- 1The Third Affiliated Hospital of Soochow University, Changzhou, China
- 2Jiangsu University of Technology, Changzhou, China
- 3Changzhou Maternity and Child Health Care Hospital Affiliated to Nanjing Medical University, Changzhou, China
Although some advances have been made in the treatment of osteosarcoma in recent years, surgical resection remains the mainstream treatment. Initial and early diagnosis of osteosarcoma could be very difficult to achieve due to the insufficient sensitivity for the means of examination. The distal metastasis of osteosarcoma also predicts the poor prognosis of osteosarcoma. In order to solve this series of problems, people begin to discover a new method of diagnosing and treating osteosarcoma. Ubiquitination, as an emerging posttranslational modification, has been shown to be closely related to osteosarcoma in studies over the past decades. In general, this review describes the cellular functions and molecular mechanisms of ubiquitination during the development of osteosarcoma.
1. Osteosarcoma
In 1804, John Abernathy, a British surgeon, first proposed the concept of “sarcoma” (1). In 1805, French surgeon Alexis Boyer first used osteosarcoma to describe related types of disease. Boyer believed that osteosarcoma is a novel type of bone tumor that differs from other types of bone tumors (2). Osteosarcoma, a malignant aggressive tumor arising from the mesenchyme, accounts for 20% of all primary bone tumors and is characterized by the formation of immature osteoid tissue (3, 4). Osteosarcoma occurs in the metaphysis of long bones and higher rates are reported among male than female youth, with a male-to-female ratio close to 1.4:1 (5, 6). Patients usually present initially with local pain and limitation of movement at the lesion site and distal metastases in the late stage. The most metastatic organ is the lung (7). Imaging changes in osteosarcoma usually have patchy calcifications. The classic imaging changes are called Codman triangle and sunburst appearance (8).
The treatment of osteosarcoma is based on surgical treatment and supplemented by chemotherapy. Surgical treatment requires complete resection of the patient ‘s tumor site (amputation or limb salvage surgery) (9). Surgeons should record the size of the tumor in the resected bone at the time of the resection of the primary tumor and judge whether it is thorough according to pathological section (10). Chemotherapy includes preoperative chemotherapy and postoperative chemotherapy, but the effect of postoperative chemotherapy is not satisfactory, and whether postoperative chemotherapy can significantly prolong the survival period of patients remains questionable (11). Some of the most effective drugs for treating osteosarcoma include cidplatin, doxorubicin, methotrexate, and ifosfamide (see Table 1). The effect of radiation therapy is generally considered negligible for osteosarcoma (12). The standard treatment is to use neoadjuvant chemotherapy (chemotherapy before surgery) followed by surgical resection (9). The prognosis of osteosarcoma is usually dismal and important for patients to keep lifelong follow-up monitoring because distant metastatic osteosarcoma usually occurs within about a decade of diagnosis (13, 14).
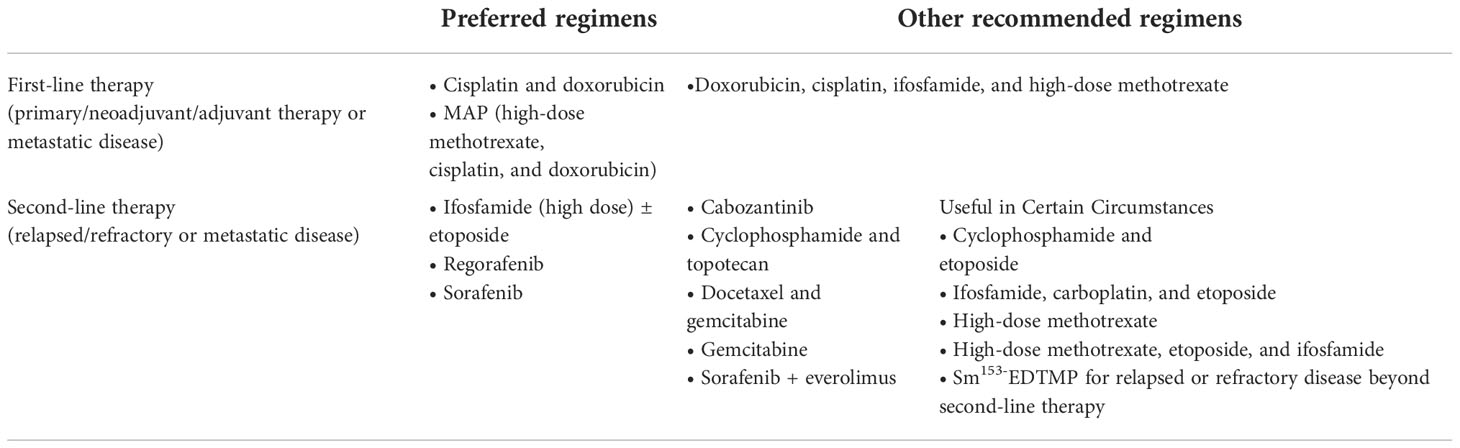
Table 1 NCCN Guidelines Version 2.2023 about Osteosarcoma (www.nccn.org/patients).
2. Ubiquitin
2.1. Ubiquitination
Gideon Goldstein first discovered 74 highly conserved amino acid sequences in cattle and humans since 1975. Gideon Goldstein defined them as ubiquitin (Ub) (15). As delving into study of ubiquitination, key roles for the ubiquitination has been reported in some cancers such as lung cancer, pancreatic cancer and so on (16, 17). Jin et al. summarized the role of ubiquitination in lung cancer such as tumor initiation, metabolism and survival (16). Chen et al. found that UBR5, an E3 ubiquitin ligase, was significantly upregulated in pancreatic cancer. UBR5-induced aerobic glycolysis is dependent on the ubiquitination of fructose-1,6-bisphosphatase (FBP1) in pancreatic cancer cells. These results provide the role of UBR5 in pancreatic cancer cell adaptation to metabolic stresses (17). Ubiquitin, a regulatory protein of 8.6 kDa, is widely distributed in eukaryotes (15). UBB, UBC, UBA52, and RPS27A are recognized as the genes that encode ubiquitin in the human body (18). Ubiquitination refers to the process by which ubiquitin molecules recognize and specifically modify intracellular target proteins under a cascade of the actions of ubiquitin-related enzymes. This process is accomplished by ubiquitin-activating enzymes (E1s), ubiquitin-conjugating enzymes (E2s), and ubiquitin ligases (E3s). The specific process is characterized by the first activation of Ub by E1 ubiquitin-activating enzyme, and Mg2+ -ATP of E1 ubiquitin-activating enzyme catalyzes the formation of covalent thioesters between cysteine on E1 ubiquitin-activating enzyme and the diglycine sequence at the C-terminus in Ub. Afterwards, E1 transfers Ub to the catalytic cysteine of E2 via a diglycine motif, forming an E2-Ub thioester complex. Then E3 binds the substrate with the E2- Ub complex. The ϵ-amino group or free N-terminal amino group of the lysine side chain of the C-terminal carboxy-linked substrate of Ub forms peptide bonds (see Figure 1). Successive rounds of E3 catalytic reactions can produce substrates with polyubiquitin chains that achieve labeling of the substrate through the seven lysine residues of Ub (Lys6, Lys11, Lys27, Lys29, Lys33, Lys48, and Lys63) or the N-terminal methionine of Ub (see Figure 2). Different lysine residues or methionine activate different downstream signals. For example, Lys48 activates the 26S proteasome to degrade the labeled substrate and Lys63 guides the substrate to the endocytic pathway and regulates kinase activation in the NF-κB pathway (19, 20). Recent studies reveal that K63-linked ubiquitination of IKKγ (also known as NEMO) is also critical for IκB kinase (IKK) activation. Degradation of IκB releases NF-κB from heterdimerizing with IκB and facilitates NF-κB nuclear translocation and activation (21). The family members of E1s and E2s are relatively simple. E1s are UBA1, UBA4, UBA5, UBA6, UBA7, ATG7 (22). 40 members have been described and found to be the members of E2s family. All E2s contain a highly conserved domain, termed the UBC domain, and some of the E2s have an additional N- and/or C- terminal domain. Therefore, it has been divided into four major classes. Table 2 shows E2s with their specific domain. With nearly 600 members, the E3 ubiquitin ligases family is the most studied today, specifically divided into three families: HECT, RING-finger, and RBR domain (23–26).
