- 1Dermatological Department, Hospital of Chengdu University of Traditional Chinese Medicine, Chengdu, China
- 2School of Clinical Medicine, Chengdu University of Traditional Chinese Medicine, Chengdu, China
- 3Sichuan Evidence-Based Medicine Center of Traditional Chinese Medicine, Hospital of Chengdu University of Traditional Chinese Medicine, Chengdu, China
- 4State Key Laboratory of Southwestern Chinese Medicine Resources, School of Pharmacy, Chengdu University of Traditional Chinese Medicine, Chengdu, China
- 5TCM Regulating Metabolic Diseases Key Laboratory of Sichuan Province, Hospital of Chengdu University of Traditional Chinese Medicine, Chengdu, China
- 6Department of Geriatrics, Hospital of Chengdu University of Traditional Chinese Medicine, Chengdu, China
Background: Immune checkpoint inhibitors (ICIs) have revolutionized metastatic melanoma (MM) treatment in just a few years. Ultraviolet (UV) in sunlight is the most significant environmental cause of melanoma, which is considered to be the main reason for tumor mutation burden (TMB) increase in melanoma. High TMB usually predicts that PD-1 inhibitors are effective. The sunlight exposure pattern of MM might be a clinical feature that matches TMB. The relationship between sunlight exposure patterns and immunotherapy response in MM is unclear. This study aims to investigate the correlation between sunlight exposure patterns and immunotherapy response in MM and establish nomograms that predict 3- and 5-year overall survival (OS) rate.
Methods: We searched the Surveillance, Epidemiology, and End Results (SEER) database and enrolled MM cases from 2005-2016. According to the advent of ICIs in 2011, the era was divided into the non-ICIs era (2005-2010) and the ICIs era (2011-2016). Patients were divided into three cohorts according to the primary site sunlight exposure patterns: head and neck in the first cohort, trunk arms and legs in the second cohort, and acral sites in the third cohort. We compared survival differences for each cohort between the two eras, performed stratified analysis, established nomograms for predicting 3- and 5-year OS rate, and performed internal validation.
Results: Comparing the survival difference between the ICIs and non-ICIs era, head and neck melanoma showed the greatest improvement in survival, with 3- and 5-year OS rate increasing by 10.2% and 9.1%, respectively (P=0.00011). In trunk arms and legs melanoma, the 3- and 5-year OS rate increased by 4.6% and 3.9%, respectively (P<0.0001). There is no improvement in survival in acral melanoma (AM) between the two eras (P=0.78). The receiver operating characteristic (ROC) curve, area under the ROC curve (AUC) and calibration graphs show good discrimination and accuracy of nomograms. Decision curve analysis (DCA) suggests good clinical utility of nomograms.
Conclusions: Based on the classification of sunlight exposure patterns, there is a gradient difference in immunotherapy efficacy for MM. The degree of sunlight exposure is positively correlated with immunotherapy response. The nomograms are sufficiently accurate to predict 3- and 5-year OS rate for MM, allowing for individualized clinical decisions for future clinical work.
1 Introduction
Since ipilimumab was approved by the FDA (U.S. Food and Drug Administration) in 2011, metastatic melanoma (MM) treatments have revolutionized in just a few years (1). Immune checkpoint inhibitors (ICIs) significantly improved overall survival (OS) in MM (2–4). So far, ICIs, such as anti-cytotoxic T lymphocyte-associated protein 4 (anti-CTLA-4) and anti-programmed death protein-1/programmed death-ligand 1 (anti-PD-1/PD-L1), have emerged as the first-line MM treatment regimen.
Ultraviolet (UV) in sunlight is the most significant environmental cause of melanoma (5, 6). The detrimental effects of UV are mainly associated with defective immune surveillance and DNA damage. UV can suppress adaptive immunity and escape tumor immune surveillance by increasing Treg cells and decreasing effector T cells, eventually promoting melanoma development (7). UV irradiation can cause DNA damage, inhibit DNA repair and induce gene mutations (8). Patients with melanoma usually have the strong UV mutation signature (9). C-T conversion in the bipyrimidine moiety is the most common mutation in malignant melanoma cells, mainly caused by UV-induced DNA damage (10). More importantly, UV mutation signatures of primary site are preserved in metastatic sites in MM (11). This suggests that the UV exposure signatures of MM depend on the primary site.
There are significant differences in sunlight exposure between different areas on the human body’s surface. Head and neck generally considered as chronic sunlight exposure areas, trunk arms and legs are intermittent sunlight exposure areas, and acral sites are lesser sunlight exposure areas (12–14). This classification is widely used in clinical practice and showed different immunotherapy responses. Acral melanoma (AM) as a subtype of cutaneous melanoma (CM), has been shown lower ICIs efficacy than other CM (15).
Tumor mutation burden (TMB) has been identified as a promising predictive biomarker for immunotherapy in various cancers (16). High TMB increases the chance that more T cells will recognize neoantigens, leading to clinically relevant better ICIs outcomes (17). MM patients with high TMB have more improvement in OS and progression-free survival (PFS) after receiving ICIs (18, 19). UV exposure is thought to be the main cause of increased TMB in melanoma (20, 21). Dousset et al. measured TMB in chronically sun-exposed areas (face and neck), intermittently sun-exposed areas (trunk arms and legs), and sun-protected areas (mucosal, uveal, foot, toes, etc), found that the values of TMB were 37.2 muts/Mb, 13.6 muts/Mb, and 4 muts/Mb successively, and indicated that TMB was significantly associated with sunlight exposure (20). More importantly, there is a strong concordance between the TMB of primary site and metastatic site cells in MM patients (22). It is consistent with the phenomenon that the UV mutation signature of the primary site is retained at metastasis site. Therefore, we speculated that the sunlight exposure pattern may be a clinical characteristic that matches TMB for MM, causing differences in immunotherapy response.
However, systematic studies on the correlation between sunlight exposure and immunotherapy response in MM are lacking. We hypothesized that immunotherapy efficacy for MM may vary depending on the sunlight exposure pattern at primary site. According to the advent of ICIs in 2011, we divided the era into the non-ICIs era (2005-2010) and the ICIs era (2011-2016). We analyzed immunotherapy efficacy by comparing the survival differences between the two eras and exploring the differences in immunotherapy efficacy between different sunlight exposure pattern cohorts. We also created nomograms to predict 3-and 5-years OS rate in MM patients.
2 Materials and methods
2.1 Data source
The study is based on the Surveillance, Epidemiology, and End Results (SEER) database. The SEER database has collected survival data of about 34.6% from the United States population cancer registry. It is one of the most authoritative sources of tumor information in the United States. According to the exemption regulations released by the SEER database, the use of the data does not require informed patient consent. SEER database does not contain personally-identifying information.
2.2 Patient selection criteria
Research data use agreement was obtained at the SEER program (https://seer.cancer.gov/data). Data was downloaded by using the SEER*Stat software (Version 8.4.0.1) and the SEER-18 registries dataset (November 2019 submission). During 2005-2016, MM patients were selected based on the following criteria: Select melanoma (8720-8799, nevi and melanomas) codes and CM codes in the international classification of diseases for oncology, third edition (ICD-O-3) site/histology. This article only included microscopically confirmed cases, first primary or first only, patients older than or equal to 18 years and surviving more than 0 days. The appropriate codes for MM (stage III and IV) were selected according to Derived AJCC Stage Group 6th Ed (2004-2015), Derived AJCC Stage 7th Ed (2010-2015) and Derived SEER Cmb Stg Grp (2016+).
Data were filtered using the following exclusion criteria: This article excluded primary site ambiguous (“C44.9 Skin, NOS” and “C44.8 Overlapping lesion of skin”), type of reporting source coming from “Autopsy only” and “Death certificate only”, and T0 stage (no evidence of primary tumor).
We collected a large dataset concerning demographic, clinical characteristics and curative treatments. The following variables were evaluated: primary site (head and neck, trunk arms and legs or acral sites); age (18–50 years, 51–70 years or >71 years); sex (male or female); race (white or others); marital status (married or others); T stage (T1, T2, T3 or T4); N stage (N0, N1, N2 or N3); M stage (M0 or M1); ulceration (yes or no); distant organ metastasis (yes or no); surgical margin of primary lesion (narrow, wide or others); local lymph node resection (yes, no or others); metastasectomy (yes or no); radiotherapy (yes or no); chemotherapy (yes or no); vital status (alive or dead); patient ID.
Distant organ metastasis only included bone, brain, liver and lung. Among the surgical margin of primary lesion variables, margins less than or equal to 1 cm were considered narrow margins, and vice versa. Without conventional surgery (such as laser ablation, photodynamic therapy, electrocautery and cryosurgery) and without surgery were defined as others. Among the local lymph node resection variables, needle aspirate biopsy of regional lymph nodes was performed but not known to have been removed was defined as others.
