res = Chung-kuo yen cheng yen chiu (2016) 28(3):330–8. doi: 10.21147/j.issn.1000-9604.2016.03.07
83. Wilken JA, Maihle NJ. Primary Trastuzumab Resistance: New Tricks for an Old Drug. Ann New York Acad Sci (2010) 1210:53–65. doi: 10.1111/j.1749-6632.2010.05782.x
84. Shi Y, Fan X, Deng H, Brezski RJ, Rycyzyn M, Jordan RE, et al. Trastuzumab Triggers Phagocytic Killing of High Her2 Cancer Cells in Vitro and in Vivo by Interaction With Fcγ Receptors on Macrophages. J Immunol (Baltimore Md 1950) (2015) 194(9):4379–86. doi: 10.4049/jimmunol.1402891
85. Ishikawa-Sekigami T, Kaneko Y, Saito Y, Murata Y, Okazawa H, Ohnishi H, et al. Enhanced Phagocytosis of Cd47-Deficient Red Blood Cells by Splenic Macrophages Requires Shps-1. Biochem Biophys Res Commun (2006) 343(4):1197–200. doi: 10.1016/j.bbrc.2006.03.094
86. Buatois V, Johnson Z, Salgado-Pires S, Papaioannou A, Hatterer E, Chauchet X, et al. Preclinical Development of a Bispecific Antibody That Safely and Effectively Targets Cd19 and Cd47 for the Treatment of B-Cell Lymphoma and Leukemia. Mol Cancer Ther (2018) 17(8):1739–51. doi: 10.1158/1535-7163.Mct-17-1095
Keywords: CD47, SIRPα, breast cancer, immunotherapy, immune checkpoint inhibitors
Citation: Chen C, Wang R, Chen X, Hou Y and Jiang J (2022) Targeting CD47 as a Novel Immunotherapy for Breast Cancer. Front. Oncol. 12:924740. doi: 10.3389/fonc.2022.924740
Received: 20 April 2022; Accepted: 07 June 2022;
Published: 04 July 2022.
Edited by:
Francesco Schettini, Institut de Recerca Biomèdica August Pi i Sunyer (IDIBAPS), SpainReviewed by:
Reona Sakemura, Mayo Clinic, United StatesLinchun Jin, University of Florida, United States
Copyright © 2022 Chen, Wang, Chen, Hou and Jiang. This is an open-access article distributed under the terms of the Creative Commons Attribution License (CC BY). The use, distribution or reproduction in other forums is permitted, provided the original author(s) and the copyright owner(s) are credited and that the original publication in this journal is cited, in accordance with accepted academic practice. No use, distribution or reproduction is permitted which does not comply with these terms.
*Correspondence: Jingting Jiang, jiangjingting@suda.edu.cn