- 1Department of Thoracic Surgery, West China Hospital of Sichuan University, Chengdu, China
- 2West China School of Medicine, Sichuan University, Chengdu, China
Esophageal cancer (EC) is a common malignant tumor with poor prognosis, and current treatments for patients with advanced EC remain unsatisfactory. Recently, immunotherapy has been recognized as a new and promising approach for various tumors. EC cells present a high tumor mutation burden and harbor abundant tumor antigens, including tumor-associated antigens and tumor-specific antigens. The latter, also referred to as neoantigens, are immunogenic mutated peptides presented by major histocompatibility complex class I molecules. While current genomics and bioinformatics technologies have greatly facilitated the identification of tumor neoantigens, identifying individual neoantigens systematically for successful therapies remains a challenging problem. Owing to the initiation of strong, specific tumor-killing cytotoxic T cell responses, neoantigens are emerging as promising targets to develop personalized treatment and have triggered the development of cancer vaccines, adoptive T cell therapies, and combination therapies. This review aims to give a current understanding of the clinical application of neoantigens in EC and provide direction for future investigation.
Introduction
EC ranks as the seventh most common malignant tumor and the sixth leading cause of cancer-related death worldwide. An estimated 572,000 new cancers of EC and 509,000 deaths occurred in 2018 (1). To date, multidisciplinary therapy involving neoadjuvant chemoradiotherapy followed by surgery has formed the standard treatment for local advanced EC (2, 3). However, only 29% of patients who undergo resection after chemoradiotherapy show a pathological complete response, and the incidence of grade 3 or 4 treatment-related adverse events during chemoradiotherapy are common (2). The long-term survival for patients with locally advanced EC remains unsatisfactory despite therapeutic improvements (4).
Immunotherapy, including immune checkpoint blockade (5, 6), cancer vaccination (7), and adoptive T cell therapy (8), has been explored as a novel strategy for improving survival outcomes of EC patients recently. Immune checkpoints on the surface of T cells are receptors that are crucial for preventing autoimmune damage to healthy tissues. However, tumor cells exploit immune checkpoint inhibition to evade the immune response (9, 10). Immune checkpoint blockade therapy has dramatically changed the treatment of melanoma and advanced non-small-cell lung cancer (11, 12). Several randomized clinical trials have shown that immune checkpoint blockade is a promising prospect for the treatment of EC (5, 13, 14).
It is known that accumulation of genetic alterations can result in cancer development. Neoantigens, referred to as immunogenic mutated peptides in tumor cells, are presented by major histocompatibility complex class I molecules and stimulate a strong cytotoxic T cell–mediated immune response (15).
EC appears to be associated with a high tumor mutation burden and neoantigen load compared with esophageal adenocarcinoma (16, 17). Due to their strong tumor-specific immunogenicity, neoantigens are emerging as a promising target for tumor immunotherapy. Immune checkpoint blockade achieves tumor control by releasing immune inhibition, while neoantigens aim to elicit the immunogenicity of tumors and trigger cytotoxic T cell responses, thereby improving antitumor efficacy (18).
In this review, we focus on the clinical application of neoantigens and highlight advances in the use of neoantigens in cancer vaccines, adoptive T cell therapy, and combination therapy for EC, and we discuss the present barriers and strategies.
Cancer Vaccines
Traditional cancer vaccines mainly use tumor-associated antigens, which are also expressed in normal human tissue. Owing to their high tumor-specific and exceptional immunogenicity, tumor neoantigens are ideal targets for cancer vaccine design (19, 20). Neoantigen vaccines, a new type of tumor immunotherapy, can induce a strong, specific immune response and elicit stable therapeutic effects.
New York esophageal squamous cell carcinoma 1 (NY-ESO-1) is known as a member of the testis cancer gene family with high immunogenicity. Oshima et al. screened 1,969 patients with various cancers and identified serum NY-ESO-1 antibody as a tumor-specific biomarker for EC (21). Subsequently, Wada et al. performed a phase I clinical trial using NY-ESO-1 protein as a cancer vaccine for eight patients with advanced EC (22). Antibody, CD4+, and CD8+ T cell responses were recognized in 87.5% (7/8), 87.5% (7/8), and 75.0% (6/8) of patients, respectively. Nevertheless, disease progression was eventually observed in all patients after vaccination for various reasons, such as primary tumor growth or paratracheal or abdominal lymph node metastases.
In a phase I dose-escalation trial of a two-dose cohort, cholesteryl pullulan (CHP)-NY-ESO-1 vaccine was confirmed to be safe and to induce immunogenicity in patients with advanced metastatic EC. The 200 μg dose cohort elicited stronger immune responses and displayed better survival outcomes than the 100 μg dose cohort (23). In addition, a phase II comparative study of CHP-NY-ESO-1 vaccine was performed in NY-ESO-1-expressing patients with esophageal squamous cell carcinoma who underwent neoadjuvant chemotherapy followed by surgery. No significant difference in survival benefit was noted between the CHP-NY-ESO-1 vaccine group and untreated controls (24).
Several studies have shown that the neoantigen vaccine can successfully induce cytotoxic T cells and trigger immune effects (25–27). However, sufficient and persistent immune effects are difficult to achieve with antitumor vaccines (25, 26). Rosenberg et al. suggested tumor cells possibly possess multiple immune escape mechanisms, such as insufficient tumor antigen expression, the tumor produces local immunosuppressive factors, T cells are “tolerized”, or there is downregulation of T cell receptor signal transduction, among other things (28).