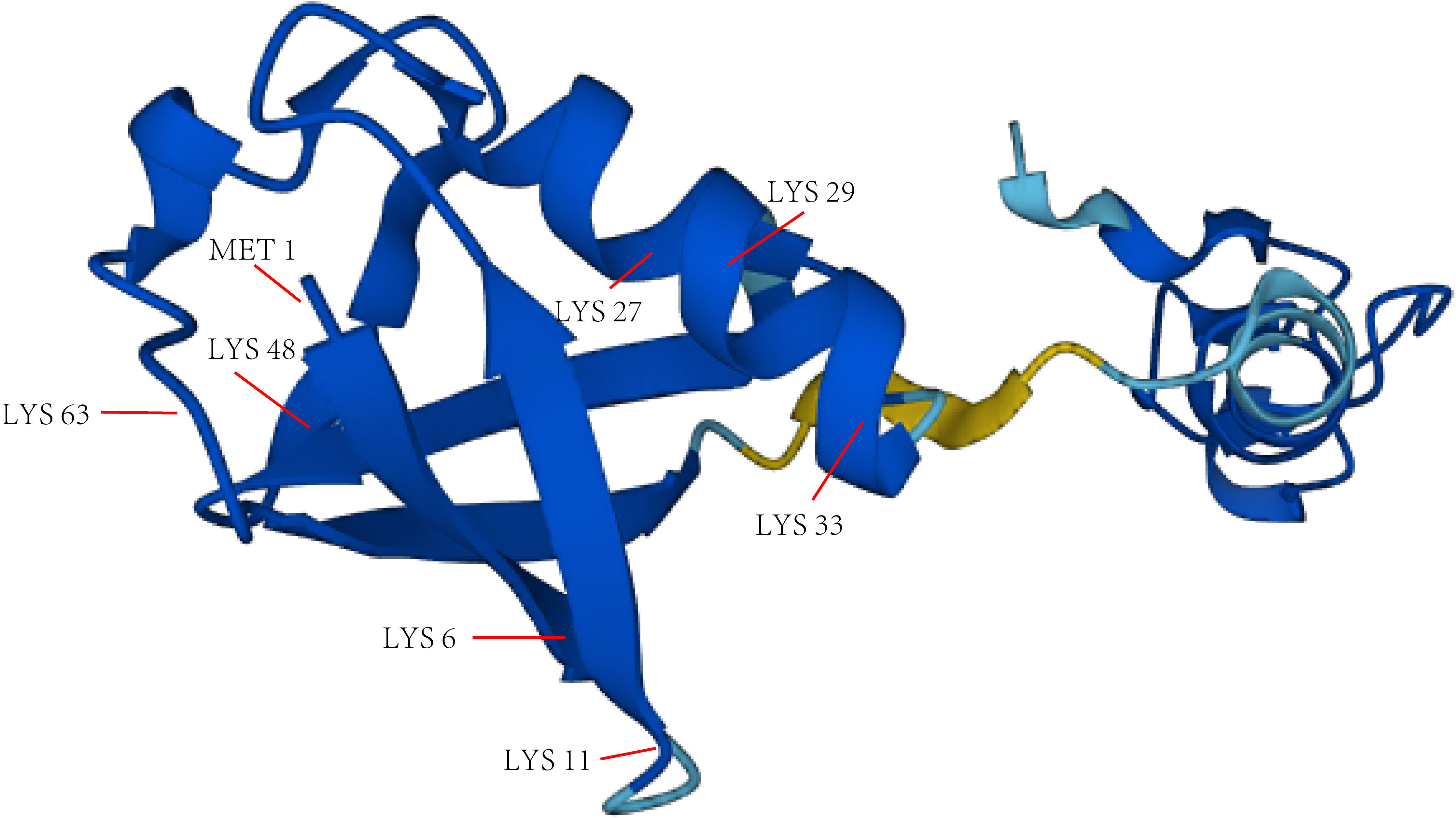
Figure 2 The main lysine residues and N-terminal methionine of Ub. The structure picture of Ub is from alphafold.ebi.ac.uk.
Ubiquitination is widely involved in intracellular substance transport, autophagy, DNA repair, and protein-protein interactions (27, 28). It is generally accepted that there are two types of ubiquitination, monoubiquitination and polyubiquitylation. The monoubiquitination process involves adding a ubiquitin protein to a substrate protein (29, 30), while polyubiquitination is the process of covalent bonding between multiple ubiquitin molecules and different amino acid residues (29, 31), and protein monoubiquitination can have an effect on the polyubiquitination of this protein (29). The HECT-type E3 ligase NEDD4-1 promotes monoubiquitination and polyubiquitylation of PTEN, which allows PTEN to enter the nucleus to prevent its degradation, while polyubiquitylation leads to PTEN degradation in the cytoplasm (32). The mechanisms that determine whether proteins undergo monoubiquitination or polyubiquitylation are unknown. There are currently two views, one that suggests that compatibility between amino acid residues in the E2 catalytic region and those in the substrate an Ub together determine ubiquitin type (33). For example, the E2-E3 complex Cdc34-SCF Cdc4 in yeast is more affinity for K53 residues for six residues at the N-terminus in substrate Sic1. Substitution of amino acid residues around K53 residues for poorly affinity K32 or K84 residues, on the other hand, decreases the affinity of Cdc34-SCF Cdc4 for K53 residues. Whereas mutants in the E2 catalytic center Cdc34 similarly alter the affinity for the K53 residue. These factors together influence whether ubiquitin complexes monoubiquitinate or polyubiquitinate substrates (34). Another view is that monoubiquitination leads to structural changes in the substrate that limit polyubiquitylation of the substrate. For example, the RING family E3 ligase Parkin blocks other ubiquitin-binding domains of Eps15 following its monoubiquitination, thereby limiting elongation of ubiquitin chain (35).
2.2. Deubiquitination
Deubiquitylation is usually mediated by deubiquitinase and is roughly characterized by the separation of ubiquitin from ubiquitinated proteins in the presence of deubiquitinase, and in turn reverses ubiquitination (36). For example, A20 causes K48-linked proteasomal degradation instead of K63-linked polyubiquitination of receptor-interacting serine-threonine kinase 1 (RIPK1) (37). More than 100 deubiquitinases have been identified and can be divided into seven subcategories, and the largest of which is ubiquitin-specific proteases (USP). Others are the ubiquitin C-terminal hydrolases (UCHs), the ovarian tumor proteases (OTUs), the Machado-Josephin domain proteases (MJDs), the JAB1/MPN+/MOV34 (JAMM) domain proteases (JAMMs), the monocyte chemotactic protein-induced proteins (MCPIPs), and the novel motif interacting with ubiquitin-containing DUB family (MINDY) (36, 38). Recently, some scholars have proposed new subclasses including Zn-finger and UFSP domain proteins (ZUFSPs) (36).
2.3. The function of ubiquitination/deubiquitination in cancer
Current evidence suggests that ubiquitination/deubiquitination impacts cancer development in a number of ways. The TGF-β pathway is considered to be an important signal pathway in the development process of cancer, preventing cancer from development by inhibiting cell proliferation and promoting apoptosis in the early stage, and promoting the growth of tumors by stimulating epithelial-mesenchymal transition, distant metastasis of tumors, and evading the immune system in the late stage (39, 40). USP4 and USP15 have been shown to play an important role in the process of tumor metastasis. USP4 regulates breast cancer metastasis through the Relaxin/TGF-β1/Smad2/MMP-9 pathway (41). USP15 can bind SMURF2 to deubiquitinate the TGF-β type I receptor and thus affect tumor development (42). NF-κB pathway is generally thought to suppress apoptosis and promote inflammatory responses which significantly regulate the development of cancer (43, 44). As an important deubiquitinating enzyme, A20 regulates the NF-κB pathway through the OUT domain and zinc finger domain (45). In addition, deubiquitinating enzymes such as USP4 and USP21 can also regulate the NF-κB pathway (46, 47). As a well-known tumor suppressor gene that regulate cell cycle and apoptosis, P53 deletion leads to enhanced glycolysis and maintenance of redox homeostasis in tumor cells (48). The MDM2/MDMX complex is an important E3 ubiquitin ligase and negative regulator of P53 which reduces P53 expression and promotes degradation of P53 during tumor development (49). USP7 is thought to inhibit the ubiquitination process of MDM2 and MDM4, and inhibition of USP7 expression induces apoptosis and leads to cell cycle arrest in tumors (50, 51). Therefore, USP7 may be a useful therapeutic target. In addition, several other deubiquitinating enzymes like USP2a, USP4, USP5, USP9X, USP10, USP11, USP15, USP24, USP29, and USP49 have also been shown to be associated with P53 (52). Moreover ubiquitination/deubiquitination also regulates tumor development in some other pathways. Previous study has indicated that USP9X can regulate tumor development through the Wnt signaling pathway by regulating the expression of DVL2 (53). USP7 can then affect hepatocarcinogenesis by regulating the PI3K-AKT pathway (54).
3. Ubiquitination/deubiquitination in osteosarcoma
3.1. E2 ubiquitin conjugating enzymes
E2 ubiquitin-conjugating enzymes have not been extensively studied in osteosarcoma. Chen et al. found that the E2 ubiquitin-conjugating enzyme UBE2O decreased Bmal1 expression, and UBE2O knockdown enhanced the amplitude of the U2OS circadian clock in osteosarcoma cells (55). UBE2T, a member of the E2 ubiquitin-conjugating enzyme family, was significantly highly expressed in osteosarcoma. Wang et al. showed that UBE2T knockdown could inhibit the PI3K/Akt signaling pathway to exert its inhibitory effect on osteosarcoma development (56).
