- Institut de Recherches Internationales Servier, Translational Medicine Department, Servier, Suresnes, France
Pancreatic ductal adenocarcinoma (PDAC) is among the most lethal cancers, with a 5-year relative survival rate of 5%. The desmoplastic stroma found in the tumor microenvironment of PDAC is suggested to be partly responsible for the resistance to most therapeutic strategies. This review outlines the clinical results obtained with an immune checkpoint inhibitor in PDAC and discusses the rationale to use a combination of chemotherapy and immune checkpoint therapy. Moreover, essential parameters to take into account in designing an efficient combination have been highlighted.
1 Introduction
Pancreatic ductal adenocarcinoma (PDAC) represents 90% of all pancreatic malignancies. PDAC is currently the fourth most common cancer worldwide with the worst 5-year overall survival (OS) rate of 5% across many solid tumors (1, 2). The PDAC incidence is higher in Western countries than in Asia and Africa and is expected to rise over the coming years due to lifestyle, longer lifespan, and public health problems such as obesity and diabetes (3). In 2030, PDAC would be the second leading cause of cancer-related deaths in the United States (US) (4).
At the time of diagnosis, 80% of newly diagnosed patients present with locally advanced or metastatic disease while only about 20% of PDAC patients are candidates for surgical resection (4). For patients with advanced diseases, adjuvant cytotoxic chemotherapy with drugs such as FOLFIRINOX (5-fluorouracil, leucovorin, irinotecan, oxaliplatin) or nab-paclitaxel and gemcitabine is the current treatment option. Indeed, in a phase 2/3 study (NCT00112658), the FOLFIRINOX combination increased the median OS (11.1 months) and median progression free-survival (PFS) (6.4 months) compared to gemcitabine monotherapy (median OS 6.8 months and median PFS 3.3 months) but was associated with an increase in side effects. This regimen is therefore an option for the treatment of metastatic pancreatic cancer with a good performance status (5). Recently, the combination of nab-paclitaxel and gemcitabine has shown to have superior efficacy in terms of OS (8.5 months), PFS (5.5 months), and overall response rate (ORR) compared with gemcitabine monotherapy (with median OS of 6.7 months and median PFS of 3.7 months) in the MPACT Phase 3 study (NCT00844649) (6). Despite these improvements, medians of survival remain insufficient, which show that efforts must continue to provide new strategies to patients.
In recent years, the discovery of immune checkpoints (ICP) has revolutionized immuno-oncology treatments. It has been shown that tumors develop escape mechanisms to avoid recognition by the immune system by expressing ligands such as PD-L1 which binds co-inhibitory receptors like PD-1. By preventing this interaction, ICP inhibitors restore the activation of the immune system which translates into clinical benefit for about 13% patients in many solid tumors (7). Furthermore, biomarker studies have now described the association between high expression of PD-L1 (8) and/or high microsatellite instability (MSI-H) (9) and the response to these treatments.
The study of the PDAC tumor microenvironment (TME) has highlighted several factors which suggest that immunotherapy could have a clinical impact in this cancer type. First, the presence of an immune infiltrate correlated with the prognosis of patients, suggesting the presence of preexisting antitumor immune responses (10–12). Furthermore, in a study, 1/3 of pancreatic tumors has an immune infiltrate similar to that of melanoma supporting the notion that PDAC is a heterogeneous group of tumors and some patients harbor immunogenic tumors (13). Second, the characterization of the tumor microenvironment has highlighted that the expressions of ICP such as CTLA-4, PD-L1, LAG-3, and TIM-3 are associated with poor survival in PDAC tumors (13–15). Finally, reports have shown the presence of tertiary lymphoid structures (TLS), mostly located in the tumor periphery of PDAC tissues. In accordance with their favorable prognosis role in many solid tumors (16), PDAC tumors harboring TLS are enriched with IgG1 memory B cells and memory CD4+ T-cells and a higher expression of Th1- and Th17-related genes (17, 18) combined with a lower infiltration of immunosuppressive cells. Moreover, these TLS are associated with longer survival for patients in PDAC (17). Recently, numerous papers have highlighted the predictive role of these TLS in the response to ICP inhibitors in solid tumors (19) including melanoma (20), sarcoma (21). These findings suggest that PDAC tumors, or at least some of them, could be responsive to ICP inhibitors.
Despite the presence of biological factors that may suggest a potential response to single-agent ICP inhibitors, PDAC tumors do not respond to these immunotherapies. Indeed, although ipilimumab increased the survival of melanoma patients, this anti-CTLA-4 antibody did not meet its primary endpoint in a phase 2 clinical trial for advanced pancreatic cancer patients (22). The combination of two ICP inhibitors (anti-CTLA-4 + anti-PD-L1) remains ineffective for PDAC patients (23). Multiple hypotheses have arisen to explain this lack of response in PDAC. Understanding these resistance factors is a key element in defining new therapeutic strategies and improving responses to the ICP inhibitors of these cold tumors, also called immunological deserts.
2 Resistance Factors to Immune Checkpoint Therapy in PDAC
2.1 Immune Cell Content
It has been suggested that the TME mediated the suppression of T-cell priming and function in PDAC, thus contributing to resistance to these treatments. Observation of a high density of immunosuppressive cells in the pancreatic intraepithelial neoplasia (PanIN) (24) combined with a dysfunctional T-cell phenotype suggests an impairment of T-cell mediated antitumor responses from the early stages.
It has been shown that tumor-associated macrophages (TAM) accumulate in the stroma of the PDAC TME tumor microenvironment via CCL2 (25) secreted by cancer cells. In the tumor, multiple cells including cancer-associated fibroblasts (CAF), regulatory T-cells (Treg), and Th2 cells promote the TAM polarization toward the M2 phenotype (26). Accordingly, many studies revealed that a high density of TAM is associated with poor survival (27, 28), supporting their involvement in the tumor development and progression. TAM can also contribute to T-cell exclusion from tumor islets (29). These conclusions are in line with observations made in mouse models showing that the depletion of TAM not only impaired PDAC cell proliferation (30) but also induced T-cell recruitment within the tumor bed (31) and restored the antitumor activity of T-cells (32).
A study analyzing the Treg contribution in PDAC supports the notion that myeloid immunosuppressive cells are the most contributor to the tumor progression in PDAC. Interestingly, Treg depletion has failed to inhibit tumor growth due to the establishment of compensatory mechanisms such as an increase of myeloid cells and CAF reprogramming (33). CAFs are known to generate dense fibrosis or desmoplasia within and around the tumor. This desmoplastic stroma is composed of pancreatic stellate cells (PSC), CAF, and extracellular matrix (ECM) components and represents up to 80% of the tumor volume which is a key feature of the PDAC TME. Whether this desmoplastic stroma prevents immune infiltration is still a matter of debate. Indeed, ex vivo models support the notion that excessive collagen deposition impedes T-cell entry into the TME as the collagen degradation has increased T-cell infiltrates (34). However, the study of the spatial relationships between T-cell subpopulations and cancer cells has shown that the desmoplastic stroma has no impact on T-cell infiltration (35). Surprisingly, the depletion of αSMA+ CAF in mice could reduces desmoplasia but enhances hypoxia and epithelial-to-mesenchymal transition, promotes tumor progression, and is associated with reduced survival (36). These findings suggest that desmoplastic stroma is also involved in the control of tumor growth.
2.2 Low Immunogenicity
Immunogenicity is defined as the ability of antigens to induce immune responses. In the TME, some of the somatic mutations occurring in cancer cells generate neoepitopes that can be loaded on to major histocompatibility complex (MHC) molecules and eventually activate specific T-cells. It is likely that tumors with a high number of somatic mutations have statistically more immunogenic neoantigens, that is why the tumor mutational burden (TMB) representing the number of somatic mutations per mega base in the genome of a cancer cell is currently used as an estimation of antigen load in a tumor. However, a recent study has demonstrated that TMB is not a predictive biomarker of ICP-inhibitor therapy for all solid tumors including PDAC, in which CD8 T-cell infiltration is not associated with neoantigen load (37). In the same line, data from long-term survivors in PDAC have shown that neoantigen quality rather than quantity conferred higher tumor immunogenicity (38).
Unlike some other solid tumors such as melanoma and lung cancer with high TMB (>10 mutations/Mb), PDAC tumors exhibit a low median TMB (2.7 mutations/Mb), which may explain the ineffectiveness of ICP inhibitors (39). Impairment of mismatch repair (MMR) leads to an increase in the number of mutations and neoantigens conferring microsatellite instability status. As observed with pembrolizumab, MSI-high (MSI-H) tumors are more likely to respond to ICP inhibitor therapy. However, about 1% of PDAC tumors harbor MSI-status, suggesting that only a small number of PDAC patients may benefit from pembrolizumab (40, 41).
Taken together, these data clearly demonstrate that many immunological parameters interfere with the clinical activity of immunotherapy in PDAC. The current challenge is therefore to overcome the barriers of this “cold” TME tumor microenvironment and to use certain strategies improving tumor immunogenicity in order to convert them into “hot” tumors. In this review, we discuss the use of the chemo-immunotherapy combination to sensitize pancreatic tumors to immune therapy and modalities to take into account in designing efficient combinatorial approaches.
3 Immune Checkpoint-Based Chemoimmunotherapy Combination
3.1 Rationale of the Combinatorial Strategy
As observed in previous clinical studies, the targeting the TME using single-agent ICP inhibitors is not enough for PDAC patients. Although conventional chemotherapies have immunosuppressive effects as seen with lymphopenia and neutropenia in treated patients, these chemotherapeutic agents also have immunostimulatory properties that can be exploited to improve the survival of PDAC patients.
For example, an in vivo study has demonstrated that 5-FU drug depletes immunosuppressive cells (MDSC, Treg) while increasing IFN-γ production by tumor-infiltrating CD8 T-cells and is associated with antitumor immune responses (42). It appears that many chemotherapeutic agents could bypass the permissive TME in favor of antitumor immunity by using different mechanisms such as the depletion of immunosuppressive populations combined with a recruitment of cytotoxic T-cells within the TME, an induction of DC maturation (43) with an increased antigen presentation ability, as well as an upregulation of MHC-I (44) and PD-L1 expression on tumors cells (45). the immunological effects of chemotherapeutic drugs used in the clinical management of PDAC tumors are summarized in the Table 1. By promoting the generation of neoantigens, chemotherapy enables T-cell recruitment and priming in addition to cell depletion of immunosuppressive cells while ICP inhibitor stimulates exhausted T cells (Figure 1). This synergistic effect has been demonstrated in several preclinical (122) and clinical (123, 124) studies where the combination lengthened the median OS with an acceptable safety profile (123).