Compared with traditional peptide vaccines, mRNA vaccines are a genetic vaccine that might stimulate an effective immune response. Notably, Forghanifard et al. were the first to report that a novel chimeric mRNA-loaded dendritic-cell (DC) vaccine can elicit an effective immune response and induce cytotoxicity against EC ex vivo. Although this was a preclinical study, it provided a new approach to use DC-based vaccines for EC immunotherapy (29).
Adoptive T Cell Therapy
Tumor-specific neoantigens can also serve as promising targets for adoptive cell therapy. This involves patient T cells that have been genetically engineered to target a tumor cell surface antigen. The current strategies mostly use antigen-specific T cell receptor (TCR) gene-engineered T cells, chimeric antigen receptor (CAR) T cells, or tumor-infiltrating lymphocytes (TIL). These therapies have yielded remarkable tumor responses in clinical trials of hematological tumors and high mutation tumors (30, 31). However, these therapeutic options have limited therapeutic effect or are poorly understood in solid tumors.
Kageyama et al. conducted a first-in-man trial using adoptive cell therapy of MAGE-A4 T cell receptor gene-transduced lymphocytes in patients with MAGE-A4-expressing EC. Results revealed that T cell receptor gene-engineered T cells can be detected in the peripheral blood of all patients at the initial administered level for 1 month. Notably, transferred T cells in five patients persisted for more than 5 months. However, the antitumor effect was limited. Despite the long persistence of transferred T cells in blood, disease progression occurred in 70% (7/10) of patients after treatment. It is noteworthy that 30% (3/10) of patients with minimal tumor lesions had progression-free survival for 27 months (32). These findings suggest that adoptive T cell therapy may provide better survival outcomes in patients with early-stage tumors compared with those with advanced or recurrent EC. An explanation may be that various mechanisms underlie the benefits of adoptive T cell therapy. Another explanation might be cross-reactivity to corresponding wild-type peptide sequences in the identification of neoantigens. A recent study reported the establishment of a protocol for effective construction of neoantigen-specific TCR T cells to improve therapeutic outcomes (33).
Advances in genomics and bioinformatics have accelerated the emerging technology of CAR T cells, which transformed the field of adoptive T cell therapy. To our knowledge, no clinical trial using neoantigen target CAR T cell therapy in EC patients has been published. It has been reported that CAR T cells targeting EphA2 receptors stimulated antitumor activity of EC cells in a preclinical trial (34). The only clinical trial of CAR T cell therapy for EC, targeting the EpCAM adhesion molecule, is ongoing (NCT03013712). Both EphA2 and EpCAM are tumor-associated antigens that are overexpressed in patients with esophageal squamous cell carcinoma but are not tumor-specific antigens (34–36).
TIL is another candidate for T-cell therapy. Several clinical studies have demonstrated successful adoptive TIL therapy for solid tumors such as melanoma and ovarian cancer (37–40). In EC, TIL positivity is associated with better survival outcomes (41). Tan et al. used TIL to construct neoantigen-specific TCR T cells for esophageal squamous cell cancer and identified antitumor activity in vivo and in vitro (42). Due to this study only enrolling one patient, it is difficult to comprehensively assess the efficacy of adoptive TIL therapy. We recommend future studies enroll larger populations. To date, a clinical trial of adoptive TIL transfer therapy for EC has not been reported.
Combination Therapy
Although neoantigen-specific cancer vaccines or adoptive T cell therapy can elicit specific immune responses, tumor cells can escape from the immune system through multiple mechanisms (43–45). Additionally, the tumor microenvironment is rather complex and quite different from an in vitro milieu. Components derived from a neoantigen-enriched tumor microenvironment could suppress the function of effector T cells (46, 47). Therefore, combinations of neoantigen immunotherapy and different modes of treatment are now much more attractive.
Studies found that cancer vaccines could stimulate the proliferation of specific T cells; however, the expression of the programmed death ligand PD-L1 correspondingly increased (48, 49). This might suggest that cancer vaccines combined with a PD-L1 inhibitor or a PD-1 receptor inhibitor may be a possible therapeutic strategy. In a preclinical trial, Ishihara et al. tested a combination therapy of CHP-NY-ESO-1 cancer vaccine and an anti-PD-1 monoclonal antibody and induced significant tumor suppression (P = 0.029) compared with the no-treatment group (27). This combination therapy is a promising strategy and merits future attention.
Beyond this, it has been reported that a tumor-specific vaccine combined with immune checkpoint therapy can lead to a more efficient response than monotherapy in pancreatic and prostate cancer in preclinical comparative studies (50, 51). A neoantigen-specific cancer vaccine combined with adoptive T cell therapy has also been successfully used to achieve an antitumor response. Kageyama et al. conducted a phase I clinical trial of MAGE-A4 peptide vaccinations combined with TCR gene-engineered T cell in EC. Persistence of tumor-specific reactivity was detected although tumor regression was not observed.
Chemotherapy and radiation are important components in the treatment arsenal for EC patients. Several studies have confirmed that chemotherapy or radiotherapy can stimulate tumors to release antigens and induce the production of tumor antigen-specific effector cells in tumor tissue (52, 53). In EC, a phase I clinical trial of a multiple-epitope peptide vaccine combined with chemoradiation therapy has successfully been performed. Notably, six of 11 patients achieved complete response, and four patients with complete response experienced long-term survival benefit (54). Approaches to integrate chemoradiotherapy with immunotherapy provide novel therapeutic strategies and are now in the ascendance.
