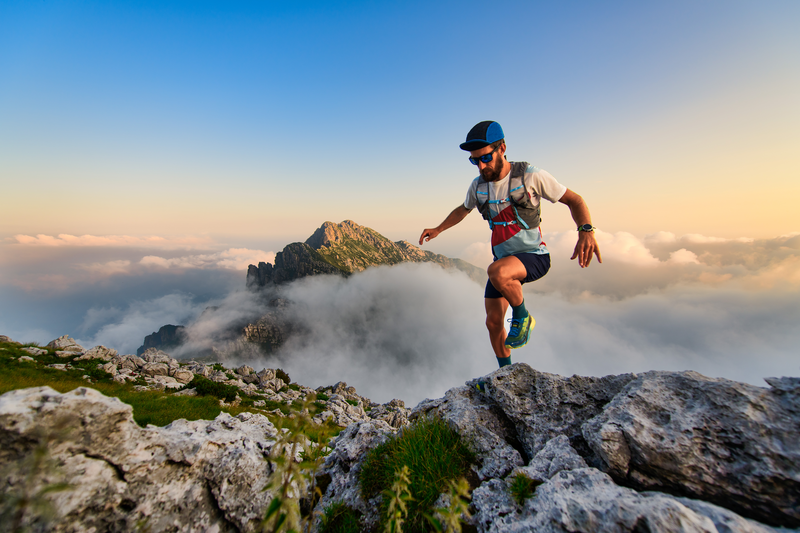
94% of researchers rate our articles as excellent or good
Learn more about the work of our research integrity team to safeguard the quality of each article we publish.
Find out more
REVIEW article
Front. Oncol. , 16 February 2022
Sec. Cancer Molecular Targets and Therapeutics
Volume 12 - 2022 | https://doi.org/10.3389/fonc.2022.830561
Ferroptosis is a newly discovered form of iron-dependent cell death, which is different from other death forms. The main characteristics of ferroptosis are: (1) Amino acid metabolism. (2) Iron metabolism; (3) Lipid metabolism and Reactive oxygen species (ROS). Ferroptosis is related to the occurrence and development of a variety of cancers, especially in the drug resistance. This article reviews the research progress of iron death in tumors, and provides a theoretical reference for its further research and clinical application.
Cell death is essential for maintaining the body’s normal development, homeostasis, and preventing hyperproliferative diseases (such as cancer). The process of regulatory cell death is regulated by specialized molecular mechanisms, so it can be regulated by specific pharmacological methods or genetic intervention (1). Ferroptosis is a newly discovered programmed cell death event. Ferroptosis is different from death forms such as apoptosis, necrosis, and autophagy in terms of morphology, biochemistry, and gene regulation. It does not require energy consumption, is not inhibited by apoptosis inhibitors, and has no intracellular calcium overload (2). Ferroptosis is mainly marked by a significant increase in cytoplasmic iron and lipid ROS, a decrease in mitochondrial volume, and an increase in the thickness of the bilayer membrane (3). In clinical treatment, the multi-drug resistance of cancer cells is one of the important reasons for treatment failure (4). The discovery of ferroptosis provides new ideas for the treatment of cancer and the solution of drug resistance.
DOLMA et al. (4) discovered an anti-tumor drug in 2003, which can induce cell death without causing changes in nuclear morphology, DNA fragmentation and caspase3 activation, and caspase inhibitors cannot reverse this process. Yang et al. (5) discovered the compounds RSL3 and RSL5 that cause this new type of cell death and found that iron chelating agents can inhibit this type of death. In 2012, Dixon et al. (6) were studying tumor cells with RAS mutations. At the time, for the first time, a non-apoptotic iron-dependent cell death method caused by Erastin was defined as ferroptosis (Figure 1). In the following years, several studies confirmed that the reduction of intracellular cysteine content and the massive consumption of GSH played a key role in inducing cell death and proved that lipophilic antioxidants and iron chelators can inhibit such death way, these are the main features of ferroptosis (Figure 2).
Figure 1 Mechanism and treatment of ferroptosis. TFR-1, transferrin receptor 1; PUFA, polyunsaturated fatty acids; SCARA5, scavenger receptor class A member 5; SLC1A5, solute carrier family member 1 member A5; System Xc-,contribution of the cystine-glutamate antiporter; NCOA4; Nuclear receptor coactivator 4; GSH, glutathione; Fer-1, ferrostatin-1;Lip-1, lipoxstatin-1; FSP1, ferroptosis suppressor protein 1; GPX4, glutathione peroxidase 4; p53, tumor suppressor protein 53; TXNRD1, thioredoxin reductase 1; CDO, cysteine dioxygenase 1; RSL3, RAS-selective lethal 3.
The main characteristics of ferroptosis are: (1) Amino acid metabolism. (2) Iron metabolism; (3) Lipid metabolism and ROS
Amino acid metabolism is an important part of the organism’s metabolic circuit. Abnormal amino acid metabolism is closely related to ferroptosis (7). Glutathione is a tripeptide compound composed of 3 amino acids glutamic acid, cysteine and glycine. It is important in the body Antioxidant and free radical scavenger (8). Glutathione can combine with free radicals, heavy metals, etc., to convert harmful poisons in the body into harmless substances to be excreted from the body (9). The structure of glutathione contains a lively sulfhydryl group-SH, which is easily oxidatively dehydrogenated. This specific structure makes it the first line of defense for the elimination of free radicals in the body (10). System Xc- plays an important role in maintaining the balance and distribution of glutathione. Xc- depends on sodium ion and is composed of light chain subunits and heavy chain subunits (11). Xc-can transfer glutamic acid in the cell to the outside of the cell, and at the same time transfer the extracellular cystine into the cell and regulate the synthesis of glutathione by affecting the level of extracellular glutamate. Studies have found that Xc- knockout mice have significantly lower levels of glutamate outside the brain cells and have a milder neurotoxic response to drugs (12).
