- 1Department of Anesthesiology, The First Affiliated Hospital of Anhui Medical University, Hefei, China
- 2Ambulatory Surgery Center, The First Affiliated Hospital of Anhui Medical University, Hefei, China
Cancer is a leading contributor to deaths worldwide. Surgery is the primary treatment for resectable cancers. Nonetheless, it also results in inflammatory response, angiogenesis, and stimulated metastasis. Local anesthetic lidocaine can directly and indirectly effect different cancers. The direct mechanisms are inhibiting proliferation and inducing apoptosis via regulating PI3K/AKT/mTOR and caspase-dependent Bax/Bcl2 signaling pathways or repressing cytoskeleton formation. Repression invasion, migration, and angiogenesis through influencing the activation of TNFα-dependent, Src-induced AKT/NO/ICAM and VEGF/PI3K/AKT signaling pathways. Moreover, the indirect influences are immune regulation, anti-inflammation, and postoperative pain relief. This review summarizes the latest evidence that revealed potential clinical benefits of lidocaine in cancer treatment to explore the probable molecular mechanisms and the appropriate dose.
Introduction
Cancer remains a major cause of human death worldwide, with increasing mortality and incidence as the population ages, greatly endangering human health (1, 2). In 2017, tracheal, bronchus, lung cancer, colorectal cancer, breast cancer, and prostate cancer were the four primary reasons for cancer-related deaths in humans, which also were the main causes of cancer disability-adjusted life-years (3). Moreover, the number of deaths caused by cancer was 14 million in 2012, was estimated to reach about 9.6 million in 2018, the incidence of new cancer cases was calculated to 18.1 million in 2018 and expected to reach 24 million and 34 million by 2035 and 2050 overall global (4–6). Thus, cancer is universally considered as a great challenge and threat to global human health, with a wider social and economic burden worldwide (7). Thus, improvements in investigations and treatments are urgently needed.
Surgery is essential for global cancer therapy, especially solid organ cancers, which has a long and distinguished history. It plays a vital role in cancer prevention, diagnosis, treatment, and reconstruction (8, 9). Previous evidence indicated that more than 80% of patients with cancer may require surgery for the removal in 2015. The number of surgical procedures is expected to reach about 45 million annually worldwide by 2030 (10, 11). However, long-term cancer outcomes after surgery have not significantly improved as expectantly, conversely, tumor recurrence and metastasis may be enhanced and accelerated by surgical removal thereby causing higher mortality in comparison with the primary tumor (12, 13). Recurrence and metastatic diseases following surgical resection are reported in numerous studies, involving many molecules and elements. Demicheli and his colleagues first revealed that tumor growth may be promoted by postoperative trauma and inflammation, involving in many growth factors such as Vascular endothelial growth factor (VEGF), epidermal growth factor-like growth factors (EGFR), and endostatin (14, 15). The activation of VEGF and EGFR are crucial in postoperative wound healing via promoting the new angiogenesis and growth of epidermal cell, but resulting in cancer metastasis by unintentionally providing more opportunities for cancer cells to enter into vessels and enhancing their proliferation. The surgery could also open a window for the tumor cells entering into circulation known as circulating tumor cells (CTCs) by causing inflammatory response thereby finishing remote metastasis in prostate cancer (16). Tohme and colleagues demonstrated that the growth of new metastatic cancer in hepatoma was significantly enhanced for the stress after surgery via influencing the formation of neutrophil extracellular traps (NET) (17). The other mechanisms of tumor metastasis and recurrence after surgery are immunosuppression, gene mutations, and inflammation. These alterations in the tumor microenvironment were attributed to the harmful effects of surgery and were critical to tumor progression. Hence, the intervention of these changes may be an appropriate and significant approach to improve cancer outcomes (18, 19).
Lidocaine is commonly used in anesthesia management as one of a local anesthetics. Evidence from clinical and laboratory studies have suggested that lidocaine is beneficial to cancer patients by reducing cancer progression and recurrence and improving the survival ratio (20–22). The primary aim of this review is to document the conducive effects of using lidocaine during cancer surgery and outline the mechanisms of lidocaine inhibiting cancer invasion and metastasis.
Lidocaine
Lidocaine is an amide local anesthetic. The analgesic efficacy of lidocaine after operation was first investigated in 1951 in intravenous administration (23). Local administration or intravenous lidocaine (IVL) leads to better airway management in the context of general anesthesia by reducing the incidence of irritating cough and sore throat (24, 25). Recently, IVL plays an increasing critical role in “day surgery” and Enhanced Recovery After Surgery programs (26, 27). Furthermore, lidocaine is one of the leading and common researched and used local anesthetics. It is routinely administered regionally for topical or surface anesthesia, injection into sub-arachnoid space and epidural space for blocking the local motor and sensory nerves (28, 29). All of these are the reasons why we choose to investigate lidocaine, and our main purpose is to review the beneficial effects of lidocaine on cancer.
Lidocaine and Cancer
Thus far, several retrospective studies have found that lidocaine has indirect effects and direct effects on tumor progression. These effects are shown in Figure 1.
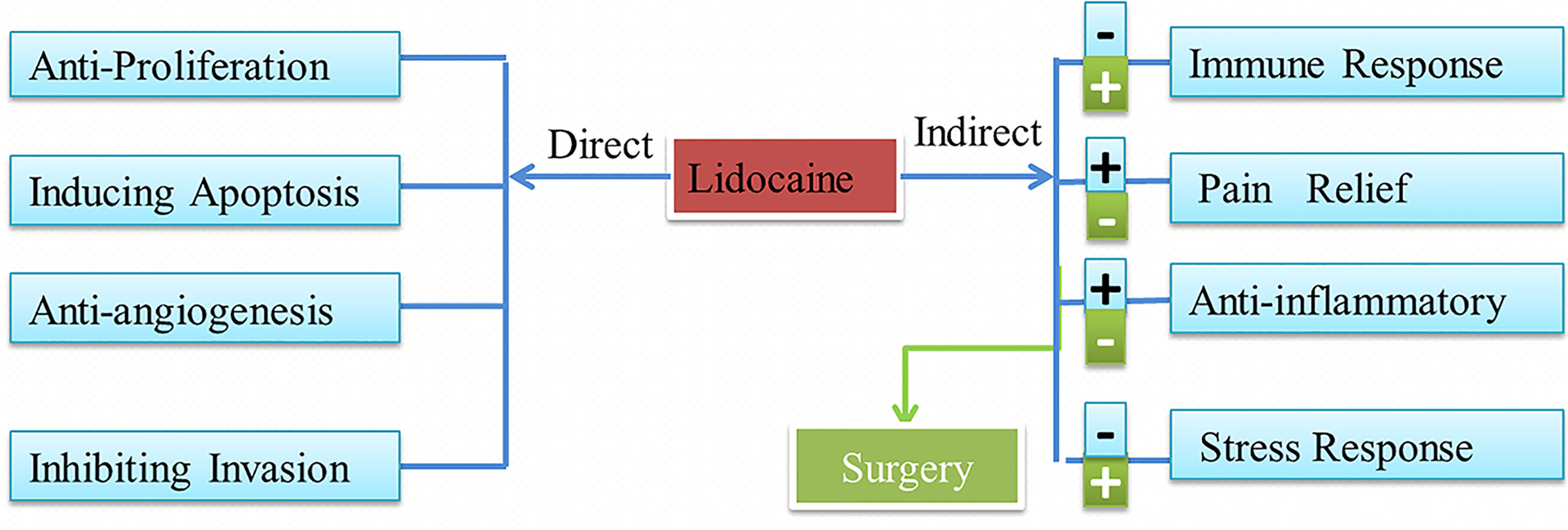
Figure 1 Lidocaine has direct and indirect effects on cancer and the influences of the surgery. Lidocaine also can inhibit the negative attack of surgery.
Indirect Effect
Lidocaine can influence the tumor microenvironment by regulating the immune and inflammatory response, alleviating the pain of surgery, and modulating the response of the neuroendocrine system. The in vitro study of Ramirez and colleagues showed that the function of natural killer (NK) cells can be enhanced by clinical concentrations of lidocaine via regulating the release of lytic granules. NK cells are one of the crucial elements of the anti-tumor immune response (30). Furthermore, in different phases of tumor development, immune and inflammatory responses play pivotal roles, including initiation, progression, malignant transformation, invasion, and metastasis (31). More importantly, Piegeler and colleagues demonstrated that lidocaine blocked metalloproteinase-9 (MMP-9) release by suppressing Src-dependent inflammatory signaling pathway at concentrations of 10 µM in vitro (32, 33). A review summarized that the inflammatory response in tumorigenesis included three parts (34):
i. Immune cells: (e.g. NK cells and neutrophils).
ii. Inflammatory entities: [e.g. cytokines, growth factors, and interleukin-6 (IL-6)].
iii. Inflammatory tumor microenvironment (e.g. fibroblasts, myeloid cells, and endothelium of new blood vessels).
Altogether, if lidocaine does have antitumor effects, its anti-inflammatory properties may have a greater effect on this process via influencing inflammatory cells, entities, and microenvironment. Although the underlying mechanisms require further investigation, previous studies have shown that these inflammation-driven changes and immune responses resemble alterations after the surgery of tumor removal that significantly contribute to tumor growth and progression (34, 35), which are suppressed by different concentrations of lidocaine. Moreover, lidocaine can also relieve pain, reduce surgical strikes, and alleviate the stress response in different cancers.
Direct Effect
Lidocaine suppresses tumor cell proliferation by acting negatively impacts on cancer cell signaling and modification of genes. A number of retrospective studies found that lidocaine inhibits the process of proliferation, the ability of invasion, migration, and induce apoptosis in several cancers (22, 36, 37). A schematic diagram of the possible mechanisms is shown in Figure 2. The study of Sun showed that the proliferation of lung cancer cells could be inhibited via regulation of miR-539/EGFR axis and decrease the activation of ERK and PI3K/AKT pathways (41). Moreover, lidocaine may induce apoptosis by promoting caspase-3 production via up-regulating the Bax and decreasing Bcl-2 associated with the signaling pathways of ERK1/2 and p38 (22). Lidocaine blocks tumor necrosis factor α (TNF-α)–dependent activity of tyrosine protein kinase (Src) via repressing function of TNF receptor 1 (TNF-R1), thereby preventing Akt and focal adhesion kinase (FAK) from activating, caveolin-1 from phosphorylating (33).