Summary and Future Directions
This review summarized the trials with neoantigens for EC or solid tumors including EC (Table 1) and the major types of neoantigens in EC with clinical application (Figure 1). Compared with immunotherapy based on tumor-related antigens, neoantigen-specific cancer vaccines or adoptive T cell transfer can drive specific immune responses and reduce adverse effects on normal tissue. However, effective antitumor activity and even survival benefit are currently not readily achievable with neoantigen monotherapy (55, 56). The maturation of technologies identifying non-synonymous mutations and evaluating the immunogenicity of mutated peptides will determine the success of future clinical applications (15, 57). Although substantial challenges lie ahead on the road to enabling neoantigen-specific effector T cells to completely eliminate tumor cells, recent advances in whole exome sequencing and bioinformatics technology will greatly facilitate the journey (58–60). There are three major strategies for the selection of candidate neoantigens—in silico peptide prediction, mass spectrometry-based immunopeptidomics, and whole-exome sequencing database list (15).
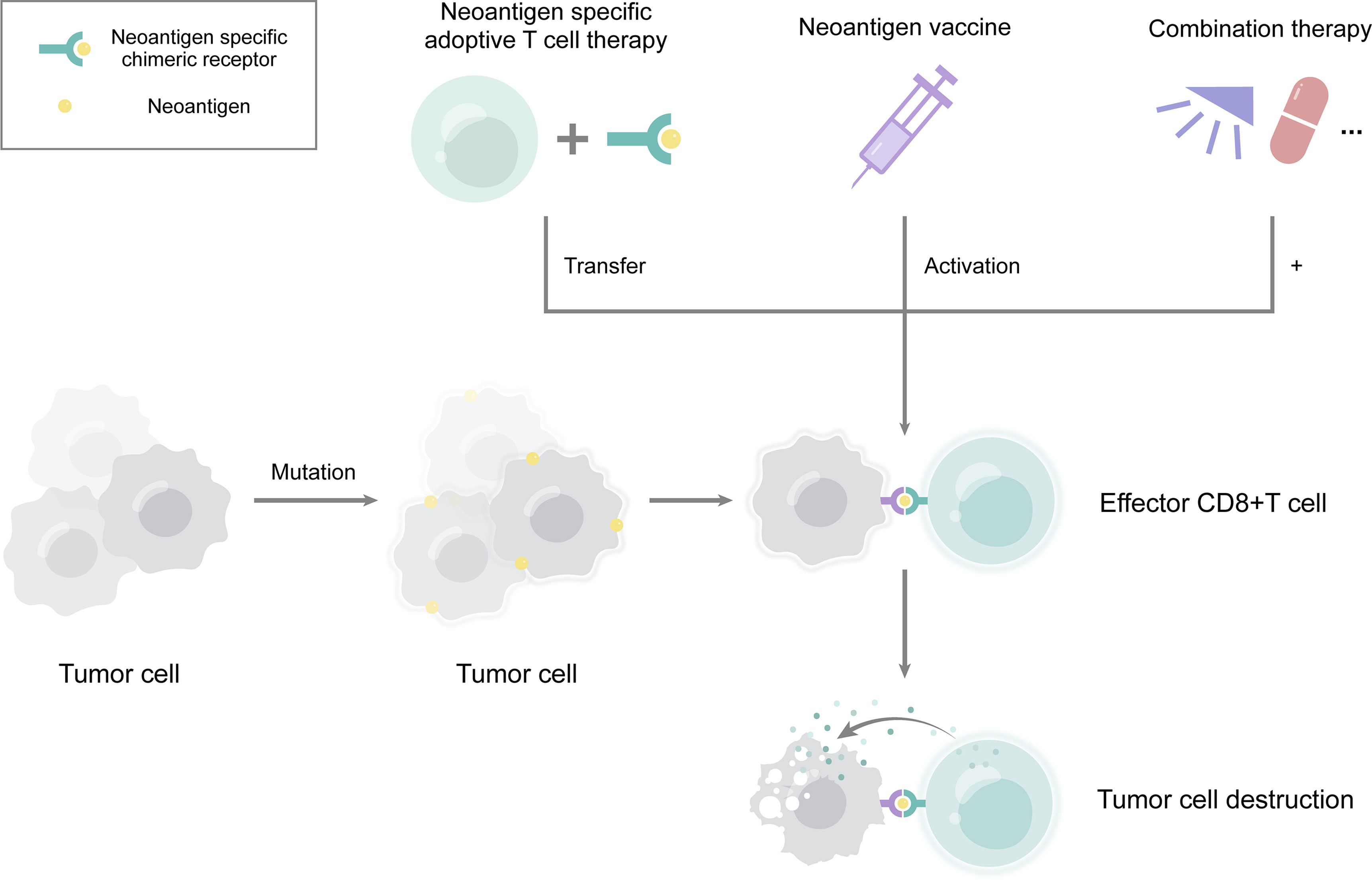
Figure 1 The major clinical application of neoantigens in esophageal cancer. Neoantigens derived from mutations in tumor cells. They can serve many purposes, including neoantigen vaccination, neoantigen specific adoptive T cell therapy, and combination therapy. Combinations of neoantigens with radiotherapy, chemotherapy, or immune checkpoint inhibitor therapy may lead to a better effect. Neoantigens induce the production of effector CD8+ T cells, thereby eliminating tumor cells.