Erastin is one of the small molecules found in chemical screening that can induce ferroptosis in oncogenic RAS mutant cell lines. In the process of Erastin-induced ferroptosis, System Xc- is the most important mechanism (13). The transporter is composed of SLC7A11 and SLC3A2. It can take up extracellular cystine into the cell at a ratio of 1:1 and is quickly reduced to cysteine to participate in the synthesis of GSH (14). GPX4 is an antioxidant enzyme containing selenoprotein in cells, which is mainly synthesized in the proximal tubules of the kidney and then secreted into the blood to play a role. GPX4 is one of the strongest antioxidant enzymes in the human body It can convert reduced GSH in the cell into oxidized glutathione (GSSG) and at the same time convert the toxic lipid hydrogen peroxide in the cell into non-toxic ester alcohol and regulate intracellular redox balance and protect cell membrane structure and function (15). Erastin inhibits GSH production by inhibiting System Xc- and preventing cystine uptake. When GSH is depleted, it will cause GPX4 inactivation and cause ferroptosis (16). Hao et al. (17) discovered that silencing cysteine dioxygenase 1 (CDO1) can inhibit Erastin-induced ferroptosis of gastric cancer cells. Inhibition of CDO1 can restore cell GSH levels, prevent the production of ROS, and reduce malondialdehyde, which is one of the final products of lipid peroxides. Hayano et al. (18) found in the study of the mechanism of ferroptosis that knocking out the gene producing cysteamide-transport RNA synthetase significantly inhibited the ferroptosis caused by Erastin.
Iron is an indispensable and most abundant trace element in the body, and it participates in many important physiological and biochemical functions in the body. Iron is the main raw material for the synthesis of hemoglobin and myoglobin. It not only participates in the biosynthesis of DNA and ATP but is also an important electron transport chain in mitochondria and a cofactor of metalloproteinases (19). Under normal conditions, the body maintains the homeostasis of iron through food sources of iron and the “iron cycle” (a process in which aging red blood cells release iron ions under the action of heme oxygenase, and macrophages re-engulf and recycle iron). The hepcidin synthesized and secreted by the liver directly regulates the level of serum iron, while the regulation of iron homeostasis in the body’s cells is mainly played by the iron responsive element, the hepcidin system (20). The trivalent iron ion (Fe3+) in the peripheral circulation combines with transferrin to form a complex and then binds to the transferrin receptor on the cell membrane and enters the endosomes in the cell. At this time, Fe3+ is reduced to divalent iron ions (Fe2+) through the Steap3 (six- transmembrane epithelial antigen of the prostate), and then under the mediation of Transferrin Receptor-1(TFR-1), Fe2+ is released from the endosome to the cytoplasm, and part of it is stored in unstable iron tanks. The excess iron is stored in the iron storage protein complex composed of ferritin light chain polypeptide and ferritin heavy chain polypeptide 1, and the remaining part of Fe2+ will be oxidized to Fe3+ and transferred out of cells to participate in iron renewal in the body cycle (21). Increased iron intake or decreased iron output can enhance the sensitivity of cancer cells to oxidative damage and ferroptosis. Fe2+ level in cells is a key factor for lipid peroxidation and induction of ferroptosis, and TF-mediated iron uptake or iron autophagy can increase its level (3). Iron autophagy is the selective autophagy of ferritin, which increases the sensitivity to ferroptosis by controlling the available iron (22). Nuclear receptor coactivator 4 (NCOA4) is a specific transport receptor for iron autophagy, which can transport ferritin to autophagosomes for lysosomal degradation and release of free iron (23).
Lipids are important regulators of cell death. In mammals, both apoptosis and non-apoptotic pathways can be induced, regulated or inhibited by different lipid signals (24). Unlike other types of cell death, ferroptosis does not require protein effectors like pore-forming proteins. Lipid oxidative stress and the membrane damage caused by it are the key to ferroptosis, especially polyunsaturated fatty acids (PUFA), which forms lipid peroxides and induces ferroptosis (25). PUFA participates in the lipid bilayer structure that composes the cell membrane, and it is an important target of lipid peroxidation in the ferroptosis process of the cell membrane (2).
ROS are produced by normal physiological processes and play an important role in cell signal transduction and tissue homeostasis. However, excessive reactive oxygen free radicals produce unfavorable modifications to cell components, such as lipid, protein and DNA damage (26). Biological cell membranes or organelle membranes have lots of PUFAs and they are particularly vulnerable to ROS damage, which is called “lipid peroxidation” (27). Lipid peroxidation induced by ROS plays an important role in cell death. Lipid peroxidation directly damages phospholipids and can also be used as a cell death signal to induce programmed cell death. Accompanied by the accumulation of ROS, ferroptosis is induced by erastin and RSL3 (a selective ferroptosis inducer). It was found that ferrostatin-1 (Fer-1) and lipoxstatin-1 (Lip-1) can prevent erastin-induced accumulation of ROS, which also verifies the important role of ROS accumulation in promoting ferroptosis (28).
Gliomas, especially glioblastoma, are the most common malignant tumors in the brain. The incidence rate of glioma is about 6/100 000 and is steadily increasing (29). Through research on ferroptosis, it is found that some transcription factors play an important role in the proliferation, migration and malignant transformation of glioma, and their mechanism of action is closely related to ferroptosis (30).