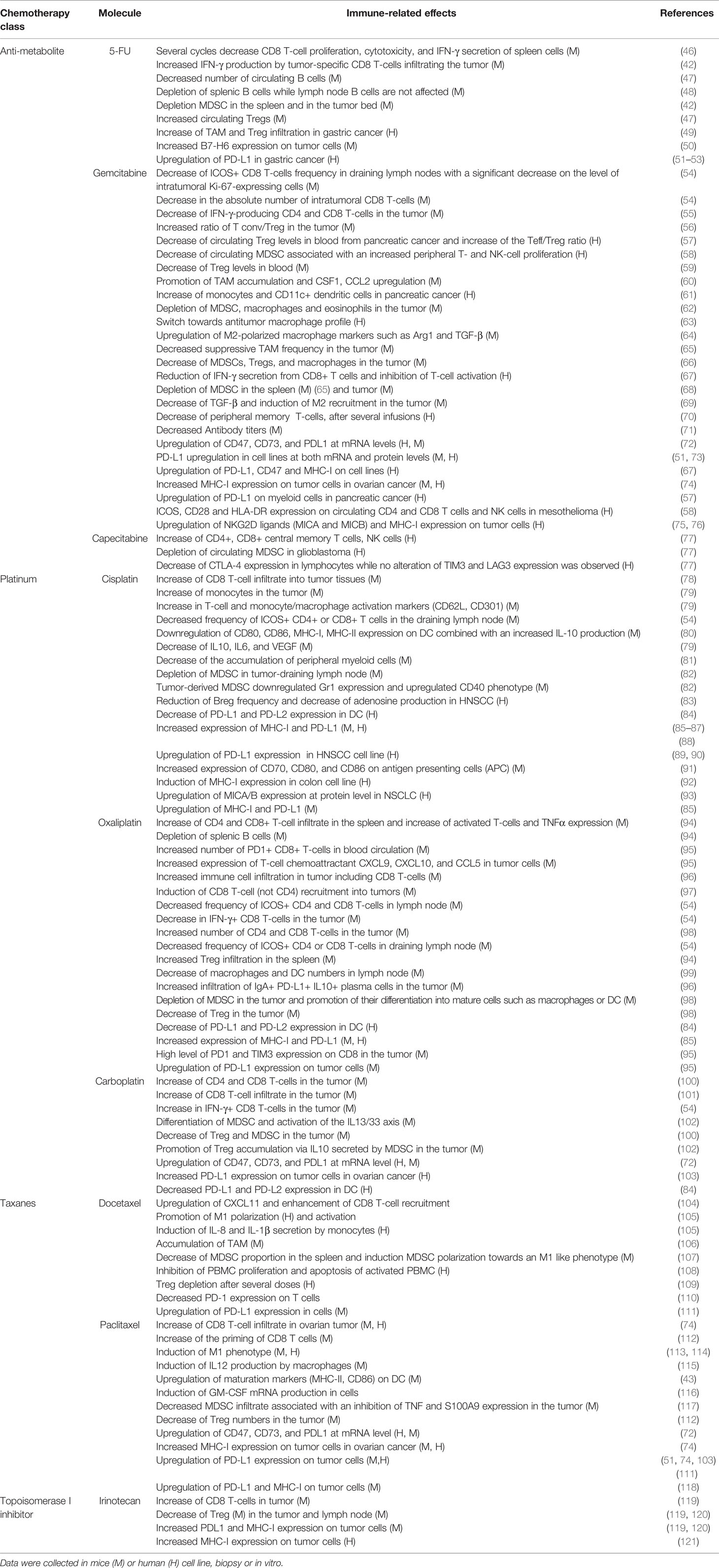
Table 1 Immunomodulatory effects of chemotherapeutic agents used for the treatment of pancreatic ductal adenocarcinoma.
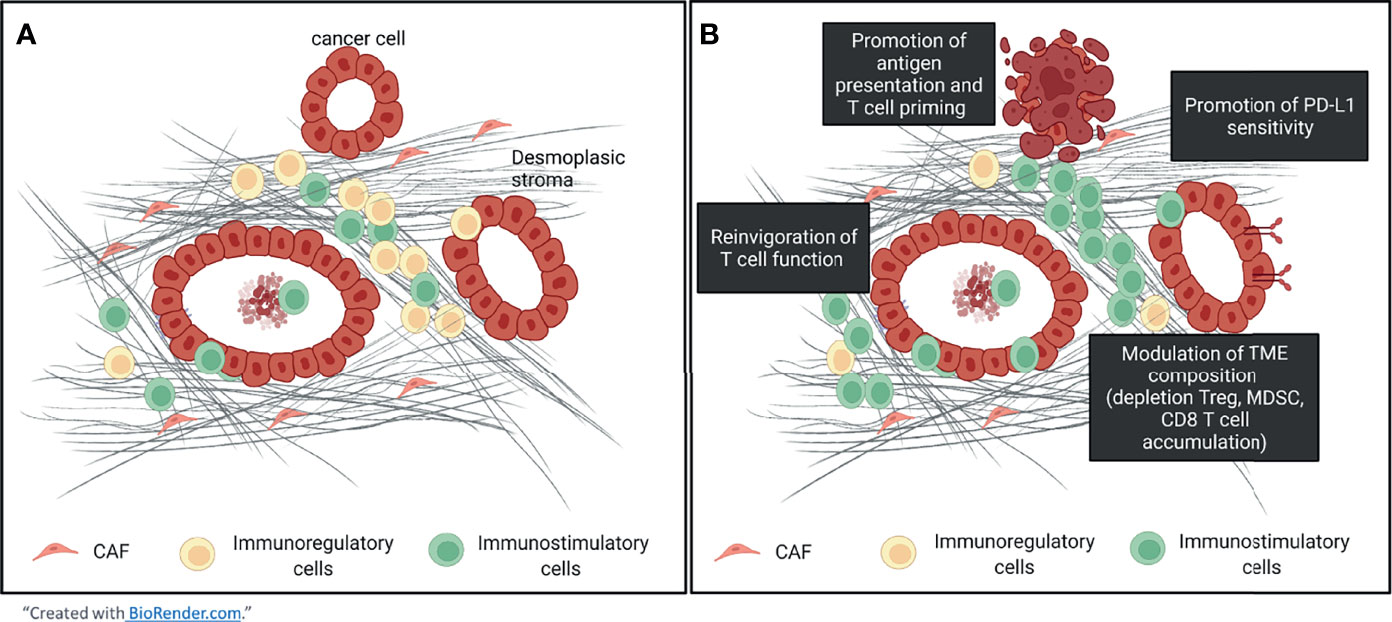
Figure 1 The tumor microenvironment of pancreatic ductal adenocarcinoma and impact of the chemo-immunotherapy. (A) The tumor microenvironment of the pancreas is highly infiltrated by immunosuppressive cells (MDSC, Treg, M2 macrophages). (B) Chemotherapy promotes T cells recruitment and their priming which could counterbalance the ratio immunosuppressive / effector cells within the TME while ICP inhibitor reinvigorates exhausted T cells. Moreover, an upregulation of PD-L1 expression has been observed and may increase the sensitivity to PD-(L)1 inhibitors.
However, this clinical benefit is not obvious, as demonstrated by the negative results of the phase 1b trial (NCT01473940) combining gemcitabine + ipilimumab in late-stage PDAC patients (125). These clinical observations suggest that some parameters (chemotherapeutic agents, dose regimen, schedule of administration) are not optimal yet and should be assessed to design efficient combinatorial strategies.
3.2 Modalities for This Combinatorial Strategy
3.2.1 Chemotherapeutic Agents
The immunological effects of some chemotherapeutic agents could be deleterious in some settings. For example, in PDAC, gemcitabine promotes the accumulation of macrophages and their polarization toward the pro-tumor M2 phenotype (64, 69). In response to some chemotherapeutic agents, TAM (126) and CAF (127) secrete VEGF-A, VEGF-C and other pro-angiogenic factors (128). In mice, gemcitabine increases the synthesis of some chemokines and TGF-β signals leading to gemcitabine resistance (129). However, this drug is currently part of the standard of care, suggesting that these pro-tumor effects must be counterbalanced by the immunostimulatory effects of the drug. Other examples of the controversial effects of chemotherapeutic agents are reported in Table 1 with the accumulation of regulatory and immunosuppressive cells after treatment in mice and in humans. For example, oxaliplatin or carboplatin favors Treg or MDSC infiltration which is related to chemoresistance (102).
These findings suggest that a better understanding of these immune-mediated effects of chemotherapy is required to find the most promising combinatorial strategies. Moreover, other parameters such as the dose regimen, schedule of administration, drug treatment schedule, and tolerability profile are not clearly understood yet and are also crucial to achieving clinical success.
3.2.2 Dose Regimen: Standard or Metronomic?
Conventional chemotherapeutic agents are commonly used at the maximum tolerated dose (MTD) which represents the highest dose of the drug acceptable for the patient in terms of toxicity. By their mechanism of action, these treatments exert strong cytotoxicity on proliferating hematopoietic cells, including immune cells, resulting in profound myelosuppression and a risk of infections. These toxicities require administration interspersed with drug-free periods to restore hematopoiesis, periods during which chemoresistant clones may emerge. In contrast, metronomic doses of chemotherapy, i.e., administration of chemotherapeutic agents at low dose but frequently, could bypass deleterious effects (130) of conventional chemotherapy with higher efficacy to control the disease (131).