Better understanding the mechanisms of resistance to immunotherapy may enable the development of new strategies to improve clinical outcomes. While tumor cell intrinsic factors for resistance of immunotherapy remain poorly understood, published evidence points to the possibility of alterations in interferon (IFN)-gamma signaling pathways and absence of antigen presentation (61). It is already documented that IFN-gamma is the primary cytokine involved in the recognition and elimination of mutant cells in the classical signaling pathway (62). However, tumor cells could escape this effect by upregulating inhibitory ligands that impede the response of T cells or by inducing mutations in the IFN-gamma signaling pathway, resulting in immune evasion (63, 64). In addition, neoantigens or immunogenic mutated peptides are processed and presented on human leukocyte antigen (HLA) molecules of cancer cells. This observation raises the possibility that resistance to tumor-infiltrating lymphocyte adoptive cell transfer therapy could be mediated through silenced HLA molecules (65).
Tumor cell extrinsic factors may also contribute to resistance. In addition to tumor cells and immune cells, as well as the surrounding stroma, abundant immunosuppressive cells, including T regulatory cells, myeloid-derived suppressor cells, and tumor-associated macrophages, in the tumor microenvironment have important roles in the activity of cytotoxic T cells (66, 67). Spranger et al. showed that tumor immune escape is associated with a mechanism whereby a cancer vaccine could elicit T cell activation and upregulate regulatory T cells simultaneously (68). Moreover, Eil et al. found that tumor necrosis releases intracellular potassium ions and blocks the ionic checkpoint, resulting in inhibition of the effector T cell response (69). Hence, the process of tumor immunogenicity and immune escape involves multiple mechanisms, and there remains much to explore in future research.
To overcome the resistance of immunotherapy, contemporary approaches primarily focus on the combination therapy of neoantigen-specific immunotherapy and traditional therapy. As described previously, chemotherapy or radiotherapy might facilitate effector T cell infiltration by eliciting tumor tissue to release more antigens (52, 54). Another actionable approach involves the use of neoantigen-specific immunotherapy and PD-1 or PD-L1 inhibitor therapy. The rationale for this combination is that blocking active checkpoints might reactivate T cell function (70). Furthermore, the strategy combining molecularly targeted therapy with immunotherapy has been studied in patients with melanoma, and a synergistic effect has been observed (71). These combination strategies may be the potential future directions in the treatment of EC.
As an emerging therapeutic approach, neoantigen-specific immunotherapy has shown potential therapeutic effect on EC in several preclinical and clinical trials. Here, we provide a clear picture of the clinical application of neoantigens in EC from tumor-specific cancer vaccines and adoptive T cells to combination therapy. We also discuss the current barriers and strategies, thus providing direction for future investigation.
Author Contributions
L-QC and YY conceptualized the study, revised the manuscript, and supervised the study. Y-MG and YZ conceptualized the study, drafted the manuscript, and made the figures. Y-MG and YZ collected the literature and revised the manuscript. All authors contributed to the article and approved the submitted version.
Funding
This study was supported by the National Natural Science Foundation of China (Grant No. 81970481) and 1.3.5 project for disciplines of excellence-Clinical Research Incubation Project, West China Hospital, Sichuan University (Grant Nos. 2020HXFH047 and 20HXJS005).
Conflict of Interest
The authors declare that the research was conducted in the absence of any commercial or financial relationships that could be construed as a potential conflict of interest.
Publisher’s Note
All claims expressed in this article are solely those of the authors and do not necessarily represent those of their affiliated organizations, or those of the publisher, the editors and the reviewers. Any product that may be evaluated in this article, or claim that may be made by its manufacturer, is not guaranteed or endorsed by the publisher.
References
1. Torre LA, Bray F, Siegel RL, Ferlay J, Lortet-Tieulent J, Jemal A. Global Cancer Statistics, 2012. CA Cancer J Clin (2015) 65(2):87–108. doi: 10.3322/caac.21262
2. van Hagen P, Hulshof MC, van Lanschot JJ, Steyerberg EW, van Berge Henegouwen MI, Wijnhoven BP, et al. Preoperative Chemoradiotherapy for Esophageal or Junctional Cancer. N Engl J Med (2012) 366(22):2074–84. doi: 10.1056/NEJMoa1112088