Chen et al. (31) found that when the expression of ATF4 increases, activated ATF4 will increase the expression of Xc-. The inhibition of Xc- can promote the occurrence of glioma cell ferroptosis. and studies have shown that down-regulating the expression of ATF4 can Inhibit the activity of Xc-, thereby enhancing the sensitivity of nerve tumor cells to ferroptosis, so that the proliferation and production of tumors and their vasculature can be controlled. Therefore, ATF4 may be an effective target for inhibiting tumor cell proliferation and tumor blood vessel growth. Fan et al. (32) found that the overexpression of Nrf2 and the knockout of Keap1 can promote the proliferation and migration of glioma tumor cells; the mechanism may be through Up-regulating the activity of Xc- changes the tumor microenvironment and inhibits the ferroptosis of tumor cells. Down-regulating the expression of Nrf2 in glioma cells can promote ferroptosis of tumor cells. The mechanism may be through inhibiting the activity of Xc-, reducing the secretion of glutamate, increasing the production of intracellular lipid ROS, and promoting ferroptosis. It was also found that Nrf2 controlled glutathione synthesis and plays a central role in IDH1 mutated glioma cell physiology (33). At the same time, down-regulating the expression of Nrf2 can increase the sensitivity of glioma cells to inducers of ferroptosis (such as erastin, RSL3).
Pseudolaricacid B (PAB) has been shown to inhibit the growth of glioma cells in animal and cell experiments (34). Because it can increase the content of ferrous iron in cells, and intracellular iron can regulate the expression of NOX4, thereby increasing intracellular hydrogen peroxide and the formation of lipid ROS; PAB can also inhibit the function of SLC7A11 by activating P53, reducing the content of GPX4 in the cell, lead to accumulation of lipid ROS in the cell, and ultimately lead to ferroptosis of glioma cells (35).
Temozolomide (TMZ) is an effective drug for the treatment of high-grade gliomas, which can delay the survival of patients (36). Sehm et al. (37) found that TMZ combined with ferroptosis inducers can enhance the therapeutic effect. Experiments have found that when TMZ is combined with erastin, it can increase its anti-cancer effect. Up-regulation of Xc- expression will make tumor cells more effective. The therapeutic effect of TMZ combined with erastin is more sensitive. Gao et al. (38) found that ibuprofen can down-regulate the expression of GPX4 and Xc- in glioblastoma, and the decrease in the expression of GPX4 and Xc- is related to the down-regulation of Nrf2 expression. Nrf2 can regulate the expression of GPX4 and Xc- in glioblastoma. The expression of ibuprofen may inhibit the activity of GPX4 and Xc- by down-regulating the expression of Nrf2, which increases the production of lipid ROS in tumor cells, which in turn promotes the occurrence of ferroptosis in glioblastoma.
Lung cancer is one of the most common malignant tumors in the world. In 2018, there are an estimated 2.1 million new cases and nearly 1.7 million deaths, while the incidence of lung cancer and mortality ranks first among all tumors in China (39). Epidemiological and laboratory studies have confirmed that iron overload is related to the occurrence and development of lung cancer, and there is a significant positive correlation between high iron intake and lung cancer risk. Data from a clinical trial showed that the serum iron, ferritin, and total iron binding capacity of lung cancer patients were significantly higher than those of healthy controls. The higher the serum iron concentration, the greater the risk of lung cancer (40). A study in Taiwan enrolled 309,443 people from 2018 to 2009. The median follow-up time of non-tumor population was 7.07 years, of which 8,060 cases were diagnosed with tumors and 3,066 cases of Death due to tumor, high serum iron (>120 μg/dL) increases the risk of morbidity and death of malignant tumors and is positively correlated with tumor morbidity and mortality (41).
In recent years, some researchers have discovered that ferroptosis is suppressed in lung cancer cells. Ji et al. (42) found that lung cancer cells can directly target GSH by up-regulating System Xc- to increase cellular antioxidant capacity. Lai et al. (43) found that lung cancer cells inhibit ferroptosis by directly upregulating the expression of GPX4. Serine threonine tyrosine kinase 1 (STYK1) is highly expressed in NSCLC cells, which in turn promotes the expression of GPX4 and promotes the Proliferation of lung cancer cells, which attenuates a variety of mitochondrial abnormalities caused by ferroptosis, leading to suppression of ferroptosis in NSCLC. FSP1 is an ferroptosis inhibitor independent of the classical GPX4 signaling pathway (44). When the GPX4 gene of lung cancer cells is deleted, FSP1 would be modified by myristoylation, and CoQ10 is reduced by NAD (P) h to produce radial trapping antioxidants (RTA) to prevent lipid peroxidation and inhibit ferroptosis (45). The higher the expression level of FSP1, the greater the ferroptosis resistance of lung cancer cells. FSP1 inhibitor (ifsp1) can reverse the ferroptosis resistance caused by FSP1, increasing the sensitivity of lung cancer cells to ferroptosis and promoting ferroptosis of lung cancer cells (46).
Among the drugs for the treatment of lung cancer, some have been proved to induce ferroptosis. Cisplatin (DDP) promotes lipid peroxidation, increases MDA, ROS, promotes the expression of HO-1 and NQO-1, and induces ferroptosis of lung cancer cells, and this process can be inhibited by Fer-1 (47). The activation of the Nrf2/Xc- pathway is one of the main mechanisms of NSCLC cell resistance to cisplatin. Erastin and sorafenib inhibit the expression of Nrf2 downstream target gene Xc- deplete GSH, induce ferroptosis, reduce cell viability, and enhance the sensitivity of NSCLC cells to cisplatin (48). On the contrary, overexpression of SLC7A11 enhances the resistance of lung cancer cells to cisplatin (49).
Ferroptosis may play a role in lung cancer radiotherapy. After radiotherapy, tumor cells showed typical ferroptosis morphological changes—mitochondria were concentrated, membrane density increased, and mitochondrial ridges decreased (50). Radiotherapy can generate a large amount of ROS and up-regulate the expression of key enzymes to promote lipid peroxidation and eventually lead to ferroptosis (51).