We took 2011 as the dividing line, 2005-2010 as the non-ICIs era, and 2011-2016 as the ICIs era. MM patients were divided into three cohorts based on the sunlight exposure patterns of primary site, patients with primary site of head and neck in the first cohort, trunk arms and legs in the second cohort, and acral sites in the third cohort (Figure 1).
2.3 Propensity score matching
We screened 9806 MM patients, 4264 cases of which were recorded in the non-ICIs era, and 5542 cases were recorded in the ICIs era. Comparing the differences in the variables between the two eras, there were statistically significant differences in surgical margin of primary lesion (P < 0.001, P=0.001) and local lymph node resection in the three cohorts (P=0.026, P < 0.001, P=0.001). The detailed results are shown in Table 1.
Propensity score matching (PSM) analysis was performed to reduce the bias. Except for distant organ metastasis, as it only contains data from the ICIs era. The patients were matched 1:1 by estimated propensity scores with a caliper width of 0.02. After matching, 4069 matched pairs (8138 cases) were included in the analysis (Figure 1), and the variables were almost balanced between the two eras shown in Table 2. This paper uses propensity score matching data for analysis and evaluation.
2.4 Construction and validation of prognostic nomograms
Univariable and multivariable Cox analyses were used to screen independent prognostic factors in the training cohorts at P<0.05 level. Independent prognostic factors as variables were used to create nomograms. Nomograms were used to predict 3- and 5-year OS rate. Evaluate nomograms performance by receiver operating characteristic (ROC) curve, area under the ROC curve (AUC), calibration graph and decision curve analysis (DCA). The discriminative power of nomograms was assessed by using the ROC curve and AUC. The accuracy of nomograms was evaluated by using calibration graph. The ROC curve reflects the specificity and sensitivity of the prediction model (23). The closer the ROC curve is to the upper left corner of the graph, the higher the accuracy of the prediction model (24). Bootstrap resampling (1000 resampling) was used for the calibration graph to determine the calibration capability of nomograms. The 45° line represents perfect calibration, the closer the better. The clinical utility of nomograms was evaluated by DCA. It is a method to determine whether use of the prediction model to inform clinic decision-making would do more good than harm (25). It is used to derive the net benefit of the prediction model across different threshold probabilities (26). Survival rate for high- and low-risk cases were compared by using Kaplan-Meier (KM) survival curves and log-rank test.
2.5 Statistical analysis
All statistical analyses were performed by R studio version 4.1.3. Statistical significance was set at P < 0.05 level. Continuous variables were expressed as mean ± standard deviation, Mann-Whitney U test was used for comparison. χ2 test or Fisher’s exact test was used for categorical variable comparison. 3- and 5-year OS rate were compared by KM survival curves and log-rank test. Univariable and multivariable Cox analyses were used to screen for independent prognostic factors. Nomograms were created by using the rms package in R Studio (http://www.r-project.org/).
3 Results
3.1 Gradient differences in immunotherapy efficacy among three cohorts
Survival was significantly improved in the ICIs era (Figure 2A).In the non-ICIs and ICIs era, there was a significant survival gap between the first (head and neck) and second (trunk, arms and legs) cohorts. Survival rate was consistently higher in the second cohort than in the first. In the non-ICIs era, when survival was longer than 24 months, the second cohort had better survival outcomes than the third cohort (acral sites) (Figure 2B). In the ICIs era, survival rate increased significantly in the first and second cohorts. The survival gap between the third cohort (acral sites) and the other cohorts had been widened. When survival was less than 24 months, the third cohort (acral sites) had the lowest survival rate (Figure 2C).
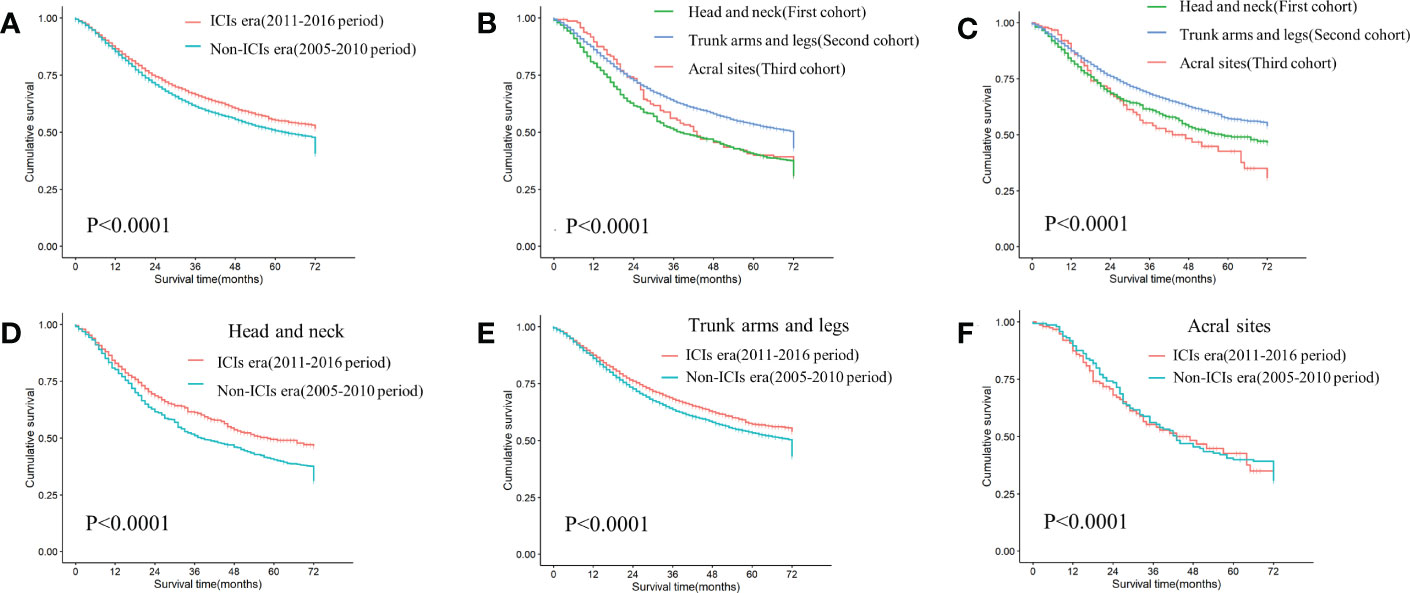
Figure 2 KM curves of differences in survival among cohorts (A) Comparing survival differences between non-ICIs era and ICIs era (P<0.0001). (B) Differences in survival among three cohorts in the non-ICIs era (P<0.0001). (C) Differences in survival among three cohorts in the ICIs era (P<0.0001). (D) Differences in survival between the non-ICIs era and the ICIs era in the first cohort (P=0.00011). (E) Differences in survival between the non-ICIs era and the ICIs era in the second cohort (P<0.0001). (F) Differences in survival between the non-ICIs era and the ICIs era in the third cohort (P=0.78).
There was a graded difference in ICIs efficacy when comparing 3-year and 5-year OS rate in three cohorts between the two eras. The first cohort benefited the most from ICIs (P=0.00011), with 3-year and 5-year OS rate increasing by 10.2% and 9.1%, respectively (Figure 2D). In the second cohort, the 3-year and 5-year OS rate increased by 4.6% and 3.9%, respectively (P<0.0001) (Figure 2E). However, there was no survival difference between the two eras in the third cohort (P=0.78) (Figure 2F).
3.2 Stratified analysis of cohorts in the ICIs era
In all cohorts, patients with N1 stage, M0 stage, and no distant organ metastases showed better survival (Figure 3) (Table 3). In addition, patients with non-acral CM (the first and second cohorts) who had T2 stage, wide surgical margin of primary lesion (>1cm), and local lymph node resection had higher OS rate (Table 3).