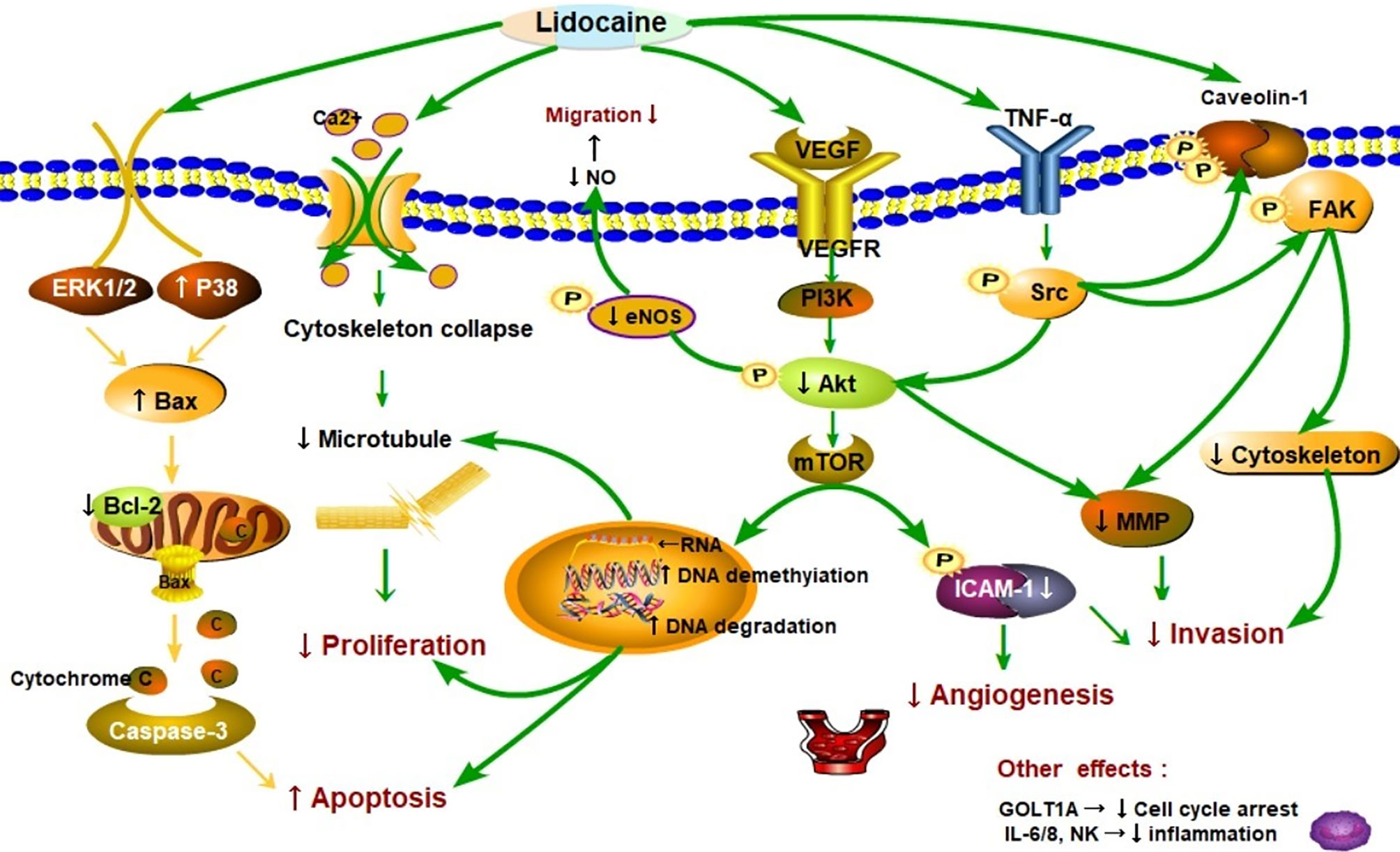
Figure 2 Schematic representation of the proposed mechanisms that lidocaine suppresses proliferation, migration, and induces apoptosis in cancer progression. As shown in the picture, lidocaine blocks tumor necrosis factor α (TNF-α)–dependent activity of Src tyrosine protein kinase (Src) via repressing function of TNF receptor 1 (TNF-R1), thereby preventing Akt kinase (Akt) and focal adhesion kinase (FAK) from activating caveolin-1 from phosphorylating (33). Moreover, lidocaine inhibited the Src-dependent intercellular adhesion molecule-1 (ICAM-1) phosphorylation to block the cancer cells adhesion and invasion (38). These signaling subways play a critical role in cancer metastasis. Cancer cells can migrate to remote sites via breaking up the cytoskeletal structure [e.g. microtubules] and releasing of matrix-metalloproteinases (MMP) (39). Lidocaine impairs cancer cells proliferation and cytoskeletal reorganization by acting on DNA methylation and repressing the (vascular endothelial growth factor) VEGF/AKT/mTOR signaling pathways (40–42). Endothelial nitric oxide synthase (eNOS) and nitric oxide (NO) generation was also inhibited by lidocaine thereby reducing vascular dilatation and directly decreasing the cells migration (38). Lidocaine depressed tumor angiogenesis via suppressing the VEGF/AKT/mTOR/ICAM-1 signaling pathway (43, 44). Consequently, AKT related signaling pathways were essential in cancer metastasis. Additionally, lidocaine may induce apoptosis by promoting caspase-3 production by up-regulating the Bax and decreasing of Bcl-2 associating with the signaling pathways of ERK1/2 and p38 (22). In particular, lidocaine may aggravate the apoptosis through directly inhibited PI3K/AKT/mTOR or influenced AKT/Bcl2/Bax signaling pathway (42, 45, 46). The other effects are related to molecules such as natural killer cells (NK) (22).
These signaling pathways play a critical role in cancer metastasis (Figure 2). Additionally, cancer cells can migrate to remote sites via breaking up the cytoskeletal structure [e.g. microtubules] and decreasing release of matrix-metalloproteinases (MMP) (39). The other effects are related to molecules such as vascular endothelial growth factor (VEGF), interleukin 6, 8 (IL6, IL8), and Golgi transport 1A (GOLT1A). The proliferation of lung cancer cells can be repressed by lidocaine via decreasing GOLT1A generation (37). In another study, Lirk and his colleagues examined that the methylation of cancer cell deoxyribonucleic acid (DNA) was inhibited by lidocaine (40). This alteration results in the re-expression of the previously hypermethylated silenced genes (e.g. tumor suppressor genes) and repression of cancer formation. Piegeler and colleagues reported that lidocaine reduced tumor cell ability of invasion by repressing MMP-9 formation and release (33). The extracellular matrix and the basal lamina can be degrade and broken up by MMP-9, thereby the cancer cells will finish invasion and remote metastasis (47, 48). In a word, lidocaine inhibits the process of proliferation, suppresses the capabilities of invasion and migration, and induces apoptosis in several cancers.
Mechanisms of Cancer Metastasis
The metastatic spread of cancer cells to distant anatomical locations is a more common cause of cancer-related death compared with primary tumor in malignant tumor (49). In addition, invasion and metastasis are the marked characteristics of a malignant tumor and also the major reasons for poor prognosis in clinical therapy (12). The three primary pathways for tumor metastasis are hematogenous, lymphatic, and implant metastasis. The potential mechanisms and relative components of tumor metastasis and recurrence are the following (50–52):
(e.g., hematogenous metastasis)
i. The adhesion between cancer cells is weakened (cell adhesion molecules, integrin family, selectin family).
ii. The cancer cells attach to the basement membrane (integrin families, β1, β2).
iii. Basement membrane degradation (fibrinolytic enzyme activators, cathepsin D, MMP, AKT).
iv. Cancer cells intravasate into blood vessels (autocrine migration factor, FAK, growth factor).
The process of cancer invasion and metastasis includes a series of steps, for example, tumor growth in situ, invasion of surrounding tissues, contact with small-vessel walls, tumor cells entering blood circulation, and growing in distant organs, in which the tumor cells entering into blood circulation and extracellular matrix (ECM) degradation are of great clinical significance (53, 54). Currently, numerous studies have revealed that Akt-dependent signaling plays a crucial role in tumor invasion and functions as a potent pro-metastatic mechanism. The study of Tian and colleagues showed that AKT-induced lncRNA VAL promotes EMT-independent metastasis via reducing Trim16-dependent Vimentin degradation (55). Moreover, PI3K/Akt pathway was demonstrated as a therapeutic target in breast cancer associated with tumor suppressor miRNA-204-5p (56). AKT excessive activation participates in many signaling pathways via regulation the expression oncogenes and tumor suppressors, such as EGFR, Ras, PI3K, BRAF, AKT itself, and natural AKT inhibitor PTEN, and is of great importance during tumor metastases and progression (57, 58). As for ECM degradation, lysosomes have been considered a pivotal element in tumor invasion and metastasis as well. It could facilitate tumor cell migration and invasion via secreting acid hydrolase thereby increasing matrix remodeling (59). Lysosomes also promote cell adhesion and influence integrin secretion through attaching to ECM and regulating the dynamics of focal adhesions, activating AKT inducing cancer and metastasis (60–62). Taken together, the process is tanglesome, but has great therapeutic potential, such repression by the lidocaine, intervention by the stress of surgery.
Lidocaine and Surgery
Surgery is the foremost treatment strategy for the majority of patients with solid tumors. Also, it is a severe attack on human, resulting in immunosuppression, angiogenesis, inflammatory response, and stimulating pain. Moreover, the more significant of the surgery the greater the surgical stress response. It is considered that surgical stress contributes to cell mediated immune system (CMI) suppression and promotes tumor progression (63). Radical treatment of cancers may inadvertently provide malignant cancer cells with chances to break down the host barriers and to form remote metastatic tumors that may denote poor prognosis (64). Surgery modulates and induces tumor metastasis, but lidocaine can inhibit activity and chemotaxis of leukocyte to the sites of surgical incision both in animal and clinical studies, which is also associated with damping of the surgery-induced generation of inflammatory cytokines (34, 65, 66). Therefore, the perioperative use of lidocaine may improve the outcomes of cancer patients by decreasing surgical strike as showed in Figure 1. At the same time, studies have shown that lidocaine can inhibit inflammation, analgesia, and angiogenesis, which can be associated with indirect effects on cancer and the negative changes after cancer surgery.