3. Yang H, Liu H, Chen Y, Zhu C, Fang W, Yu Z, et al. Neoadjuvant Chemoradiotherapy Followed by Surgery Versus Surgery Alone for Locally Advanced Squamous Cell Carcinoma of the Esophagus (NEOCRTEC5010): A Phase III Multicenter, Randomized, Open-Label Clinical Trial. J Clin Oncol (2018) 36(27):2796–803. doi: 10.1200/jco.2018.79.1483
4. Shapiro J, van Lanschot JJB, Hulshof M, van Hagen P, van Berge Henegouwen MI, Wijnhoven BPL, et al. Neoadjuvant Chemoradiotherapy Plus Surgery Versus Surgery Alone for Oesophageal or Junctional Cancer (CROSS): Long-Term Results of a Randomised Controlled Trial. Lancet Oncol (2015) 16(9):1090–8. doi: 10.1016/s1470-2045(15)00040-6
5. Kato K SJ, Shah MA. Pembrolizumab Plus Chemotherapy Versus Chemotherapy as First-Line Therapy in Patients With Advanced Esophageal Cancer: The Phase 3 KEYNOTE-590 Study. Ann Oncol (2020) 31(Suppl 4):S1192–S3. doi: 10.1016/j.annonc.2020.08.2298
6. Kelly RJ AJ, Kuzdzal J. Adjuvant Nivolumab in Resected Esophageal or Gastroesophageal Junction Cancer (EC/GEJC) Following Neoadjuvant Chemoradiation Therapy (CRT): First Results of the CheckMate 577 Study. Ann Oncol (2020) 31(Suppl 4):S1193–4. doi: 10.1016/j.annonc.2020.08.2299
7. Iwahashi M, Katsuda M, Nakamori M, Nakamura M, Naka T, Ojima T, et al. Vaccination With Peptides Derived From Cancer-Testis Antigens in Combination With CpG-7909 Elicits Strong Specific CD8+ T Cell Response in Patients With Metastatic Esophageal Squamous Cell Carcinoma. Cancer Sci (2010) 101(12):2510–7. doi: 10.1111/j.1349-7006.2010.01732.x
8. Yamamoto TN, Kishton RJ, Restifo NP. Developing Neoantigen-Targeted T Cell-Based Treatments for Solid Tumors. Nat Med (2019) 25(10):1488–99. doi: 10.1038/s41591-019-0596-y
9. Yu J, Qin B, Moyer AM, Nowsheen S, Tu X, Dong H, et al. Regulation of Sister Chromatid Cohesion by Nuclear PD-L1. Cell Res (2020) 30(7):590–601. doi: 10.1038/s41422-020-0315-8
10. Raimondi C, Carpino G, Nicolazzo C, Gradilone A, Gianni W, Gelibter A, et al. PD-L1 and Epithelial-Mesenchymal Transition in Circulating Tumor Cells From Non-Small Cell Lung Cancer Patients: A Molecular Shield to Evade Immune System? Oncoimmunology (2017) 6(12):e1315488. doi: 10.1080/2162402x.2017.1315488
11. Larkin J, Chiarion-Sileni V, Gonzalez R, Grob JJ, Rutkowski P, Lao CD, et al. Five-Year Survival With Combined Nivolumab and Ipilimumab in Advanced Melanoma. N Engl J Med (2019) 381(16):1535–46. doi: 10.1056/NEJMoa1910836
12. Hellmann MD, Paz-Ares L, Bernabe Caro R, Zurawski B, Kim SW, Carcereny Costa E, et al. Nivolumab Plus Ipilimumab in Advanced Non-Small-Cell Lung Cancer. N Engl J Med (2019) 381(21):2020–31. doi: 10.1056/NEJMoa1910231
13. Kelly RJ, Ajani JA, Kuzdzal J, Zander T, Van Cutsem E, Piessen G, et al. Adjuvant Nivolumab in Resected Esophageal or Gastroesophageal Junction Cancer. N Engl J Med (2021) 384(13):1191–203. doi: 10.1056/NEJMoa2032125
14. Kojima T, Shah MA, Muro K, Francois E, Adenis A, Hsu CH, et al. Randomized Phase III KEYNOTE-181 Study of Pembrolizumab Versus Chemotherapy in Advanced Esophageal Cancer. J Clin Oncol (2020) 38(35):4138–48. doi: 10.1200/jco.20.01888
15. Garcia-Garijo A, Fajardo CA, Gros A. Determinants for Neoantigen Identification. Front Immunol (2019) 10:1392. doi: 10.3389/fimmu.2019.01392
16. Secrier M, Li X, de Silva N, Eldridge MD, Contino G, Bornschein J, et al. Mutational Signatures in Esophageal Adenocarcinoma Define Etiologically Distinct Subgroups With Therapeutic Relevance. Nat Genet (2016) 48(10):1131–41. doi: 10.1038/ng.3659
17. Gao YB, Chen ZL, Li JG, Hu XD, Shi XJ, Sun ZM, et al. Genetic Landscape of Esophageal Squamous Cell Carcinoma. Nat Genet (2014) 46(10):1097–102. doi: 10.1038/ng.3076
18. Peng M, Mo Y, Wang Y, Wu P, Zhang Y, Xiong F, et al. Neoantigen Vaccine: An Emerging Tumor Immunotherapy. Mol Cancer (2019) 18(1):128. doi: 10.1186/s12943-019-1055-6
19. Melero I, Gaudernack G, Gerritsen W, Huber C, Parmiani G, Scholl S, et al. Therapeutic Vaccines for Cancer: An Overview of Clinical Trials. Nat Rev Clin Oncol (2014) 11(9):509–24. doi: 10.1038/nrclinonc.2014.111
20. Zhang Y, Lin Z, Wan Y, Cai H, Deng L, Li R. The Immunogenicity and Anti-Tumor Efficacy of a Rationally Designed Neoantigen Vaccine for B16F10 Mouse Melanoma. Front Immunol (2019) 10:2472. doi: 10.3389/fimmu.2019.02472
21. Oshima Y, Shimada H, Yajima S, Nanami T, Matsushita K, Nomura F, et al. NY-ESO-1 Autoantibody as a Tumor-Specific Biomarker for Esophageal Cancer: Screening in 1969 Patients With Various Cancers. J Gastroenterol (2016) 51(1):30–4. doi: 10.1007/s00535-015-1078-8