3.2. E3 ubiquitin ligases
3.2.1. RING E3 ubiquitin ligases
In previous studies, it was found that two proteins in the MDM family, MDM2 and MDMX, can either individually regulate the P53 gene or synergistically inhibit the expression of the P53 gene (57). The Ring domain of MDM2 exhibits E3 ubiquitin ligase activity, whereas the Ring domain of MDMX does not (58). As a result of their interactions with P53, both proteins are known to influence osteosarcoma development (58). Long noncoding RNAs (lncRNAs) have been shown to play critical regulatory roles in the proliferation, differentiation, and apoptosis of osteosarcoma cells (59). Guan et al. found that lncRNA PCAT6 promoted proliferation, migration, and invasion of osteosarcoma by increasing MDM2 expression (60).
As a member of the E3 ubiquitin ligase family, the Skp1-cullin-F-box (SCF) complex is able to participate in both substrate recognition, ubiquitination recruitment, and degradation in the ubiquitin proteasome system. F-box family members belong to a critical subunit of the SCF complex, and F-box39 is aberrantly expressed in many tumors (61), Inhibition of F-box39 expression in U2OS cells has been found to inhibit tumor metastasis and promote tumor cell apoptosis (62).
TRIM family members are E3 ubiquitin ligases whose structure contains a RING loop, one or two B-Box and coiled-coil (RBCC). Among them, the RING finger region has E3 ubiquitin ligase activity and can specifically bind to E2 ubiquitin conjugating enzyme in order to regulate different substrates (63). The TRIM family has been extensively studied in osteosarcoma. Wang et al. demonstrated that TRIM11 expression was significantly upregulated in osteosarcoma cells. TRIM11 was able to ubiquitinate DUSP6, regulate the ERK1/2 pathway and promote osteosarcoma growth (64). Jiang et al. demonstrated that TRIM46 expression was upregulated in osteosarcoma by interacting with PPARα and promoting its ubiquitination. Meanwhile, TRIM46 regulated the NF-κB signaling pathway to promote osteosarcoma cell growth and inhibit their apoptosis (65). Yuan et al. found that TRIM58 expression was markedly downregulated in osteosarcoma cells and co-acted with PKM2 to inhibit glucose consumption and lactate secretion in order to inhibit osteosarcoma development (66). Zhou et al. found that TRIM7 expression was up-regulated in osteosarcoma and could also bind to BRMS1 and promote its ubiquitination, resulting in enhanced migration and invasion of osteosarcoma cells and drug resistance activity, especially against MTX (67).
CRL (Cullin-RING E3 ubiquitin ligase) is the largest E3 ligase family in eukaryotes, and the human cullin family is mainly composed of eight closely related proteins (CUL1, CUL2, CUL3, CUL4A, CUL4B, CUL5, CUL7, and CUL9) (68). CUL4 usually complexes with E3 ubiquitin ligases composed of RBX1, DDB1, and DCAF, and CRL4 all share a similar core structure, with E3 ligase activity determined by CUL4-RBX1 and substrate specificity controlled by DCAF, and the CUL4 subfamily includes two members, CUL4A and CUL4B (69). Chen et al. demonstrated that CRL4B expression was overexpressed in osteosarcoma and could ubiquitinate p21. CRL4B knockdown can arrest the osteosarcoma cell cycle in S phase and attenuate cell proliferation (70). TNF as a well-known inflammatory cytokine can induce activation of the NF-κB pathway (71). Activation of the TNF-α/NF-κB axis enhanced E3 ubiquitin ligase CRL4BDCAF11 activity and modulated cell cycle progression in human osteosarcoma cells (72). Recent studies have identified a subset of microRNAs (miRNAs/miRs) that regulate osteosarcoma development and may serve as molecular drug targets (73, 74). MiR-300 regulates PTEN ubiquitination through the E3 ligase CRL4BDCAF13 to influence osteosarcoma development (75). As an artificially selected biological small molecule, TSC01131 showed toxicity against tumor cells and inhibited the growth of yeast cells and osteosarcoma cells (75). Chen et al. showed that TSC01131 inhibited osteosarcoma cell growth by reducing substrate ubiquitination by the E3 ubiquitinase CRL4B (76).
In addition to the ubiquitin enzymes in these families regulating the process of osteosarcoma, some ubiquitin enzymes are still closely related to osteosarcoma progression. RLIM, encoding an E3 ligase, has been found to ubiquitinate STMN1 in osteosarcoma in order to decrease STMN1 expression. However, compared with normal cells, G2/M phase processes were significantly increased in MG-63 cells overexpressing RLIM and Saos-2 cells (77). Chen et al. found that the E3 ubiquitin ligase SPOP was down-regulated in osteosarcoma and could inhibit osteosarcoma invasion through the PI3K/AKT/NF-κB signaling pathway (78). The E3 ubiquitin ligase c-Cbl can inhibit tumor cell growth by inhibiting targeted receptor tyrosine kinases (RTKs) and inhibit lung metastasis of osteosarcoma (79). DTX1, as a RING domain-containing E3 ubiquitin ligase, is able to cooperate with HES1 to regulate NOTCH signaling pathway to inhibit osteosarcoma invasion (80). Li et al. found that the E3 ligase TRAIP was highly expressed in osteosarcoma and could polyubiquitinate KANK1 to activate the IGFBP3/AKT pathway and promote proliferation of osteosarcoma cells (81). Geranylgeranylacetone (GGA) as an oral antiulcer agent that acts as an inducer of heat shock protein 70 (Hsp70) (82), has been shown to promote apoptosis in human osteosarcoma cells by inducing PRMT1 degradation via the E3 ubiquitin ligase CHIP (83). Cdc20 is generally considered an activator of the E3 ubiquitin ligase APC/C (84). Studies have demonstrated that acpin (85), an inhibitor of Cdc20, is thought to inhibit proliferation and metastasis of osteosarcoma by inhibiting APC/C activation by Cdc20 (86).
3.2.2. HECT E3 ubiquitin ligases
SMURF1 and SMURF2 are HECT-type E3 ubiquitin ligases, which have been found to regulate SMAD family protein stability in the TGF-β/BMP signaling pathway (87). Uev1A is a member of the UEV family of E2 ubiquitin-conjugating enzyme variants, which lack active Cys residues with ubiquitination (88). Zhang et al. found that Uev1A could promote smad1 ubiquitination by SMURF1 to promote cell differentiation of osteosarcoma (89). Huang et al. found that SMURF2 bound the E3 ubiquitin ligase RLIM and regulated the TGF-β pathway to promote metastasis in osteosarcoma (90).
HACE1, a HECT E3s family member (91), reduces ROS levels in vitro and in vivo by blocking NADPH oxidase-mediated superoxide generation (92). It has been found that the HACE1 expression is decreased in osteosarcoma and inhibits tumor development by ubiquitinating RAC1 (93).
However, we find that there are few studies of RBR E3 ubiquitin ligase in osteosarcoma.
3.3. Deubiquitinylating enzymes
Numerous studies have confirmed that deubiquitinating enzymes are widely involved in the development of osteosarcoma. Previous studies have demonstrated that DNA-binding inhibitors (IDs) antagonize basic-helix-loop-helix (bHLH) transcription factors to inhibit cell differentiation and keep maintenance of stem cell status (94, 95). Williams et al. found that USP1 expression was overexpressed in osteosarcoma and USP1 could bind and deubiquitinates ID1, ID2, and ID3. Moreover, USP1 inhibits bHLH-dependent expression of CDKI p21, inhibits osteoblast differentiation, and leads to uncontrolled proliferation of osteosarcoma cells (96, 97). In addition, it has been found that down-regulation of USP1 in osteosarcoma cells inhibits the expression of a variety of genes including SIK2, MMP-2, GSK-3β, Bcl-2, STAT3, cyclin E1, Notch1, Wnt-1 and cyclin A1. Moreover, inhibition of USP1 expression suppresses tumor growth in osteosarcoma (98). miR-192-5p has been identified to suppress osteosarcoma initiation and progression by targeting USP1 (99).
Through statistical studies, Lavaud et al. found that patients with highly expressed USP6 and USP41 had significantly decreased survival (100). BRCA1-associated protein-1 (BAP1), an important nucleus-associated deubiquitinating enzyme, was significantly decreased in osteosarcoma, and was able to affect metastasis and invasion of osteosarcoma cells by regulating the PI3K/AKT pathway (101). Zhang et al. demonstrated that USP22 was highly expressed and acted as a pro-oncogene via PI3K/Akt pathway in osteosarcoma (102). Zeng et al. found that USP7 was significantly upregulated in osteosarcoma and could directly bind to β-catenin and activate the Wnt/β-catenin signaling pathway in order to induce epithelial-mesenchymal transition (EMT) (103). Gan et al. demonstrated that USP39 expression was overexpressed in osteosarcoma and that USP39 knockdown arrested osteosarcoma cells in G2/M phase, thereby inhibiting tumor growth and metastasis (104). MiR-140 suppresses osteosarcoma progression by inhibiting USP22-mediated LSD1 stability, resulting in promoting ubiquitination of LSD1 and increasing p21 expression (105). lncRNA DSCAM-AS1 has been demonstrated to increase USP47 expression through sponging miR-101-3p to promote osteosarcoma progression (106).