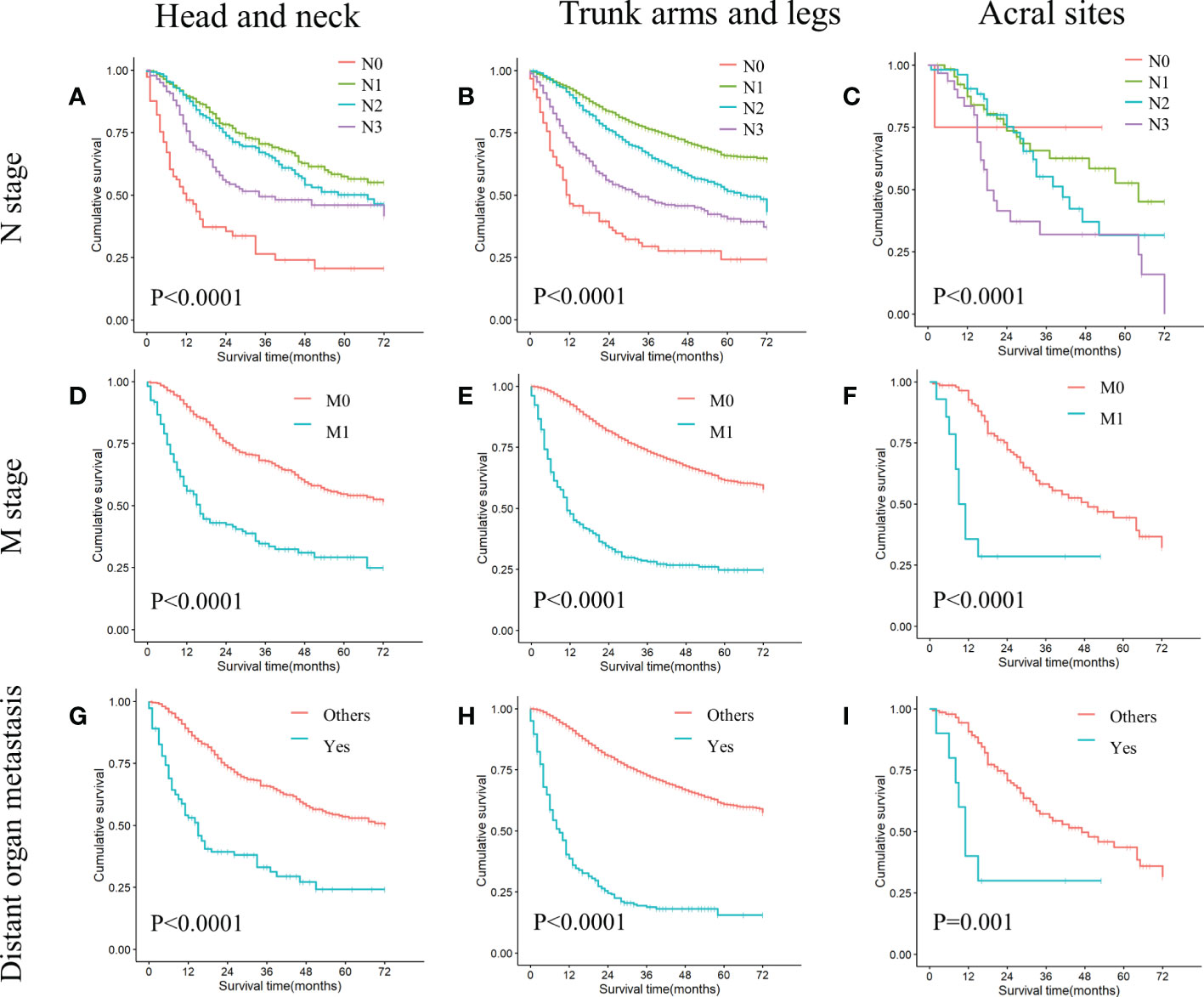
Figure 3 KM curves of stratified analysis in the ICIs era: N stage, M stage, distant organ metastasis. (A) Comparing survival differences in N stage in head and neck melanoma (P<0.0001). (B) Comparing survival differences in N stage in trunk arms and legs melanoma (P<0.0001). (C) Comparing survival differences in N stage in acral melanoma (P<0.0001). (D) Comparing survival differences in M stage in head and neck melanoma (P<0.0001). (E) Comparing survival differences in M stage in trunk arms and legs melanoma (P<0.0001). (F) Comparing survival differences in M stage in acral melanoma (P<0.0001). (G) Comparing survival differences in distant organ metastasis in head and neck melanoma (P<0.0001). (H) Comparing survival differences in distant organ metastasis in trunk arms and legs melanoma (P<0.0001). (I) Comparing survival differences in distant organ metastasis in acral melanoma (P<0.0001).
3.3 Univariable and multivariable cox regression analysis in the ICIs era
In the univariable analysis, M0 stage, no distant organ metastasis, routine surgery at the primary site, no radiotherapy and chemotherapy showed significant positive survival benefits in all cohorts of the ICIs era. Multivariable analyses of the first and second cohorts showed a significant reduction in survival among patients older than 70 years, with ulcers, and without routine surgery at the primary site. Only female showed positive survival in the first (head and neck) and second (trunk, arms and legs) cohorts. Age, sex, ulceration, and surgical margin of primary lesion were independent prognostic factors in non-acral CM (the first and second cohorts). M stage and radiotherapy were independent prognostic factors in AM (the third cohort) (Table 4).
3.4 Prognostic nomograms of metastatic melanoma
We include data from two eras to create nomograms and set the era as a new variable. The ICIs era as yes (assuming to receive ICIs therapy) and the non-ICIs era as no (not receiving ICIs therapy). All used a 2-to-1 ratio to randomly assign cases to the training set for nomogram construction and the validation set used to perform internal validation. We use data from the training set to select variables by univariable and multivariable Cox regression analysis at P<0.05 level (Table 5). Multivariable Cox proportional hazards models were built to create nomograms.
The nomogram of the first cohort is constructed by age, marital status, M stage, ulceration, distant organ metastasis, surgical margin of primary lesion and ICIs therapy (Figure 4A). The AUC of the training set predicting 3- and 5-year OS rate are 0.706 and 0.676 respectively (Figure 5A), and the AUC of the validation set are 0.689 and 0.670, respectively (Figure 5B). The nomogram of the second cohort is constructed based on variables such as age, sex, marital status, T stage, N stage, M stage, ulceration, distant organ metastasis, surgical margin of primary lesion, local lymph node resection, radiotherapy and ICIs therapy (Figure 4B). The AUC of the training set predicting 3- and 5-year OS rate are 0.800 and 0.786 respectively (Figure 5C), and the AUC of the validation set are 0.794 and 0.783, respectively (Figure 5D). The nomogram variables for the third cohort consist of age, race, ulceration and radiotherapy (Figure 4C). The AUC of the training set predicting 3- and 5-year OS rate are 0.680 and 0.729 respectively (Figure 5E), and the AUC of the validation set are 0.627 and 0.590, respectively (Figure 5F).
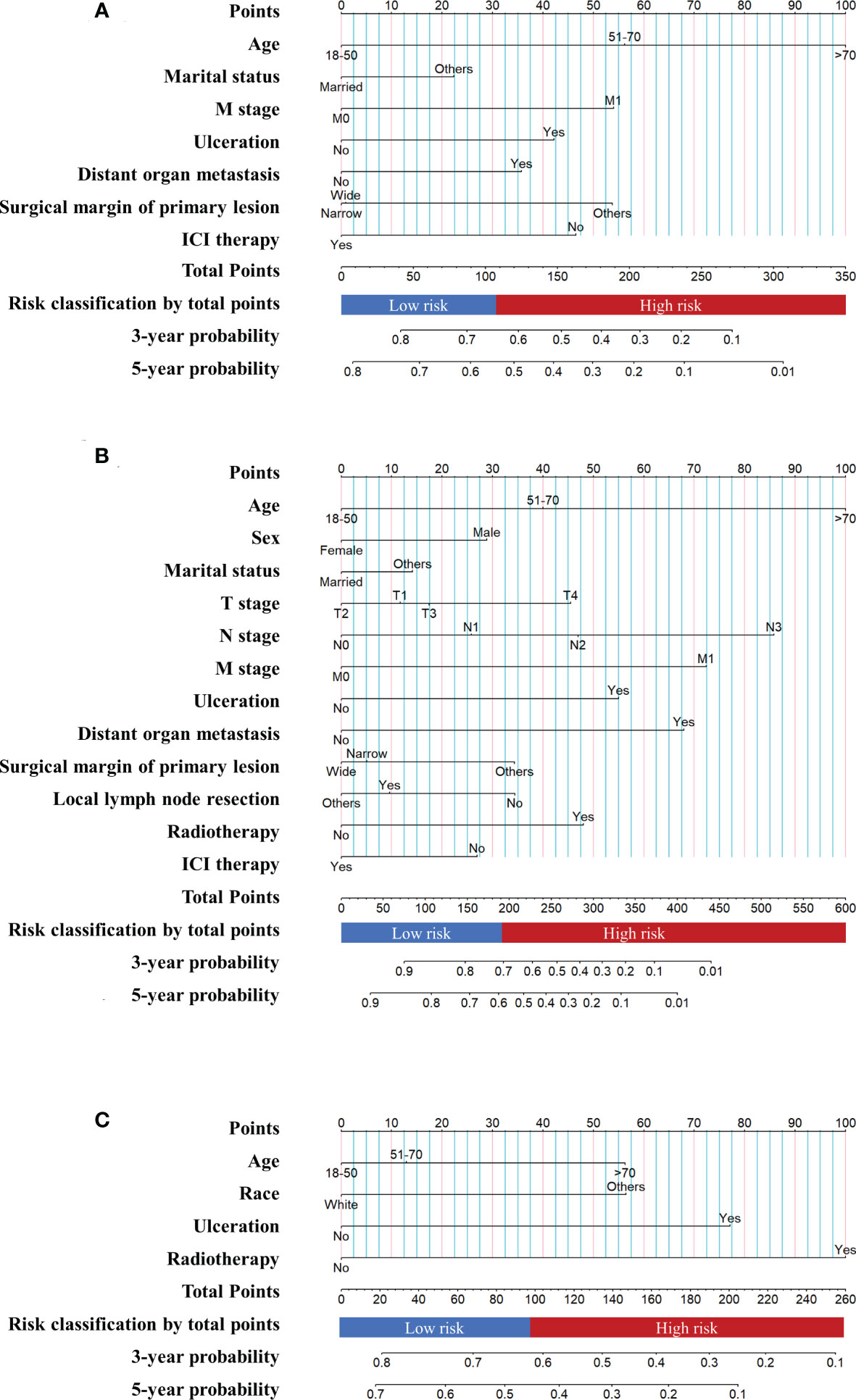
Figure 4 Prognostic nomograms of metastatic melanoma (A) The nomogram of the first cohort. (B) The nomogram of the second cohort. (C) The nomogram of the third cohort.