22. Wada H, Sato E, Uenaka A, Isobe M, Kawabata R, Nakamura Y, et al. Analysis of Peripheral and Local Anti-Tumor Immune Response in Esophageal Cancer Patients After NY-ESO-1 Protein Vaccination. Int J Cancer (2008) 123(10):2362–9. doi: 10.1002/ijc.23810
23. Kageyama S, Wada H, Muro K, Niwa Y, Ueda S, Miyata H, et al. Dose-Dependent Effects of NY-ESO-1 Protein Vaccine Complexed With Cholesteryl Pullulan (CHP-NY-ESO-1) on Immune Responses and Survival Benefits of Esophageal Cancer Patients. J Transl Med (2013) 11:246. doi: 10.1186/1479-5876-11-246
24. Kageyama S, Nagata Y, Ishikawa T, Abe T, Murakami M, Kojima T, et al. Randomized Phase II Clinical Trial of NY-ESO-1 Protein Vaccine Combined With Cholesteryl Pullulan (CHP-NY-ESO-1) in Resected Esophageal Cancer Patients. Ann Oncol (2019) 30(Suppl 5):1214. doi: 10.1093/annonc/mdz253.040
25. Valmori D, Souleimanian NE, Tosello V, Bhardwaj N, Adams S, O’Neill D, et al. Vaccination With NY-ESO-1 Protein and CpG in Montanide Induces Integrated Antibody/Th1 Responses and CD8 T Cells Through Cross-Priming. Proc Natl Acad Sci USA (2007) 104(21):8947–52. doi: 10.1073/pnas.0703395104
26. Davis ID, Chen W, Jackson H, Parente P, Shackleton M, Hopkins W, et al. Recombinant NY-ESO-1 Protein With ISCOMATRIX Adjuvant Induces Broad Integrated Antibody and CD4(+) and CD8(+) T Cell Responses in Humans. Proc Natl Acad Sci USA (2004) 101(29):10697–702. doi: 10.1073/pnas.0403572101
27. Ishihara M, Tono Y, Miyahara Y, Muraoka D, Harada N, Kageyama S, et al. First-In-Human Phase I Clinical Trial of the NY-ESO-1 Protein Cancer Vaccine With NOD2 and TLR9 Stimulants in Patients With NY-ESO-1-Expressing Refractory Solid Tumors. Cancer Immunol Immunother (2020) 69(4):663–75. doi: 10.1007/s00262-020-02483-1
28. Rosenberg SA, Dudley ME. Cancer Regression in Patients With Metastatic Melanoma After the Transfer of Autologous Antitumor Lymphocytes. Proc Natl Acad Sci USA (2004) 101(Suppl 2):14639–45. doi: 10.1073/pnas.0405730101
29. Forghanifard MM, Gholamin M, Moaven O, Farshchian M, Ghahraman M, Aledavood A, et al. Neoantigen in Esophageal Squamous Cell Carcinoma for Dendritic Cell-Based Cancer Vaccine Development. Med Oncol (2014) 31(10):191. doi: 10.1007/s12032-014-0191-5
30. Lauss M, Donia M, Harbst K, Andersen R, Mitra S, Rosengren F, et al. Mutational and Putative Neoantigen Load Predict Clinical Benefit of Adoptive T Cell Therapy in Melanoma. Nat Commun (2017) 8(1):1738. doi: 10.1038/s41467-017-01460-0
31. Raje N, Berdeja J, Lin Y, Siegel D, Jagannath S, Madduri D, et al. Anti-BCMA CAR T-Cell Therapy bb2121 in Relapsed or Refractory Multiple Myeloma. N Engl J Med (2019) 380(18):1726–37. doi: 10.1056/NEJMoa1817226
32. Kageyama S, Ikeda H, Miyahara Y, Imai N, Ishihara M, Saito K, et al. Adoptive Transfer of MAGE-A4 T-Cell Receptor Gene-Transduced Lymphocytes in Patients With Recurrent Esophageal Cancer. Clin Cancer Res (2015) 21(10):2268–77. doi: 10.1158/1078-0432.Ccr-14-1559
33. Kato T, Matsuda T, Ikeda Y, Park JH, Leisegang M, Yoshimura S, et al. Effective Screening of T Cells Recognizing Neoantigens and Construction of T-Cell Receptor-Engineered T Cells. Oncotarget (2018) 9(13):11009–19. doi: 10.18632/oncotarget.24232
34. Shi H, Yu F, Mao Y, Ju Q, Wu Y, Bai W, et al. EphA2 Chimeric Antigen Receptor-Modified T Cells for the Immunotherapy of Esophageal Squamous Cell Carcinoma. J Thorac Dis (2018) 10(5):2779–88. doi: 10.21037/jtd.2018.04.91
35. Matsuda T, Takeuchi H, Matsuda S, Hiraiwa K, Miyasho T, Okamoto M, et al. EpCAM, a Potential Therapeutic Target for Esophageal Squamous Cell Carcinoma. Ann Surg Oncol (2014) 21(Suppl 3):S356–64. doi: 10.1245/s10434-014-3579-8
36. Kiesgen S, Chicaybam L, Chintala NK, Adusumilli PS. Chimeric Antigen Receptor (CAR) T-Cell Therapy for Thoracic Malignancies. J Thorac Oncol (2018) 13(1):16–26. doi: 10.1016/j.jtho.2017.10.001
37. Chandran SS, Somerville RPT, Yang JC, Sherry RM, Klebanoff CA, Goff SL, et al. Treatment of Metastatic Uveal Melanoma With Adoptive Transfer of Tumour-Infiltrating Lymphocytes: A Single-Centre, Two-Stage, Single-Arm, Phase 2 Study. Lancet Oncol (2017) 18(6):792–802. doi: 10.1016/s1470-2045(17)30251-6
38. Khammari A, Nguyen JM, Leccia MT, Guillot B, Saiagh S, Pandolfino MC, et al. Tumor Infiltrating Lymphocytes as Adjuvant Treatment in Stage III Melanoma Patients With Only One Invaded Lymph Node After Complete Resection: Results From a Multicentre, Randomized Clinical Phase III Trial. Cancer Immunol Immunother (2020) 69(8):1663–72. doi: 10.1007/s00262-020-02572-1
39. Aoki Y, Takakuwa K, Kodama S, Tanaka K, Takahashi M, Tokunaga A, et al. Use of Adoptive Transfer of Tumor-Infiltrating Lymphocytes Alone or in Combination With Cisplatin-Containing Chemotherapy in Patients With Epithelial Ovarian Cancer. Cancer Res (1991) 51(7):1934–9.