Hepatocellular carcinoma (HCC) is one of the malignant tumors that cause a serious global burden of disease, ranking sixth among the most common cancers and second among cancer-related deaths (52). In recent years, many studies have confirmed that HCC is related to ferroptosis (53). Ferroptosis is mainly regulated by system Xc- and GPX4 and affecting the activity of system Xc- or GPX4 can induce ferroptosis of liver cancer cells (54). p53 is a tumor suppressor gene that affects the occurrence and development of HCC by regulating ferroptosis (55). Xie et al. found that p53 down-regulates the transcription of SLC7A11 and affects the activity of system Xc-, thereby inducing ferroptosis of liver cancer cells (56). Glutaminase-2 is a key enzyme for the conversion of glutamine to glutamate and regulates GSH synthesis. p53 up-regulates glutaminase 2 transcription, and its overexpression inhibits HCC tumor cell growth and colony formation (57).
Sorafenib, as a first-line drug for advanced HCC, has been proven to induce ferroptosis in HCC cells. Sorafenib can inhibit the expression of SLC7A11 and activate the downstream ferroptosis pathway with its own RAF kinase inhibitor effect (58). Sun et al. (8) found that liver cancer cells under oxidative stress activate their own P62-Keap1-NRF2 signaling pathway to up-regulate target genes involved in iron and ROS metabolism downstream of NRF2, such as quinone oxido-reductase 1 (NQO1), heme oxygenase 1 (HO-1) and ferritin heavy chain-1 (FTH1), thereby enhancing Sorafenib’s ferroptosis resistance (59). In addition, a psychotropic drug, haloperidol, was reported to have a sensitizing effect on sorafenib through the ferroptosis route (60).
Osteosarcoma is the most common primary bone malignant tumor, which is common in young people. The 5-year survival rate is 60% ~ 70%, and the mortality caused by lung metastasis is 30% ~ 40% (61).
WU et al. (34) reported the high expression of TFR1 in osteosarcoma and further confirmed that the high expression of TFR1 is significantly related to the histological grade, stage and distant metastasis of the tumor (62). TFR1 is the main protein for iron absorption, which is very important in ferroptosis. Liu et al. (63)found that promoting ferroptosis of osteosarcoma cells can enhance the sensitivity of target cells to cisplatin. Further research found that the resistance of osteosarcoma cells to cisplatin was also enhanced after treatment of non-resistant strains with ferroptosis inhibitors (64).
Ovarian cancer was the seventh most common women cancer in the world, with around 240,000 new cases each year. Ovarian cancer is the second most common malignancy in women over the age of 40, particularly in developed countries (65). It also has many connections with ferroptosis. Basuli et al. (66) found that increased intracellular iron levels are related to the occurrence of ovarian cancer. Compared with normal ovarian tissue, high-grade serous ovarian cancer tissues have decreased FPN, increased TFR1 and TF, and increased iron levels. The genetic model of the initiating cells of ovarian cancer also shows that the iron outflow pump is reduced, and the expression of iron transport-related proteins is up-regulated (67).
Many studies have confirmed that increased intracellular glutathione levels and high expression of related metabolic enzymes are closely related to the drug resistance of ovarian cancer (68). Liu et al. (69) established an Erastin-resistant cell line and found that the cell line can still maintain the content of glutathione, suggesting that there are other ways to synthesize cystine in the cell. Verschoor et al. (70) used Xc- system inhibitors and transsulfide pathway inhibitors to treat two ovarian cancer cell models, and found that intracellular glutathione levels were significantly reduced after the transsulfuration pathway was inhibited, indicating that the transsulfuration pathway has an effect on ovarian cancer cells. The synthesis of glutathione is very important. Chakraborty et al. (71) found that in a small number of ovarian cancer cell lines, the expression of CBS in the transsulfide pathway was increased, and CBS gene silencing could inhibit cell migration and invasion of ovarian cancer cells.
Artemisinin is a classic antimalarial drug. The cellular response of tumor cells to artemisinin and its derivatives involves ferroptosis, apoptosis, necrosis and other cell death methods (72). After the treatment of ovarian cancer cells with artemisinate, a large amount of ROS is produced in the cells, and the proliferation is reduced, which shows that artemisinin may play a role in ovarian cancer therapy (73).
Ferroptosis is an important form of regulatory necrosis, which is different from other cell necrosis and apoptosis in morphology, biochemistry and genetics. The mechanism of ferroptosis is closely related to cell metabolism, involving a variety of key molecules and signal pathways, and regulating the synthesis or decomposition of these key molecules and the signal pathways involved will change the sensitivity of cells to ferroptosis. Reasonable induction or inhibition of ferroptosis will improve the treatment of a variety of diseases, especially cancer-associated malignancies. However, many problems remain to be solved in anti-tumor treatments that target ferroptosis. Firstly, several studies have shown that inhibiting the expression of GPX4 gene can effectively kill tumor cells through ferroptosis. Furthermore, GPX4 is a candidate prognostic biomarker for many cancers (74), so it is necessary to further clarify whether GPX4 is an oncogene. Secondly, immunotherapy is a relatively new anti-tumor treatment, and its relationship with ferroptosis is in the preliminary exploration stage. The relevant mechanism has not yet been fully clarified, and further research is needed. Thirdly, Although some scholars have proposed initial ideas to target SLC7A11 for cancer treatment, such as directly inhibiting the activity of SLC7A11 transport protein, or starting from the metabolic vulnerability of cancer and SLC7A11. However, further research and marketing of drugs targeting SLC7A11 is still necessary, and more studies are needed to determine the safety and efficacy of p53-related drugs (75). In addition, whether the nanomedicine designed with ferroptosis as the target has obvious anti-tumor effects in the human body (76) should be further confirmed by clinical experiments. In short, opportunities and challenges coexist. Ferroptosis is a promising target for anti-tumor therapy and its clinical application will surely bring good news to cancer patients.
CY: Conceptualization, writing - original draft, writing - review and editing. ZF: Formal analysis, investigation, writing - review and editing. SH: Investigation, Resources, Writing - review and editing. CL, Supervision, writing - review and editing. YX: Grammar curation, investigation. SL: Supervision, editing. ZF and SH are co-first authors. All authors contributed to the article and approved the submitted version.