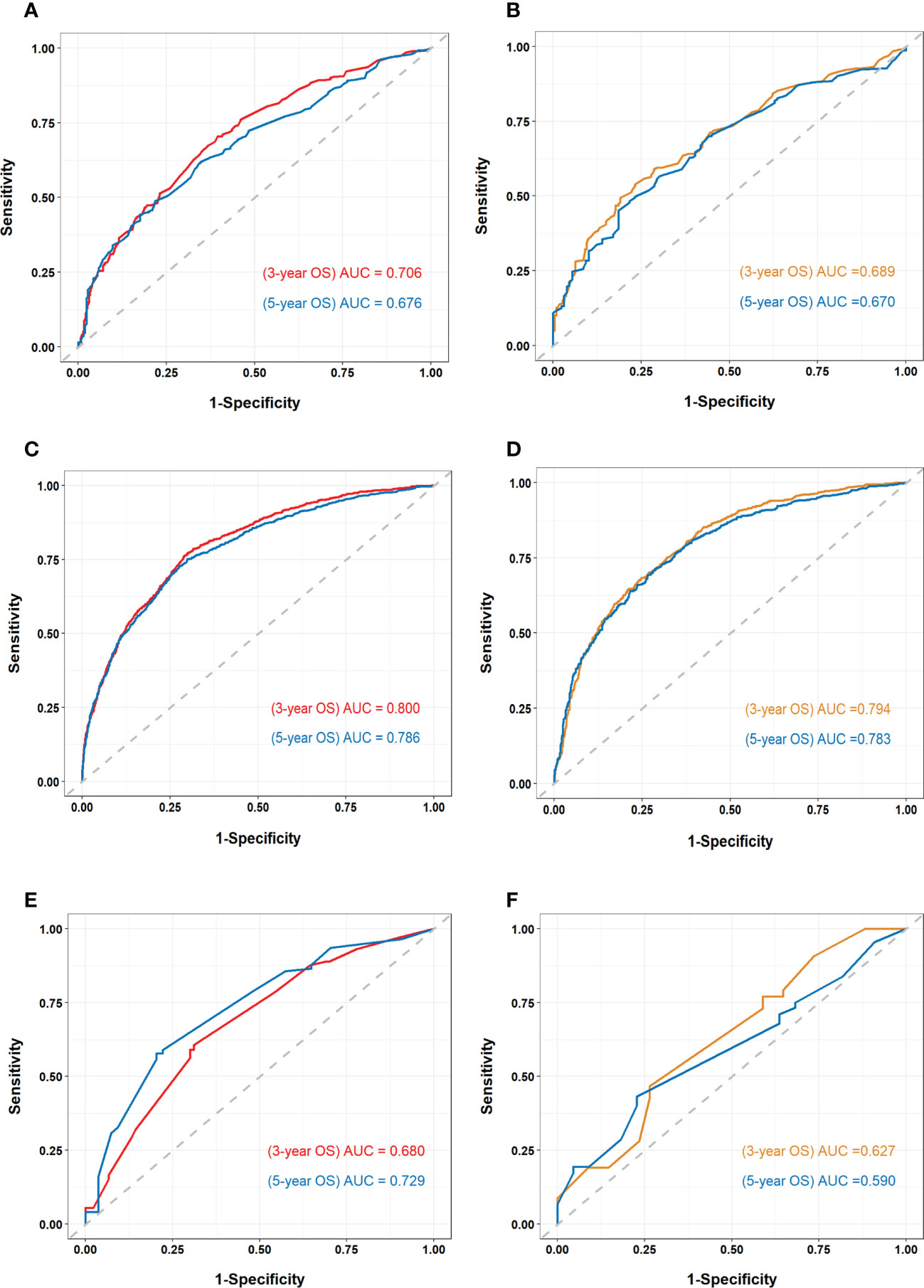
Figure 5 Receiver operating characteristic (ROC) curve and area under the ROC curve (AUC) of all cohorts (A) ROC curve and AUC of the first cohort training set. (B) ROC curve and AUC of the first cohort validation set. (C) ROC curve and AUC of the second cohort training set. (D) ROC curve and AUC of the second cohort validation set. (E) ROC curve and AUC of the third cohort training set. (F) ROC curve and AUC of the third cohort validation set.
There is appropriate consistency between the observed and predicted probabilities of 3- and 5-year OS rate for the three nomograms, and both training and validation set calibration graphs are close to the 45-degree line (Figure 6). The internal validation for the nomograms suggests good predictive consistency. DCA for both the training and validation sets is performed to show the clinical usefulness of the nomogram (Figure 7).
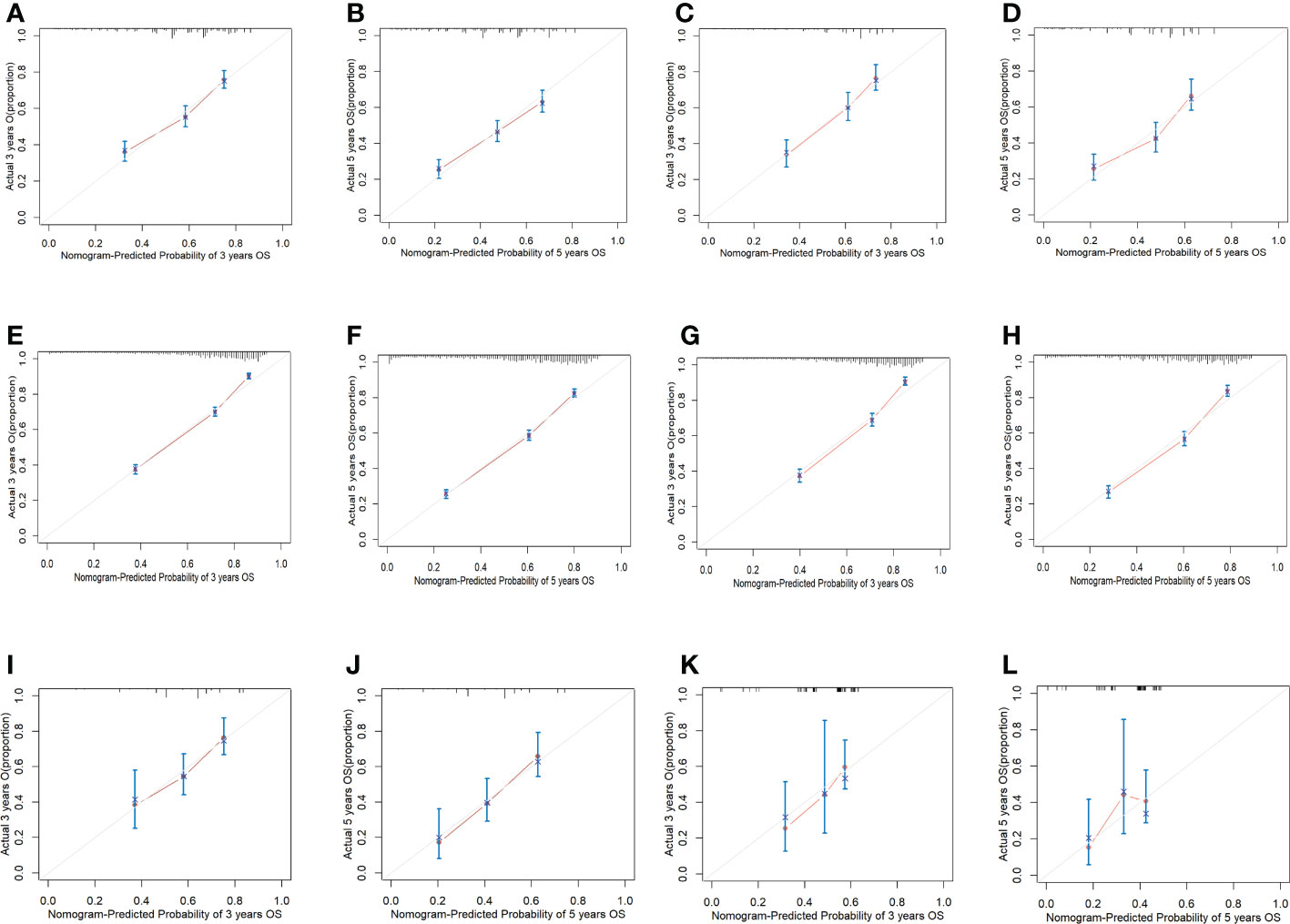
Figure 6 Calibration graphs of all cohorts. (A) Calibration graph of predicting 3-year OS for the first cohort training set. (B) Calibration graph of predicting 5-year OS for the first cohort training set. (C) Calibration graph of predicting 3-year OS for the first cohort validation set. (D) Calibration graph of predicting 5-year OS for the first cohort validation set. (E) Calibration graph of predicting 3-year OS for the second cohort training set. (F) Calibration graph of predicting 5-year OS for the second cohort training set. (G) Calibration graph of predicting 3-year OS for the second cohort validation set. (H) Calibration graph of predicting 5-year OS for the second cohort validation set. (I) Calibration graph of predicting 3-year OS for the third cohort training set. (J) Calibration graph of predicting 5-year OS for the third cohort training set. (K) Calibration graph of predicting 3-year OS for the third cohort validation set. (L) Calibration graph of predicting 5-year OS for the third cohort validation set.