40. Fujita K, Ikarashi H, Takakuwa K, Kodama S, Tokunaga A, Takahashi T, et al. Prolonged Disease-Free Period in Patients With Advanced Epithelial Ovarian Cancer After Adoptive Transfer of Tumor-Infiltrating Lymphocytes. Clin Cancer Res (1995) 1(5):501–7.
41. Yagi T, Baba Y, Ishimoto T, Iwatsuki M, Miyamoto Y, Yoshida N, et al. PD-L1 Expression, Tumor-Infiltrating Lymphocytes, and Clinical Outcome in Patients With Surgically Resected Esophageal Cancer. Ann Surg (2019) 269(3):471–8. doi: 10.1097/sla.0000000000002616
42. Tan Q, Zhang C, Yang W, Liu Y, Heyilimu P, Feng D, et al. Isolation of T Cell Receptor Specifically Reactive With Autologous Tumour Cells From Tumour-Infiltrating Lymphocytes and Construction of T Cell Receptor Engineered T Cells for Esophageal Squamous Cell Carcinoma. J Immunother Cancer (2019) 7(1):232. doi: 10.1186/s40425-019-0709-7
43. Rosenthal R, Cadieux EL, Salgado R, Bakir MA, Moore DA, Hiley CT, et al. Neoantigen-Directed Immune Escape in Lung Cancer Evolution. Nature (2019) 567(7749):479–85. doi: 10.1038/s41586-019-1032-7
44. Li L, Goedegebuure SP, Gillanders WE. Preclinical and Clinical Development of Neoantigen Vaccines. Ann Oncol (2017) 28(suppl_12):xii11–xii7. doi: 10.1093/annonc/mdx681
45. Ichiki Y, Hanagiri T, Takenoyama M, Baba T, Nagata Y, Mizukami M, et al. Differences in Sensitivity to Tumor-Specific CTLs Between Primary and Metastatic Esophageal Cancer Cell Lines Derived From the Same Patient. Surg Today (2012) 42(3):272–9. doi: 10.1007/s00595-011-0083-7
46. Wang M, Zhao J, Zhang L, Wei F, Lian Y, Wu Y, et al. Role of Tumor Microenvironment in Tumorigenesis. J Cancer (2017) 8(5):761–73. doi: 10.7150/jca.17648
47. Jiang X, Wang J, Deng X, Xiong F, Ge J, Xiang B, et al. Role of the Tumor Microenvironment in PD-L1/PD-1-Mediated Tumor Immune Escape. Mol Cancer (2019) 18(1):10. doi: 10.1186/s12943-018-0928-4
48. Karyampudi L, Lamichhane P, Scheid AD, Kalli KR, Shreeder B, Krempski JW, et al. Accumulation of Memory Precursor CD8 T Cells in Regressing Tumors Following Combination Therapy With Vaccine and Anti-PD-1 Antibody. Cancer Res (2014) 74(11):2974–85. doi: 10.1158/0008-5472.Can-13-2564
49. Ali OA, Lewin SA, Dranoff G, Mooney DJ. Vaccines Combined With Immune Checkpoint Antibodies Promote Cytotoxic T-Cell Activity and Tumor Eradication. Cancer Immunol Res (2016) 4(2):95–100. doi: 10.1158/2326-6066.Cir-14-0126
50. Soares KC, Rucki AA, Wu AA, Olino K, Xiao Q, Chai Y, et al. PD-1/PD-L1 Blockade Together With Vaccine Therapy Facilitates Effector T-Cell Infiltration Into Pancreatic Tumors. J Immunother (2015) 38(1):1–11. doi: 10.1097/cji.0000000000000062
51. Rekoske BT, Smith HA, Olson BM, Maricque BB, McNeel DG. PD-1 or PD-L1 Blockade Restores Antitumor Efficacy Following SSX2 Epitope-Modified DNA Vaccine Immunization. Cancer Immunol Res (2015) 3(8):946–55. doi: 10.1158/2326-6066.Cir-14-0206
52. Lugade AA, Moran JP, Gerber SA, Rose RC, Frelinger JG, Lord EM. Local Radiation Therapy of B16 Melanoma Tumors Increases the Generation of Tumor Antigen-Specific Effector Cells That Traffic to the Tumor. J Immunol (2005) 174(12):7516–23. doi: 10.4049/jimmunol.174.12.7516
53. Zhang B, Bowerman NA, Salama JK, Schmidt H, Spiotto MT, Schietinger A, et al. Induced Sensitization of Tumor Stroma Leads to Eradication of Established Cancer by T Cells. J Exp Med (2007) 204(1):49–55. doi: 10.1084/jem.20062056
54. Iinuma H, Fukushima R, Inaba T, Tamura J, Inoue T, Ogawa E, et al. Phase I Clinical Study of Multiple Epitope Peptide Vaccine Combined With Chemoradiation Therapy in Esophageal Cancer Patients. J Transl Med (2014) 12:84. doi: 10.1186/1479-5876-12-84
55. Kojima T, Doi T. Immunotherapy for Esophageal Squamous Cell Carcinoma. Curr Oncol Rep (2017) 19(5):33. doi: 10.1007/s11912-017-0590-9
56. Davidson M, Chau I. Immunotherapy for Oesophagogastric Cancer. Expert Opin Biol Ther (2016) 16(10):1197–207. doi: 10.1080/14712598.2016.1213233
57. Jiang T, Shi T, Zhang H, Hu J, Song Y, Wei J, et al. Tumor Neoantigens: From Basic Research to Clinical Applications. J Hematol Oncol (2019) 12(1):93. doi: 10.1186/s13045-019-0787-5