The authors declare that the research was conducted in the absence of any commercial or financial relationships that could be construed as a potential conflict of interest.
All claims expressed in this article are solely those of the authors and do not necessarily represent those of their affiliated organizations, or those of the publisher, the editors and the reviewers. Any product that may be evaluated in this article, or claim that may be made by its manufacturer, is not guaranteed or endorsed by the publisher.
1. Kist M, Vucic D. Cell Death Pathways: Intricate Connections and Disease Implications. EMBO J (2021) 40(5):e106700. doi: 10.15252/embj.2020106700
2. Wan SY, Stockwell BR. Ferroptosis: Death by Lipid Peroxidation. Trends Cell Biol (2015) 26(3):165–76. doi: 10.1016/j.tcb.2015.10.014
3. Xie Y, Hou W, Song X, Yu Y, Huang J, Sun X, et al. Ferroptosis: Process and Function. Cell Death Differ (2016) 23(3):369–79. doi: 10.1038/cdd.2015.158
4. Larsson R, Kristensen J, Sandberg C, Nygren P. Laboratory Determination of Chemotherapeutic Drug Resistance in Tumor Cells From Patients With Leukemia, Using a Fluorometric Microculture Cytotoxicity Assay (FMCA). Int J Cancer (2010) 50(2):177–85. doi: 10.1002/ijc.2910500204
5. Wan SY, Stockwell BR. Synthetic Lethal Screening Identifies Compounds Activating Iron-Dependent, Nonapoptotic Cell Death in Oncogenic-RAS-Harboring Cancer Cells. Chem Biol (2008) 15(3):234–45. doi: 10.1016/j.chembiol.2008.02.010
6. Dixon S, Skouta R, Zaitsev E, Gleason C. Ferroptosis: An Iron-Dependent Form of Nonapoptotic Cell Death. Cell (2012) 149(5):1060–72. doi: 10.1016/j.cell.2012.03.042
7. Angeli J, Shah R, Pratt DA. Ferroptosis Inhibition: Mechanisms and Opportunities. Trends Pharmacol Sci (2017) 38(5):489–98. doi: 10.1016/j.tips.2017.02.005
8. Hayes JD, Flanagan JU, Jowsey IR. Glutathione Transferases. Arabidopsis Book (2005) 8(45):e0131. doi: 10.1199/tab.0131
9. Ursini F, Maiorino M. Lipid Peroxidation and Ferroptosis: The Role of GSH and Gpx4. Free Radical Biol Med (2020) 152:175–85. doi: 10.1016/j.freeradbiomed.2020.02.027
10. Depuydt M, Messens J, Collet JF. How Proteins Form Disulfide Bonds. Antioxid Redox Signal. Antioxid Redox Signaling (2011) 15(1):49–66. doi: 10.1089/ars.2010.3575
11. Zimmermann P, Bette M, Giel G, Stuck BA, Mandic R. Influence of the Xc-Cystine/Glutamate Antiporter Inhibitor Sulfasalazine on the Growth of Head and Neck Squamous Cell Carcinoma Cell Lines. Laryngo-Rhino-Otologie (2018) 97(S 02):S146. doi: 10.1055/s-0038-1640217
12. Taguchi K, Tamba M, Bannai S, Sato H. Induction of Cystine/Glutamate Transporter in Bacterial Lipopolysaccharide Induced Endotoxemia in Mice. J Inflamm (2007) 4(1):20. doi: 10.1186/1476-9255-4-20
13. Wang YQ, Chang SY, Wu Q, Gou YJ, Jia LP, Cui YM, et al. The Protective Role of Mitochondrial Ferritin on Erastin-Induced Ferroptosis. Front Aging Neurosci (2016) 8:308–. doi: 10.3389/fnagi.2016.00308
14. Chen L, Qiao L, Bian Y, Sun X. GDF15 Knockdown Promotes Erastin-Induced Ferroptosis by Decreasing SLC7A11 Expression. Biochem Biophys Res Commun (2020) 526(2):293–9. doi: 10.1016/j.bbrc.2020.03.079
15. Yang W, Sriramaratnam R, Welsch M, Shimada K, Skouta R, Viswanathan V, et al. Regulation of Ferroptotic Cancer Cell Death by GPX4. Cell (2014) 156(1-2):317–31. doi: 10.1016/j.cell.2013.12.010
16. Sato M, Kusumi R, Hamashima S, Kobayashi S, Sasaki S, Komiyama Y, et al. The Ferroptosis Inducer Erastin Irreversibly Inhibits System Xc and Synergizes With Cisplatin to Increase Cisplatin's Cytotoxicity in Cancer Cells. Sci Rep (2018) 8(1):968. doi: 10.1038/s41598-018-19213-4
17. Hao S, Yu J, He W, Huang Q, Zhao Y, Liang B, et al. Cysteine Dioxygenase 1 Mediates Erastin-Induced Ferroptosis in Human Gastric Cancer Cells. Neoplasia (2017) 19(12):1022–32. doi: 10.1016/j.neo.2017.10.005
18. Hayano M, Yang WS, Corn CK, Pagano NC, Stockwell BR. Loss of cysteinyl-tRNA Synthetase (CARS) Induces the Transsulfuration Pathway and Inhibits Ferroptosis Induced by Cystine Deprivation. Cell Death Differ (2016) 23(2):270–8. doi: 10.1038/cdd.2015.93