3.4. Ubiquitination altered by other genes
In some osteosarcoma related studies, although the relevant ubiquitinase has not been well clarified, ubiquitination is considered to be involved in tumor progression. Previous studies have demonstrated that DCB1 can synergize with androgen receptor (AR) and is concomitantly expressed in malignancies (107, 108). Wagle et al. showed that DCB1 knockdown enhanced AR ubiquitination and degradation, which in turn inhibited proliferation and invasion of osteosarcoma cells (109). All-trans retinoic acid (ATRA) induced osteoblastic differentiation of osteosarcoma cells both in vivo and in vitro (110). Zhang et al. found that E2F1 specifically bound with RARα and promoted its ubiquitination leading to RARα degradation, which in turn disrupted the function of ATRA-induced osteogenic differentiation (111). lncRNA EPIC1 inhibits viability and invasion of osteosarcoma cells by promoting ubiquitination (112). Overexpression of MEF2D is associated with progression of bone malignancies (113). FAM83H can influence osteosarcoma progression by regulating β-catenin expression and ubiquitinating β-catenin (114). NRF2 has been recognized as a central hub for neutralizing ROS and restoring cellular redox balance (115), and pu et al. found that lncRNA-LAMTOR5-AS1 inhibited the ubiquitination degradation of NRF2 and thereby inhibited osteosarcoma cell proliferation and multidrug resistance in osteosarcoma (116).
4. Conclusions and prospects
At present, the important difficulty in the treatment of osteosarcoma lies in diagnosis, integrated strategy for inhibiting the distant metastasis, and improving the sensitivity of tumors to chemotherapeutic drugs. Increasing studies have demonstrated a relationship between ubiquitination and osteosarcoma progression (see Table 3). Ubiquitination process usually affects the invasion and migration, cell resistance, cell growth and other aspects to regulate the development of tumors. Thus, the studies of ubiquitination have a very positive significance for treatment of osteosarcoma. Moreover, some ubiquitin enzyme families and deubiquitinase families are widely involved in the regulation of osteosarcoma, such as, TRIM family and USP subgroup. Whether these ubiquitin enzyme families will become targets for the treatment of osteosarcoma remains further exploration. In addition, no-coding RNAs, such as miRNAs and lncRNAs, can also affect the progression of tumors by affecting the expression of ubiquitinase. They also reveal the link between osteosarcoma and ubiquitination from a more microscopic perspective.
At present, targeted therapy is tried to replace traditional chemotherapy for cancer treatment. Targeted therapy inhibits cancer proliferation and progression by interacting with protein of interest (POI). The advantage is that it only targets specific proteins or cells and has little effect on normal tissues. However, it has been found that conventional targeted small molecule drugs probably develop drug resistance, and one of the mainstreams is mutation which cause POI to no longer strongly interact with drugs. Another resistance mechanism is that cancer can evade or become insensitive to drugs through overexpression of POI or adaptation to another signaling pathway for growth or survival. In response to these problems, attempts are being made to target proteins for degradation by endogenous mechanisms. Importantly traditional idea about protein regulation are to modify proteins through DNA or RNA. In recent years, with the maturity of CRISPR/Cas9 and RNA interference (RNAi) technology (117–119), DNA and RNA technologies have become increasingly important for protein regulation. However, both types of protein regulation are indirect, and some highly active proteins may take a long time to be completely degraded, and even resistance to degradation may occur (120, 121). At this stage, there are increasing attempts to perform endogenous protein degradation by ubiquitinase. For example, the Trim-Away system has been established to target the E3 ligase TRIM21 to proteins by using antibodies, resulting in protein degradation (122). Similar studies have also included the Shield-1 system (123). At present, the relatively mature targeted protein degradation mode is proteolytic targeted chimera (PROTAC). PROTAC is a heterobifunctional small molecule consisting of two active domains and an adaptor capable of removing specific unwanted proteins. PROTAC, first introduced by Sakamoto KM et al. in 2001 (124), consists of two covalently linked protein-binding molecules: one capable of binding to an E3 ubiquitin ligase and the other to a target protein for degradation (see Figure 3) (125–127). At present, some E3 ubiquitin ligases can be degraded by PROTAC technology, such as VHL (128, 129), CRBN (130, 131), MDM2 (132), β-TRCP (124), cIAP (133), RNF4 (134), RNF14 (135), and DCAF16 (136). PROTAC which consists of VHL as E3s has been studied in osteosarcoma (137). PROTAC formulations for other tumors have begun to gradually enter clinical trials, with PROTACs ARV-110 (NCT03888612) and ARV-471 (NCT04072952) entering clinical phase II trials (127). In addition, deubiquitylation techniques have also achieved some results, and cancers are believed to be driven by abnormally ubiquitinated and degraded proteins. Cancers would benefit therapeutically from targeting protein stabilization (TPS). Therefore, deubiquitinase targeting chimeras (DUBTACs), a heterobifunctional small molecule consisting of a targeting ligand and deubiquitinase recruitment group that stabilize targeted proteins degraded by abnormal ubiquitination, have been proposed. Scholars have verified the function of this molecule by experiments on the stability of the hepatocellular carcinoma inhibitory kinase WEE1 (138). During the next few years, it is expected that more and more ubiquitinases or other targeted protein degradation methods will become key treatment options for osteosarcoma.
Data availability statement
The original contributions presented in the study are included in the article/supplementary material. Further inquiries can be directed to the corresponding author.
Author contributions
JS, XY, reviewed the literature and wrote the manuscript. LP, JW, and PW revised the manuscript. MZ, JL, and ZL reviewed, revised the manuscript and performed figures and tables of the manuscript. All authors contributed to the article and approved the submitted version.
Funding
This work was supported by the Youth Science and Technology Talent Program of Changzhou Health and Family Planning Commission (QN202106 and QN202007), Changzhou Sci&Tech Program (CJ20210085), and Youth Talent Development Plan of Changzhou Health Commission (CZQM2020047).
Conflict of interest
The authors declare that the research was conducted in the absence of any commercial or financial relationships that could be construed as a potential conflict of interest.
Publisher’s note
All claims expressed in this article are solely those of the authors and do not necessarily represent those of their affiliated organizations, or those of the publisher, the editors and the reviewers. Any product that may be evaluated in this article, or claim that may be made by its manufacturer, is not guaranteed or endorsed by the publisher.