Lidocaine Indirect Influence
Repression of Immune and Inflammatory Response
Numerous researches have demonstrated the capabilities of lidocaine that could repress the inflammatory response by obstructing the secretion of inflammatory mediator and down-regulating the activation of immune cells, such as macrophages, NK cells, and neutrophils (67, 68). Studies that may predict the anti-inflammatory effects of lidocaine are shown in Table 1. They can be divided into laboratory studies and clinical studies.
Laboratory Studies
Lidocaine inhibits immune cell activation and adhesion in both in vitro and in vivo models to the site of injury. The invasion ability of cancer cells could be intercepted by lidocaine, which was related to the decrease of TNF-α. Piegeler and his colleagues showed that clinically relevant concentrations of lidocaine significantly restricted the TNFα-dependent inflammatory response by reducing activating of Src and phosphorylation of MAPK in lung cancer cells (69). Moreover, lidocaine decreased tumor cells metastasis and MMP-9 formation and release by down-regulating Src-induced immune and inflammatory signaling pathway (33). Ramirez and his colleagues showed that NK cells activation in vitro was promoted by lidocaine at clinical concentration in changing the secretions of lytic granules (30). Although investigated completely in laboratory, these research results state meaningful insights into the mechanism associated with immune and inflammatory response by which local anesthetics, such as lidocaine, might diminish metastasis.
A study using an animal model also showed that lidocaine treatment preoperative could reduce microglias initiation and genes modification of pro-inflammatory factors, and lidocaine systemic administration decreased the dead and dying neurons in the hippocampus area (70). Another study indicated that inflammatory cytokines secretions were inhibited by lidocaine in a dose-dependent manner, thereby providing protection of anti-inflammatory for mice (71). In a murine model, Johnson and colleagues (73) found that compared with general anesthesia alone, the combination of lidocaine and sevoflurane anesthesia could effectively reduce postoperative metastasis of lung cancer by inhibiting inflammatory response, angiogenesis, and surgical stress. Hence, lidocaine, whether used alone or in combination with sevoflurane, may certainly have beneficial effects on reducing cancer metastasis. The anesthesia method of combining lidocaine local administration and sevoflurane anesthesia inhalation is also commonly used in clinical practice. Furthermore, these animal studies may provide significant insights for clinical studies. All these previous evidence suggest that lidocaine, which is capable of attenuating the activation of immune cells, e.g., microglia and the production of inflammatory factor, e.g. IL-1β, TNF-α, thereby decreasing tumor growth.
Clinical Studies
The study of Galos and colleagues revealed that the perioperative use of lidocaine reduced the surgically stimulated activation of NETosis and secretion of MMP3 (72). The consequence explains the underlying mechanisms that IVL might decrease the invasion and metastasis after surgery. NETosis may enhance cancer cell growth and invasion, resulting in breast cancer recurrences, poor prognosis, and thrombosis (74). In conclusion, lidocaine can depress immune and inflammatory response by influencing immune cells (e.g., NK cells and macrophages), reducing the release of inflammatory entities (e.g., CA3 and IL-6), inhibiting signaling pathways (e.g., Src-dependent inflammatory signaling), and damaging the inflammatory tumor microenvironment, thereby reducing and inhibiting cancer metastasis. As the number of relevant studies, as well as clinical studies on the underlying mechanisms, is limited, further research is needed.
Alleviating the Pain of Surgery and Cancer
Clinical studies and a few laboratory studies have investigated the analgesic effects of lidocaine that are shown in Table 2. Systemic use of lidocaine has been observed to exhibit analgesic effects by promoting postoperation pain relief (84) and regulating the function of the central nervous system (77). Kawamata and colleagues showed that the incision-induced hyperalgesia in human skin can be reduced by treatment with lidocaine via suppressing the formation of superabundant pain inputs and the function of peripheral and central nerves (89). One of the most significant effects of lidocaine was analgesic effect during tumor removal and the management of cancer pain, including acute stimulations and dull pain, for the intervention of hyperpathia and the production of pain inputs. Recently, clinical studies have shown that the administration method of injecting lidocaine into epidural spaces or subcutaneous tissues was safe in controlling pain of cancer and could be effectively applied to clinical practice (82). Moreover, treatment with lidocaine, including local administration or intravenous injection, is beneficial and effective for dull pain (76), neuropathic pain (78), and acute pain (90). Nonetheless, the specific mechanism of perioperative use of lidocaine for intervention the generation and propagation of pain impulses remains unclear.
Several retrospective and randomized control trials have indicated that the intravenous or local use of lidocaine can lead to opioid saving and chronic postsurgical pain reduction for certain types of surgery, a reduced incidence of pain, and enhanced analgesic effects in cancer patients (83, 84). An in vitro study (90) demonstrated that the activities of voltage-gated sodium channels, calcium, potassium channels, and N-methyl-D-aspartic acid receptors may be inhibited by lidocaine at a low concentration, and be blocked by high concentration lidocaine. Therefore, lidocaine at different concentrations can affect transmission of pain-related electrical signals by inhibiting the activation of the relative ion channels and the function of their receptors.
Pain is common in cancer patients and considerably impairs their quality of life (91). About 30–90% of cancer patients suffer from pain, including neuropathic pain (92). Lidocaine is routinely administered regionally for topical or surface anesthesia, injection into sub-arachnoid space and epidural space to block the local motor and sensory nerves. The two ways were also applied to clinical treatment for intention of pain relief (77, 93). The intra-arterial administration of lidocaine is safely and effectively used for prevention and reduction of pain in perioperative period and results from transarterial chemoembolization of hepatocellular carcinoma (94–96). Khan and colleagues (84) showed that lidocaine persistently reduced the generation and enlargement of NP (43.1 vs 63.3%; relative risk = .68; 95% confidence interval = .47–1.0) in patients with breast cancer needing surgical treatment.
Overall, lidocaine exhibits analgesic, anti-inflammatory, and anti-hyperalgesic properties in cancer pain management. The pain-relieving and anti-inflammatory properties of lidocaine have been proven. Several studies also have shown that lidocaine could attenuate the inflammatory response for postoperative pain of surgery and long time NP of cancer, decrease the risk of cancer metastasis, and modulate the neuroendocrine stress response. All of these are indirect effects of lidocaine on cancers.
Direct Influence of Lidocaine
Suppressing Cancer Cell Proliferation and Inducing Apoptosis
Laboratory Studies In Vitro
Table 3 shows that lidocaine suppresses tumor cell proliferation though the effects on cell signaling and influences the cell cycle and the demethylation of DNA or other genes. Chang and colleagues indicated that the cell viability and colony formation in thyroid cancer cells were suppressed by lidocaine (98). Moreover, lidocaine induced tumor cell apoptosis via dose-dependent manners by destroying potential of mitochondrial membrane and decreasing the release of cytochrome c. This effect is closely associated with cell signaling of p38 mitogen-activated protein kinase (MAPK) and extracellular signal-regulated kinase 1/2 (ERK1/2), along with the production of caspase-3 and -7, and reaction of a higher ratio of Bax/Bcl-2. The signal pathway is shown in Figure 2. Ye and colleagues (112) also showed that the activation of Bcl-2 was down-regulated and the level of Bax was increased by lidocaine treatment, so Bax/Bcl-2 may be a potential mechanism of apoptosis. In addition, lidocaine suppressed the growth of HepG2 cells, interrupted cell proliferation cycle, resulted in programmed cell death with the increase of Bax protein, and promoted caspase-3 and an accompanying reduction with Bcl-2 protein in dose-dependent and time-dependent manners (22). In particular, lidocaine may aggravate the apoptosis through directly inhibited PI3K/AKT/mTOR or indirectly influenced the AKT/Bcl2/Bax signaling pathway (45, 46). By affecting these signaling pathways, lidocaine can affect the growth, metabolism, and cytoskeleton formation of cancer cells (39) thereby repressing cells’ ability of proliferation and resulting in caspase-mediated cell death.