19. Akatsuka S, Toyokuni S. Iron Function and Carcinogenesis. Nihon Rinsho Jpn J Clin Med (2016) 74(7):1168.
20. Andrews NC, Schmidt PJ. Iron Homeostasis. Annu Rev Physiol (2007) 69(1):69–85. doi: 10.1146/annurev.physiol.69.031905.164337
21. Yu Y, Kovacevic Z, Richardson DR. Tuning Cell Cycle Regulation With an Iron Key. Cell Cycle (2007) 6(16):1982–94. doi: 10.4161/cc.6.16.4603
22. Uberti VH, Freitas B, Molz P, Bromberg E, Schrder N. Iron Overload Impairs Autophagy: Effects of Rapamycin in Ameliorating Iron-Related Memory Deficits. Mol Neurobiol (2020) 57(Pt 8):1044–54. doi: 10.1007/s12035-019-01794-4
23. Fujimaki M, Furuya N, Saiki S, Amo T, Hattori N. Iron Supply via NCOA4-Mediated Ferritin Degradation Maintains Mitochondrial Functions. Mol Cell Biol (2019) 39(14):e00010–19. doi: 10.1128/MCB.00010-19
24. Skouta R, Dixon SJ, Wang J, Dunn DE, Orman M, Shimada K, et al. Ferrostatins Inhibit Oxidative Lipid Damage and Cell Death in Diverse Disease Models. J Am Chem Soc (2014) 136(12):4551–6. doi: 10.1021/ja411006a
25. Qi J, Kim JW, Zhou Z, Lim CW, Kim B. Ferroptosis Affects the Progression of Nonalcoholic Steatohepatitis via the Modulation of Lipid Peroxidation-Mediated Cell Death in Mice. Am J Of Pathol (2019) 190(1):68–81. doi: 10.1016/j.ajpath.2019.09.011
26. Apel K, Hirt H. Reactive Oxygen Species: Metabolism, Oxidative Stress, and Signal Transduction. Annu Rev Plant Biol (2004) 55(1):373–99. doi: 10.1146/annurev.arplant.55.031903.141701
27. Esterbauer H, Gebicki J, Puhl H, Jürgens G. The Role of Lipid Peroxidation and Antioxidants in Oxidative Modification of LDL. Free Radical Biol Med (1992) 13(4):341–90. doi: 10.1016/0891-5849(92)90181-F
28. Li J, Cao F, Yin H, Huang Z, Lin Z, Mao N, et al. Ferroptosis: Past, Present and Future. Cell Death Dis (2020) 11(2):1–13. doi: 10.1038/s41419-020-2298-2
29. Bush NAO, Chang SM, Berger MS. Current and Future Strategies for Treatment of Glioma. Neurosurg Rev (2017) 40(1):1–14. doi: 10.1007/s10143-016-0709-8
30. Liu H-J, Hu H, Li G, Zhang Y, Wu F, Liu X, et al. Ferroptosis-Related Gene Signature Predicts Glioma Cell Death and Glioma Patient Progression. Front Cell Dev Biol (2020) 8:538. doi: 10.3389/fcell.2020.00538
31. Chen D, Fan Z, Rauh M, Buchfelder M, Eyupoglu IY, Savaskan N. ATF4 Promotes Angiogenesis and Neuronal Cell Death and Confers Ferroptosis in a xCT-Dependent Manner. Oncogene (2017) 36(40):5593–608. doi: 10.1038/onc.2017.146
32. Fan Z, Wirth AK, Chen D, Wruck CJ, Rauh M, Buchfelder M, et al. Nrf2-Keap1 Pathway Promotes Cell Proliferation and Diminishes Ferroptosis. Oncogenesis (2017) 6(8):e371. doi: 10.1038/oncsis.2017.65
33. Tang X, Fu X, Liu Y, Yu D, Cai SJ, Yang C. Blockade of Glutathione Metabolism in IDH1-Mutated Glioma. Mol Cancer Ther (2020) 19(1):221–30. doi: 10.1158/1535-7163.MCT-19-0103
34. Chen ZJ, Sun DT, Yuan Y. Inhibitory Effect of Pseudolaric Acid B on Proliferation of Rat Glioma C6 Cells. Acad J Second Military Med Univ (2012) 32(5):484–7. doi: 10.3724/SP.J.1008.2012.00484
35. Wang Z, Ding Y, Wang X, Lu S, Wang C, He C, et al. Pseudolaric Acid B Triggers Ferroptosis in Glioma Cells via Activation of Nox4 and Inhibition of xCT. Cancer Lett (2018) 428:21–33. doi: 10.1016/j.canlet.2018.04.021
36. Ostermann and S. Plasma and Cerebrospinal Fluid Population Pharmacokinetics of Temozolomide in Malignant Glioma Patients. Clin Cancer Res (2004) 10(11):3728. doi: 10.1158/1078-0432.CCR-03-0807
37. Sehm T, Rauh M, Wiendieck K, Buchfelder M, Eyüpoglu I, Savaskan NE. Temozolomide Toxicity Operates in a xCT/SLC7a11 Dependent Manner and Is Fostered by Ferroptosis. Oncotarget (2016) 7(46):74630–47. doi: 10.18632/oncotarget.11858
38. Gao X, Guo N, Xu H, Pan T, Xu L. Ibuprofen Induces Ferroptosis of Glioblastoma Cells via Downregulation of Nuclear Factor Erythroid 2-Related Factor 2 Signaling Pathway. Anti-Cancer Drugs (2019) 31(1):1. doi: 10.1097/CAD.0000000000000825
39. Kuang Y, Wang Q. Iron and Lung Cancer. Cancer Lett (2019) 464:56–61. doi: 10.1016/j.canlet.2019.08.007
40. Sukiennicki GM, Marciniak W, Muszyńska M, Baszuk P, Gupta S, Białkowska K, et al. Iron Levels, Genes Involved in Iron Metabolism and Antioxidative Processes and Lung Cancer Incidence. PloS One (2019) 14(1):e0208610. doi: 10.1371/journal.pone.0208610