References
3. Fletcher CD, Unni KK, Mertens F. Pathology and genetics of tumours of soft tissue and bone. Iarc (2002).
4. Luetke A, Meyers PA, Lewis I, Juergens H. Osteosarcoma treatment - where do we stand? A state of the art review. Cancer Treat Rev (2014) 40(4):523–32. doi: 10.1016/j.ctrv.2013.11.006
5. Stiller C, Bielack S, Jundt G, Steliarova-Foucher E. Bone tumours in European children and adolescents, 1978–1997. Report from the automated childhood cancer information system project. Eur J cancer (2006) 42(13):2124–35. doi: 10.1016/j.ejca.2006.05.015
6. Ottaviani G, Jaffe N. The epidemiology of osteosarcoma. Cancer Treat Res (2009) 152:3–13. doi: 10.1007/978-1-4419-0284-9_1
7. Bielack SS, Kempf-Bielack B, Delling Gn, Exner GU, Flege S, Helmke K, et al. Prognostic factors in high-grade osteosarcoma of the extremities or trunk: An analysis of 1,702 patients treated on neoadjuvant cooperative osteosarcoma study group protocols. J Clin Oncol (2002) 20(3):776–90. doi: 10.1200/JCO.2002.20.3.776
8. Kundu ZS. Classification, imaging, biopsy and staging of osteosarcoma. Indian J Orthop (2014) 48(3):238–46. doi: 10.4103/0019-5413.132491
9. Casali PG, Bielack S, Abecassis N, Aro H, Bauer S, Biagini R, et al. Bone sarcomas: ESMO–PaedCan–EURACAN clinical practice guidelines for diagnosis, treatment and follow-up. Ann Oncol (2018) 29:iv79–95. doi: 10.1093/annonc/mdy310
10. Strauss SJ, Frezza AM, Abecassis N, Bajpai J, Bauer S, Biagini R, et al. Bone sarcomas: ESMO-EURACAN-GENTURIS-ERN PaedCan clinical practice guideline for diagnosis, treatment and follow-up. Ann Oncol (2021) 32(12):1520–36. doi: 10.1016/j.annonc.2021.08.1995
11. Ta HT, Dass CR, Choong PF, Dunstan DE. Osteosarcoma treatment: State of the art. Cancer Metastasis Rev (2009) 28(1):247–63. doi: 10.1007/s10555-009-9186-7
12. Schwarz R, Bruland O, Cassoni A, Schomberg P, Bielack S. The role of radiotherapy in oseosarcoma. Pediatr Adolesc Osteosarcoma (2009) 152:147–64. doi: 10.1007/978-1-4419-0284-9_7
13. Ferguson WS, Goorin AM. Current treatment of osteosarcoma. Cancer Invest (2001) 19(3):292–315. doi: 10.1081/CNV-100102557
14. Langer T, Stöhr W, Paulides M, Kremers A, Dörr H-G, Göbel U, et al. Prospective multicenter registration of major late sequelae in sarcoma patients using the late effects surveillance system (LESS). Klinische Pädiatrie (2005) 217(03):176–81. doi: 10.1055/s-2005-836503
15. Schlesinger DH, Goldstein G. Molecular conservation of 74 amino acid sequence of ubiquitin between cattle and man. Nature (1975) 255(5507):423–4. doi: 10.1038/255423a0
16. Jin JO, Puranik N, Bui QT, Yadav D, Lee PC. The ubiquitin system: An emerging therapeutic target for lung cancer. Int J Mol Sci (2021) 22(17):9629. doi: 10.3390/ijms22179629
17. Chen L, Yuan R, Wen C, Liu T, Feng Q, Deng X, et al. E3 ubiquitin ligase UBR5 promotes pancreatic cancer growth and aerobic glycolysis by downregulating FBP1 via destabilization of C/EBPalpha. Oncogene (2021) 40(2):262–76. doi: 10.1038/s41388-020-01527-1
18. Kimura Y, Tanaka K. Regulatory mechanisms involved in the control of ubiquitin homeostasis. J Biochem (2010) 147(6):793–8. doi: 10.1093/jb/mvq044
19. Hershko A, Ciechanover A. The ubiquitin system. Annu Rev Biochem (1998) 67(1):425–79. doi: 10.1146/annurev.biochem.67.1.425
20. Pickart CM, Eddins MJ. Ubiquitin: structures, functions, mechanisms. Biochim Biophys Acta (2004) 1695(1-3):55–72. doi: 10.1016/j.bbamcr.2004.09.019
21. Yang WL, Zhang X, Lin HK. Emerging role of lys-63 ubiquitination in protein kinase and phosphatase activation and cancer development. Oncogene (2010) 29(32):4493–503. doi: 10.1038/onc.2010.190
22. Barghout SH, Schimmer AD. E1 enzymes as therapeutic targets in cancer. Pharmacol Rev (2021) 73(1):1–58. doi: 10.1124/pharmrev.120.000053
23. Nakayama KI, Nakayama K. Ubiquitin ligases: cell-cycle control and cancer. Nat Rev Cancer (2006) 6(5):369–81. doi: 10.1038/nrc1881
24. Buetow L, Huang DT. Structural insights into the catalysis and regulation of E3 ubiquitin ligases. Nat Rev Mol Cell Biol (2016) 17(10):626–42. doi: 10.1038/nrm.2016.91
25. Deshaies RJ, Joazeiro CA. RING domain E3 ubiquitin ligases. Annu Rev Biochem (2009) 78:399–434. doi: 10.1146/annurev.biochem.78.101807.093809
26. Clague MJ, Heride C, Urbé S. The demographics of the ubiquitin system. Trends Cell Biol (2015) 25(7):417–26. doi: 10.1016/j.tcb.2015.03.002
27. Grabbe C, Husnjak K, Dikic I. The spatial and temporal organization of ubiquitin networks. Nat Rev Mol Cell Biol (2011) 12(5):295–307. doi: 10.1038/nrm3099
28. Schnell JD, Hicke L. Non-traditional functions of ubiquitin and ubiquitin-binding proteins. J Biol Chem (2003) 278(38):35857–60. doi: 10.1074/jbc.R300018200
29. Komander D. The emerging complexity of protein ubiquitination. Biochem Soc Trans (2009) 37(Pt 5):937–53. doi: 10.1042/BST0370937
30. Hicke L. Protein regulation by monoubiquitin. Nat Rev Mol Cell Biol (2001) 2(3):195–201. doi: 10.1038/35056583
31. Martinez-Forero I, Rouzaut A, Palazon A, Dubrot J, Melero I. Lysine 63 polyubiquitination in immunotherapy and in cancer-promoting inflammation. Clin Cancer Res (2009) 15(22):6751–7. doi: 10.1158/1078-0432.CCR-09-1225
32. Trotman LC, Wang X, Alimonti A, Chen Z, Teruya-Feldstein J, Yang H, et al. Ubiquitination regulates PTEN nuclear import and tumor suppression. Cell (2007) 128(1):141–56. doi: 10.1016/j.cell.2006.11.040
33. Sadowski M, Sarcevic B. Mechanisms of mono-and poly-ubiquitination: Ubiquitination specificity depends on compatibility between the E2 catalytic core and amino acid residues proximal to the lysine. Cell division (2010) 5(1):1–5. doi: 10.1186/1747-1028-5-19
34. Sadowski M, Suryadinata R, Lai X, Heierhorst, Sarcevic B. Molecular basis for lysine specificity in the yeast ubiquitin-conjugating enzyme Cdc34. Mol Cell Biol (2010) 30(10):2316–29. doi: 10.1128/MCB.01094-09
35. Fallon L, Bélanger CM, Corera AT, Kontogiannea M, Regan-Klapisz E, Moreau F, et al. A regulated interaction with the UIM protein Eps15 implicates parkin in EGF receptor trafficking and PI (3) K–akt signalling. Nat Cell Biol (2006) 8(8):834–42. doi: 10.1038/ncb1441
36. Antao AM, Tyagi A, Kim KS, Ramakrishna S. Advances in deubiquitinating enzyme inhibition and applications in cancer therapeutics. Cancers (Basel) (2020) 12(6):1579. doi: 10.3390/cancers12061579
37. Hymowitz SG, Wertz IE. A20: from ubiquitin editing to tumour suppression. Nat Rev Cancer (2010) 10(5):332–41. doi: 10.1038/nrc2775
38. Sun T, Liu Z, Yang Q. The role of ubiquitination and deubiquitination in cancer metabolism. Mol Cancer (2020) 19(1):146. doi: 10.1186/s12943-020-01262-x
39. Park S-H. Fine tuning and cross-talking of TGF-β signal by inhibitory smads. BMB Rep (2005) 38(1):9–16. doi: 10.5483/BMBRep.2005.38.1.009
40. Pardali K, Moustakas A. Actions of TGF-β as tumor suppressor and pro-metastatic factor in human cancer. Biochim Biophys Acta (BBA)-Reviews Cancer (2007) 1775(1):21–62. doi: 10.1016/j.bbcan.2006.06.004
41. Cao W, Liu X, Meng S, Gao Y, Wang Y, Ma Z, et al. USP4 promotes invasion of breast cancer cells via Relaxin/TGF-β1/Smad2/MMP-9 signal. Eur Rev Med Pharmacol Sci (2016) 20(6):1115–22.