The antitumor effects of the metronomic vs. standard dose of chemotherapy were assessed in several PDAC mouse models especially for cyclophosphamide and gemcitabine agents (132–134). The studies showed that the reduction in tumor growth was equivalent in both regimens (132, 133), but a metronomic administration of gemcitabine induces anti-angiogenic effects as observed by the induction of thrombospodin-1 (TSP-1), an angiogenic inhibitor factor (132). Similarly, Cham et al. demonstrated the decrease of pro-angiogenic factors such as such EGF, IL-1α, IL-8, ICAM-1, and VCAM-1 in the tumor after a metronomic dose of gemcitabine as well as decreased hypoxia (133, 134). In addition to this anti-angiogenic activity, a low dose of gemcitabine could also impact the immune cell content. In an orthotopic model of PDAC, low-dose gemcitabine depletes Treg, thus inducing a concomitant increase of conventional T-cell percentages but have no impact on the frequency of MDSC (56).
A metronomic dose of chemotherapy is also effective for PDAC patients while being less toxic. Indeed, the combination of a low dose of nab-paclitaxel (60 mg/m²) + oxaliplatin (50 mg/m²) plus a continuous infusion of 5-FU and bevacizumab (anti-VEGF) was effective with an ORR of 49% and a disease control rate (DCR) of 81%. Surprisingly, 82% of patients were still alive beyond 1 year (135).
This beneficial effect at a metronomic dose might be mediated by depletion of immunosuppressive populations such as Treg (136, 137), promotion of DC maturation, enhancement of T-cell-mediated antitumor immunity (138) and/or anti-angiogenic properties as initially described with cyclophosphamide (139, 140). However, although there is a certain amount of preclinical evidence demonstrating the positive impact of low-dose chemotherapy on the TME, this benefit is not well documented in clinic. Indeed, few ongoing clinical trials ongoing clinical trials in combination with an ICP inhibitor use this dose regimen (Table 2).
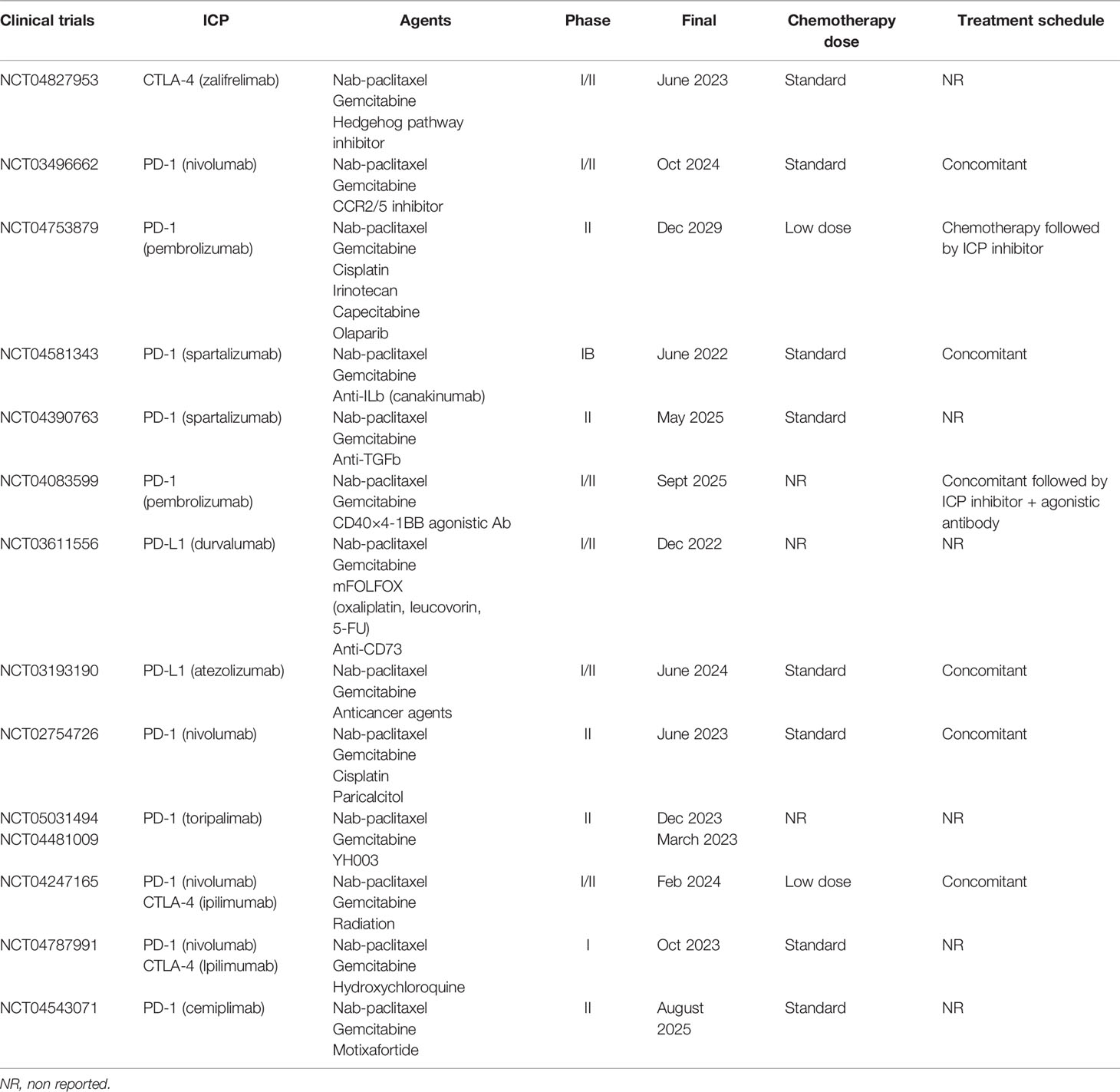
Table 2 Ongoing clinical trials in PDAC using chemotherapy in combination with immune checkpoint with associated doses and schedules.
3.2.3 The Administration Schedule : Concomitant or Sequenced Regimen?
To date, several clinical trials investigating the efficacy and safety of concomitant chemoimmunotherapy are ongoing. However, according to the scientific rationale, the best treatment sequence would be the administration of chemotherapy first, which sensitizes the TME by releasing neoantigens and promoting T-cell priming, followed by ICP inhibitor therapy which may sustain T-cell-mediated antitumor activity.
However, in a preclinical pancreatic model, the concomitant administration of chemotherapy and PD-L1 blockade results in complete responses compared to the sequenced administration of chemotherapy followed by an anti-PD-L1 therapy (15). The same result was reported in a mesothelioma mouse model where the synergistic effect of the combination was only observed when both drugs were administered simultaneously (141). Conversely, in a phase 2 study, the efficacy of sequential administration versus concomitant administration of chemoimmunotherapy combination has been tested for the treatment of metastatic melanoma. In this case, the sequential use of ipilimumab followed by chemotherapy confers a PFS benefit (142). This effect was not seen in PDAC, where sequential administration of chemotherapy and immunotherapy is not associated with an improved OS (143). As with current clinical guidelines, most clinical trials ongoing in PDAC (Table 2) deliver chemotherapy concomitantly with immunotherapy, but data are lacking to support the current treatment schedule suggesting a window for improvement. These findings demonstrate that a scheduled regimen may require to be adapted according to the tumor type and therapeutic agents.
4 Conclusion
Pancreatic ductal adenocarcinoma is one of the most aggressive and deadly cancers. With the lowest 5-year survival rate among solid tumors, the medical need is high and requires a great deal of effort from researchers to find therapeutic strategies that are more effective than current chemotherapies. Unfortunately, despite the arrival of breakthrough ICP inhibitor therapy, survival for PDAC patients has not improved significantly. The study of the microenvironment has highlighted that low immunogenicity of PDAC tumors limits the effectiveness of current treatments. One strategy to circumvent these barriers is the use of chemotherapy to sensitize this permissive microenvironment in combination with ICP inhibitors. The efficacy of this combinatorial strategy has been reported in multiple tumor types including PDAC. However, the observed clinical benefit of these combinations is not universal and seems to be dependent on several parameters. In this review, we have shown that some chemotherapeutic agents have pro- and antitumor effects. However, the molecular characteristics of the modulations induced by these treatments are not sufficiently established and could be informative to designing more efficient combination strategies. These immunological effects can be modulated by the type, the dose regimen and the administration schedule. Accumulating evidence has demonstrated equivalent antitumor effects between low-dose chemotherapy and standard dose chemotherapy; these studies reported additional activities of low-dose chemotherapy such as inhibiting hypoxia and reducing angiogenesis. Despite this rationale, few combinations under investigation in clinical trials use. This dose regimen which could improve combination tolerability. Regarding the administration schedule regimen, to date, many clinical trials are testing the efficacy of chemotherapy administered concomitantly with ICP inhibitor therapy, mainly at MTD levels. Preclinical and clinical data obtained in studying the impact of this parameter on antitumor response are quite confusing. This administration schedule is likely to depend on the dose as well as the therapeutic agent chosen. Currently, data obtained in studying the combination of these parameters are lacking. Future research should therefore explore the impact of these treatment modalities on preclinical models and subsequently in clinical trials to guide the development of appropriate synergistic combinations.
Finally, the heterogeneity of PDAC patients is also a crucial parameter to consider. Some of PDAC subtypes are more immunogenic with a greater chance to response to ICP inhibitor therapy, while others are an immune desert. As 80% of PDAC tumors are unresectable at diagnosis, which hinders knowledge of the disease, the development of omic? Technologies will help leverage and collect as much biomarker data as possible from tumor samples in the clinic to gain a deeper understanding of the TME and monitor pharmacodynamic biomarkers to optimize combination parameters.
Author Contributions
The author confirms being the sole contributor of this work and has approved it for publication.
Conflict of Interest
Author HK was employed by Servier.
Publisher’s Note
All claims expressed in this article are solely those of the authors and do not necessarily represent those of their affiliated organizations, or those of the publisher, the editors and the reviewers. Any product that may be evaluated in this article, or claim that may be made by its manufacturer, is not guaranteed or endorsed by the publisher.