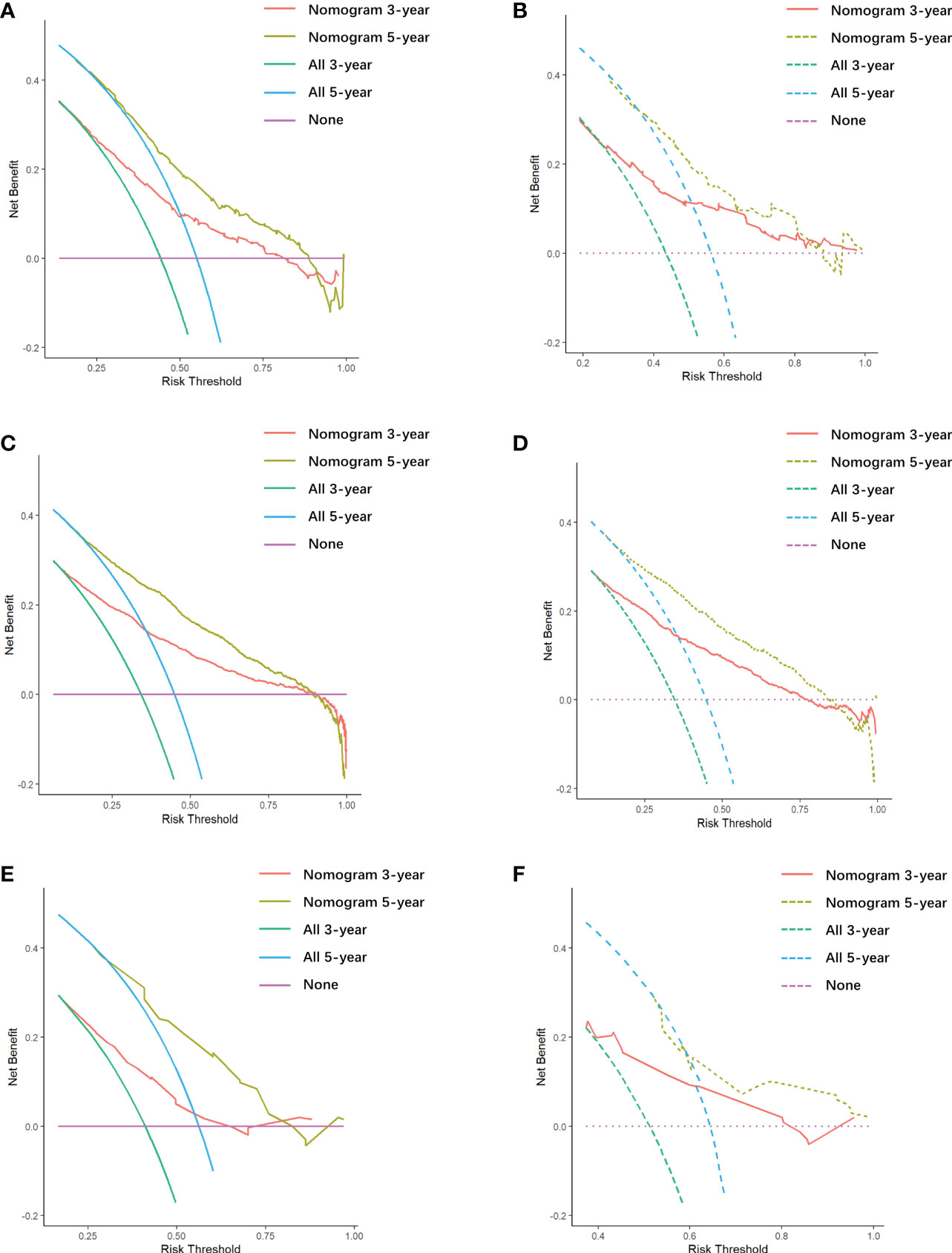
Figure 7 Decision curve analysis (DCA) of all cohorts. (A) DCA of the first cohort training set. (B) DCA of the first cohort validation set. (C) DCA of the second cohort training set. (D) DCA of the second cohort validation set. (E) DCA of the third cohort training set. (F) DCA of the third cohort validation set.
3.5 Risk classification
Each patient is scored according to nomograms and divided into high-risk and low-risk groups. The KM survival curves show that there is a significant difference in survival between high-risk group and low-risk group in the training and validation sets (Figure 8). Nomograms are good at identifying cases with different risks of death.