42. Eichhorn PJ, Rodón L, Gonzàlez-Juncà A, Dirac A, Gili M, Martínez-Sáez E, et al. USP15 stabilizes TGF-β receptor I and promotes oncogenesis through the activation of TGF-β signaling in glioblastoma. Nat Med (2012) 18(3):429–35. doi: 10.1038/nm.2619
43. Ben-Neriah Y, Karin M. Inflammation meets cancer, with NF-κB as the matchmaker. Nat Immunol (2011) 12(8):715–23. doi: 10.1038/ni.2060
44. Vucic D, Dixit VM, Wertz IE. Ubiquitylation in apoptosis: A post-translational modification at the edge of life and death. Nat Rev Mol Cell Biol (2011) 12(7):439–52. doi: 10.1038/nrm3143
45. Evans PC, Ovaa H, Hamon M, Kilshaw PJ, Hamm S, Bauer S, et al. Zinc-finger protein A20, a regulator of inflammation and cell survival, has de-ubiquitinating activity. Biochem J (2004) 378(3):727–34. doi: 10.1042/bj20031377
46. Xu G, Tan X, Wang H, Sun W, Shi Y, Burlingame S, et al. Ubiquitin-specific peptidase 21 inhibits tumor necrosis factor α-induced nuclear factor κB activation via binding to and deubiquitinating receptor-interacting protein 1. J Biol Chem (2010) 285(2):969–78. doi: 10.1074/jbc.M109.042689
47. Hou X, Wang L, Zhang L, Pan X, Zhao W. Ubiquitin-specific protease 4 promotes TNF-α-induced apoptosis by deubiquitination of RIP1 in head and neck squamous cell carcinoma. FEBS letters (2013) 587(4):311–6. doi: 10.1016/j.febslet.2012.12.016
48. Li X, Wu L, Zopp M, Kopelov S, Du W. p53-TP53-Induced glycolysis regulator mediated glycolytic suppression attenuates DNA damage and genomic instability in fanconi anemia hematopoietic stem cells. Stem Cells (2019) 37(7):937–47. doi: 10.1002/stem.3015
49. Wade M, Wang YV, Wahl GM. The p53 orchestra: Mdm2 and mdmx set the tone. Trends Cell Biol (2010) 20(5):299–309. doi: 10.1016/j.tcb.2010.01.009
50. Zhou J, Wang J, Chen C, Yuan H, Wen X, Sun H. USP7: target validation and drug discovery for cancer therapy. Medicinal Chem (2018) 14(1):3–18. doi: 10.2174/1573406413666171020115539
51. Qi S-M, Cheng G, Cheng X-D, Xu Z, Xu B, Zhang W-D, et al. Targeting USP7-mediated deubiquitination of MDM2/MDMX-p53 pathway for cancer therapy: are we there yet? Front Cell Dev Biol (2020) 8:233. doi: 10.3389/fcell.2020.00233
52. Kwon S-K, Saindane M, Baek K-H. p53 stability is regulated by diverse deubiquitinating enzymes. Biochim Biophys Acta (BBA)-Reviews Cancer (2017) 1868(2):404–11. doi: 10.1016/j.bbcan.2017.08.001
53. Nielsen CP, Jernigan KK, Diggins NL, Webb DJ, MacGurn JA. USP9X deubiquitylates DVL2 to regulate WNT pathway specification. Cell Rep (2019) 28(4):1074–89. e5. doi: 10.1016/j.celrep.2019.06.083
54. Yan G, Liu N, Tian J, Fu Y, Wei W, Zou J, et al. Deubiquitylation and stabilization of ARMC5 by ubiquitin-specific processing protease 7 (USP7) are critical for RCC proliferation. J Cell Mol Med (2021) 25(6):3149–59. doi: 10.1111/jcmm.16306
55. Chen S, Yang J, Zhang Y, Duan C, Liu Q, Huang Z, et al. Ubiquitin-conjugating enzyme UBE2O regulates cellular clock function by promoting the degradation of the transcription factor BMAL1. J Biol Chem (2018) 293(29):11296–309. doi: 10.1074/jbc.RA117.001432
56. Wang Y, Leng H, Chen H, Wang L, Jiang N, Huo X, et al. Knockdown of UBE2T inhibits osteosarcoma cell proliferation, migration, and invasion by suppressing the PI3K/Akt signaling pathway. Oncol Res (2016) 24(5):361–9. doi: 10.3727/096504016X14685034103310
57. Shadfan M, Lopez-Pajares V, Yuan Z-M. MDM2 and MDMX: Alone and together in regulation of p53. Trans Cancer Res (2012) 1(2):88.
58. Egorova O, Lau HH, McGraphery K, Sheng Y. Mdm2 and MdmX RING domains play distinct roles in the regulation of p53 responses: A comparative study of Mdm2 and MdmX RING domains in U2OS cells. Int J Mol Sci (2020) 21(4):1309. doi: 10.3390/ijms21041309
59. Shi D, Wu F, Mu S, Hu B, Zhong B, Gao F, et al. LncRNA AFAP1-AS1 promotes tumorigenesis and epithelial-mesenchymal transition of osteosarcoma through RhoC/ROCK1/p38MAPK/Twist1 signaling pathway. J Exp Clin Cancer Res (2019) 38(1):1–12. doi: 10.1186/s13046-019-1363-0
60. Guan X, Xu Y, Zheng J. Long noncoding RNA PCAT6 promotes the development of osteosarcoma by increasing MDM2 expression. Oncol Rep (2020) 44(6):2465–74. doi: 10.3892/or.2020.7813
61. Seifi-Alan M, Shamsi R, Ghafouri-Fard S, Mirfakhraie R, Zare-Abdollahi D, Movafagh A, et al. Expression analysis of two cancer-testis genes, FBXO39 and TDRD4, in breast cancer tissues and cell lines. Asian Pacific J Cancer Prev (2013) 14(11):6625–9. doi: 10.7314/APJCP.2013.14.11.6625
62. Zheng J, You W, Zheng C, Wan P, Chen J, Jiang X, et al. Knockdown of FBXO39 inhibits proliferation and promotes apoptosis of human osteosarcoma U-2OS cells. Oncol Lett (2018) 16(2):1849–54. doi: 10.3892/ol.2018.8876
63. Tomar D, Singh R. TRIM family proteins: emerging class of RING E3 ligases as regulator of NF-κB pathway. Biol Cell (2015) 107(1):22–40. doi: 10.1111/boc.201400046
64. Wang Z, Xu X, Tang W, Zhu Y, Hu J, Zhang X. Tripartite motif containing 11 interacts with DUSP6 to promote the growth of human osteosarcoma cells through regulating ERK1/2 pathway. BioMed Res Int (2019) 2019:9612125. doi: 10.1155/2019/9612125
65. Jiang W, Cai X, Xu T, Liu K, Yang D, Fan L, et al. Tripartite motif-containing 46 promotes viability and inhibits apoptosis of osteosarcoma cells by activating NF-b signaling through ubiquitination of PPAR. Oncol Res (2020) 28(4):409–21. doi: 10.3727/096504020X15868639303417
66. Yuan P, Zhou Y, Wang R, Chen S, Wang Q, Xu Z, et al. TRIM58 interacts with pyruvate kinase M2 to inhibit tumorigenicity in human osteosarcoma cells. BioMed Res Int (2020) 2020:8450606. doi: 10.1155/2020/8450606
67. Zhou C, Zhang Z, Zhu X, Qian G, Zhou Y, Sun Y, et al. N6-methyladenosine modification of the TRIM7 positively regulates tumorigenesis and chemoresistance in osteosarcoma through ubiquitination of BRMS1. EBioMedicine (2020) 59:102955. doi: 10.1016/j.ebiom.2020.102955
68. Harper JW, Schulman BA. Cullin-RING ubiquitin ligase regulatory circuits: A quarter century beyond the f-box hypothesis. Annu Rev Biochem (2021) 90:403–29. doi: 10.1146/annurev-biochem-090120-013613
69. Chen Z, Sui J, Zhang F, Zhang C. Cullin family proteins and tumorigenesis: genetic association and molecular mechanisms. J Cancer (2015) 6(3):233. doi: 10.7150/jca.11076
70. Chen Z, Wang K, Hou C, Jiang K, Chen B, Chen J, et al. CRL4B(DCAF11) E3 ligase targets p21 for degradation to control cell cycle progression in human osteosarcoma cells. Sci Rep (2017) 7(1):1175. doi: 10.1038/s41598-017-00073-3
71. Hsu H, Xiong J, Goeddel DV. The TNF receptor 1-associated protein TRADD signals cell death and NF-κB activation. Cell (1995) 81(4):495–504. doi: 10.1016/0092-8674(95)90070-5
72. Zhang C, Chen B, Jiang K, Lao L, Shen H, Chen Z. Activation of TNF-alpha/NF-kappaB axis enhances CRL4B(DCAF)(11) E3 ligase activity and regulates cell cycle progression in human osteosarcoma cells. Mol Oncol (2018) 12(4):476–94. doi: 10.1002/1878-0261.12176
73. Poos K, Smida J, Nathrath M, Maugg D, Baumhoer D, Korsching E. How microRNA and transcription factor co-regulatory networks affect osteosarcoma cell proliferation. PloS Comput Biol (2013) 9(8):e1003210. doi: 10.1371/journal.pcbi.1003210
74. Lulla RR, Costa FF, Bischof JM, Chou PM, Bonaldo M, Vanin EF, et al. Identification of differentially expressed microRNAs in osteosarcoma. Sarcoma (2011) 2011:732690. doi: 10.1155/2011/732690
75. Chen Z, Zhang W, Jiang K, Chen B, Wang K, Lao L, et al. MicroRNA-300 regulates the ubiquitination of PTEN through the CRL4B(DCAF13) E3 ligase in osteosarcoma cells. Mol Ther Nucleic Acids (2018) 10:254–68. doi: 10.1016/j.omtn.2017.12.010
76. Chen B, Feng Y, Zhang M, Cheng G, Chen B, Wang H. Small molecule TSC01682 inhibits osteosarcoma cell growth by specifically disrupting the CUL4B-DDB1 interaction and decreasing the ubiquitination of CRL4B E3 ligase substrates. Am J Cancer Res (2019) 9(9):1857–70.