Abbreviations
CAF, cancer-associated fibroblast; DAMPS, damage-associated molecular pattern; DC, dendritic cells; DCR, durable clinical response; ECM, extracellular matrix; HIF-a, hypoxia-inducible factor 1; ICP, immune checkpoint; MDSC, myeloid-derived suppressor cells; MHC, major histocompatibility complex; MMR, mismatch repair; MTD, maximum tolerated dose; NR, not reported; ORR, overall response rate; OS, overall survival; PanIN, pancreatic intraepithelial neoplasia; PDAC, pancreatic ductal adenocarcinoma; PFS, progression-free survival; PRR, pattern recognition receptors; PSC, pancreatic stellate cells; TAM, tumor-associated macrophages; TLR, toll-like receptors; TLS, tertiary lymphoid structure; TMB, tumor mutational burden; TME, tumor microenvironment; Treg, regulatory T cells; TSP-1 thrombospondin-1; US, United States.
References
1. Ushio J, Kanno A, Ikeda E, Ando K, Nagai H, Miwata T, et al. Pancreatic Ductal Adenocarcinoma: Epidemiology and Risk Factors. Diagnostics (2021) 11:562. doi: 10.3390/diagnostics11030562
2. Survival Rates for Pancreatic Cancer. Available at: https://www.cancer.org/cancer/pancreatic-cancer/detection-diagnosis-staging/survival-rates.html.
3. Orth M, Metzger P, Gerum S, Mayerle J, Schneider G, Belka C, et al. Pancreatic Ductal Adenocarcinoma: Biological Hallmarks, Current Status, and Future Perspectives of Combined Modality Treatment Approaches. Radiat Oncol (2019) 14:141. doi: 10.1186/s13014-019-1345-6
4. Rahib L, Smith BD, Aizenberg R, Rosenzweig AB, Fleshman JM, Matrisian LM. Projecting Cancer Incidence and Deaths to 2030: The Unexpected Burden of Thyroid, Liver, and Pancreas Cancers in the United States. Cancer Res (2014) 74:2913–21. doi: 10.1158/0008-5472.CAN-14-0155
5. Conroy T, Desseigne F, Ychou M, Bouché O, Guimbaud R, Bécouarn Y, et al. FOLFIRINOX Versus Gemcitabine for Metastatic Pancreatic Cancer. N Engl J Med (2011) 364:1817–25. doi: 10.1056/NEJMoa1011923
6. Jose L-M, Wee MW, Christos K, Peter B, Hagan K, Michael S, et al. Nab-Paclitaxel Plus Gemcitabine Vs Gemcitabine Alone for Patients With Metastatic Pancreatic Cancer: Influence of Primary Pancreatic Tumor Location on Efficacy and Treatment Exposure in the Mpact Phase III Trial. Ann Oncol (2014) 25:ii21. doi: 10.1093/annonc/mdu165.26
7. Haslam A, Prasad V. Estimation of the Percentage of US Patients With Cancer Who Are Eligible for and Respond to Checkpoint Inhibitor Immunotherapy Drugs. JAMA Netw Open (2019) 2:e192535. doi: 10.1001/jamanetworkopen.2019.2535
8. Doroshow DB, Bhalla S, Beasley MB, Sholl LM, Kerr KM, Gnjatic S, et al. PD-L1 as a Biomarker of Response to Immune-Checkpoint Inhibitors. Nat Rev Clin Oncol (2021) 18:345–62. doi: 10.1038/s41571-021-00473-5
9. Zhao P, Li L, Jiang X, Li Q. Mismatch Repair Deficiency/Microsatellite Instability-High as a Predictor for Anti-PD-1/PD-L1 Immunotherapy Efficacy. J Hematol OncolJ Hematol Oncol (2019) 12:54. doi: 10.1186/s13045-019-0738-1
10. Tewari N, Zaitoun AM, Arora A, Madhusudan S, Ilyas M, Lobo DN. The Presence of Tumour-Associated Lymphocytes Confers a Good Prognosis in Pancreatic Ductal Adenocarcinoma: An Immunohistochemical Study of Tissue Microarrays. BMC Cancer (2013) 13:436. doi: 10.1186/1471-2407-13-436
11. Ino Y, Yamazaki-Itoh R, Shimada K, Iwasaki M, Kosuge T, Kanai Y, et al. Immune Cell Infiltration as an Indicator of the Immune Microenvironment of Pancreatic Cancer. Br J Cancer (2013) 108:914–23. doi: 10.1038/bjc.2013.32
12. Fukunaga A, Miyamoto M, Cho Y, Murakami S, Kawarada Y, Oshikiri T, et al. CD8+ Tumor-Infiltrating Lymphocytes Together With CD4+ Tumor-Infiltrating Lymphocytes and Dendritic Cells Improve the Prognosis of Patients With Pancreatic Adenocarcinoma. Pancreas (2004) 28:e26–31. doi: 10.1097/00006676-200401000-00023
13. Blando J, Sharma A, Higa MG, Zhao H, Vence L, Yadav SS, et al. Comparison of Immune Infiltrates in Melanoma and Pancreatic Cancer Highlights VISTA as a Potential Target in Pancreatic Cancer. Proc Natl Acad Sci (2019) 116:1692–7. doi: 10.1073/pnas.1811067116
14. Farren MR, Mace TA, Geyer S, Mikhail S, Wu C, Ciombor K, et al. Systemic Immune Activity Predicts Overall Survival in Treatment-Naïve Patients With Metastatic Pancreatic Cancer. Clin Cancer Res (2016) 22:2565–74. doi: 10.1158/1078-0432.CCR-15-1732
15. Nomi T, Sho M, Akahori T, Hamada K, Kubo A, Kanehiro H, et al. Clinical Significance and Therapeutic Potential of the Programmed Death-1 Ligand/Programmed Death-1 Pathway in Human Pancreatic Cancer. Clin Cancer Res (2007) 13:2151–7. doi: 10.1158/1078-0432.CCR-06-2746
16. Sautès-Fridman C, Petitprez F, Calderaro J, Fridman WH. Tertiary Lymphoid Structures in the Era of Cancer Immunotherapy. Nat Rev Cancer (2019) 19:307–25. doi: 10.1038/s41568-019-0144-6
17. Hiraoka N, Ino Y, Yamazaki-Itoh R, Kanai Y, Kosuge T, Shimada K. Intratumoral Tertiary Lymphoid Organ Is a Favourable Prognosticator in Patients With Pancreatic Cancer. Br J Cancer (2015) 112:1782–90. doi: 10.1038/bjc.2015.145
18. Gunderson A J, Rajamanickam V, Bui C, Bernard B, Pucilowska J, Ballesteros-Merino C, et al. Germinal Center Reactions in Tertiary Lymphoid Structures Associate With Neoantigen Burden, Humoral Immunity and Long-Term Survivorship in Pancreatic Cancer. OncoImmunology (2021) 10:1900635. doi: 10.1080/2162402X.2021.1900635
19. Vanhersecke L, Brunet M, Guégan J-P, Rey C, Bougouin A, Cousin S, et al. Mature Tertiary Lymphoid Structures Predict Immune Checkpoint Inhibitor Efficacy in Solid Tumors Independently of PD-L1 Expression. Nat Cancer (2021) 2:794–802. doi: 10.1038/s43018-021-00232-6
20. Helmink BA, Reddy SM, Gao J, Zhang S, Basar R, Thakur R, et al. B Cells and Tertiary Lymphoid Structures Promote Immunotherapy Response. Nature (2020) 577:549–55. doi: 10.1038/s41586-019-1922-8
21. Petitprez F, de Reyniès A, Keung EZ, Chen TW-W, Sun C-M, Calderaro J, et al. B Cells Are Associated With Survival and Immunotherapy Response in Sarcoma. Nature (2020) 577:556–60. doi: 10.1038/s41586-019-1906-8
22. Royal RE, Levy C, Turner K, Mathur A, Hughes M, Kammula US, et al. Phase 2 Trial of Single Agent Ipilimumab (Anti-CTLA-4) for Locally Advanced or Metastatic Pancreatic Adenocarcinoma. J Immunother (2010) 33:828–33. doi: 10.1097/CJI.0b013e3181eec14c
23. O’Reilly EM, Oh D-Y, Dhani N, Renouf DJ, Lee MA, Sun W, et al. Durvalumab With or Without Tremelimumab for Patients With Metastatic Pancreatic Ductal Adenocarcinoma: A Phase 2 Randomized Clinical Trial. JAMA Oncol (2019) 5:1431. doi: 10.1001/jamaoncol.2019.1588
24. Clark CE, Hingorani SR, Mick R, Combs C, Tuveson DA, Vonderheide RH. Dynamics of the Immune Reaction to Pancreatic Cancer From Inception to Invasion. Cancer Res (2007) 67:9518–27. doi: 10.1158/0008-5472.CAN-07-0175
25. Monti P, Leone BE, Marchesi F, Balzano G, Zerbi A, Scaltrini F, et al. The CC Chemokine MCP-1/CCL2 in Pancreatic Cancer Progression: Regulation of Expression and Potential Mechanisms of Antimalignant Activity. Cancer Res (2003) 63:7451–61.
