- 1Department of Clinical Research, EpicentRx, San Diego, CA, United States
- 2Department of Medical Oncology, UC San Diego School of Medicine, San Diego, CA, United States
- 3Department of Clinical Research, InterWest Partners, Menlo Park, CA, United States
- 4Department of Radiation Oncology, Stanford University School of Medicine, Stanford, CA, United States
Glioblastoma is an aggressive and inevitably recurrent primary intra-axial brain tumor with a dismal prognosis. The current mainstay of treatment involves maximally safe surgical resection followed by radiotherapy over a 6-week period with concomitant temozolomide chemotherapy followed by temozolomide maintenance. This review provides a summary of the epidemiological, clinical, histologic and genetic characteristics of newly diagnosed disease as well as the current standard of care and potential future therapeutic prospects.
Introduction
Glioblastoma, a World Health Organization grade IV astrocytoma, with an incidence in North America of 5.0 per 100,000 population, representing 15 to 20% of all primary intracranial neoplasms, in adults (1), is highly aggressive, with an unusually dismal prognosis (death typically results in the first 15-16 months after diagnosis) (2), with a 5-year survival rate of 5%. These tumors arise from astrocytes and oligodendrocytes. The majority of glioblastoma are found in the supratentorial brain (frontal, temporal, parietal, and occipital lobes), with rare occurrence in the cerebellum, the brain stem and the spinal cord (3). Glioblastomas may develop in de novo patients as a primary glioblastoma or through progression from lower-grade astrocytomas in as secondary glioblastomas (4).
The incidence of glioblastoma is low compared with other cancers (5) such as lung, breast, prostate and colon cancer, but it dwarfs these other tumor types in terms of “average years of life lost” (20.1 years vs. 6.1 years for prostate cancer and 11.8 years for lung cancer) (6), often affecting patients in the prime of their lives. From this perspective, glioblastomas represent a very important problem in oncology, whose grim prognosis has changed little since the 1970s (7).
The surgical approach, if feasible, is the initial mainstay of treatment for patients with newly diagnosed glioblastoma. However, glioblastoma is a highly diffusive, invasive and vascularized tumor, and is not curable with surgical intervention. Therefore, concomitant and adjuvant temozolomide (TMZ), combined with radiation therapy has become established as the standard of care after surgery (8), with high dose steroids (dexamethasone) often prescribed to reduce vasogenic edema. FDA approved treatments for newly diagnosed glioblastomas are limited and include the Optune portable device, also known as Tumor Treating Fields (TTFs), which delivers an electric field to the tumor (9).
Unfortunately, GBM recurrence is inevitable and virtually all patients will relapse, with the majority of relapses occurring centrally within 2 cm of the original gadolinium-enhanced mass on MRI (10). This accounts for its unfavorable prognosis, and, despite decades of investment and research, there is still an unmet medical need for a more efficacious treatment (11). Extracranial metastasis are rare, occurring in less than 2% of patients. This is thought to be due, in part, to the dura mater and the thickened basement membrane of blood vessels that can prevent hematogenous and lymphatic spread, and also because central nervous system (CNS) tumor cells lack extracellular matrix protein required to invade surrounding connective tissue (12). Quality of life in these patients is progressively and significantly impacted due to the emergence of debilitating symptoms arising from infiltrative tumor growth far into functionally intact brain tissue that restricts and disrupts normal day-to-day activities (13).
For all these reasons, the identification and integration of new treatment options that enhance the cytotoxic effects of the standard-of-care radiation/temozolomide regimen constitute an urgent unmet medical need.
Classification and Histology
The WHO (World Health Organization) Classification of Tumors of the Central Nervous System is the current international standard for the nomenclature used for the classification of gliomas. It designates gliomas according to level of histologic malignancy and mitotic activity (14). Grades I and II gliomas are referred to as low-grade due to their low proliferative potential, while grade III and IV gliomas are high-grade because of their high proliferative rate and aggressive phenotype. As the most aggressive, invasive and undifferentiated type of tumor, glioblastoma is classified as Grade IV.
Glioblastoma were previously classified into 4 subtypes: 1) proneural, 2) neural, 3) classical, and 4) mesenchymal, with the proneural subtype possibly conferring a more favorable prognosis in younger patients. However, recent analysis of transcriptomes of glioma have revealed 3 subtypes strongly enriched with mRNAs associated with classical, proneural and mesenchymal subtypes, but not with the neural subtype, suggesting that this subtype may have arisen from contamination of the original samples with nontumor cells (15).