41. Wen CP, Lee JH, Tai YP, Wen C, Wu SB, Tsai MK, et al. High Serum Iron Is Associated With Increased Cancer Risk. Cancer Res (2014) 74(22):6589–97. doi: 10.1158/0008-5472.CAN-14-0360
42. Ji X, Qian J, Rahman SJ, Siska PJ, Zou Y, Harris BK, et al. xCT (SLC7A11)-Mediated Metabolic Reprogramming Promotes Non-Small Cell Lung Cancer Progression. Oncogene (2018) 37(36):5007–19. doi: 10.1038/s41388-018-0307-z
43. Lai Y, Zhang Z, Li J, Li W, Huang Z, Zhang C, et al. STYK1/NOK Correlates With Ferroptosis in Non-Small Cell Lung Carcinoma. Biochem Biophys Res Commun (2019) 519(4):659–66. doi: 10.1016/j.bbrc.2019.09.032
44. Doll S, Freitas FP, Shah R, Aldrovandi M, Silva MC, Ingold I, et al. FSP1 Is a Glutathione-Independent Ferroptosis Suppressor. Nature (2019) 575(7784):693–8. doi: 10.1038/s41586-019-1707-0
45. Bersuker K, Hendricks JM, Li Z, Magtanong L, Ford B, Tang PH, et al. The CoQ Oxidoreductase FSP1 Acts Parallel to GPX4 to Inhibit Ferroptosis. Nature (2019) 575(7784):688–92. doi: 10.1038/s41586-019-1705-2
46. Conrad M, Pratt DA. The Chemical Basis of Ferroptosis. Nat Chem Biol (2019) 15(12):1137–47. doi: 10.1038/s41589-019-0408-1
47. Song Z, Jia G, Ma P, Cang S. Exosomal miR-4443 Promotes Cisplatin Resistance in Non-Small Cell Lung Carcinoma by Regulating FSP1 M6a Modification-Mediated Ferroptosis. Life Sci (2021) 276:119399. doi: 10.1016/j.lfs.2021.119399
48. Li Y, Yan H, Xu X, Liu H, Wu C, Zhao L. Erastin/sorafenib Induces Cisplatin−Resistant Non−Small Cell Lung Cancer Cell Ferroptosis Through Inhibition of the Nrf2/xCT Pathway. Oncol Lett (2020) 19(1):323–33. doi: 10.3892/ol.2019.11066
49. Lou J-S, Zhao L-P, Huang Z-H, Chen X-Y, Xu JT, Tai C-S, et al. Ginkgetin Derived From Ginkgo Biloba Leaves Enhances the Therapeutic Effect of Cisplatin via Ferroptosis-Mediated Disruption of the Nrf2/HO-1 Axis in EGFR Wild-Type Non-Small-Cell Lung Cancer. Phytomedicine (2021) 80:153370. doi: 10.1016/j.phymed.2020.153370
50. Lang X, Green MD, Wang W, Yu J, Choi JE, Jiang L, et al. Radiotherapy and Immunotherapy Promote Tumoral Lipid Oxidation and Ferroptosis via Synergistic Repression of SLC7A11. Cancer Discov (2019) 9(12):1673–85. doi: 10.1158/2159-8290.CD-19-0338
51. Wu Y, Yu C, Luo M, Cen C, Qiu J, Zhang S, et al. Ferroptosis in Cancer Treatment: Another Way to Rome. Front Oncol (2020) 10:571127. doi: 10.3389/fonc.2020.571127
52. McGlynn KA, Petrick JL, El-Serag HB. Epidemiology of Hepatocellular Carcinoma. Hepatology (2021) 73:4–13. doi: 10.1002/hep.31288
53. Liang J-Y, Wang D-S, Lin H-C, Chen X-X, Yang H, Zheng Y, et al. A Novel Ferroptosis-Related Gene Signature for Overall Survival Prediction in Patients With Hepatocellular Carcinoma. Int J Biol Sci (2020) 16(13):2430. doi: 10.7150/ijbs.45050
54. Wang Q, Bin C, Xue Q, Gao Q, Huang A, Wang K, et al. GSTZ1 Sensitizes Hepatocellular Carcinoma Cells to Sorafenib-Induced Ferroptosis via Inhibition of NRF2/GPX4 Axis. Cell Death Dis (2021) 12(5):1–16. doi: 10.1038/s41419-021-03718-4
55. Zhang H, Liu R, Sun L, Guo W, Hu X. The Effect of Ferroptosis-Related Genes on Prognosis and Tumor Mutational Burden in Hepatocellular Carcinoma. J Oncol (2021) 2021:7391560. doi: 10.1155/2021/7391560
56. Xie Y, Zhu S, Song X, Sun X, Fan Y, Liu J, et al. The Tumor Suppressor P53 Limits Ferroptosis by Blocking DPP4 Activity. Cell Rep (2017) 20(7):1692–704. doi: 10.1016/j.celrep.2017.07.055
57. Kang R, Kroemer G, Tang D. The Tumor Suppressor Protein P53 and the Ferroptosis Network. Free Radical Biol Med (2018) S0891584918309079. doi: 10.1016/j.freeradbiomed.2018.05.074
58. Nie J, Lin B, Zhou M, Wu L, Zheng T. Role of Ferroptosis in Hepatocellular Carcinoma. J Cancer Res Clin Oncol (2018) 144(12):2329–37. doi: 10.1007/s00432-018-2740-3
59. Sun J, Zhou C, Zhao Y, Zhang X, Chen W, Zhou Q, et al. Quiescin Sulfhydryl Oxidase 1 Promotes Sorafenib-Induced Ferroptosis in Hepatocellular Carcinoma by Driving EGFR Endosomal Trafficking and Inhibiting NRF2 Activation. Redox Biol (2021) 41:101942. doi: 10.1016/j.redox.2021.101942
60. Bai T, Wang S, Zhao Y, Zhu R, Wang W, Sun Y. Haloperidol, A Sigma Receptor 1 Antagonist, Promotes Ferroptosis in Hepatocellular Carcinoma Cells. Biochem Biophys Res Commun (2017) 491(4):919–25. doi: 10.1016/j.bbrc.2017.07.136
61. Czarnecka AM, Synoradzki K, Firlej W, Bartnik E, Sobczuk P, Fiedorowicz M, et al. Molecular Biology of Osteosarcoma. Cancers (2020) 12(8):2130. doi: 10.3390/cancers12082130