26. Zhang A, Qian Y, Ye Z, Chen H, Xie H, Zhou L, et al. Cancer-Associated Fibroblasts Promote M2 Polarization of Macrophages in Pancreatic Ductal Adenocarcinoma. Cancer Med (2017) 6:463–70. doi: 10.1002/cam4.993
27. Xiong C, Zhu Y, Xue M, Jiang Y, Zhong Y, Jiang L, et al. Tumor-Associated Macrophages Promote Pancreatic Ductal Adenocarcinoma Progression by Inducing Epithelial-to-Mesenchymal Transition. Aging (2021) 13:3386–404. doi: 10.18632/aging.202264
28. Yu M, Guan R, Hong W, Zhou Y, Lin Y, Jin H, et al. Prognostic Value of Tumor-Associated Macrophages in Pancreatic Cancer: A Meta-Analysis. Cancer Manag Res (2019) 11:4041–58. doi: 10.2147/CMAR.S196951
29. Peranzoni E, Lemoine J, Vimeux L, Feuillet V, Barrin S, Kantari-Mimoun C, et al. Macrophages Impede CD8 T Cells From Reaching Tumor Cells and Limit the Efficacy of Anti–PD-1 Treatment. Proc Natl Acad Sci (2018) 115:E4041–50. doi: 10.1073/pnas.1720948115
30. Zhu Y, Herndon JM, Sojka DK, Kim K-W, Knolhoff BL, Zuo C, et al. Tissue-Resident Macrophages in Pancreatic Ductal Adenocarcinoma Originate From Embryonic Hematopoiesis and Promote Tumor Progression. Immunity (2017) 47:323–38.e6. doi: 10.1016/j.immuni.2017.07.014
31. Beatty GL, Winograd R, Evans RA, Long KB, Luque SL, Lee JW, et al. Exclusion of T Cells From Pancreatic Carcinomas in Mice Is Regulated by Ly6Clow F4/80+ Extratumoral Macrophages. Gastroenterology (2015) 149:201–10. doi: 10.1053/j.gastro.2015.04.010
32. Borgoni S, Iannello A, Cutrupi S, Allavena P, D’Incalci M, Novelli F, et al. Depletion of Tumor-Associated Macrophages Switches the Epigenetic Profile of Pancreatic Cancer Infiltrating T Cells and Restores Their Anti-Tumor Phenotype. OncoImmunology (2018) 7:e1393596. doi: 10.1080/2162402X.2017.1393596
33. Zhang Y, Lazarus J, Steele NG, Yan W, Lee H-J, Nwosu ZC, et al. Regulatory T-Cell Depletion Alters the Tumor Microenvironment and Accelerates Pancreatic Carcinogenesis. Cancer Discov (2020) 10:422–39. doi: 10.1158/2159-8290.CD-19-0958
34. Salmon H, Franciszkiewicz K, Damotte D, Dieu-Nosjean M-C, Validire P, Trautmann A, et al. Matrix Architecture Defines the Preferential Localization and Migration of T Cells Into the Stroma of Human Lung Tumors. J Clin Invest (2012) 122:899–910. doi: 10.1172/JCI45817
35. Carstens JL, Correa de Sampaio P, Yang D, Barua S, Wang H, Rao A, et al. Spatial Computation of Intratumoral T Cells Correlates With Survival of Patients With Pancreatic Cancer. Nat Commun (2017) 8:15095. doi: 10.1038/ncomms15095
36. Özdemir BC, Pentcheva-Hoang T, Carstens JL, Zheng X, Wu C-C, Simpson TR, et al. Depletion of Carcinoma-Associated Fibroblasts and Fibrosis Induces Immunosuppression and Accelerates Pancreas Cancer With Reduced Survival. Cancer Cell (2014) 25:719–34. doi: 10.1016/j.ccr.2014.04.005
37. McGrail DJ, Pilié PG, Rashid NU, Voorwerk L, Slagter M, Kok M, et al. High Tumor Mutation Burden Fails to Predict Immune Checkpoint Blockade Response Across All Cancer Types. Ann Oncol (2021) 32:661–72. doi: 10.1016/j.annonc.2021.02.006
38. Australian Pancreatic Cancer Genome Initiative, Balachandran VP, Łuksza M, Zhao JN, Makarov V, Moral JA, et al. Identification of Unique Neoantigen Qualities in Long-Term Survivors of Pancreatic Cancer. Nature (2017) 551:512–6. doi: 10.1038/nature24462
39. Goodman AM, Kato S, Bazhenova L, Patel SP, Frampton GM, Miller V, et al. Tumor Mutational Burden as an Independent Predictor of Response to Immunotherapy in Diverse Cancers. Mol Cancer Ther (2017) 16:2598–608. doi: 10.1158/1535-7163.MCT-17-0386
40. Hu ZI, Shia J, Stadler ZK, Varghese AM, Capanu M, Salo-Mullen E, et al. Evaluating Mismatch Repair Deficiency in Pancreatic Adenocarcinoma: Challenges and Recommendations. Clin Cancer Res (2018) 24:1326–36. doi: 10.1158/1078-0432.CCR-17-3099
41. Luchini C, Brosens LAA, Wood LD, Chatterjee D, Shin JI, Sciammarella C, et al. Comprehensive Characterisation of Pancreatic Ductal Adenocarcinoma With Microsatellite Instability: Histology, Molecular Pathology and Clinical Implications. Gut (2021) 70:148–56. doi: 10.1136/gutjnl-2020-320726
42. Vincent J, Mignot G, Chalmin F, Ladoire S, Bruchard M, Chevriaux A, et al. 5-Fluorouracil Selectively Kills Tumor-Associated Myeloid-Derived Suppressor Cells Resulting in Enhanced T Cell–Dependent Antitumor Immunity. Cancer Res (2010) 70:3052–61. doi: 10.1158/0008-5472.CAN-09-3690
43. Pfannenstiel LW, Lam SSK, Emens LA, Jaffee EM, Armstrong TD. Paclitaxel Enhances Early Dendritic Cell Maturation and Function Through TLR4 Signaling in Mice. Cell Immunol (2010) 263:79–87. doi: 10.1016/j.cellimm.2010.03.001
44. Wan S, Pestka S, Jubin RG, Lyu YL, Tsai Y-C, Liu LF. Chemotherapeutics and Radiation Stimulate MHC Class I Expression Through Elevated Interferon-Beta Signaling in Breast Cancer Cells. PloS One (2012) 7:e32542. doi: 10.1371/journal.pone.0032542
45. Shin J, Chung J-H, Kim SH, Lee KS, Suh KJ, Lee JY, et al. Effect of Platinum-Based Chemotherapy on PD-L1 Expression on Tumor Cells in Non-Small Cell Lung Cancer. Cancer Res Treat (2019) 51:1086–97. doi: 10.4143/crt.2018.537
46. Wu Y, Deng Z, Wang H, Ma W, Zhou C, Zhang S. Repeated Cycles of 5-Fluorouracil Chemotherapy Impaired Anti-Tumor Functions of Cytotoxic T Cells in a CT26 Tumor-Bearing Mouse Model. BMC Immunol (2016) 17:29. doi: 10.1186/s12865-016-0167-7
47. Orecchioni S, Talarico G, Labanca V, Calleri A, Mancuso P, Bertolini F. Vinorelbine, Cyclophosphamide and 5-FU Effects on the Circulating and Intratumoural Landscape of Immune Cells Improve Anti-PD-L1 Efficacy in Preclinical Models of Breast Cancer and Lymphoma. Br J Cancer (2018) 118:1329–36. doi: 10.1038/s41416-018-0076-z
48. Vetvicka V, Kincade PW, Witte PL. Effects of 5-Fluorouracil on B Lymphocyte Lineage Cells. J Immunol Baltim Md 1950 (1986) 137:2405–10.
49. Wang B, Xu D, Yu X, Ding T, Rao H, Zhan Y, et al. Association of Intra-Tumoral Infiltrating Macrophages and Regulatory T Cells Is an Independent Prognostic Factor in Gastric Cancer After Radical Resection. Ann Surg Oncol (2011) 18:2585–93. doi: 10.1245/s10434-011-1609-3
50. Cao G, Wang J, Zheng X, Wei H, Tian Z, Sun R. Tumor Therapeutics Work as Stress Inducers to Enhance Tumor Sensitivity to Natural Killer (NK) Cell Cytolysis by Up-Regulating NKp30 Ligand B7-H6. J Biol Chem (2015) 290:29964–73. doi: 10.1074/jbc.M115.674010
51. Doi T, Ishikawa T, Okayama T, Oka K, Mizushima K, Yasuda T, et al. The JAK/STAT Pathway Is Involved in the Upregulation of PD-L1 Expression in Pancreatic Cancer Cell Lines. Oncol Rep (2017) 37:1545–54. doi: 10.3892/or.2017.5399
52. Van Der Kraak L, Goel G, Ramanan K, Kaltenmeier C, Zhang L, Normolle DP, et al. 5-Fluorouracil Upregulates Cell Surface B7-H1 (PD-L1) Expression in Gastrointestinal Cancers. J Immunother Cancer (2016) 4:65. doi: 10.1186/s40425-016-0163-8
53. Zhang M, Fan Y, Che X, Hou K, Zhang C, Li C, et al. 5-FU-Induced Upregulation of Exosomal PD-L1 Causes Immunosuppression in Advanced Gastric Cancer Patients. Front Oncol (2020) 10:492. doi: 10.3389/fonc.2020.00492
54. Cubas R, Moskalenko M, Cheung J, Yang M, McNamara E, Xiong H, et al. Chemotherapy Combines Effectively With Anti–PD-L1 Treatment and Can Augment Antitumor Responses. J Immunol (2018) 201:2273–86. doi: 10.4049/jimmunol.1800275
55. Wu C, Tan X, Hu X, Zhou M, Yan J, Ding C. Tumor Microenvironment Following Gemcitabine Treatment Favors Differentiation of Immunosuppressive Ly6C High Myeloid Cells. J Immunol (2020) 204:212–23. doi: 10.4049/jimmunol.1900930
56. Shevchenko I, Karakhanova S, Soltek S, Link J, Bayry J, Werner J, et al. Low-Dose Gemcitabine Depletes Regulatory T Cells and Improves Survival in the Orthotopic Panc02 Model of Pancreatic Cancer: Regulatory T Cells in Pancreatic Cancer. Int J Cancer (2013) 133:98–107. doi: 10.1002/ijc.27990
57. Eriksson E, Wenthe J, Irenaeus S, Loskog A, Ullenhag G. Gemcitabine Reduces MDSCs, Tregs and Tgfβ-1 While Restoring the Teff/Treg Ratio in Patients With Pancreatic Cancer. J Transl Med (2016) 14:282. doi: 10.1186/s12967-016-1037-z
58. Dammeijer F, De Gooijer CJ, van Gulijk M, Lukkes M, Klaase L, Lievense LA, et al. Immune Monitoring in Mesothelioma Patients Identifies Novel Immune-Modulatory Functions of Gemcitabine Associating With Clinical Response. EBioMedicine (2021) 64:103160. doi: 10.1016/j.ebiom.2020.103160
59. Rettig L, Seidenberg S, Parvanova I, Samaras P, Knuth A, Pascolo S. Gemcitabine Depletes Regulatory T-Cells in Human and Mice and Enhances Triggering of Vaccine-Specific Cytotoxic T-Cells. Int J Cancer (2011) 129:832–8. doi: 10.1002/ijc.25756
60. Mitchem JB, Brennan DJ, Knolhoff BL, Belt BA, Zhu Y, Sanford DE, et al. Targeting Tumor-Infiltrating Macrophages Decreases Tumor-Initiating Cells, Relieves Immunosuppression, and Improves Chemotherapeutic Responses. Cancer Res (2013) 73:1128–41. doi: 10.1158/0008-5472.CAN-12-2731
61. Soeda A, Morita-Hoshi Y, Makiyama H, Morizane C, Ueno H, Ikeda M, et al. Regular Dose of Gemcitabine Induces an Increase in CD14+ Monocytes and CD11c+ Dendritic Cells in Patients With Advanced Pancreatic Cancer. Jpn J Clin Oncol (2009) 39:797–806. doi: 10.1093/jjco/hyp112