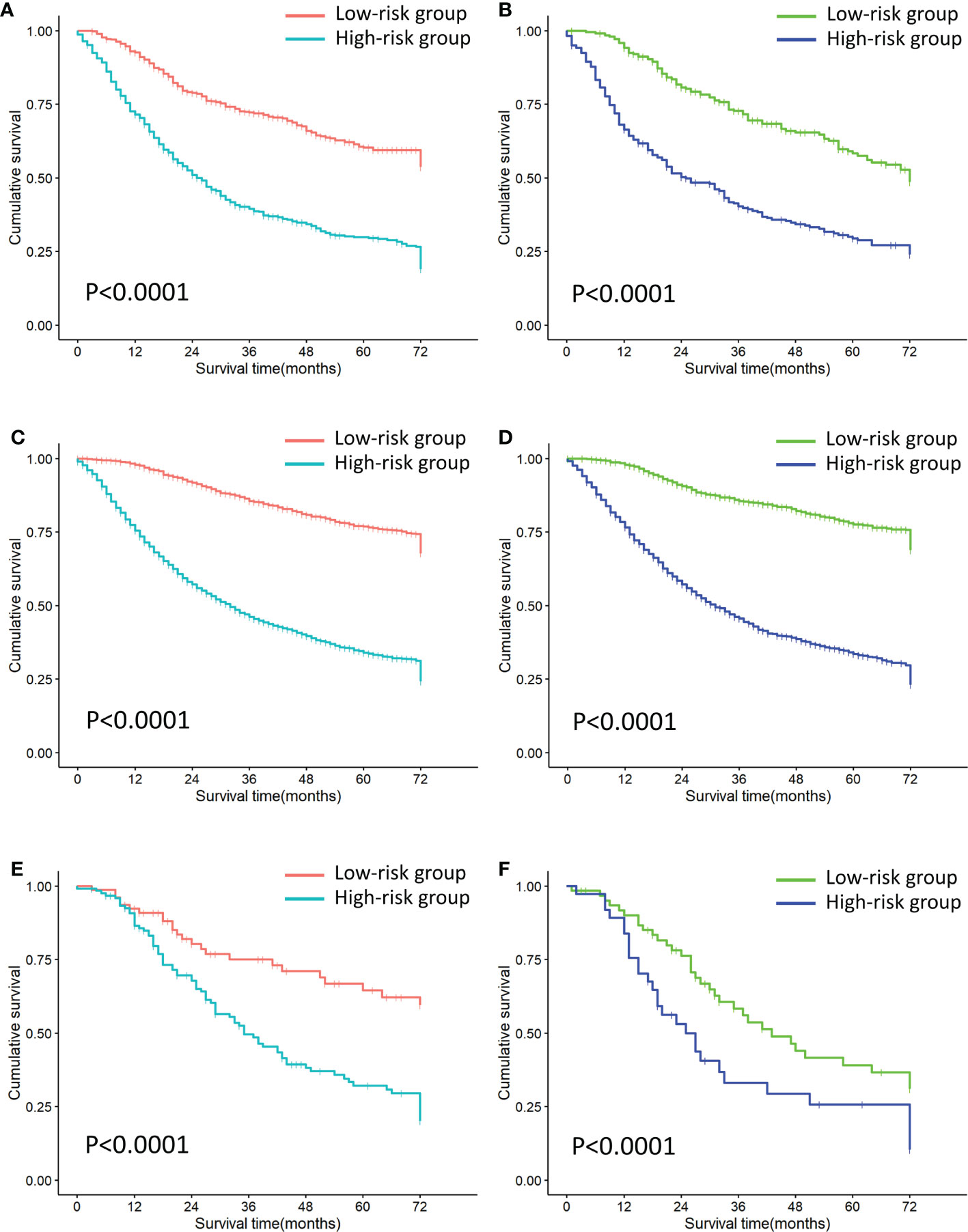
Figure 8 Risk stratification of all cohorts. (A) Risk stratification of the first cohort training set. (B) Risk stratification of the first cohort validation set. (C) Risk stratification of the second cohort training set. (D) Risk stratification of the second cohort validation set. (E) Risk stratification of the third cohort training set. (F) Risk stratification of the third cohort validation set.
4 Discussion
The present study shows that there is a gradient difference in immunotherapy efficacy for MM based on the sunlight exposure patterns. The degree of sunlight exposure is positively correlated with immunotherapy response. Head and neck melanoma has the best curative effect, trunk, arms and legs melanoma is the second and AM is the last. AM does not significantly improve OS in ICIs era. Similar studies have also shown that ICIs is less effective in AM than other CM (27). Lower TMB due to little UV exposure in AM may be the underlying reason (28).
In the present study, during the ICIs era, trunk, arms and legs melanoma has the best prognosis, followed by head and neck melanoma, and AM. However, head and neck melanoma showed the greatest improvement in survival rate and the best ICIs efficacy between the two eras. This contradictory result may be explained by the following reasons: According to epidemiological surveys, head and neck melanoma has a worse prognosis than CM from other body areas (29, 30). Head and neck have rich vascularization and lymphatic drainage, melanoma is prone to metastases and even more likely to develop brain metastases (31, 32). Patients diagnosed MM in the ICIs era may not receive immunotherapy. This may be the reason why survival rate for head and neck melanoma are still lower than other melanoma from a macro-population perspective. However, a relevant study has shown that MM patients with primary site chronically exposed to sunlight (head and neck) had significantly higher OS than other CM after receiving anti-PD-1 (33). The survival disadvantage of head and neck melanoma has been reversed by immunotherapy. But this research did not include patients who did not receive ICIs as a control group. There are still some limitations.
Meanwhile, we took the following measures to ensure the accuracy of the results: Firstly, we used PSM to eliminate the bias caused by other mainstay treatments such as chemotherapy, radiotherapy, and surgery. There is no statistical difference in the frequency of using these treatments between the two eras. Secondly, we try to ensure that the survival difference between the two eras was mainly due to immunotherapy. It is still difficult to eliminate the bias of patients receiving other comorbid treatments, such as hormonal and antibiotics. However, the number of people receiving ICIs therapy is rising every year. According to statistics, the utilization rate of ICIs was 8%-12% of patients from 2004 to 2010, with utilization increasing to 29.7% in 2014, so it is reasonable to assume that immunotherapy is the main reason for the increase of survival rate in ICIs era (34). Lastly, we included patients who did not receive ICIs and objectively compared 3- and 5-year OS rate improvements in two eras.
We observed that female patients with non-acral CM exhibited better survival than male during the ICIs era. It has been widely reported that female diagnosed with CM have a survival advantage compared to male, and this survival advantage still persisted in MM (35–38). The immune system response has sex-related dimorphism which is mainly caused by sex hormones, and female tends to have a more active immune system (39–42). This may explain the female survival advantage. However, we did not find survival difference between male and female in AM. This may indicate an interplay between body sites and sex in terms of survival outcomes. Arce et al. demonstrated that female had better 5-year disease-specific survival (DSS) compared to male patients in cutaneous head and neck melanoma (43). Further research is needed to confirm this hypothesis. Nonetheless, studies have shown that male benefit more from ICIs than female (44–46). Jang et al. showed that female with melanoma have a 2-fold higher mortality risk than their male counterparts in nivolumab plus ipilimumab combination immunotherapy (47). Female mount stronger innate and adaptive immune responses than male on average (48). Tumors in female are more able to evade immune surveillance than male, making tumors less immunogenic and more resistant to immunotherapy (49). The relationship between sex, survival outcome and immunotherapy response is complex and contradictory. The survival gap between male and female MM patients may be narrowed with the widespread use of immunotherapy.
The combination of surgical treatment and ICIs therapy may achieve superior therapeutic effects. We observed that, in non-acral CM, the surgical margin of primary lesion more than 1cm and local lymph node resection combined with immunotherapy show synergistic effects. However, this remains speculative since the number and extent of local lymph node resection are unknown.
Patients showed gradient differences in OS based on sunlight exposure patterns of primary site. Thus, refined categorical management might be needed for MM patients. According to sunlight exposure patterns of primary site, we attempt to create three nomograms to predict 3- and 5-year OS rate. All nomograms showed good discrimination, clinical usability, and risk stratification. This nomogram risk stratification is used to manage patients so that they can receive immunotherapy or combination therapy with different intensities. High-risk patients may consider multidrug immunotherapy, targeted drugs combined with immunotherapy, or immunotherapy combined with radiotherapy and chemotherapy. Lower-intensity treatment options, such as single-agent immunotherapy or single-agent immunotherapy combined with surgery, should be considered in low-risk patients to reduce economical burden and immunotoxicity.
There are still some limitations to our research. We did not directly measure the UV exposure of the skin. Based on previous literature and clinical practice, we divided cohorts according to the amount of sun exposure the skin received, assuming that head and neck were chronically sunlight exposure areas, trunk, arms and legs were intermittent sunlight exposure areas, and acral sites were lesser sunlight exposure areas (12–14). We actually classify cohorts according to sunlight exposure pattern at the anatomical location of the primary tumor. Due to the limitations of the SEER database, we do not have detailed information on the use of ICIs or targeted drugs, comorbidities, and receiving comorbidity-related treatments. The difference in survival between patients in the non-ICIs and ICIs era was used to represent the immunotherapy efficacy. With the development of medical technology, the advancement of non-immunotherapeutic such as surgery, radiotherapy and chemotherapy could also play a more positive role in improving the survival outcome of patients (50).
Nivolumab (a PD-1 checkpoint inhibitor) and ipilimumab (a CTLA-4 checkpoint inhibitor) have complementary activities in MM, compared with ipilimumab monotherapy, two-drug treatment significantly improved the PFS of patients (51, 52). If more information is available on the use of ICIs, we could study the relationship between sunlight exposure patterns in primary site and the efficacy of anti-CTLA-4, anti-PD-1/PD-L1 single- or multiple-agent treatment. This is what we need to study in depth next.
5 Conclusion
In summary, based on the classification of sunlight exposure patterns, there is a gradient difference in immunotherapy efficacy for MM. The degree of sunlight exposure is positively correlated with immunotherapy response. Head and neck melanoma has the best curative effect, trunk, arms and legs melanoma is the second and AM is the last. In the ICIs era, age, sex, ulceration, and surgical margin of primary lesion are independent prognostic factors for non-acral CM. M stage and radiotherapy are independent prognostic factors for AM during the ICIs era. The AUC and calibration graphs of the nomogram perform well. The nomograms are of good clinical utility and are internally validated to provide accurate predictions of 3- and 5- year OS rate.
Data availability statement
The original contributions presented in the study are included in the article/supplementary material. Further inquiries can be directed to the corresponding authors.