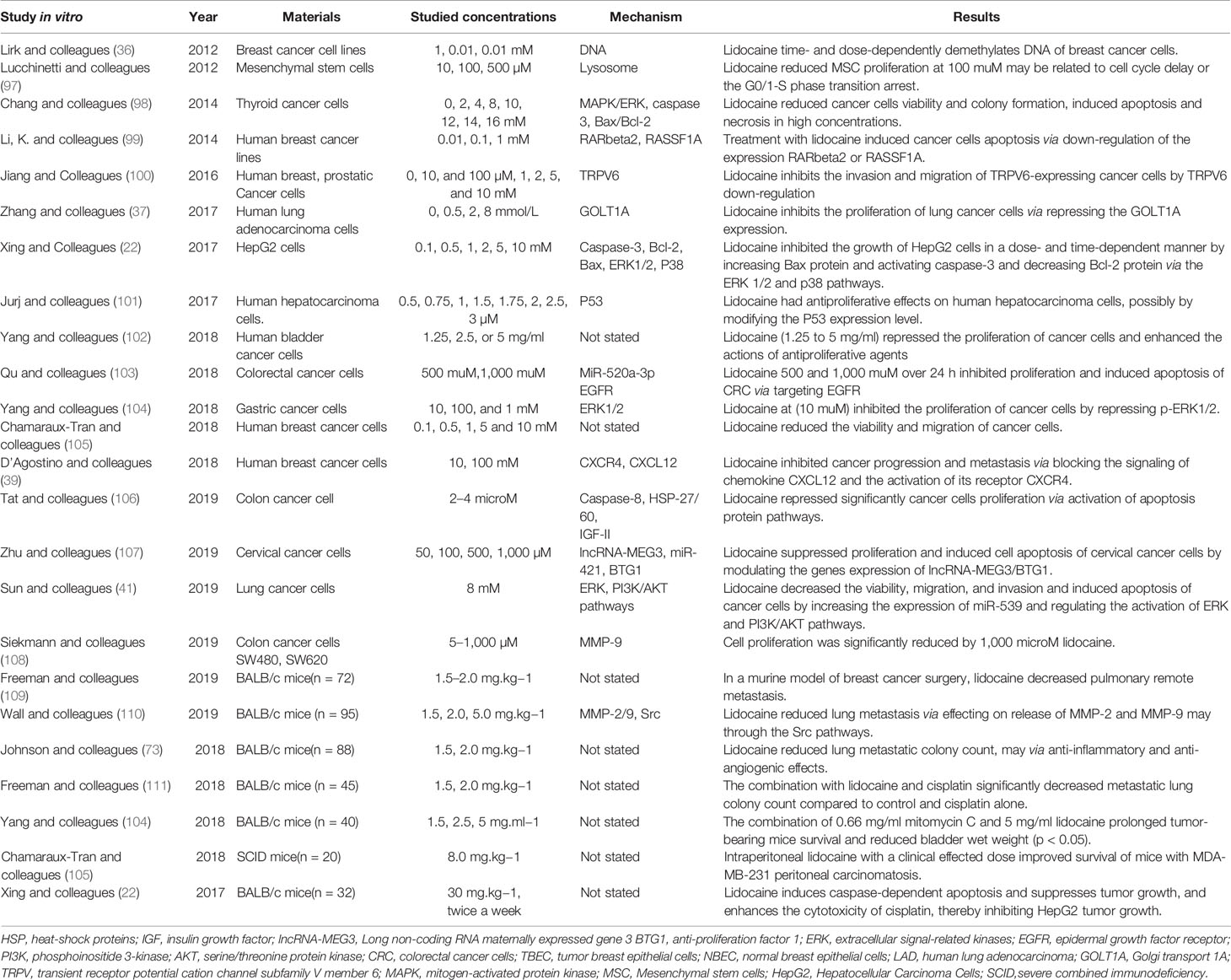
Table 3 The direct effects of lidocaine, suppressing the tumor cell proliferation, invasion and inducing apoptosis.
With regard to the changes of genes, Lirk and colleagues demonstrated that clinically relevant concentrations of lidocaine may demethylate the DNA of breast cancer cell lines in vitro (36). Lidocaine represses the activation of ERK and PI3K/AKT pathways by elevating the expression of miR-539 (41). It also strengthens the cytotoxicity of cisplatin by increasing the expression of RARbeta2 and RASSF1A demethylation in vitro study (99). Moreover, the expression of tumor suppressor gene IncRNA-MEG3 and oncogenes miR-421 were intervened by lidocaine thereby inhibiting cervical cancer cell proliferation and induces cell apoptosis (107). In malignancy, decreased methylation generally contributes to the up-regulation of tumor suppressor genes, inhibition cancer development. Therefore, these modifications caused by lidocaine mean reduction in methylation that may increase the re-expression of tumor suppressor genes and restrain cancer progression.
Laboratory Studies In Vivo
The increase of tumor in size and weight was inhibited by lidocaine in vivo by repressing cancer cells proliferation, sensitizing the cytotoxic chemotherapy drugs, and inducing programmed cell death (22). Moreover, previous study demonstrated that injecting clinical concentration of lidocaine into intraperitoneal is probably able to improve the prognosis of mice with breast cancer models, resulting in the increase of survival number (105). In animal research of cancer surgery, the remote metastasis such as lung of breast cancer could be reduced by lidocaine, which may be related to the reduction of MMP-2 and MMP-9 (109, 110). MMP-9 is a critical molecule in cancer development. Recently, a study also found that the combination administration of lidocaine and cisplatin can markedly induce caspase-mediated cell death of MCF-7 cells compared with the use of cisplatin alone.
Finally, lidocaine inhibits tumor cell proliferation by affecting the cancer cell signaling and influences the cell cycle and demethylation of DNA or other genes in vivo. Moreover, it enhances the cytotoxicity of drugs, such as cisplatin, and decreases metastasis by reducing MMP-2 and MMP-9 activation in vivo; moreover, relevant discoveries and research in clinical practice are still needed.
Inhibiting the Process of Invasion
The process of invasion is extremely complicated, which consists of a series of steps, including basement membrane degradation and invasion of surrounding tissues, contacting with small-vessel walls, and entering of tumor cells into the circulatory system. One of the most studied is basement membrane degradation. Piegeler and colleagues (33) showed that lidocaine dramatically inhibited the TNFα-dependent signaling pathway by avoiding activating/phosphorylation of Akt, FAK, and caveolin-1 (Cav1), thus attenuating MMP release and invasion in NCI-H838 cells. FAK also plays a crucial role in the process of cancer cells remote movement, involving in orienting appropriate sites, assembling/reorganizing of actin cytoskeletal, and eventually migrating of cancer cells. Cav1 is a fundamental element of construction of caveolae, which is related to adhesion and invasion of cancer cells (33, 113, 114).
The malignant cells may have analogous characteristics of secreting MMPs to neutrophils, the invasion and migration of cancer cells are enhanced and basal lamina as well as the extracellular matrix are disintegrated by MMPs, which provides tumor cells with an opportunity entering into circulatory system and finish the distant metastasis (115). Wall and colleagues (110) also demonstrated that lidocaine reduced MMP-9 and MMP-2 activities via an inhibitory effect on the Src pathway in an in vivo model. Furthermore, the study of Zhang and colleagues (116) reported that lidocaine inhibited the invasion and migration of cancer cells via down-regulating the AKT/mTOR and β-catenin pathway. The AKT/mTOR pathway was demonstrated closely associated with the activation of lysosome, the release of hydrolase, the degradation of the ECM (59, 117).
Overall, with the use of lidocaine, inhibiting the signaling pathways is perhaps an effective way of repressing the invasion of malignant cells. Furthermore, these findings can provide significant insights for further clinical studies by which lidocaine might decrease invasion and metastasis.
Depressing Tumor Angiogenesis
Angiogenesis plays a vital role in tumorigenesis and metastasis, which provides sufficient nutrient substances as well as oxygen for cancer cells, efficiently drains the metabolic waste, supplies more opportunities for cancer cell attaining remote migration (118). Moreover, the level of tumor vascularization is closely connected to the development of hematogenous metastasis as well as tumor grade (119). Previous studies have demonstrated that the VEGF and its receptor were significantly critical in angiogenesis through the AKT/PI3K signaling pathways to up-regulation of ICAM-1 eventually (120). The up-regulation ICAM-1 indicates more cancer cells and endothelial cells adhesion and migration associated with activation of VEGF and AKT/PI3K signaling pathways (121). The tumor growth and progression require angiogenesis, which inhibitions are a crucial therapeutic strategy for cancer patients. Hence, Lan and colleagues (122) (123) reported that the expression of endothelial ICAM-1 was reduced by lidocaine treatment, especially when concentrations higher than on clinically effective blood concentrations. Furthermore, the activation of VEGF-A and phosphorylation VEGFR-2 were suppressed by lidocaine, thereby decreasing the number and degree of angiogenesis on a clinically relevant concentrations without causing cell death (124). Lidocaine also inhibits endothelial cell capillary network for construction and VEGF, decreases endothelial cells to migration and propagation by interfering in the preliminary period of the formation of new blood vessels in vitro (43). Additionally, lidocaine has been reported to significantly suppress the activation of ERK and PI3K/AKT pathways (41), which are essential in VEGF secretion, eNOS phosphorylation, vasorelaxation, and angiogenesis (125). The angiogenic ability of vascular endothelial cells could be pathologically enhanced by VEGF. Moreover, VEGF as well as its receptor (VEGFR) are of great significance in anti-angiogenesis treatment (126). These findings could provide more evidence on the ability of lidocaine to inhibit cancer metastasis via repressing angiogenesis. Moreover, the underlying mechanism is partly associated with down-regulation of PI3K/AKT pathways, VEGF and ICAM-1.
Relationship Between Direct and Indirect Effects
Cancer metastasis is closely related to the microenvironment and involves the interaction of surrounding non-cancerous stromal cells, immune system cells (127), extracellular matrix, chemokines, cytokines, and other factors (18). This fragile microenvironment is easily disrupted by surgical procedures; anxiety of surgery creates a window for tumor cells metastasis, leading to immunosuppression, angiogenesis, inflammatory response, and stimulated pain (12). The previous studies also indicated that the development of cancer could be promoted by the inflammatory immune response postoperation for pain and surgical attack (12). Therefore, to investigate the relationship between lidocaine, cancer metastasis, and surgery, ideas for future studies on cancer and anesthetic drugs, as well as for the selection of clinical anesthesia methods and the treatment of cancer are needed.
The cancer microenvironment is extremely complex, with various mechanisms involved. Moreover, as the direct and indirect effects of lidocaine are interactive, they cannot be separated directly. Thus far, clinical studies on the ability of lidocaine to suppress proliferation and induce apoptosis are few, and most of these are relevant to the effects of enhancing pain relief (Table 1) (128). So more studies are still required to investigate the specific mechanisms. Data from recent animal and cell studies, to some extent, have explained the proposed mechanisms of lidocaine (Table 2), which may be closely correlated to the effects on cancer cell signaling, decreasing immune and inflammatory disruption, and gene modification and instability. Nonetheless, the concentration of lidocaine used in laboratory studies is generally higher than that used in clinical therapy, and lidocaine may be cytotoxic at high concentration (22, 129). Consequently, it may be inappropriate to directly apply the dosage of experimental study to clinical treatment, and it is hard to obtain similar laboratory results in clinical trials. Hence, clinical studies are still needed to determine the appropriate concentration of lidocaine.
Conclusions
In conclusion, some relevant investigations have demonstrated connections among perioperative events such as lidocaine treatment, surgical stress, and cancer progression. This review summarized and investigated the underlying mechanisms of lidocaine effects on cancer metastasis and recurrence after surgery, and explained the beneficial properties of lidocaine in cancer prognosis which provided some ideas for the clinical treatment and research of cancer. The specific mechanisms of these effects are dependent on further studies.