62. Bosiljcic M, Cederberg RA, Hamilton MJ, LePard NE, Harbourne BT, Collier JL, et al. Targeting Myeloid-Derived Suppressor Cells in Combination With Primary Mammary Tumor Resection Reduces Metastatic Growth in the Lungs. Breast Cancer Res (2019) 21:103. doi: 10.1186/s13058-019-1189-x
63. Di Caro G, Cortese N, Castino GF, Grizzi F, Gavazzi F, Ridolfi C, et al. Dual Prognostic Significance of Tumour-Associated Macrophages in Human Pancreatic Adenocarcinoma Treated or Untreated With Chemotherapy. Gut (2016) 65:1710–20. doi: 10.1136/gutjnl-2015-309193
64. Deshmukh SK, Tyagi N, Khan MA, Srivastava SK, Al-Ghadhban A, Dugger K, et al. Gemcitabine Treatment Promotes Immunosuppressive Microenvironment in Pancreatic Tumors by Supporting the Infiltration, Growth, and Polarization of Macrophages. Sci Rep (2018) 8:12000. doi: 10.1038/s41598-018-30437-2
65. Suzuki E, Kapoor V, Jassar AS, Kaiser LR, Albelda SM. Gemcitabine Selectively Eliminates Splenic Gr-1 + /CD11b + Myeloid Suppressor Cells in Tumor-Bearing Animals and Enhances Antitumor Immune Activity. Clin Cancer Res (2005) 11:6713–21. doi: 10.1158/1078-0432.CCR-05-0883
66. Chang L-S, Yan W-L, Chang Y-W, Yeh Y-C, Chen H-W, Leng C-H, et al. Gemcitabine Enhances Antitumor Efficacy of Recombinant Lipoimmunogen-Based Immunotherapy. OncoImmunology (2016) 5:e1095433. doi: 10.1080/2162402X.2015.1095433
67. Smith PL, Yogaratnam Y, Samad M, Kasow S, Dalgleish AG. Effect of Gemcitabine Based Chemotherapy on the Immunogenicity of Pancreatic Tumour Cells and T-Cells. Clin Transl Oncol (2021) 23:110–21. doi: 10.1007/s12094-020-02429-0
68. Le HK, Graham L, Cha E, Morales JK, Manjili MH, Bear HD. Gemcitabine Directly Inhibits Myeloid Derived Suppressor Cells in BALB/c Mice Bearing 4T1 Mammary Carcinoma and Augments Expansion of T Cells From Tumor-Bearing Mice. Int Immunopharmacol (2009) 9:900–9. doi: 10.1016/j.intimp.2009.03.015
69. Bulle A, Dekervel J, Deschuttere L, Nittner D, Libbrecht L, Janky R, et al. Gemcitabine Recruits M2-Type Tumor-Associated Macrophages Into the Stroma of Pancreatic Cancer. Transl Oncol (2020) 13:100743. doi: 10.1016/j.tranon.2020.01.004
70. Plate JMD, Plate AE, Shott S, Bograd S, Harris JE. Effect of Gemcitabine on Immune Cells in Subjects With Adenocarcinoma of the Pancreas. Cancer Immunol Immunother (2005) 54:915–25. doi: 10.1007/s00262-004-0638-1
71. Nowak AK, Robinson BWS, Lake RA. Gemcitabine Exerts a Selective Effect on the Humoral Immune Response: Implications for Combination Chemo-Immunotherapy. Cancer Res (2002) 62:2353–8.
72. Samanta D, Park Y, Ni X, Li H, Zahnow CA, Gabrielson E, et al. Chemotherapy Induces Enrichment of CD47 + /CD73 + /PDL1 + Immune Evasive Triple-Negative Breast Cancer Cells. Proc Natl Acad Sci (2018) 115:E1239–48. doi: 10.1073/pnas.1718197115
73. Miyake M, Hori S, Ohnishi S, Toritsuka M, Fujii T, Shimizu T, et al. Supplementary Granulocyte Macrophage Colony-Stimulating Factor to Chemotherapy and Programmed Death-Ligand 1 Blockade Decreases Local Recurrence After Surgery in Bladder Cancer. Cancer Sci (2019) 110:3315–27. doi: 10.1111/cas.14158
74. Peng J, Hamanishi J, Matsumura N, Abiko K, Murat K, Baba T, et al. Chemotherapy Induces Programmed Cell Death-Ligand 1 Overexpression via the Nuclear Factor-κb to Foster an Immunosuppressive Tumor Microenvironment in Ovarian Cancer. Cancer Res (2015) 75:5034–45. doi: 10.1158/0008-5472.CAN-14-3098
75. Morisaki T, Onishi H, Koya N, Kiyota A, Tanaka H, Umebayashi M, et al. Combinatorial Cytotoxicity of Gemcitabine and Cytokine-Activated Killer Cells in Hepatocellular Carcinoma via the NKG2D-MICA/B System. Anticancer Res (2011) 31:2505–10.
76. Miyashita T, Miki K, Kamigaki T, Makino I, Nakagawara H, Tajima H, et al. Low-Dose Gemcitabine Induces Major Histocompatibility Complex Class I-Related Chain a/B Expression and Enhances an Antitumor Innate Immune Response in Pancreatic Cancer. Clin Exp Med (2017) 17:19–31. doi: 10.1007/s10238-015-0394-x
77. Peereboom DM, Alban TJ, Grabowski MM, Alvarado AG, Otvos B, Bayik D, et al. Metronomic Capecitabine as an Immune Modulator in Glioblastoma Patients Reduces Myeloid-Derived Suppressor Cells. JCI Insight (2019) 4:e130748. doi: 10.1172/jci.insight.130748
78. Wakita D, Iwai T, Harada S, Suzuki M, Yamamoto K, Sugimoto M. Cisplatin Augments Antitumor T-Cell Responses Leading to a Potent Therapeutic Effect in Combination With PD-L1 Blockade. Anticancer Res (2019) 39:1749–60. doi: 10.21873/anticanres.13281
79. Hopkins D, Sanchez H, Berwin B, Wilkinson-Ryan I. Cisplatin Increases Immune Activity of Monocytes and Cytotoxic T-Cells in a Murine Model of Epithelial Ovarian Cancer. Transl Oncol (2021) 14:101217. doi: 10.1016/j.tranon.2021.101217
80. Kim WS, Kim H, Kwon KW, Im S-H, Lee BR, Ha S-J, et al. Cisplatin Induces Tolerogenic Dendritic Cells in Response to TLR Agonists via the Abundant Production of IL-10, Thereby Promoting Th2- and Tr1-Biased T-Cell Immunity. Oncotarget (2016) 7:33765–82. doi: 10.18632/oncotarget.9260
81. Balog JÁ, Hackler J, Kovács AK, Neuperger P, Alföldi R, Nagy LI, et al. Single Cell Mass Cytometry Revealed the Immunomodulatory Effect of Cisplatin Via Downregulation of Splenic CD44+, IL-17a+ MDSCs and Promotion of Circulating IFN-γ+ Myeloid Cells in the 4T1 Metastatic Breast Cancer Model. Int J Mol Sci (2019) 21:170. doi: 10.3390/ijms21010170
82. Huang X, Cui S, Shu Y. Cisplatin Selectively Downregulated the Frequency and Immunoinhibitory Function of Myeloid-Derived Suppressor Cells in a Murine B16 Melanoma Model. Immunol Res (2016) 64:160–70. doi: 10.1007/s12026-015-8734-1
83. Ziebart A, Huber U, Jeske S, Laban S, Doescher J, Hoffmann TK, et al. The Influence of Chemotherapy on Adenosine-Producing B Cells in Patients With Head and Neck Squamous Cell Carcinoma. Oncotarget (2018) 9:5834–47. doi: 10.18632/oncotarget.23533
84. Lesterhuis WJ, Punt CJA, Hato SV, Eleveld-Trancikova D, Jansen BJH, Nierkens S, et al. Platinum-Based Drugs Disrupt STAT6-Mediated Suppression of Immune Responses Against Cancer in Humans and Mice. J Clin Invest (2011) 121:3100–8. doi: 10.1172/JCI43656
85. Park S-J, Ye W, Xiao R, Silvin C, Padget M, Hodge JW, et al. Cisplatin and Oxaliplatin Induce Similar Immunogenic Changes in Preclinical Models of Head and Neck Cancer. Oral Oncol (2019) 95:127–35. doi: 10.1016/j.oraloncology.2019.06.016
86. Okita R, Maeda A, Shimizu K, Nojima Y, Saisho S, Nakata M. Effect of Platinum−Based Chemotherapy on the Expression of Natural Killer Group 2 Member D Ligands, Programmed Cell Death−1 Ligand 1 and HLA Class I in Non−Small Cell Lung Cancer. Oncol Rep (2019) 42:839–48. doi: 10.3892/or.2019.7185
87. Grabosch S, Bulatovic M, Zeng F, Ma T, Zhang L, Ross M, et al. Cisplatin-Induced Immune Modulation in Ovarian Cancer Mouse Models With Distinct Inflammation Profiles. Oncogene (2019) 38:2380–93. doi: 10.1038/s41388-018-0581-9
88. Fournel L, Wu Z, Stadler N, Damotte D, Lococo F, Boulle G, et al. Cisplatin Increases PD-L1 Expression and Optimizes Immune Check-Point Blockade in Non-Small Cell Lung Cancer. Cancer Lett (2019) 464:5–14. doi: 10.1016/j.canlet.2019.08.005
89. Zhang P, Ma Y, Lv C, Huang M, Li M, Dong B, et al. Upregulation of Programmed Cell Death Ligand 1 Promotes Resistance Response in Non-Small-Cell Lung Cancer Patients Treated With Neo-Adjuvant Chemotherapy. Cancer Sci (2016) 107:1563–71. doi: 10.1111/cas.13072
90. Ock C-Y, Kim S, Keam B, Kim S, Ahn Y-O, Chung E-J, et al. Changes in Programmed Death-Ligand 1 Expression During Cisplatin Treatment in Patients With Head and Neck Squamous Cell Carcinoma. Oncotarget (2017) 8:97920–7. doi: 10.18632/oncotarget.18542
91. Beyranvand Nejad E, van der Sluis TC, van Duikeren S, Yagita H, Janssen GM, van Veelen PA, et al. Tumor Eradication by Cisplatin Is Sustained by CD80/86-Mediated Costimulation of CD8 + T Cells. Cancer Res (2016) 76:6017–29. doi: 10.1158/0008-5472.CAN-16-0881
92. Ohtsukasa S, Okabe S, Yamashita H, Iwai T, Sugihara K. Increased Expression of CEA and MHC Class I in Colorectal Cancer Cell Lines Exposed to Chemotherapy Drugs. J Cancer Res Clin Oncol (2003) 129:719–26. doi: 10.1007/s00432-003-0492-0
93. Okita R, Yukawa T, Nojima Y, Maeda A, Saisho S, Shimizu K, et al. MHC Class I Chain-Related Molecule A and B Expression Is Upregulated by Cisplatin and Associated With Good Prognosis in Patients With Non-Small Cell Lung Cancer. Cancer Immunol Immunother (2016) 65:499–509. doi: 10.1007/s00262-016-1814-9
94. Stojanovska V, Prakash M, McQuade R, Fraser S, Apostolopoulos V, Sakkal S, et al. Oxaliplatin Treatment Alters Systemic Immune Responses. BioMed Res Int (2019) 2019:1–15. doi: 10.1155/2019/4650695
95. Fu D, Wu J, Lai J, Liu Y, Zhou L, Chen L, et al. T Cell Recruitment Triggered by Optimal Dose Platinum Compounds Contributes to the Therapeutic Efficacy of Sequential PD-1 Blockade in a Mouse Model of Colon Cancer. Am J Cancer Res (2020) 10:473–90.