Author contributions
ML and WL conceptualized the study. XM, YC and BW contributed to process data. MC and LZ contributed to validation. SZ, AC, and YP contributed to visualization. JG and JZ administered the project. All authors contributed to the article and approved the submitted version.
Funding
This study was supported by the National Natural Science Foundation of China (grant nos. 82074443), and by Xinglin Scholar Research Promotion Project of Chengdu University of Traditional Chinese Medicine (grant nos. QNXZ2020003), and the “Hundred Talents Program” of the Hospital of Chengdu University of Traditional Chinese Medicine (grant nos. 20-B01) and by the Program of Science and Technology Department of Sichuan Province (2021ZYD0089).
Conflict of interest
The authors declare that the research was conducted in the absence of any commercial or financial relationships that could be construed as a potential conflict of interest.
Publisher’s note
All claims expressed in this article are solely those of the authors and do not necessarily represent those of their affiliated organizations, or those of the publisher, the editors and the reviewers. Any product that may be evaluated in this article, or claim that may be made by its manufacturer, is not guaranteed or endorsed by the publisher.
References
1. Su MY, Fisher DE. Immunotherapy in the precision medicine era: Melanoma and beyond. PloS Med (2016) 13(12):e1002196. doi: 10.1371/journal.pmed.1002196
2. Weber JS, D’Angelo SP, Minor D, Hodi FS, Gutzmer R, Neyns B, et al. Nivolumab versus chemotherapy in patients with advanced melanoma who progressed after anti-Ctla-4 treatment (Checkmate 037): A randomised, controlled, open-label, phase 3 trial. Lancet Oncol (2015) 16(4):375–84. doi: 10.1016/s1470-2045(15)70076-8
3. Hodi FS, Chiarion-Sileni V, Gonzalez R, Grob JJ, Rutkowski P, Cowey CL, et al. Nivolumab plus ipilimumab or nivolumab alone versus ipilimumab alone in advanced melanoma (Checkmate 067): 4-year outcomes of a multicentre, randomised, phase 3 trial. Lancet Oncol (2018) 19(11):1480–92. doi: 10.1016/s1470-2045(18)30700-9
4. Weber J, Mandala M, Del Vecchio M, Gogas HJ, Arance AM, Cowey CL, et al. Adjuvant nivolumab versus ipilimumab in resected stage iii or iv melanoma. New Engl J Med (2017) 377(19):1824–35. doi: 10.1056/NEJMoa1709030
5. Leiter U, Garbe C. Epidemiology of melanoma and nonmelanoma skin cancer–the role of sunlight. Adv Exp Med Biol (2008) 624:89–103. doi: 10.1007/978-0-387-77574-6_8
6. Pfeifer GP, You YH, Besaratinia A. Mutations induced by ultraviolet light. Mutat Res (2005) 571(1-2):19–31. doi: 10.1016/j.mrfmmm.2004.06.057
7. Bernard JJ, Gallo RL, Krutmann J. Photoimmunology: How ultraviolet radiation affects the immune system. Nat Rev Immunol (2019) 19(11):688–701. doi: 10.1038/s41577-019-0185-9
8. Yang W. Surviving the sun: Repair and bypass of DNA uv lesions. Protein Sci: Publ Protein Soc (2011) 20(11):1781–9. doi: 10.1002/pro.723
9. Jespersen H, Lindberg MF, Donia M, Söderberg EMV, Andersen R, Keller U, et al. Clinical responses to adoptive T-cell transfer can be modeled in an autologous immune-humanized mouse model. Nat Commun (2017) 8(1):707. doi: 10.1038/s41467-017-00786-z
10. Pleasance ED, Cheetham RK, Stephens PJ, McBride DJ, Humphray SJ, Greenman CD, et al. A comprehensive catalogue of somatic mutations from a human cancer genome. Nature (2010) 463(7278):191–6. doi: 10.1038/nature08658
11. Turajlic S, Furney SJ, Lambros MB, Mitsopoulos C, Kozarewa I, Geyer FC, et al. Whole Genome Sequencing of Matched Primary and Metastatic Acral Melanomas. Genome research (2012) 22(2):196–207. doi: 10.1101/gr.125591.111
12. Whiteman DC, Stickley M, Watt P, Hughes MC, Davis MB, Green AC. Anatomic site, sun exposure, and risk of cutaneous melanoma. J Clin Oncol: Off J Am Soc Clin Oncol (2006) 24(19):3172–7. doi: 10.1200/jco.2006.06.1325
13. Dal H, Boldemann C, Lindelöf B. Does relative melanoma distribution by body site 1960-2004 reflect changes in intermittent exposure and intentional tanning in the Swedish population? Eur J Dermatol: EJD (2007) 17(5):428–34. doi: 10.1684/ejd.2007.0242
14. Bulliard JL. Site-specific risk of cutaneous malignant melanoma and pattern of sun exposure in new Zealand. Int J Cancer (2000) 85(5):627–32. doi: 10.1002/(sici)1097-0215(20000301)85:5<627::aid-ijc5>3.0.co;2-y
15. van Not OJ, de Meza MM, van den Eertwegh AJM, Haanen JB, Blank CU, Aarts MJB, et al. Response to immune checkpoint inhibitors in acral melanoma: A nationwide cohort study. Eur J Cancer (Oxford England: 1990) (2022) 167:70–80. doi: 10.1016/j.ejca.2022.02.026
16. Yarchoan M, Albacker LA, Hopkins AC, Montesion M, Murugesan K, Vithayathil TT, et al. Pd-L1 expression and tumor mutational burden are independent biomarkers in most cancers. JCI Insight (2019) 4(6):2. doi: 10.1172/jci.insight.126908
17. Jardim DL, Goodman A, de Melo Gagliato D, Kurzrock R. The challenges of tumor mutational burden as an immunotherapy biomarker. Cancer Cell (2021) 39(2):154–73. doi: 10.1016/j.ccell.2020.10.001
18. Ning B, Liu Y, Wang M, Li Y, Xu T, Wei Y. The predictive value of tumor mutation burden on clinical efficacy of immune checkpoint inhibitors in melanoma: A systematic review and meta-analysis. Front Pharmacol (2022) 13:748674. doi: 10.3389/fphar.2022.748674
19. Wu Y, Xu J, Du C, Wu Y, Xia D, Lv W, et al. The predictive value of tumor mutation burden on efficacy of immune checkpoint inhibitors in cancers: A systematic review and meta-analysis. Front Oncol (2019) 9:1161. doi: 10.3389/fonc.2019.01161
20. Dousset L, Poizeau F, Robert C, Mansard S, Mortier L, Caumont C, et al. Positive association between location of melanoma, ultraviolet signature, tumor mutational burden, and response to anti-Pd-1 therapy. JCO Precis Oncol (2021) 5:1823. doi: 10.1200/po.21.00084
21. Russo D, Poizeau F, Dinulescu M, Baggio R, Orion C, Soethoudt C, et al. 1133p skin photoaging around the site of occurrence of primary melanoma as a clinical predictive biomarker of response to pd-1 inhibitors. Ann Oncol (2020) 31:S760. doi: 10.1016/j.annonc.2020.08.1256
22. Zou Y, Hu X, Zheng S, Yang A, Li X, Tang H, et al. Discordance of immunotherapy response predictive biomarkers between primary lesions and paired metastases in tumours: A systematic review and meta-analysis. EBioMedicine (2021) 63:103137. doi: 10.1016/j.ebiom.2020.103137
23. Mandrekar JN. Receiver operating characteristic curve in diagnostic test assessment. J Thorac Oncol: Off Publ Int Assoc Study Lung Cancer (2010) 5(9):1315–6. doi: 10.1097/JTO.0b013e3181ec173d
24. Nahm FS. Receiver operating characteristic curve: Overview and practical use for clinicians. Korean J Anesthesiol (2022) 75(1):25–36. doi: 10.4097/kja.21209
25. Vickers AJ, Holland F. Decision curve analysis to evaluate the clinical benefit of prediction models. Spine J: Off J North Am Spine Soc (2021) 21(10):1643–8. doi: 10.1016/j.spinee.2021.02.024
26. Fitzgerald M, Saville BR, Lewis RJ. Decision curve analysis. Jama (2015) 313(4):409–10. doi: 10.1001/jama.2015.37
27. Bhave P, Ahmed T, Lo SN, Shoushtari A, Zaremba A, Versluis JM, et al. Efficacy of anti-Pd-1 and ipilimumab alone or in combination in acral melanoma. J Immunother Cancer (2022) 10(7):8–9. doi: 10.1136/jitc-2022-004668