Author Contributions
CZ and CX reviewed the literature and drafted the article. YL carefully revised the final manuscript and provided suggestions to improve it. All authors contributed to the article and approved the submitted version.
Funding
This work is supported by the National Natural Science Foundation of China (No. 81770295) and the Natural Science Foundation of Anhui Province for Outstanding Youth (2008085J34).
Conflict of Interest
The authors declare that the research was conducted in the absence of any commercial or financial relationships that could be construed as a potential conflict of interest.
Acknowledgments
The authors thank Qiuyue Wang, MD (Department of Anesthesiology, The First Affiliated Hospital of Anhui Medical University, Hefei 230022, China) for her great assistance.
References
1. McGuire S. World Cancer Report 2014. Geneva, Switzerland: World Health Organization, International Agency for Research on Cancer, Who Press, 2015. Adv Nutr (2016) 7:418–19. doi: 10.3945/an.116.012211
2. Anisimov VN, Sidorenko AV. It is Necessary to Establish an International Agency for Research on Aging. Aging (Albany NY) (2018) 10:863–67. doi: 10.18632/aging.101451
3. Fitzmaurice C, Abate D, Abbasi N, Abbastabar H, Abd-Allah F, Abdel–Rahman O, et al. Global, Regional, and National Cancer Incidence, Mortality, Years of Life Lost, Years Lived With Disability, and Disability-Adjusted Life-Years for 29 Cancer Groups, 1990 to 2017: A Systematic Analysis for the Global Burden of Disease Study. JAMA Oncol (2019) 5:1749–68. doi: 10.1001/jamaoncol.2019.2996
4. Bray F, Ferlay J, Soerjomataram I, Siegel mataram RL, Torre LA, Jemal A. Global Cancer Statistics 2018: GLOBOCAN Estimates of Incidence and Mortality Worldwide for 36 Cancers in 185 Countries. CA Cancer J Clin (2018) 68:394–424. doi: 10.3322/caac.21492
5. Pilleron S, Sarfati D, Janssen-Heijnen M, Vignat J, Ferlay J, Bray F, et al. Global Cancer Incidence in Older Adults, 2012 and 2035: A Population-Based Study. Int J Cancer (2019) 144:49–58. doi: 10.1002/ijc.31664
6. Pilleron S, Soto-Perez-de-Celis E, Vignat J, Ferlay J, Soerjomataram I, Bray F, et al. Estimated Global Cancer Incidence in the Oldest Adults in 2018 and Projections to 2050. Int J Cancer (2021) 148:601–08. doi: 10.1002/ijc.33232
7. Ganczak M, Miazgowski T, Kozybska M, Kotwas A, Korzen M, Rudnicki B, et al. Changes in Disease Burden in Poland Between 1990-2017 in Comparison With Other Central European Countries: A Systematic Analysis for the Global Burden of Disease Study 2017. PloS One (2020) 15:e226766. doi: 10.1371/journal.pone.0226766
8. Sullivan R. Proto-Surgery in Ancient Egypt. Acta Med (Hradec Kralove) (1998) 41:109–20. doi: 10.14712/18059694.2019.174
9. Chen QY, Zhong Q, Liu ZY, Huang XB, Que SJ, Zheng WZ, et al. Advances in Laparoscopic Surgery for the Treatment of Advanced Gastric Cancer in China. Eur J Surg Oncol (2020) 46:e7–13. doi: 10.1016/j.ejso.2020.07.015
10. Meara JG, Greenberg SL. The Lancet Commission on Global Surgery Global Surgery 2030: Evidence and Solutions for Achieving Health, Welfare and Economic Development. Surgery (2015) 157:834–35. doi: 10.1016/j.surg.2015.02.009
11. Sullivan R, Alatise OI, Anderson BO, Audisio R, Autier P, Aggarwal A, et al. Global Cancer Surgery: Delivering Safe, Affordable, and Timely Cancer Surgery. Lancet Oncol (2015) 16:1193–224. doi: 10.1016/S1470-2045(15)00223-5
12. Hiller JG, Perry NJ, Poulogiannis G, Riedel B, Sloan EK. Perioperative Events Influence Cancer Recurrence Risk After Surgery. Nat Rev Clin Oncol (2018) 15:205–18. doi: 10.1038/nrclinonc.2017.194
13. Mehlen P, Puisieux A. Metastasis: A Question of Life or Death. Nat Rev Cancer (2006) 6:449–58. doi: 10.1038/nrc1886
14. Demicheli R, Retsky MW, Hrushesky WJ, Baum M, Gukas ID. The Effects of Surgery on Tumor Growth: A Century of Investigations. Ann Oncol (2008) 19:1821–28. doi: 10.1093/annonc/mdn386
15. Wu FP, Hoekman K, Meijer S, Cuesta MA. VEGF and Endostatin Levels in Wound Fluid and Plasma After Breast Surgery. Angiogenesis (2003) 6:255–57. doi: 10.1023/B:AGEN.0000029410.32264.b0
16. Pak S, Suh YS, Lee DE, Kim SH, Joung JY, Park WS, et al. Association Between Postoperative Detection of Circulating Tumor Cells and Recurrence in Patients With Prostate Cancer. J Urol (2020) 203:1128–34. doi: 10.1097/JU.0000000000000704
17. Tohme S, Yazdani HO, Al-Khafaji AB, Chidi AP, Loughran P, Mowen K, et al. Neutrophil Extracellular Traps Promote the Development and Progression of Liver Metastases After Surgical Stress. Cancer Res (2016) 76:1367–80. doi: 10.1158/0008-5472.CAN-15-1591
18. Casey SC, Amedei A, Aquilano K, Azmi AS, Benencia F, Bhakta D, et al. Cancer Prevention and Therapy Through the Modulation of the Tumor Microenvironment. Semin Cancer Biol (2015) 35(Suppl):S199–223. doi: 10.1016/j.semcancer.2015.02.007
19. Zubair H, Khan MA, Anand S, et al. Modulation of the Tumor Microenvironment by Natural Agents: Implications for Cancer Prevention and Therapy. Semin Cancer Biol (2020). doi: 10.1016/j.semcancer.2020.05.009
20. Wigmore TJ, Mohammed K, Jhanji S. Long-Term Survival for Patients Undergoing Volatile Versus IV Anesthesia for Cancer Surgery: A Retrospective Analysis. Anesthesiology (2016) 124:69–79. doi: 10.1097/ALN.0000000000000936
21. Xuan W, Hankin J, Zhao H, Yao S, Ma D. The Potential Benefits of the Use of Regional Anesthesia in Cancer Patients. Int J Cancer (2015) 137:2774–84. doi: 10.1002/ijc.29306
22. Xing W, Chen DT, Pan JH, Chen YH, Yan Y, Li Q, et al. Lidocaine Induces Apoptosis and Suppresses Tumor Growth in Human Hepatocellular Carcinoma Cells In Vitro and in a Xenograft Model In Vivo. Anesthesiology (2017) 126:868–81. doi: 10.1097/ALN.0000000000001528
23. Gilbert CR, Hanson IR, Brown AB, Hingson RA. Intravenous Use of Xylocaine. Curr Res Anesth Analg (1951) 30:301–13. doi: 10.1213/00000539-195101000-00057
24. Subedi A, Tripathi M, Pokharel K, Khatiwada S. Effect of Intravenous Lidocaine, Dexamethasone, and Their Combination on Postoperative Sore Throat: A Randomized Controlled Trial. Anesth Analg (2019) 129:220–25. doi: 10.1213/ANE.0000000000003842
25. Li H, Yue Y, Qu Y, Mu D. Lidocaine for Postoperative Sore Throat: A Meta-Analysis of Randomized Controlled Trials. Minerva Anestesiol (2020) 86:546–53. doi: 10.23736/S0375-9393.20.14170-1
26. Helander EM, Webb MP, Bias M, Whang EE, Kaye AD, Urman RD. A Comparison of Multimodal Analgesic Approaches in Institutional Enhanced Recovery After Surgery Protocols for Colorectal Surgery: Pharmacological Agents. J Laparoendosc Adv Surg Tech A (2017) 27:903–08. doi: 10.1089/lap.2017.0338
27. Dunn LK, Durieux ME. Perioperative Use of Intravenous Lidocaine. Anesthesiology (2017) 126:729–37. doi: 10.1097/ALN.0000000000001527
28. Biki B, Mascha E, Moriarty DC, Fitzpatrick JM, Sessler DI, Buggy DJ. Anesthetic Technique for Radical Prostatectomy Surgery Affects Cancer Recurrence: A Retrospective Analysis. Anesthesiology (2008) 109:180–87. doi: 10.1097/ALN.0b013e31817f5b73
29. Herminghaus A, Wachowiak M, Wilhelm W, Gottschalk A, Eggert K, Gottschalk A. Intravenous Administration of lidocaine for Perioperative Analgesia. Review and Recommendations for Practical Usage. Anaesthesist (2011) 60:152–60. doi: 10.1007/s00101-010-1829-0
30. Ramirez MF, Tran P, Cata JP. The Effect of Clinically Therapeutic Plasma Concentrations of Lidocaine on Natural Killer Cell Cytotoxicity. Reg Anesth Pain Med (2015) 40:43–8. doi: 10.1097/AAP.0000000000000191
31. Coussens LM, Werb Z. Inflammation and Cancer. Nature (2002) 420:860–67. doi: 10.1038/nature01322
32. Grivennikov SI, Greten FR, Karin M. Immunity, Inflammation, and Cancer. Cell (2010) 140:883–99. doi: 10.1016/j.cell.2010.01.025
33. Piegeler T, Schlapfer M, Dull RO, Schwartz DE, Borgeat A, Minshall RD, et al. Clinically Relevant Concentrations of Lidocaine and Ropivacaine Inhibit TNFalpha-induced Invasion of Lung Adenocarcinoma Cells In Vitro by Blocking the Activation of Akt and Focal Adhesion Kinase. Br J Anaesth (2015) 115:784–91. doi: 10.1093/bja/aev341
34. Greten FR, Grivennikov SI. Inflammation and Cancer: Triggers, Mechanisms, and Consequences. Immunity (2019) 51:27–41. doi: 10.1016/j.immuni.2019.06.025
35. Crusz SM, Balkwill FR. Inflammation and Cancer: Advances and New Agents. Nat Rev Clin Oncol (2015) 12:584–96. doi: 10.1038/nrclinonc.2015.105
36. Lirk P, Berger R, Hollmann MW, Fiegl H. Lidocaine Time- and Dose-Dependently Demethylates Deoxyribonucleic Acid in Breast Cancer Cell Lines In Vitro. Br J Anaesth (2012) 109:200–07. doi: 10.1093/bja/aes128
37. Zhang L, Hu R, Cheng Y, Wu X, Xi S, Sun Y, et al. Lidocaine Inhibits the Proliferation of Lung Cancer by Regulating the Expression of GOLT1A. Cell Prolif (2017) 50. doi: 10.1111/cpr.12364