62. Wu H, Zhang J, Dai R, Xu J, Feng H. Transferrin Receptor-1 and VEGF Are Prognostic Factors for Osteosarcoma. J Orthopaedic Surg Res (2019) 14(1):1–5. doi: 10.1186/s13018-019-1301-z
63. Liu Q, Wang K. The Induction of Ferroptosis by Impairing STAT3/Nrf2/GPx4 Signaling Enhances the Sensitivity of Osteosarcoma Cells to Cisplatin. Cell Biol Int (2019) 43(11):1245–56. doi: 10.1002/cbin.11121
64. Zhao J, Zhao Y, Ma X, Zhang B, Feng H. Targeting Ferroptosis in Osteosarcoma. J Bone Oncol (2021) p:100380. doi: 10.1016/j.jbo.2021.100380
65. Stenzel AE, Buas MF, Moysich KB. Survival Disparities Among Racial/Ethnic Groups of Women With Ovarian Cancer: An Update on Data From the Surveillance, Epidemiology and End Results (SEER) Registry. Cancer Epidemiol (2019) 62:101580. doi: 10.1016/j.canep.2019.101580
66. Basuli D, Tesfay L, Deng Z, Paul B, Yamamoto Y, Ning G, et al. Iron Addiction: A Novel Therapeutic Target in Ovarian Cancer. Oncogene (2017) 36(29):4089–99. doi: 10.1038/onc.2017.11
67. Carbone M, Melino G. Stearoyl CoA Desaturase Regulates Ferroptosis in Ovarian Cancer Offering New Therapeutic Perspectives. Cancer Res (2019) 79(20):5149–50. doi: 10.1158/0008-5472.CAN-19-2453
68. Nagle CM, Chenevix-Trench G, Spurdle AB, Webb PM. The Role of Glutathione-S-Transferase Polymorphisms in Ovarian Cancer Survival. Eur J Cancer (2007) 43(2):283–90. doi: 10.1016/j.ejca.2006.09.011
69. Liu N, Lin X, Huang C. Activation of the Reverse Transsulfuration Pathway Through NRF2/CBS Confers Erastin-Induced Ferroptosis Resistance. Br J Cancer (2020) 122(2):279–92. doi: 10.1038/s41416-019-0660-x
70. Verschoor ML, Singh G. Ets-1 Regulates Intracellular Glutathione Levels: Key Target for Resistant Ovarian Cancer. Mol Cancer (2013) 12:138. doi: 10.1186/1476-4598-12-138
71. Chakraborty PK, Xiong X, Mustafi SB, Saha S, Mukherjee P. Role of Cystathionine Beta Synthase in Lipid Metabolism in Ovarian Cancer. Oncotarget (2015) 6(35):37367–84. doi: 10.18632/oncotarget.5424
72. Ooko E, Saeed MEM, Kadioglu O, Sarvi S, Colak M, Elmasaoudi K, et al. Artemisinin Derivatives Induce Iron-Dependent Cell Death (Ferroptosis) in Tumor Cells. Phytomedicine (2015) 22(11):1045–54. doi: 10.1016/j.phymed.2015.08.002
73. Yu H, Guo P, Xie X, Wang Y, Chen G. Ferroptosis, A New Form of Cell Death, and Its Relationships With Tumourous Diseases. J Cell Mol Med (2017) 21(4):648–57. doi: 10.1111/jcmm.13008
74. Shi ZZ, Tao H, Fan ZW, Song SJ, Bai J. Prognostic and Immunological Role of Key Genes of Ferroptosis in Pan-Cancer. Front Cell Dev Biol (2021) 9:748925. doi: 10.3389/fcell.2021.748925
75. Koppula P, Zhuang L, Gan B. Cystine Transporter SLC7A11/xCT in Cancer: Ferroptosis, Nutrient Dependency, and Cancer Therapy. Protein Cell (2021) 12(8):599–620. doi: 10.1007/s13238-020-00789-5
Keywords: ferroptosis, cancer, iron, programmed cell death, clinical application
Citation: Chen Y, Fan Z, Hu S, Lu C, Xiang Y and Liao S (2022) Ferroptosis: A New Strategy for Cancer Therapy. Front. Oncol. 12:830561. doi: 10.3389/fonc.2022.830561
Received: 07 December 2021; Accepted: 25 January 2022;
Published: 16 February 2022.
Edited by:
Giuseppe Giaccone, Cornell University, United StatesReviewed by:
Michael Wayne Epperly, University of Pittsburgh, United StatesCopyright © 2022 Chen, Fan, Hu, Lu, Xiang and Liao. This is an open-access article distributed under the terms of the Creative Commons Attribution License (CC BY). The use, distribution or reproduction in other forums is permitted, provided the original author(s) and the copyright owner(s) are credited and that the original publication in this journal is cited, in accordance with accepted academic practice. No use, distribution or reproduction is permitted which does not comply with these terms.
*Correspondence: Yu Chen, anVud3VqMzQ1QGdtYWlsLmNvbQ==
†These authors share first authorship
Disclaimer: All claims expressed in this article are solely those of the authors and do not necessarily represent those of their affiliated organizations, or those of the publisher, the editors and the reviewers. Any product that may be evaluated in this article or claim that may be made by its manufacturer is not guaranteed or endorsed by the publisher.
Research integrity at Frontiers
Learn more about the work of our research integrity team to safeguard the quality of each article we publish.