77. Chen X, Shen J, Li X, Wang X, Long M, Lin F, et al. Rlim, an E3 ubiquitin ligase, influences the stability of stathmin protein in human osteosarcoma cells. Cell Signal (2014) 26(7):1532–8. doi: 10.1016/j.cellsig.2014.03.018
78. Chen L, Pei H, Lu SJ, Liu ZJ, Yan L, Zhao XM, et al. SPOP suppresses osteosarcoma invasion via PI3K/AKT/NF-kappaB signaling pathway. Eur Rev Med Pharmacol Sci (2018) 22(3):609–15. doi: 10.26355/eurrev_201802_14275
79. Severe N, Dieudonne FX, Marty C, Modrowski D, Patino-Garcia A, Lecanda F, et al. Targeting the E3 ubiquitin casitas b-lineage lymphoma decreases osteosarcoma cell growth and survival and reduces tumorigenesis. J Bone Miner Res (2012) 27(10):2108–17. doi: 10.1002/jbmr.1667
80. Zhang P, Yang Y, Nolo R, Zweidler-McKay PA, Hughes DP. Regulation of NOTCH signaling by reciprocal inhibition of HES1 and deltex 1 and its role in osteosarcoma invasiveness. Oncogene (2010) 29(20):2916–26. doi: 10.1038/onc.2010.62
81. Li M, Wu W, Deng S, Shao Z, Jin X. TRAIP modulates the IGFBP3/AKT pathway to enhance the invasion and proliferation of osteosarcoma by promoting KANK1 degradation. Cell Death Dis (2021) 12(8):767. doi: 10.1038/s41419-021-04057-0
82. Kamal MM, Omran OM. The role of heat shock protein 70 induced by geranylgeranylacetone in carbon tetrachloride-exposed adult rat testes. Pathophysiology (2013) 20(2):139–46. doi: 10.1016/j.pathophys.2013.03.003
83. Jiang L, Liao J, Liu J, Wei Q, Wang Y. Geranylgeranylacetone promotes human osteosarcoma cell apoptosis by inducing the degradation of PRMT1 through the E3 ubiquitin ligase CHIP. J Cell Mol Med (2021) 25(16):7961–72. doi: 10.1111/jcmm.16725
84. Sewart K, Hauf S. Different functionality of Cdc20 binding sites within the mitotic checkpoint complex. Curr Biol (2017) 27(8):1213–20. doi: 10.1016/j.cub.2017.03.007
85. Sackton KL, Dimova N, Zeng X, Tian W, Zhang M, Sackton TB, et al. Synergistic blockade of mitotic exit by two chemical inhibitors of the APC/C. Nature (2014) 514(7524):646–9. doi: 10.1038/nature13660
86. Gao Y, Zhang B, Wang Y, Shang G. Cdc20 inhibitor apcin inhibits the growth and invasion of osteosarcoma cells. Oncol Rep (2018) 40(2):841–8. doi: 10.3892/or.2018.6467
87. Fu L, Cui CP, Zhang X, Zhang L. The functions and regulation of smurfs in cancers. Semin Cancer Biol (2020) 67(Pt 2):102–16. doi: 10.1016/j.semcancer.2019.12.023
88. Sancho E, Vilá MR, Sánchez-Pulido L, Lozano JJ, Paciucci R, Nadal M, et al. Role of UEV-1, an inactive variant of the E2 ubiquitinconjugating enzymes, in in vitro differentiation and cell cycle behavior of HT-29-M6 intestinal mucosecretory cells. Mol Cell Biol (1998) 18(1):576–89. doi: 10.1128/MCB.18.1.576
89. Zhang W, Zhuang Y, Zhang Y, Yang X, Zhang H, Wang G, et al. Uev1A facilitates osteosarcoma differentiation by promoting Smurf1-mediated Smad1 ubiquitination and degradation. Cell Death Dis (2017) 8(8):e2974. doi: 10.1038/cddis.2017.366
90. Huang Y, Yang Y, Gao R, Yang X, Yan X, Wang C, et al. RLIM interacts with Smurf2 and promotes TGF-beta induced U2OS cell migration. Biochem Biophys Res Commun (2011) 414(1):181–5. doi: 10.1016/j.bbrc.2011.09.053
91. Zhang L, Anglesio MS, O'Sullivan M, Zhang F, Yang G, Sarao R, et al. The E3 ligase HACE1 is a critical chromosome 6q21 tumor suppressor involved in multiple cancers. Nat Med (2007) 13(9):1060–9. doi: 10.1038/nm1621
92. Daugaard M, Nitsch R, Razaghi B, McDonald L, Jarrar A, Torrino S, et al. Hace1 controls ROS generation of vertebrate Rac1-dependent NADPH oxidase complexes. Nat Commun (2013) 4(1):1–13. doi: 10.1038/ncomms3180
93. El-Naggar AM, Clarkson PW, Negri GL, Turgu B, Zhang F, Anglesio MS, et al. HACE1 is a potential tumor suppressor in osteosarcoma. Cell Death Dis (2019) 10(1):21. doi: 10.1038/s41419-018-1276-4
94. Lasorella A, Uo T, Iavarone A. Id proteins at the cross-road of development and cancer. Oncogene (2001) 20(58):8326–33. doi: 10.1038/sj.onc.1205093
95. Yokota Y, Mori S. Role of id family proteins in growth control. J Cell Physiol (2002) 190(1):21–8. doi: 10.1002/jcp.10042
96. Williams SA, Maecker HL, French DM, Liu J, Gregg A, Silverstein LB, et al. USP1 deubiquitinates ID proteins to preserve a mesenchymal stem cell program in osteosarcoma. Cell (2011) 146(6):918–30. doi: 10.1016/j.cell.2011.07.040
97. Funato N, Ohtani K, Ohyama K, Kuroda T, Nakamura M. Common regulation of growth arrest and differentiation of osteoblasts by helix-loop-helix factors. Mol Cell Biol (2001) 21(21):7416–28. doi: 10.1128/MCB.21.21.7416-7428.2001
98. Liu J, Zhu H, Zhong N, Jiang Z, Xu L, Deng Y, et al. Gene silencing of USP1 by lentivirus effectively inhibits proliferation and invasion of human osteosarcoma cells. Int J Oncol (2016) 49(6):2549–57. doi: 10.3892/ijo.2016.3752
99. Zhou S, Xiong M, Dai G, Yu L, Zhang Z, Chen J, et al. MicroRNA-192-5p suppresses the initiation and progression of osteosarcoma by targeting USP1. Oncol Lett (2018) 15(5):6947–56. doi: 10.3892/ol.2018.8180
100. Lavaud M, Mullard M, Tesfaye R, Amiaud J, Legrand M, Danieau G, et al. Overexpression of the ubiquitin specific proteases USP43, USP41, USP27x and USP6 in osteosarcoma cell lines: Inhibition of osteosarcoma tumor growth and lung metastasis development by the USP antagonist PR619. Cells (2021) 10(9):2268. doi: 10.3390/cells10092268
101. Gao S, Sun H, Cheng C, Wang G. BRCA1-associated protein-1 suppresses osteosarcoma cell proliferation and migration through regulation PI3K/Akt pathway. DNA Cell Biol (2017) 36(5):386–93. doi: 10.1089/dna.2016.3579
102. Zhang D, Jiang F, Wang X, Li G. Downregulation of ubiquitin-specific protease 22 inhibits proliferation, invasion, and epithelial-mesenchymal transition in osteosarcoma cells. Oncol Res (2017) 25(5):743–51. doi: 10.3727/096504016X14772395226335
103. Zeng Q, Li Z, Zhao X, Guo L, Yu C, Qin J, et al. Ubiquitinspecific protease 7 promotes osteosarcoma cell metastasis by inducing epithelialmesenchymal transition. Oncol Rep (2019) 41(1):543–51. doi: 10.3892/or.2018.6835
104. Gan Z, Han K, Lin S, Hu H, Shen Z, Min D. Knockdown of ubiquitin-specific peptidase 39 inhibited the growth of osteosarcoma cells and induced apoptosis in vitro. Biol Res (2017) 50(1):15. doi: 10.1186/s40659-017-0121-z
105. Liu W, Wang D, Liu L, Wang L, Yan M. miR-140 inhibits osteosarcoma progression by impairing USP22-mediated LSD1 stabilization and promoting p21 expression. Mol Ther Nucleic Acids (2021) 24:436–48. doi: 10.1016/j.omtn.2021.01.029
106. Zhang S, Ding L, Gao F, Fan H. Long non-coding RNA DSCAM-AS1 upregulates USP47 expression through sponging miR-101-3p to accelerate osteosarcoma progression. Biochem Cell Biol (2020) 98(5):600–11. doi: 10.1139/bcb-2020-0031
107. Park HS, Bae JS, Noh SJ, Kim KM, Lee H, Moon WS, et al. Expression of DBC1 and androgen receptor predict poor prognosis in diffuse large b cell lymphoma. Trans Oncol (2013) 6(3):370–81. doi: 10.1593/tlo.13250
108. Noh SJ, Kang MJ, Kim KM, Bae JS, Park HS, Moon WS, et al. Acetylation status of P53 and the expression of DBC1, SIRT1, and androgen receptor are associated with survival in clear cell renal cell carcinoma patients. Pathology (2013) 45(6):574–80. doi: 10.1097/PAT.0b013e3283652c7a
109. Wagle S, Park SH, Kim KM, Moon YJ, Bae JS, Kwon KS, et al. DBC1/CCAR2 is involved in the stabilization of androgen receptor and the progression of osteosarcoma. Sci Rep (2015) 5:13144. doi: 10.1038/srep13144