96. Shalapour S, Font-Burgada J, Di Caro G, Zhong Z, Sanchez-Lopez E, Dhar D, et al. Immunosuppressive Plasma Cells Impede T-Cell-Dependent Immunogenic Chemotherapy. Nature (2015) 521:94–8. doi: 10.1038/nature14395
97. Zhou J, Yang T, Liu L, Lu B. Chemotherapy Oxaliplatin Sensitizes Prostate Cancer to Immune Checkpoint Blockade Therapies via Stimulating Tumor Immunogenicity. Mol Med Rep (2017) 16:2868–74. doi: 10.3892/mmr.2017.6908
98. Kim N-R, Kim Y-J. Oxaliplatin Regulates Myeloid-Derived Suppressor Cell-Mediated Immunosuppression via Downregulation of Nuclear Factor-κb Signaling. Cancer Med (2019) 8:276–88. doi: 10.1002/cam4.1878
99. Stojanovska V, McQuade RM, Fraser S, Prakash M, Gondalia S, Stavely R, et al. Oxaliplatin-Induced Changes in Microbiota, TLR4+ Cells and Enhanced HMGB1 Expression in the Murine Colon. PloS One (2018) 13:e0198359. doi: 10.1371/journal.pone.0198359
100. Zhu X, Xu J, Cai H, Lang J. Carboplatin and Programmed Death-Ligand 1 Blockade Synergistically Produce a Similar Antitumor Effect to Carboplatin Alone in Murine ID8 Ovarian Cancer Model: Chemotherapy and Immunotherapy in ID8. J Obstet Gynaecol Res (2018) 44:303–11. doi: 10.1111/jog.13521
101. Zhou L, Xu Q, Huang L, Jin J, Zuo X, Zhang Q, et al. Low-Dose Carboplatin Reprograms Tumor Immune Microenvironment Through STING Signaling Pathway and Synergizes With PD-1 Inhibitors in Lung Cancer. Cancer Lett (2021) 500:163–71. doi: 10.1016/j.canlet.2020.11.049
102. Anestakis D, Petanidis S, Domvri K, Tsavlis D, Zarogoulidis P, Katopodi T. Carboplatin Chemoresistance Is Associated With CD11b+/Ly6C+ Myeloid Release and Upregulation of TIGIT and LAG3/CD160 Exhausted T Cells. Mol Immunol (2020) 118:99–109. doi: 10.1016/j.molimm.2019.11.008
103. Wahba J, Natoli M, Whilding LM, Parente-Pereira AC, Jung Y, Zona S, et al. Chemotherapy-Induced Apoptosis, Autophagy and Cell Cycle Arrest Are Key Drivers of Synergy in Chemo-Immunotherapy of Epithelial Ovarian Cancer. Cancer Immunol Immunother (2018) 67:1753–65. doi: 10.1007/s00262-018-2199-8
104. Gao Q, Wang S, Chen X, Cheng S, Zhang Z, Li F, et al. Cancer-Cell-Secreted CXCL11 Promoted CD8+ T Cells Infiltration Through Docetaxel-Induced-Release of HMGB1 in NSCLC. J Immunother Cancer (2019) 7:42. doi: 10.1186/s40425-019-0511-6
105. Millrud CR, Mehmeti M, Leandersson K. Docetaxel Promotes the Generation of Anti-Tumorigenic Human Macrophages. Exp Cell Res (2018) 362:525–31. doi: 10.1016/j.yexcr.2017.12.018
106. Guan W, Hu J, Yang L, Tan P, Tang Z, West BL, et al. Inhibition of TAMs Improves the Response to Docetaxel in Castration-Resistant Prostate Cancer. Endocr Relat Cancer (2019) 26:131–40. doi: 10.1530/ERC-18-0284
107. Kodumudi KN, Woan K, Gilvary DL, Sahakian E, Wei S, Djeu JY. A Novel Chemoimmunomodulating Property of Docetaxel: Suppression of Myeloid-Derived Suppressor Cells in Tumor Bearers. Clin Cancer Res (2010) 16:4583–94. doi: 10.1158/1078-0432.CCR-10-0733
108. Si M, Imagawa DK, Ji P, Wei X, Holm B, Kwok J, et al. Immunomodulatory Effects of Docetaxel on Human Lymphocytes. Invest New Drugs (2003) 21:281–90. doi: 10.1023/A:1025408425660
109. Li J-Y, Duan X-F, Wang L-P, Xu Y-J, Huang L, Zhang T-F, et al. Selective Depletion of Regulatory T Cell Subsets by Docetaxel Treatment in Patients With Nonsmall Cell Lung Cancer. J Immunol Res (2014) 2014:1–10. doi: 10.1155/2014/286170
110. Zhang C, Li F, Li J, Xu Y, Wang L, Zhang Y. Docetaxel Down-Regulates PD-1 Expression via STAT3 in T Lymphocytes. Clin Lung Cancer (2018) 19:e675–83. doi: 10.1016/j.cllc.2018.04.010
111. Ahmad G, Mackenzie GG, Egan J, Amiji MM. DHA-SBT-1214 Taxoid Nanoemulsion and Anti–PD-L1 Antibody Combination Therapy Enhances Antitumor Efficacy in a Syngeneic Pancreatic Adenocarcinoma Model. Mol Cancer Ther (2019) 18:1961–72. doi: 10.1158/1535-7163.MCT-18-1046
112. Vicari AP, Luu R, Zhang N, Patel S, Makinen SR, Hanson DC, et al. Paclitaxel Reduces Regulatory T Cell Numbers and Inhibitory Function and Enhances the Anti-Tumor Effects of the TLR9 Agonist PF-3512676 in the Mouse. Cancer Immunol Immunother (2009) 58:615–28. doi: 10.1007/s00262-008-0586-2
113. Yamaguchi T, Fushida S, Yamamoto Y, Tsukada T, Kinoshita J, Oyama K, et al. Low-Dose Paclitaxel Suppresses the Induction of M2 Macrophages in Gastric Cancer. Oncol Rep (2017) 37:3341–50. doi: 10.3892/or.2017.5586
114. Wanderley CW, Colon DF, Luiz JPM, Oliveira FF, Viacava PR, Leite CA, et al. Paclitaxel Reduces Tumor Growth by Reprogramming Tumor-Associated Macrophages to an M1- Profile in a TLR4-Dependent Manner. Cancer Res (2018) 78(20):5891–900. doi: 10.1158/0008-5472.CAN-17-3480
115. Mullins DW, Burger CJ, Elgert KD. Paclitaxel Enhances Macrophage IL-12 Production in Tumor-Bearing Hosts Through Nitric Oxide. J Immunol Baltim Md 1950 (1999) 162:6811–8.