38. Piegeler T, Votta-Velis EG, Bakhshi FR, Mao M, Carnegie G, Bonini MG, et al. Endothelial Barrier Protection by Local Anesthetics: Ropivacaine and Lidocaine Block Tumor Necrosis Factor-Alpha-Induced Endothelial Cell Src Activation. Anesthesiology (2014) 120:1414–28. doi: 10.1097/ALN.0000000000000174
39. D’Agostino G, Saporito A, Cecchinato V, Silvestri Y, Borgeat A, Anselmi L, et al. Lidocaine Inhibits Cytoskeletal Remodelling and Human Breast Cancer Cell Migration. Br J Anaesth (2018) 121:962–68. doi: 10.1016/j.bja.2018.07.015
40. Lirk P, Hollmann MW, Fleischer M, Weber NC, Fiegl H. Lidocaine and Ropivacaine, But Not Bupivacaine, Demethylate Deoxyribonucleic Acid in Breast Cancer Cells In Vitro. Br J Anaesth (2014) 113(Suppl 1):i32–38. doi: 10.1093/bja/aeu201
41. Sun H, Sun Y. Lidocaine Inhibits Proliferation and Metastasis of Lung Cancer Cell Via Regulation of miR-539/EGFR Axis. Artif Cells Nanomed Biotechnol (2019) 47:2866–74. doi: 10.1080/21691401.2019.1636807
42. Zhang Y, Jia J, Jin W, Cao J, Fu T, Ma D. Lidocaine Inhibits the Proliferation and Invasion of Hepatocellular Carcinoma by Downregulating USP14 Induced PI3K/Akt Pathway. Pathol Res Pract (2020) 216:152963. doi: 10.1016/j.prp.2020.152963
43. Gao J, Hu H, Wang X. Clinically Relevant Concentrations of Lidocaine Inhibit Tumor Angiogenesis Through Suppressing VEGF/VEGFR2 Signaling. Cancer Chemother Pharmacol (2019) 83:1007–15. doi: 10.1007/s00280-019-03815-4
44. Radisavljevic Z. AKT as Locus of Fragility in Robust Cancer System. J Cell Biochem (2008) 104:2071–77. doi: 10.1002/jcb.21777
45. Zhang X, Pang W, Liu H, Wang J. Lidocine Potentiates the Cytotoxicity of 5-Fluorouracil to Choriocarcinoma Cells by Downregulating ABC Transport Proteins Expression. J Cell Biochem (2019) 120:16533–42. doi: 10.1002/jcb.28913
46. Ediriweera MK, Tennekoon KH, Samarakoon SR. Role of the PI3K/AKT/mTOR Signaling Pathway in Ovarian Cancer: Biological and Therapeutic Significance. Semin Cancer Biol (2019) 59:147–60. doi: 10.1016/j.semcancer.2019.05.012
47. Dong H, Diao H, Zhao Y, Xu H, Pei S, Gao J. Overexpression of Matrix Metalloproteinase-9 in Breast Cancer Cell Lines Remarkably Increases the Cell Malignancy Largely Via Activation of Transforming Growth Factor Beta/SMAD Signalling. Cell Prolif (2019) 52:e12633. doi: 10.1111/cpr.12633
48. Huang H. Matrix Metalloproteinase-9 (Mmp-9) as a Cancer Biomarker and MMP-9 Biosensors: Recent Advances. Sensors (Basel) (2018) 18(10):3249. doi: 10.3390/s18103249
49. Sun T, Zhang W, Li Y, Jin Z, Du Y, Tian J, et al. Combination Immunotherapy With Cytotoxic T-Lymphocyte-Associated Antigen-4 and Programmed Death Protein-1 Inhibitors Prevents Postoperative Breast Tumor Recurrence and Metastasis. Mol Cancer Ther (2020) 19:802–11. doi: 10.1158/1535-7163.MCT-19-0495
50. Wan L, Pantel K, Kang Y. Tumor Metastasis: Moving New Biological Insights Into the Clinic. Nat Med (2013) 19:1450–64. doi: 10.1038/nm.3391
51. Gajovic N, Jurisevic M, Pantic J, Radosavljevic G, Arsenijevic N, Lukic ML, et al. Attenuation of NK Cells Facilitates Mammary Tumor Growth in Streptozotocin-Induced Diabetes in Mice. Endocr Relat Cancer (2018) 25:493–507. doi: 10.1530/ERC-17-0529
52. Lamouille S, Xu J, Derynck R. Molecular Mechanisms of Epithelial-Mesenchymal Transition. Nat Rev Mol Cell Biol (2014) 15:178–96. doi: 10.1038/nrm3758
53. Das SS, Alkahtani S, Bharadwaj P, Ansari MT, ALKahtani M, Pang Z, et al. Molecular Insights and Novel Approaches for Targeting Tumor Metastasis. Int J Pharm (2020) 585:119556. doi: 10.1016/j.ijpharm.2020.119556
54. Valastyan S, Weinberg RA. Tumor Metastasis: Molecular Insights and Evolving Paradigms. Cell (2011) 147:275–92. doi: 10.1016/j.cell.2011.09.024
55. Tian H, Lian R, Li Y, Liu C, Liang S, Li W, et al. AKT-Induced Lncrna VAL Promotes EMT-independent Metastasis Through Diminishing Trim16-dependent Vimentin Degradation. Nat Commun (2020) 11:5127. doi: 10.1038/s41467-020-18929-0
56. Hong BS, Ryu HS, Kim N, Kim J, Lee E, Moon H, et al. Tumor Suppressor Mirna-204-5p Regulates Growth, Metastasis, and Immune Microenvironment Remodeling in Breast Cancer. Cancer Res (2019) 79:1520–34. doi: 10.1158/0008-5472.CAN-18-0891
57. Mayer IA, Arteaga CL. The PI3K/AKT Pathway as a Target for Cancer Treatment. Annu Rev Med (2016) 67:11–28. doi: 10.1146/annurev-med-062913-051343
58. Dai B, Pieper RO, Li D, Wei P, Liu M, Woo SY, et al. FoxM1B Regulates NEDD4-1 Expression, Leading to Cellular Transformation and Full Malignant Phenotype in Immortalized Human Astrocytes. Cancer Res (2010) 70:2951–61. doi: 10.1158/0008-5472.CAN-09-3909
59. Dykes SS, Gray AL, Coleman DT, Saxena M, Stephens CA, Carroll JL, et al. The Arf-like Gtpase Arl8b is Essential for Three-Dimensional Invasive Growth of Prostate Cancer In Vitro and Xenograft Formation and Growth In Vivo. Oncotarget (2016) 7:31037–52. doi: 10.18632/oncotarget.8832
60. Dozynkiewicz MA, Jamieson NB, Macpherson I, Grindlay J, van den Berghe PV, von Thun A, et al. Rab25 and CLIC3 Collaborate to Promote Integrin Recycling From Late Endosomes/Lysosomes and Drive Cancer Progression. Dev Cell (2012) 22:131–45. doi: 10.1016/j.devcel.2011.11.008
61. Pu J, Guardia CM, Keren-Kaplan T, Bonifacino JS. Mechanisms and Functions of Lysosome Positioning. J Cell Sci (2016) 129:4329–39. doi: 10.1242/jcs.196287
62. Radisavljevic Z. Lysosome Activates AKT Inducing Cancer and Metastasis. J Cell Biochem (2019) 120:12123–27. doi: 10.1002/jcb.28752
63. Tavare AN, Perry NJ, Benzonana LL, Takata M, Ma D. Cancer Recurrence After Surgery: Direct and Indirect Effects of Anesthetic Agents. Int J Cancer (2012) 130:1237–50. doi: 10.1002/ijc.26448
64. Matzner P, Sorski L, Haldar R, Shaashua L, Benbenishty A, Lavon H, et al. Deleterious Synergistic Effects of Distress and Surgery on Cancer Metastasis: Abolishment Through an Integrated Perioperative Immune-Stimulating Stress-Inflammatory-Reducing Intervention. Brain Behav Immun (2019) 80:170–78. doi: 10.1016/j.bbi.2019.03.005
65. Taniguchi K, Karin M. IL-6 and Related Cytokines as the Critical Lynchpins Between Inflammation and Cancer. Semin Immunol (2014) 26:54–74. doi: 10.1016/j.smim.2014.01.001
66. Le-Wendling L, Nin O, Capdevila X. Cancer Recurrence and Regional Anesthesia: The Theories, the Data, and the Future in Outcomes. Pain Med (2016) 17:756–75. doi: 10.1111/pme.12893
67. Fischer LG, Bremer M, Coleman EJ, Conrad B, Krumm B, Gross A, et al. Local Anesthetics Attenuate Lysophosphatidic Acid-Induced Priming in Human Neutrophils. Anesth Analg (2001) 92:1041–47. doi: 10.1097/00000539-200104000-00044
68. Azuma Y, Ohura K. Immunological Modulation by Lidocaine-Epinephrine and Prilocaine-Felypressin on the Functions Related to Natural Immunity in Neutrophils and Macrophages. Curr Drug Targets Immune Endocr Metabol Disord (2004) 4:29–36. doi: 10.2174/1568008043339974
69. Piegeler T, Votta-Velis EG, Liu G, Place AT, Schwartz DE, Beck–Schimmer B, et al. Antimetastatic Potential of Amide-Linked Local Anesthetics: Inhibition of Lung Adenocarcinoma Cell Migration and Inflammatory Src Signaling Independent of Sodium Channel Blockade. Anesthesiology (2012) 117:548–59. doi: 10.1097/ALN.0b013e3182661977
70. Chiu KM, Lu CW, Lee MY, Wang MJ, Lin TY, Wang SJ. Neuroprotective and Anti-Inflammatory Effects of Lidocaine in Kainic Acid-Injected Rats. Neuroreport (2016) 27:501–07. doi: 10.1097/WNR.0000000000000570
71. Lin S, Jin P, Shao C, Lu W, Xiang Q, Jiang Z, et al. Lidocaine Attenuates Lipopolysaccharide-Induced Inflammatory Responses and Protects Against Endotoxemia in Mice by Suppressing HIF1alpha-induced Glycolysis. Int Immunopharmacol (2020) 80:106150. doi: 10.1016/j.intimp.2019.106150
72. Galos EV, Tat TF, Popa R, Efrimescu CI, Finnerty D, Buggy DJ, et al. Neutrophil Extracellular Trapping and Angiogenesis Biomarkers After Intravenous or Inhalation Anaesthesia With or Without Intravenous Lidocaine for Breast Cancer Surgery: A Prospective, Randomised Trial. Br J Anaesth (2020) 125:712–21. doi: 10.1016/j.bja.2020.05.003
73. Johnson MZ, Crowley PD, Foley AG, Xue C, Connolly C, Gallagher HC, et al. Effect of Perioperative Lidocaine on Metastasis After Sevoflurane or Ketamine-Xylazine Anaesthesia for Breast Tumour Resection in a Murine Model. Br J Anaesth (2018) 121:76–85. doi: 10.1016/j.bja.2017.12.043
74. Snoderly HT, Boone BA, Bennewitz MF. Neutrophil Extracellular Traps in Breast Cancer and Beyond: Current Perspectives on NET Stimuli, Thrombosis and Metastasis, and Clinical Utility for Diagnosis and Treatment. Breast Cancer Res (2019) 21:145. doi: 10.1186/s13058-019-1237-6
75. Kang JG, Kim MH, Kim EH, Lee SH. Intraoperative Intravenous Lidocaine Reduces Hospital Length of Stay Following Open Gastrectomy for Stomach Cancer in Men. J Clin Anesth (2012) 24:465–70. doi: 10.1016/j.jclinane.2012.02.006
76. Waraya M, Kosaka Y, Sengoku N, Kikuchi M, Nishimiya H, Enomoto T, et al. [Efficacy and Safety of Lidocaine Hydrochloride Jelly for the Treatment of Pain Caused by Breast Cancer Metastases to the Skin]. Gan To Kagaku Ryoho (2012) 39:2063–65.