28. Furney SJ, Turajlic S, Stamp G, Thomas JM, Hayes A, Strauss D, et al. The mutational burden of acral melanoma revealed by whole-genome sequencing and comparative analysis. Pigment Cell Melanoma Res (2014) 27(5):835–8. doi: 10.1111/pcmr.12279
29. Ding Y, Jiang R, Chen Y, Jing J, Yang X, Wu X, et al. Comparing the characteristics and predicting the survival of patients with head and neck melanoma versus body melanoma: A population-based study. BMC Cancer (2021) 21(1):420. doi: 10.1186/s12885-021-08105-y
30. Gillgren P, Månsson-Brahme E, Frisell J, Johansson H, Larsson O, Ringborg U. Epidemiological characteristics of cutaneous malignant melanoma of the head and neck–a population-based study. Acta Oncol (Stockholm Sweden) (1999) 38(8):1069–74. doi: 10.1080/028418699432383
31. Višnjić A, Kovačević P, Veličkov A, Stojanović M, Mladenović S. Head and neck cutaneous melanoma: 5-year survival analysis in a Serbian university center. World J Surg Oncol (2020) 18(1):312. doi: 10.1186/s12957-020-02091-4
32. Huismans AM, Haydu LE, Shannon KF, Quinn MJ, Saw RP, Spillane AJ, et al. Primary melanoma location on the scalp is an important risk factor for brain metastasis: A study of 1,687 patients with cutaneous head and neck melanomas. Ann Surg Oncol (2014) 21(12):3985–91. doi: 10.1245/s10434-014-3829-9
33. Russo D, Dalle S, Dereure O, Mortier L, Dalac S, Dutriaux C, et al. Differential gradients of efficacy of immunotherapy according to the sun-exposure pattern of the site of occurrence of primary melanoma. A Multicenter Prospective Cohort Study (Melbase) (2021) 39(15_suppl):e21545–e. doi: 10.1200/JCO.2021.39.15_suppl.e21545
34. Haque W, Verma V, Butler EB, Teh BS. Racial and socioeconomic disparities in the delivery of immunotherapy for metastatic melanoma in the united states. J Immunother (Hagerstown Md: 1997) (2019) 42(6):228–35. doi: 10.1097/cji.0000000000000264
35. Smith AJ, Lambert PC, Rutherford MJ. Understanding the impact of sex and stage differences on melanoma cancer patient survival: A seer-based study. Br J Cancer (2021) 124(3):671–7. doi: 10.1038/s41416-020-01144-5
36. Joosse A, Collette S, Suciu S, Nijsten T, Lejeune F, Kleeberg UR, et al. Superior outcome of women with stage I/Ii cutaneous melanoma: Pooled analysis of four European organisation for research and treatment of cancer phase iii trials. J Clin Oncol: Off J Am Soc Clin Oncol (2012) 30(18):2240–7. doi: 10.1200/jco.2011.38.0584
37. Buja A, Rugge M, Damiani G, Zorzi M, De Toni C, Vecchiato A, et al. Sex differences in cutaneous melanoma: Incidence, clinicopathological profile, survival, and costs. J Women’s Health (2022) 31(7):1012–9. doi: 10.1089/jwh.2021.0223
38. Joosse A, Collette S, Suciu S, Nijsten T, Patel PM, Keilholz U, et al. Sex is an independent prognostic indicator for survival and Relapse/Progression-free survival in metastasized stage iii to iv melanoma: A pooled analysis of five European organisation for research and treatment of cancer randomized controlled trials. J Clin Oncol: Off J Am Soc Clin Oncol (2013) 31(18):2337–46. doi: 10.1200/jco.2012.44.5031
39. Capone I, Marchetti P, Ascierto PA, Malorni W, Gabriele L. Sexual dimorphism of immune responses: A new perspective in cancer immunotherapy. Front Immunol (2018) 9:552. doi: 10.3389/fimmu.2018.00552
40. Pennell LM, Galligan CL, Fish EN. Sex affects immunity. J Autoimmun (2012) 38(2-3):J282–91. doi: 10.1016/j.jaut.2011.11.013
41. Nosrati A, Wei ML. Sex disparities in melanoma outcomes: The role of biology. Arch Biochem Biophysics (2014) 563:42–50. doi: 10.1016/j.abb.2014.06.018
42. Bellenghi M, Puglisi R, Pontecorvi G, De Feo A, Carè A, Mattia G. Sex and gender disparities in melanoma. Cancers (2020) 12(7):2. doi: 10.3390/cancers12071819
43. Arce PM, Camilon PR, Stokes WA, Nguyen SA, Lentsch EJ. Is sex an independent prognostic factor in cutaneous head and neck melanoma? Laryngoscope (2014) 124(6):1363–7. doi: 10.1002/lary.24439
44. Conforti F, Pala L, Bagnardi V, De Pas T, Martinetti M, Viale G, et al. Cancer immunotherapy efficacy and patients’ sex: A systematic review and meta-analysis. Lancet Oncol (2018) 19(6):737–46. doi: 10.1016/s1470-2045(18)30261-4
45. Wu Y, Ju Q, Jia K, Yu J, Shi H, Wu H, et al. Correlation between sex and efficacy of immune checkpoint inhibitors (Pd-1 and ctla-4 inhibitors). Int J Cancer (2018) 143(1):45–51. doi: 10.1002/ijc.31301
46. Wang S, Cowley LA, Liu XS. Sex differences in cancer immunotherapy efficacy, biomarkers, and therapeutic strategy. Mol (Basel Switzerland) (2019) 24(18):3–5. doi: 10.3390/molecules24183214
47. Jang SR, Nikita N, Banks J, Keith SW, Johnson JM, Wilson M, et al. Association between sex and immune checkpoint inhibitor outcomes for patients with melanoma. JAMA Netw Open (2021) 4(12):e2136823. doi: 10.1001/jamanetworkopen.2021.36823
48. Klein SL, Flanagan KL. Sex differences in immune responses. Nat Rev Immunol (2016) 16(10):626–38. doi: 10.1038/nri.2016.90
49. Schreiber RD, Old LJ, Smyth MJ. Cancer immunoediting: Integrating immunity’s roles in cancer suppression and promotion. Sci (New York NY) (2011) 331(6024):1565–70. doi: 10.1126/science.1203486
50. Eddy K, Shah R, Chen S. Decoding melanoma development and progression: Identification of therapeutic vulnerabilities. Front Oncol (2020) 10:626129. doi: 10.3389/fonc.2020.626129
51. Larkin J, Chiarion-Sileni V, Gonzalez R, Grob JJ, Cowey CL, Lao CD, et al. Combined nivolumab and ipilimumab or monotherapy in untreated melanoma. New Engl J Med (2015) 373(1):23–34. doi: 10.1056/NEJMoa1504030
52. Zimmer L, Livingstone E, Hassel JC, Fluck M, Eigentler T, Loquai C, et al. Adjuvant nivolumab plus ipilimumab or nivolumab monotherapy versus placebo in patients with resected stage iv melanoma with no evidence of disease (Immuned): A randomised, double-blind, placebo-controlled, phase 2 trial. Lancet (London England) (2020) 395(10236):1558–68. doi: 10.1016/s0140-6736(20)30417-7
Keywords: metastatic melanoma, sunlight exposure patterns, SEER database, immune-checkpoint inhibitor (ICIs), epidemiology
Citation: Liu M, Li W, Ma X, Che Y, Wei B, Chen M, Zhong L, Zhao S, Chen A, Pang Y, Zeng J and Guo J (2023) Gradient differences of immunotherapy efficacy in metastatic melanoma related to sunlight exposure pattern: A population-based study. Front. Oncol. 12:1086664. doi: 10.3389/fonc.2022.1086664
Received: 01 November 2022; Accepted: 12 December 2022;
Published: 05 January 2023.
Edited by:
Suzie Chen, Rutgers, The State University of New Jersey, United StatesReviewed by:
John August D’Orazio, University of Kentucky, United StatesArup Kumar Indra, Oregon State University, United States
Copyright © 2023 Liu, Li, Ma, Che, Wei, Chen, Zhong, Zhao, Chen, Pang, Zeng and Guo. This is an open-access article distributed under the terms of the Creative Commons Attribution License (CC BY). The use, distribution or reproduction in other forums is permitted, provided the original author(s) and the copyright owner(s) are credited and that the original publication in this journal is cited, in accordance with accepted academic practice. No use, distribution or reproduction is permitted which does not comply with these terms.
*Correspondence: Jinhao Zeng, emVuZ2ppbmhhb0BjZHV0Y20uZWR1LmNu; Jing Guo, Z3VvamluZzY2QGNkdXRjbS5lZHUuY24=
†These authors have contributed equally to this work and share first authorship