116. Michels T, Shurin GV, Naiditch H, Sevko A, Umansky V, Shurin MR. Paclitaxel Promotes Differentiation of Myeloid-Derived Suppressor Cells Into Dendritic Cells In Vitro in a TLR4-Independent Manner. J Immunotoxicol (2012) 9:292–300. doi: 10.3109/1547691X.2011.642418
117. Sevko A, Michels T, Vrohlings M, Umansky L, Beckhove P, Kato M, et al. Antitumor Effect of Paclitaxel Is Mediated by Inhibition of Myeloid-Derived Suppressor Cells and Chronic Inflammation in the Spontaneous Melanoma Model. J Immunol (2013) 190:2464–71. doi: 10.4049/jimmunol.1202781
118. Alagkiozidis I, Facciabene A, Tsiatas M, Carpenito C, Benencia F, Adams S, et al. Time-Dependent Cytotoxic Drugs Selectively Cooperate With IL-18 for Cancer Chemo-Immunotherapy. J Transl Med (2011) 9:77. doi: 10.1186/1479-5876-9-77
119. Iwai T, Sugimoto M, Wakita D, Yorozu K, Kurasawa M, Yamamoto K. Topoisomerase I Inhibitor, Irinotecan, Depletes Regulatory T Cells and Up-Regulates MHC Class I and PD-L1 Expression, Resulting in a Supra-Additive Antitumor Effect When Combined With Anti-PD-L1 Antibodies. Oncotarget (2018) 9:31411–21. doi: 10.18632/oncotarget.25830
120. Iwai T, Sugimoto M, Wakita D, Yorozu K, Kurasawa M, Yamamoto K. P2.07-006 Irinotecan Augmented Anti-Tumor Activity of Anti-PD-L1 Through Enhancing CD8 Proliferation Regardless of Its Hematotoxicity. J Thorac Oncol (2017) 12:S2417–8. doi: 10.1016/j.jtho.2017.11.065
121. Liang Y-H, Tsai J-H, Cheng Y-M, Chan K-Y, Hsu W-L, Lee C-C, et al. Chemotherapy Agents Stimulate Dendritic Cells Against Human Colon Cancer Cells Through Upregulation of the Transporter Associated With Antigen Processing. Sci Rep (2021) 11:9080. doi: 10.1038/s41598-021-88648-z
122. Ho TTB, Nasti A, Seki A, Komura T, Inui H, Kozaka T, et al. Combination of Gemcitabine and Anti-PD-1 Antibody Enhances the Anticancer Effect of M1 Macrophages and the Th1 Response in a Murine Model of Pancreatic Cancer Liver Metastasis. J Immunother Cancer (2020) 8:e001367. doi: 10.1136/jitc-2020-001367
123. Ma J, Sun D, Wang J, Han C, Qian Y, Chen G, et al. Immune Checkpoint Inhibitors Combined With Chemotherapy for the Treatment of Advanced Pancreatic Cancer Patients. Cancer Immunol Immunother (2020) 69:365–72. doi: 10.1007/s00262-019-02452-3
124. Cui J, Yang H, Hu J, Yao J, Wang Y, Liang Y, et al. Anti-PD-1 Antibody Combined With Albumin-Bound Paclitaxel and Gemcitabine (AG) as First-Line Therapy and Anti-PD-1 Monotherapy as Maintenance in Metastatic Pancreatic Ductal Adenocarcinoma (PDAC). J Clin Oncol (2021) 39:e16218. doi: 10.1200/JCO.2021.39.15_suppl.e16218
125. Kamath SD, Kalyan A, Kircher S, Nimeiri H, Fought AJ, Benson A, et al. Ipilimumab and Gemcitabine for Advanced Pancreatic Cancer: A Phase Ib Study. Oncologist (2020) 25(5):e808–15. doi: 10.1634/theoncologist.2019-0473
126. Alishekevitz D, Gingis-Velitski S, Kaidar-Person O, Gutter-Kapon L, Scherer SD, Raviv Z, et al. Macrophage-Induced Lymphangiogenesis and Metastasis Following Paclitaxel Chemotherapy Is Regulated by VEGFR3. Cell Rep (2016) 17:1344–56. doi: 10.1016/j.celrep.2016.09.083
127. Fukumura D, Xavier R, Sugiura T, Chen Y, Park E-C, Lu N, et al. Tumor Induction of VEGF Promoter Activity in Stromal Cells. Cell (1998) 94:715–25. doi: 10.1016/S0092-8674(00)81731-6
128. Ellis LM, Hicklin DJ. VEGF-Targeted Therapy: Mechanisms of Anti-Tumour Activity. Nat Rev Cancer (2008) 8:579–91. doi: 10.1038/nrc2403
129. Principe DR, Narbutis M, Kumar S, Park A, Viswakarma N, Dorman MJ, et al. Long-Term Gemcitabine Treatment Reshapes the Pancreatic Tumor Microenvironment and Sensitizes Murine Carcinoma to Combination Immunotherapy. Cancer Res (2020) 80:3101–15. doi: 10.1158/0008-5472.CAN-19-2959
130. Emmenegger U, Man S, Shaked Y, Francia G, Wong JW, Hicklin DJ, et al. A Comparative Analysis of Low-Dose Metronomic Cyclophosphamide Reveals Absent or Low-Grade Toxicity on Tissues Highly Sensitive to the Toxic Effects of Maximum Tolerated Dose Regimens. Cancer Res (2004) 64:3994–4000. doi: 10.1158/0008-5472.CAN-04-0580
131. Hermans IF, Chong TW, Palmowski MJ, Harris AL, Cerundolo V. Synergistic Effect of Metronomic Dosing of Cyclophosphamide Combined With Specific Antitumor Immunotherapy in a Murine Melanoma Model. Cancer Res (2003) 63:8408–13.
132. Laquente B, Lacasa C, Ginestà MM, Casanovas O, Figueras A, Galán M, et al. Antiangiogenic Effect of Gemcitabine Following Metronomic Administration in a Pancreas Cancer Model. Mol Cancer Ther (2008) 7:638–47. doi: 10.1158/1535-7163.MCT-07-2122
133. Cham KKY, Baker JHE, Takhar KS, Flexman JA, Wong MQ, Owen DA, et al. Metronomic Gemcitabine Suppresses Tumour Growth, Improves Perfusion, and Reduces Hypoxia in Human Pancreatic Ductal Adenocarcinoma. Br J Cancer (2010) 103:52–60. doi: 10.1038/sj.bjc.6605727
134. Yapp DT, Wong MQ, Kyle AH, Valdez SM, Tso J, Yung A, et al. The Differential Effects of Metronomic Gemcitabine and Antiangiogenic Treatment in Patient-Derived Xenografts of Pancreatic Cancer: Treatment Effects on Metabolism, Vascular Function, Cell Proliferation, and Tumor Growth. Angiogenesis (2016) 19:229–44. doi: 10.1007/s10456-016-9503-z
135. Isacoff WH, Reber HA, Bedford R, Hoos W, Rahib L, Upfill-Brown A, et al. Low-Dose Continuous 5-Fluorouracil Combined With Leucovorin, Nab-Paclitaxel, Oxaliplatin, and Bevacizumab for Patients With Advanced Pancreatic Cancer: A Retrospective Analysis. Target Oncol (2018) 13:461–8. doi: 10.1007/s11523-018-0572-3
136. Motoyoshi Y, Kaminoda K, Saitoh O, Hamasaki K, Nakao K, Ishii N, et al. Different Mechanisms for Anti-Tumor Effects of Low- and High-Dose Cyclophosphamide. Oncol Rep (2006) 16:141–6. doi: 10.3892/or.16.1.141
137. Ghiringhelli F, Menard C, Puig PE, Ladoire S, Roux S, Martin F, et al. Metronomic Cyclophosphamide Regimen Selectively Depletes CD4+CD25+ Regulatory T Cells and Restores T and NK Effector Functions in End Stage Cancer Patients. Cancer Immunol Immunother (2007) 56:641–8. doi: 10.1007/s00262-006-0225-8
138. Wu J, Waxman DJ. Metronomic Cyclophosphamide Eradicates Large Implanted GL261 Gliomas by Activating Antitumor Cd8 + T-Cell Responses and Immune Memory. OncoImmunology (2015) 4:e1005521. doi: 10.1080/2162402X.2015.1005521
139. Browder T, Butterfield CE, Kräling BM, Shi B, Marshall B, O’Reilly MS, et al. Antiangiogenic Scheduling of Chemotherapy Improves Efficacy Against Experimental Drug-Resistant Cancer. Cancer Res (2000) 60:1878–86.
140. Vacca A, Iurlaro M, Ribatti D, Minischetti M, Nico B, Ria R, et al. Antiangiogenesis Is Produced by Nontoxic Doses of Vinblastine. Blood (1999) 94:4143–55. doi: 10.1182/blood.V94.12.4143
141. Lesterhuis WJ, Salmons J, Nowak AK, Rozali EN, Khong A, Dick IM, et al. Synergistic Effect of CTLA-4 Blockade and Cancer Chemotherapy in the Induction of Anti-Tumor Immunity. PloS One (2013) 8:e61895. doi: 10.1371/journal.pone.0061895
142. Markovic SN, Suman VJ, Javed A, Reid JM, Wall DJ, Erickson LA, et al. Sequencing Ipilimumab Immunotherapy Before or After Chemotherapy (Nab-Paclitaxel and Bevacizumab) for the Treatment of BRAFwt (BRAF Wild-Type) Metastatic Malignant Melanoma: Results of a Study of Academic and Community Cancer Research United (ACCRU) RU261206I. Am J Clin Oncol (2020) 43:115–21. doi: 10.1097/COC.0000000000000644
143. Amin S, Baine MJ, Meza JL, Lin C. The Association of the Sequence of Immunotherapy With the Survival of Unresectable Pancreatic Adenocarcinoma Patients: A Retrospective Analysis of the National Cancer Database. Front Oncol (2020) 10:1518. doi: 10.3389/fonc.2020.01518
Keywords: pancreatic ductal adenocarcinoma, schedule, immune checkpoint inhibitor, chemotherapy, metronomic dose
Citation: Kaplon H (2022) Translational Learnings in the Development of Chemo-Immunotherapy Combination to Bypass the Cold Tumor Microenvironment in Pancreatic Ductal Adenocarcinoma. Front. Oncol. 12:835502. doi: 10.3389/fonc.2022.835502
Received: 14 December 2021; Accepted: 15 March 2022;
Published: 18 May 2022.
Edited by:
Alexandr Bazhin, LMU Munich University Hospital, GermanyReviewed by:
Helmout Modjathedi, Kingston University, United KingdomCopyright © 2022 Kaplon. This is an open-access article distributed under the terms of the Creative Commons Attribution License (CC BY). The use, distribution or reproduction in other forums is permitted, provided the original author(s) and the copyright owner(s) are credited and that the original publication in this journal is cited, in accordance with accepted academic practice. No use, distribution or reproduction is permitted which does not comply with these terms.
*Correspondence: Hélène Kaplon, aGVsZW5lLmthcGxvbkBzZXJ2aWVyLmNvbQ==