77. Kern KU, Nalamachu S, Brasseur L, Zakrzewska JM. Can Treatment Success With 5% Lidocaine Medicated Plaster be Predicted in Cancer Pain With Neuropathic Components or Trigeminal Neuropathic Pain? J Pain Res (2013) 6:261–80. doi: 10.2147/JPR.S39957
78. Lopez RE. Treatment of Acute and Chronic Focal Neuropathic Pain in Cancer Patients With Lidocaine 5 % Patches. A Radiation and Oncology Department Experience. Support Care Cancer (2013) 21:1329–34. doi: 10.1007/s00520-012-1670-x
79. Garzon-Rodriguez C, Casals MM, Calsina-Berna A, Lopez–Romboli E, Porta–Sales J. Lidocaine 5 % Patches as an Effective Short-Term Co-Analgesic in Cancer Pain. Preliminary Results. Support Care Cancer (2013) 21:3153–58. doi: 10.1007/s00520-013-1948-7
80. Salas S, Auquier P, Duffaud F, Garnier SR, Deschamps M, Honore S, et al. Efficacy of Lidocaine in Patients Receiving Palliative Care With Opioid-Refractory Cancer Pain With a Neuropathic Component: Study Protocol for a Randomized Controlled Study. Trials (2014) 15:318. doi: 10.1186/1745-6215-15-318
81. Gibbons K, DeMonbrun A, Beckman EJ, Keefer P, Wagner D, Stewart M, et al. Continuous Lidocaine Infusions to Manage Opioid-Refractory Pain in a Series of Cancer Patients in a Pediatric Hospital. Pediatr Blood Cancer (2016) 63:1168–74. doi: 10.1002/pbc.25870
82. Seah D, Herschtal A, Tran H, Thakerar A, Fullerton S. Subcutaneous Lidocaine Infusion for Pain in Patients With Cancer. J Palliat Med (2017) 20:667–71. doi: 10.1089/jpm.2016.0298
83. Kendall MC, McCarthy RJ, Panaro S, Goodwin E, Bialek JM, Nader A, et al. The Effect of Intraoperative Systemic Lidocaine on Postoperative Persistent Pain Using Initiative on Methods, Measurement, and Pain Assessment in Clinical Trials Criteria Assessment Following Breast Cancer Surgery: A Randomized, Double-Blind, Placebo-Controlled Trial. Pain Pract (2018) 18:350–59. doi: 10.1111/papr.12611
84. Khan JS, Hodgson N, Choi S, Reid S, Paul JE, Hong N, et al. Perioperative Pregabalin and Intraoperative Lidocaine Infusion to Reduce Persistent Neuropathic Pain After Breast Cancer Surgery: A Multicenter, Factorial, Randomized, Controlled Pilot Trial. J Pain (2019) 20:980–93. doi: 10.1016/j.jpain.2019.02.010
85. Lee JT, Sanderson CR, Xuan W, Agar M. Lidocaine for Cancer Pain in Adults: A Systematic Review and Meta-Analysis. J Palliat Med (2019) 22:326–34. doi: 10.1089/jpm.2018.0257
86. Omar E, Wallon G, Bauer C, Axiotis G, Bouix C, Soubirou JL, et al. Evaluation of Intravenous Lidocaine in Head and Neck Cancer Surgery: Study Protocol for a Randomized Controlled Trial. Trials (2019) 20:220. doi: 10.1186/s13063-019-3303-x
87. Hasuo H, Sakuma H, Uchitani K, Ohue K, Fukunaga M. Short-Term Effects of 10% Lidocaine Ointment on Allodynia in Cancer Pain: A Randomized, Double-Blind, Placebo-Controlled Crossover Study. J Palliat Med (2019) 22:1364–69. doi: 10.1089/jpm.2019.0081
88. Toner AJ, Bailey MA, Schug SA, Corcoran TB. A Pilot Multicentre Randomised Controlled Trial of Lidocaine Infusion in Women Undergoing Breast Cancer Surgery. Anaesthesia (2021). doi: 10.1111/anae.15440
89. Kawamata M, Watanabe H, Nishikawa K, Takahashi T, Kozuka Y, Kawamata T, et al. Different Mechanisms of Development and Maintenance of Experimental Incision-Induced Hyperalgesia in Human Skin. Anesthesiology (2002) 97:550–59. doi: 10.1097/00000542-200209000-00006
90. van der Wal SE, van den Heuvel SA, Radema SA, van Berkum BF, Vaneker M, Steegers MA, et al. The In Vitro Mechanisms and In Vivo Efficacy of Intravenous Lidocaine on the Neuroinflammatory Response in Acute and Chronic Pain. Eur J Pain (2016) 20:655–74. doi: 10.1002/ejp.794
91. McGeeney BE. Adjuvant Agents in Cancer Pain. Clin J Pain (2008) 24(Suppl 10):S14–20. doi: 10.1097/AJP.0b013e31816b5976
92. Garcia DPM, Del MGF, Martinez DPP, Marti CJ, Enrech FS, Cobo DM, et al. First Evidence of Oncologic Neuropathic Pain Prevalence After Screening 8615 Cancer Patients. Results of the On Study. Ann Oncol (2011) 22:924–30. doi: 10.1093/annonc/mdq449
93. Caraceni A, Portenoy RK. An International Survey of Cancer Pain Characteristics and Syndromes. IASP Task Force on Cancer Pain. International Association for the Study of Pain. Pain (1999) 82:263–74. doi: 10.1016/s0304-3959(99)00073-1
94. Lee SH, Hahn ST, Park SH. Intraarterial Lidocaine Administration for Relief of Pain Resulting From Transarterial Chemoembolization of Hepatocellular Carcinoma: Its Effectiveness and Optimal Timing of Administration. Cardiovasc Intervent Radiol (2001) 24:368–71. doi: 10.1007/s00270-001-0073-z
95. Romano M, Giojelli A, Tamburrini O, Salvatore M. Chemoembolization for Hepatocellular Carcinoma: Effect of Intraarterial Lidocaine in Peri- and Post-Procedural Pain and Hospitalization. Radiol Med (2003) 105:350–55.