58. Zhou C, Zhu C, Liu Q. Toward in Silico Identification of Tumor Neoantigens in Immunotherapy. Trends Mol Med (2019) 25(11):980–92. doi: 10.1016/j.molmed.2019.08.001
59. Yarchoan M, Johnson BA, Lutz ER, Laheru DA, Jaffee EM. Targeting Neoantigens to Augment Antitumour Immunity. Nat Rev Cancer (2017) 17(4):209–22. doi: 10.1038/nrc.2016.154
60. Balachandran VP, Łuksza M, Zhao JN, Makarov V, Moral JA, Remark R, et al. Identification of Unique Neoantigen Qualities in Long-Term Survivors of Pancreatic Cancer. Nature (2017) 551(7681):512–6. doi: 10.1038/nature24462
61. Torrejon DY, Abril-Rodriguez G, Champhekar AS, Tsoi J, Campbell KM, Kalbasi A, et al. Overcoming Genetically Based Resistance Mechanisms to PD-1 Blockade. Cancer Discov (2020) 10(8):1140–57. doi: 10.1158/2159-8290.Cd-19-1409
62. Kursunel MA, Esendagli G. The Untold Story of IFN-γ in Cancer Biology. Cytokine Growth Factor Rev (2016) 31:73–81. doi: 10.1016/j.cytogfr.2016.07.005
63. Arenas EJ, Martínez-Sabadell A, Rius Ruiz I, Román Alonso M, Escorihuela M, Luque A, et al. Acquired Cancer Cell Resistance to T Cell Bispecific Antibodies and CAR T Targeting HER2 Through JAK2 Down-Modulation. Nat Commun (2021) 12(1):1237. doi: 10.1038/s41467-021-21445-4
64. Grasso CS, Tsoi J, Onyshchenko M, Abril-Rodriguez G, Ross-Macdonald P, Wind-Rotolo M, et al. Conserved Interferon-γ Signaling Drives Clinical Response to Immune Checkpoint Blockade Therapy in Melanoma. Cancer Cell (2020) 38(4):500–15.e3. doi: 10.1016/j.ccell.2020.08.005
65. D’Urso CM, Wang ZG, Cao Y, Tatake R, Zeff RA, Ferrone S. Lack of HLA Class I Antigen Expression by Cultured Melanoma Cells FO-1 Due to a Defect in B2m Gene Expression. J Clin Invest (1991) 87(1):284–92. doi: 10.1172/jci114984
66. Oh E, Min B, Li Y, Lian C, Hong J, Park GM, et al. Cryopreserved Human Natural Killer Cells Exhibit Potent Antitumor Efficacy Against Orthotopic Pancreatic Cancer Through Efficient Tumor-Homing and Cytolytic Ability. Cancers (Basel) (2019) 11(7):966. doi: 10.3390/cancers11070966
67. Quail DF, Joyce JA. Microenvironmental Regulation of Tumor Progression and Metastasis. Nat Med (2013) 19(11):1423–37. doi: 10.1038/nm.3394
68. Spranger S, Spaapen RM, Zha Y, Williams J, Meng Y, Ha TT, et al. Up-Regulation of PD-L1, IDO, and T(regs) in the Melanoma Tumor Microenvironment Is Driven by CD8(+) T Cells. Sci Transl Med (2013) 5(200):200ra116. doi: 10.1126/scitranslmed.3006504
69. Eil R, Vodnala SK, Clever D, Klebanoff CA, Sukumar M, Pan JH, et al. Ionic Immune Suppression Within the Tumour Microenvironment Limits T Cell Effector Function. Nature (2016) 537(7621):539–43. doi: 10.1038/nature19364
70. Sharma P, Hu-Lieskovan S, Wargo JA, Ribas A. Primary, Adaptive, and Acquired Resistance to Cancer Immunotherapy. Cell (2017) 168(4):707–23. doi: 10.1016/j.cell.2017.01.017
Keywords: esophageal cancer, immunotherapy, neoantigen, cancer vaccine, adoptive cell therapy
Citation: Gu Y-M, Zhuo Y, Chen L-Q and Yuan Y (2021) The Clinical Application of Neoantigens in Esophageal Cancer. Front. Oncol. 11:703517. doi: 10.3389/fonc.2021.703517
Received: 30 April 2021; Accepted: 08 July 2021;
Published: 27 July 2021.
Edited by:
Jian-Guo Zhou, University of Erlangen Nuremberg, GermanyReviewed by:
Enrique J. Arenas, Vall d’Hebron Institute of Oncology (VHIO), SpainMatthias Bozza, German Cancer Research Center (DKFZ), Germany
Copyright © 2021 Gu, Zhuo, Chen and Yuan. This is an open-access article distributed under the terms of the Creative Commons Attribution License (CC BY). The use, distribution or reproduction in other forums is permitted, provided the original author(s) and the copyright owner(s) are credited and that the original publication in this journal is cited, in accordance with accepted academic practice. No use, distribution or reproduction is permitted which does not comply with these terms.
*Correspondence: Yong Yuan, eW9uZ3l1YW5Ac2N1LmVkdS5jbg==
†These authors have contributed equally to this work and share first authorship