110. Luo P, Yang X, Ying M, Chaudhry P, Wang A, Shimada H, et al. Retinoid-suppressed phosphorylation of RARα mediates the differentiation pathway of osteosarcoma cells. Oncogene (2010) 29(19):2772–83. doi: 10.1038/onc.2010.50
111. Zhang L, Zhou Q, Zhang N, Li W, Ying M, Ding W, et al. E2F1 impairs all-trans retinoic acid-induced osteogenic differentiation of osteosarcoma via promoting ubiquitination-mediated degradation of RARalpha. Cell Cycle (2014) 13(8):1277–87. doi: 10.4161/cc.28190
112. Zhao W, Zhang D, Qin P, Zhang J, Cui X, Gao J, et al. Long non-coding RNA EPIC1 inhibits viability and invasion of osteosarcoma cells by promoting MEF2D ubiquitylation. Int J Biol Macromol (2019) 128:566–73. doi: 10.1016/j.ijbiomac.2019.01.156
113. Zhang M, Truscott J, Davie J. Loss of MEF2D expression inhibits differentiation and contributes to oncogenesis in rhabdomyosarcoma cells. Mol cancer (2013) 12(1):1–14. doi: 10.1186/1476-4598-12-150
114. Kim KM, Hussein UK, Park SH, Kang MA, Moon YJ, Zhang Z, et al. FAM83H is involved in stabilization of beta-catenin and progression of osteosarcomas. J Exp Clin Cancer Res (2019) 38(1):267. doi: 10.1186/s13046-019-1274-0
115. Ray PD, Huang B-W, Tsuji Y. Reactive oxygen species (ROS) homeostasis and redox regulation in cellular signaling. Cell signalling (2012) 24(5):981–90. doi: 10.1016/j.cellsig.2012.01.008
116. Pu Y, Tan Y, Zang C, Zhao F, Cai C, Kong L, et al. LAMTOR5-AS1 regulates chemotherapy-induced oxidative stress by controlling the expression level and transcriptional activity of NRF2 in osteosarcoma cells. Cell Death Dis (2021) 12(12):1125. doi: 10.1038/s41419-021-04413-0
117. Doudna JA, Charpentier E. The new frontier of genome engineering with CRISPR-Cas9. Science (2014) 346(6213):1258096. doi: 10.1126/science.1258096
118. Zhan T, Rindtorff N, Betge J, Ebert MP, Boutros M. CRISPR/Cas9 for cancer research and therapy. Semin Cancer Biol (2019) 55:106–19. doi: 10.1016/j.semcancer.2018.04.001
119. Setten RL, Rossi JJ, Han S-p. The current state and future directions of RNAi-based therapeutics. Nat Rev Drug Discovery (2019) 18(6):421–46. doi: 10.1038/s41573-019-0017-4
120. Jansen LE, Black BE, Foltz DR, Cleveland DW. Propagation of centromeric chromatin requires exit from mitosis. J Cell Biol (2007) 176(6):795–805. doi: 10.1083/jcb.200701066
121. Smoak EM, Stein P, Schultz RM, Lampson MA, Black BE. Long-term retention of CENP-a nucleosomes in mammalian oocytes underpins transgenerational inheritance of centromere identity. Curr Biol (2016) 26(8):1110–6. doi: 10.1016/j.cub.2016.02.061
122. Clift D, McEwan WA, Labzin LI, Konieczny V, Mogessie B, James LC, et al. A method for the acute and rapid degradation of endogenous proteins. Cell (2017) 171(7):1692–706. e18. doi: 10.1016/j.cell.2017.10.033
123. Burslem GM, Crews CM. Small-molecule modulation of protein homeostasis. Chem Rev (2017) 117(17):11269–301. doi: 10.1021/acs.chemrev.7b00077
124. Sakamoto KM, Kim KB, Kumagai A, Mercurio F, Crews CM, Deshaies RJ. Protacs: Chimeric molecules that target proteins to the Skp1–Cullin–F box complex for ubiquitination and degradation. Proc Natl Acad Sci (2001) 98(15):8554–9. doi: 10.1073/pnas.141230798
125. Burslem GM, Crews CM. Proteolysis-targeting chimeras as therapeutics and tools for biological discovery. Cell (2020) 181(1):102–14. doi: 10.1016/j.cell.2019.11.031
126. Li X, Song Y. Proteolysis-targeting chimera (PROTAC) for targeted protein degradation and cancer therapy. J Hematol Oncol (2020) 13(1):50. doi: 10.1186/s13045-020-00885-3
127. Bekes M, Langley DR, Crews CM. PROTAC targeted protein degraders: the past is prologue. Nat Rev Drug Discovery (2022) 21(3):181–200. doi: 10.1038/s41573-021-00371-6
128. Buckley DL, Van Molle I, Gareiss PC, Tae HS, Michel J, Noblin DJ, et al. Targeting the von hippel–lindau E3 ubiquitin ligase using small molecules to disrupt the VHL/HIF-1α interaction. J Am Chem Society (2012) 134(10):4465–8. doi: 10.1021/ja209924v
129. Bondeson DP, Mares A, Smith IE, Ko E, Campos S, Miah AH, et al. Catalytic in vivo protein knockdown by small-molecule PROTACs. Nat Chem Biol (2015) 11(8):611–7. doi: 10.1038/nchembio.1858
130. Winter GE, Buckley DL, Paulk J, Roberts JM, Souza A, Dhe-Paganon S, et al. Phthalimide conjugation as a strategy for in vivo target protein degradation. Science (2015) 348(6241):1376–81. doi: 10.1126/science.aab1433
131. Lu J, Qian Y, Altieri M, Dong H, Wang J, Raina K, et al. Hijacking the E3 ubiquitin ligase cereblon to efficiently target BRD4. Chem Biol (2015) 22(6):755–63. doi: 10.1016/j.chembiol.2015.05.009
132. Hines J, Lartigue S, Dong H, Qian Y, Crews CM. MDM2-recruiting PROTAC offers superior, synergistic antiproliferative activity via simultaneous degradation of BRD4 and stabilization of p53. Cancer Res (2019) 79(1):251–62. doi: 10.1158/0008-5472.CAN-18-2918
133. Itoh Y, Ishikawa M, Naito M, Hashimoto Y. Protein knockdown using methyl bestatin– ligand hybrid molecules: design and synthesis of inducers of ubiquitination-mediated degradation of cellular retinoic acid-binding proteins. J Am Chem Society (2010) 132(16):5820–6. doi: 10.1021/ja100691p
134. Ward CC, Kleinman JI, Brittain SM, Lee PS, Chung CYS, Kim K, et al. Covalent ligand screening uncovers a RNF4 E3 ligase recruiter for targeted protein degradation applications. ACS Chem Biol (2019) 14(11):2430–40. doi: 10.1021/acschembio.8b01083
135. Spradlin JN, Hu X, Ward CC, Brittain SM, Jones MD, Ou L, et al. Harnessing the anti-cancer natural product nimbolide for targeted protein degradation. Nat Chem Biol (2019) 15(7):747–55. doi: 10.1038/s41589-019-0304-8
136. Zhang X, Crowley VM, Wucherpfennig TG, Dix MM, Cravatt BF. Electrophilic PROTACs that degrade nuclear proteins by engaging DCAF16. Nat Chem Biol (2019) 15(7):737–46. doi: 10.1038/s41589-019-0279-5
137. Shi C, Zhang H, Wang P, Wang K, Xu D, Wang H, et al. PROTAC induced-BET protein degradation exhibits potent anti-osteosarcoma activity by triggering apoptosis. Cell Death disease (2019) 10(11):1–11. doi: 10.1038/s41419-019-2022-2
Keywords: Osteosarcoma, ubiquitination, deubiquitination, post-translation modification, cell signals
Citation: Song J, Yuan X, Piao L, Wang J, Wang P, Zhuang M, Liu J and Liu Z (2022) Cellular functions and molecular mechanisms of ubiquitination in osteosarcoma. Front. Oncol. 12:1072701. doi: 10.3389/fonc.2022.1072701
Received: 17 October 2022; Accepted: 17 November 2022;
Published: 01 December 2022.
Edited by:
Matthew K. Summers, The Ohio State University, United StatesReviewed by:
Bo Zhao, Shanghai Jiao Tong University, ChinaAymeric Bailly, UMR5237 Centre de Recherche en Biologie cellulaire de Montpellier (CRBM), France
Paola Maroni, Galeazzi Orthopedic Institute (IRCCS), Italy
Copyright © 2022 Song, Yuan, Piao, Wang, Wang, Zhuang, Liu and Liu. This is an open-access article distributed under the terms of the Creative Commons Attribution License (CC BY). The use, distribution or reproduction in other forums is permitted, provided the original author(s) and the copyright owner(s) are credited and that the original publication in this journal is cited, in accordance with accepted academic practice. No use, distribution or reproduction is permitted which does not comply with these terms.
*Correspondence: Zhiwei Liu, bGl1emhpd2VpbEAxMjYuY29t
†These authors have contributed equally to this work