96. de la Gala F, Pineiro P, Reyes A, Simon C, Vara E, Rancan L, et al. Effect of Intraoperative Paravertebral or Intravenous Lidocaine Versus Control During Lung Resection Surgery on Postoperative Complications: A Randomized Controlled Trial. Trials (2019) 20:622. doi: 10.1186/s13063-019-3677-9
97. Lucchinetti E, Awad AE, Rahman M, Feng J, Lou PH, Zhang L, et al. Antiproliferative Effects of Local Anesthetics on Mesenchymal Stem Cells: Potential Implications for Tumor Spreading and Wound Healing. Anesthesiology (2012) 116:841–56. doi: 10.1097/ALN.0b013e31824babfe
98. Chang YC, Hsu YC, Liu CL, Huang SY, Hu MC, Cheng SP. Local Anesthetics Induce Apoptosis in Human Thyroid Cancer Cells Through the Mitogen-Activated Protein Kinase Pathway. PloS One (2014) 9:e89563. doi: 10.1371/journal.pone.0089563
99. Li K, Yang J, Han X. Lidocaine Sensitizes the Cytotoxicity of Cisplatin in Breast Cancer Cells Via Up-Regulation of RARbeta2 and RASSF1A Demethylation. Int J Mol Sci (2014) 15:23519–36. doi: 10.3390/ijms151223519
100. Jiang Y, Gou H, Zhu J, Tian S, Yu L. Lidocaine Inhibits the Invasion and Migration of TRPV6-expressing Cancer Cells by TRPV6 Downregulation. Oncol Lett (2016) 12:1164–70. doi: 10.3892/ol.2016.4709
101. Jurj A, Tomuleasa C, Tat TT, Berindan–Neagoe I, Vesa SV, Ionescu DC. Antiproliferative and Apoptotic Effects of Lidocaine on Human Hepatocarcinoma Cells. A Preliminary Study. J Gastrointestin Liver Dis (2017) 26:45–50. doi: 10.15403/jgld.2014.1121.261.juj
102. Yang X, Zhao L, Li M, Yan L, Zhang S, Mi Z, et al. Lidocaine Enhances the Effects of Chemotherapeutic Drugs Against Bladder Cancer. Sci Rep (2018) 8:598. doi: 10.1038/s41598-017-19026-x
103. Qu X, Yang L, Shi Q, Wang X, Wang D, Wu G. Lidocaine Inhibits Proliferation and Induces Apoptosis in Colorectal Cancer Cells by Upregulating mir-520a-3p and Targeting EGFR. Pathol Res Pract (2018) 214:1974–79. doi: 10.1016/j.prp.2018.09.012
104. Yang W, Cai J, Zhang H, Wang G, Jiang W. Effects of Lidocaine and Ropivacaine on Gastric Cancer Cells Through Down-regulation of ERK1/2 Phosphorylation In Vitro. Anticancer Res (2018) 38:6729–35. doi: 10.21873/anticanres.13042
105. Chamaraux-Tran TN, Mathelin C, Aprahamian M, Joshi GP, Tomasetto C, Diemunsch P, et al. Antitumor Effects of Lidocaine on Human Breast Cancer Cells: An in Vitro and In Vivo Experimental Trial. Anticancer Res (2018) 38:95–105. doi: 10.21873/anticanres.12196
106. Tat T, Jurj A, Selicean C, Pasca S, Ionescu D. Antiproliferative Effects of Propofol and Lidocaine on the Colon Adenocarcinoma Microenvironment. J Buon (2019) 24:106–15.
107. Zhu J, Han S. Lidocaine Inhibits Cervical Cancer Cell Proliferation and Induces Cell Apoptosis by Modulating the lncRNA-MEG3/miR-421/BTG1 Pathway. Am J Transl Res (2019) 11:5404–16.
108. Siekmann W, Tina E, Von Sydow AK, Gupta A, et al. Effect of Lidocaine and Ropivacaine on Primary (SW480) and Metastatic (SW620) Colon Cancer Cell Lines. Oncol Lett (2019) 18:395–401. doi: 10.3892/ol.2019.10332
109. Freeman J, Crowley PD, Foley AG, Gallagher HC, Iwasaki M, Ma D, et al. Effect of Perioperative Lidocaine, Propofol and Steroids on Pulmonary Metastasis in a Murine Model of Breast Cancer Surgery. Cancers (Basel) (2019) 11(5):613. doi: 10.3390/cancers11050613
110. Wall TP, Crowley PD, Sherwin A, et al. Effects of Lidocaine and Src Inhibition on Metastasis in a Murine Model of Breast Cancer Surgery. Cancers (Basel) (2019) 11(10):1414. doi: 10.3390/cancers11101414
111. Freeman J, Crowley PD, Foley AG, Gallagher HC, Iwasaki M, Ma D, et al. Effect of Perioperative Lidocaine and Cisplatin on Metastasis in a Murine Model of Breast Cancer Surgery. Anticancer Res (2018) 38:5599–606. doi: 10.21873/anticanres.12894
112. Ye L, Zhang Y, Chen YJ, Liu Q, et al. Anti-Tumor Effects of Lidocaine on Human Gastric Cancer Cells In Vitro. Bratisl Lek Listy (2019) 120:212–17. doi: 10.4149/BLL_2019_036
113. Gupton SL, Waterman-Storer CM. Spatiotemporal Feedback Between Actomyosin and Focal-Adhesion Systems Optimizes Rapid Cell Migration. Cell (2006) 125:1361–74. doi: 10.1016/j.cell.2006.05.029
114. Joshi B, Bastiani M, Strugnell SS, Boscher C, Parton RG, Nabi IR, et al. Phosphocaveolin-1 is a Mechanotransducer That Induces Caveola Biogenesis Via Egr1 Transcriptional Regulation. J Cell Biol (2012) 199:425–35. doi: 10.1083/jcb.201207089
115. Muller-Edenborn B, Roth-Z’Graggen B, Bartnicka K, Borgeat A, Hoos A, Borsig L, et al. Volatile Anesthetics Reduce Invasion of Colorectal Cancer Cells Through Down-Regulation of Matrix Metalloproteinase-9. Anesthesiology (2012) 117:293–301. doi: 10.1097/ALN.0b013e3182605df1
116. Zhang X, Gu G, Li X, Zhang C, et al. Lidocaine Alleviates Cisplatin Resistance and Inhibits Migration of MGC-803/DDP Cells Through Decreasing Mir-10b. Cell Cycle (2020) 19:2530–37. doi: 10.1080/15384101.2020.1809914
117. Jia R, Bonifacino JS. Lysosome Positioning Influences mTORC2 and AKT Signaling. Mol Cell (2019) 75:26–38. doi: 10.1016/j.molcel.2019.05.009
118. Zhang W, Liu JN, Tan XY. Vaccination With Xenogeneic Tumor Endothelial Proteins Isolated in Situ Inhibits Tumor Angiogenesis and Spontaneous Metastasis. Int J Cancer (2009) 125:124–32. doi: 10.1002/ijc.24362
119. Tanigawa N, Amaya H, Matsumura M, Shimomatsuya T, Horiuchi T, Muraoka R, et al. Extent of Tumor Vascularization Correlates With Prognosis and Hematogenous Metastasis in Gastric Carcinomas. Cancer Res (1996) 56:2671–76.
120. Radisavljevic Z, Avraham H, Avraham S. Vascular Endothelial Growth Factor Up-Regulates ICAM-1 Expression Via the Phosphatidylinositol 3 OH-kinase/AKT/Nitric Oxide Pathway and Modulates Migration of Brain Microvascular Endothelial Cells. J Biol Chem (2000) 275:20770–74. doi: 10.1074/jbc.M002448200
121. Guo P, Yang J, Jia D, Moses MA, Auguste DT, et al. Icam-1-Targeted, Lcn2 Sirna-Encapsulating Liposomes are Potent Anti-Angiogenic Agents for Triple Negative Breast Cancer. Theranostics (2016) 6:1–13. doi: 10.7150/thno.12167
122. Lan W, Harmon D, Wang JH, Shorten G, Redmond P, et al. The Effect of Lidocaine on Neutrophil CD11b/CD18 and Endothelial ICAM-1 Expression and IL-1beta Concentrations Induced by Hypoxia-Reoxygenation. Eur J Anaesthesiol (2004) 21:967–72. doi: 10.1017/s0265021504000353
123. Lan W, Harmon DC, Wang JH, Shorten GD, Redmond PH, et al. Activated Endothelial interleukin-1beta, -6, and -8 Concentrations and Intercellular Adhesion Molecule-1 Expression are Attenuated by Lidocaine. Anesth Analg (2005) 100:409–12. doi: 10.1213/01.ANE.0000142113.39092.87
124. Suzuki S, Mori A, Fukui A, Ema Y, Nishiwaki K, et al. Lidocaine Inhibits Vascular Endothelial Growth factor-A-induced Angiogenesis. J Anesth (2020) 34:857–64. doi: 10.1007/s00540-020-02830-7
125. Abeyrathna P, Su Y. The Critical Role of Akt in Cardiovascular Function. Vascul Pharmacol (2015) 74:38–48. doi: 10.1016/j.vph.2015.05.008
126. Shibuya M. Vascular Endothelial Growth Factor (VEGF) and Its Receptor (Vegfr) Signaling in Angiogenesis: A Crucial Target for Anti- and Pro-Angiogenic Therapies. Genes Cancer (2011) 2:1097–105. doi: 10.1177/1947601911423031
127. Maacha S, Bhat AA, Jimenez L, Raza A, Haris M, Uddin S, et al. Extracellular Vesicles-Mediated Intercellular Communication: Roles in the Tumor Microenvironment and Anti-Cancer Drug Resistance. Mol Cancer (2019) 18:55. doi: 10.1186/s12943-019-0965-7
128. Call TR, Pace NL, Thorup DB, Maxfield D, Chortkoff B, Christensen J, et al. Factors Associated With Improved Survival After Resection of Pancreatic Adenocarcinoma: A Multivariable Model. Anesthesiology (2015) 122:317–24. doi: 10.1097/ALN.0000000000000489
Keywords: lidocaine, cancer, metastasis, recurrence, surgery, tumor, molecular mechanisms
Citation: Zhang C, Xie C and Lu Y (2021) Local Anesthetic Lidocaine and Cancer: Insight Into Tumor Progression and Recurrence. Front. Oncol. 11:669746. doi: 10.3389/fonc.2021.669746
Received: 19 February 2021; Accepted: 03 June 2021;
Published: 24 June 2021.
Edited by:
Brian J. Czerniecki, Moffitt Cancer Center, United StatesReviewed by:
Zhengyuan Xia, The University of Hong Kong, Hong Kong, SAR ChinaZiv Radisavljevic, Brigham and Women’s Hospital and Harvard Medical School, United States
Copyright © 2021 Zhang, Xie and Lu. This is an open-access article distributed under the terms of the Creative Commons Attribution License (CC BY). The use, distribution or reproduction in other forums is permitted, provided the original author(s) and the copyright owner(s) are credited and that the original publication in this journal is cited, in accordance with accepted academic practice. No use, distribution or reproduction is permitted which does not comply with these terms.
*Correspondence: Yao Lu, luyao@ahmu.edu.cn