Over time, molecular biomarkers have played an important role in histological typing and diagnosis of glioblastomas (16), as well as for predicting survival and response to therapy. The best-known biomarker in this disease is the methylation status of the promoter for MGMT (O6-methylguanine-DNA methyltransferase). Epigenetic silencing of MGMT by methylation of the promoter is a favorable prognostic indicator and is associated with increased overall survival (OS) in patients treated with radiation therapy and alkylator chemotherapy (e.g. TMZ) (17, 18). The median OS of patients with MGMT-methylated tumors is 22-26 months compared with non-MGMT-methylated tumors of 12-15 months, respectively (17). Importantly, alteration in MGMT in MGMT-deficient glioblastoma cells has been implicated in acquired TMZ resistance in several ways, including modulation of DIP2A/MGMT signaling by Fstl1 (19), IKBKE-induced upregulation of MGMT (20) and regulation of MGMT expression by miRNAs resulting in degradation of MGMT mRNA before translation (21).
Another clinically relevant biomarker is the mutation status of IDH (isocitrate dehydrogenase)1 and IDH2, with approximately 10% of patients with glioblastoma (14) expressing a mutation in either of these genes, which is an early genetic event in gliomagenesis. Mutant IDH1 is a metabolic marker of secondary glioblastoma because of its ubiquitous expression in lower grade gliomas that eventually progress to glioblastoma. Given the increasing importance of molecular subtyping in glioblastoma (22), the WHO has classified glioblastoma into glioblastoma, IDH-wildtype, glioblastoma, IDH-mutant, and glioblastoma not otherwise specified (NOS) (14). While the WHO classification system has historically served as the primary source of updates on diagnostic classes, grades and criteria, in an effort to more rapidly integrate advances in understanding of brain tumor molecular pathogenesis into practice, cIMPACT-NOW (the Consortium to Inform Molecular and Practical Approaches to CNS Tumor Taxonomy) was established.
Examples of recent cIMPACT-NOW activities include recognition that the lack of an IDH mutation alone is insufficient for designating a glioma as WHO Grade IV. Therefore, the cIMPACT-NOW Update 3 provided diagnostic criteria for “Diffuse astrocytic glioma, IDH-wildtype, with molecular features of glioblastoma WHO Grade IV,” and recommended that histologic grade II and III IDH-wildtype diffuse astrocytic glioma that contain a high level of EGFR amplification, the combination of whole chromosome 7 gain and whole chromosome 10 loss, or TERT promoter mutations, correspond to WHO grade IV and be referred to as diffuse astrocytic glioma, IDH-wildtype, with molecular features of glioblastoma, WHO grade IV (23). Furthermore, they also concluded that subsets of IDH-wildtype diffuse astrocytic glioma with specific molecular signatures have better outcomes and should not be given high grade designation, including those with MYB/MYBL or BRAF mutations (23). cIMPACT-NOW update 1 clarified the use of the term NOS (Not Otherwise Specified) and proposed the use of NEC (Not Elsewhere Classified) (24). cIMPACT-Now Update 6 defined astrocytoma, IDH-mutant as diffusely infiltrative astrocytic glioma with microvasculature proliferation or necrosis with a CDKN2A/B homozygous deletion, connotating grade IV (25).
The clinical significance of IDH in glioblastoma is related to its function in the Krebs Cycle. IDH normally decarboxylates isocitrate to α-ketoglutarate (α-KG) and produces NADPH, which, in turn, reduces GSSG to glutathione (GSH) (22, 26). Mutated IDH instead catalyzes the conversion of α-KG to α-hydroxyglutarate (α-HG), a putative oncometabolite (27), at the expense of NADPH production. While, IDH mutations contribute to tumorigenesis, they also are associated with a better prognosis, possibly as a result of decreased antioxidative levels of NADPH, and by extension, GSH, which render the tumor more radio- and chemosensitive (28). Of note, IDH mutations are virtually absent in the elderly (29).
Besides IDH, secondary glioblastomas are characterized by TP53, PDGF and ATRX mutations, as well as loss of chromosome 19q (30), while in the majority of IDH-wildtype, epidermal growth factor receptor (EGFR) overexpression, phosphate and tensin homolog (PTEN) mutations, and loss of chromosome 10q are common (31).
Conventional histologic hallmarks of glioblastoma include cellular polymorphism, nuclear atypia, a high mitotic index (14), pseudopalisading necrosis (32) and microvascular hyperplasia or neovascularization. Glioblastoma is a highly hypoxic and prothrombotic (33) tumor, which promotes compensatory neovascularization driven by the secretion of pro-angiogenic factors, resulting in local vascular hyperplasia, stasis and thromboembolic vascular occlusion that, in turn, augment the hypoxia and accelerate progression. (Figure 1) Pseudopalisades, a pathognomic feature of glioblastoma, are densely-packed rows of cells that encircle hemorrhagic necrotic foci in which are found a multitude of thrombosed vessels (34). This local alignment and aggregation of cells is thought to represent a coordinated mass migration event, away from a nutrient- and oxygen-impoverished microenvironment (35). (Figure 2)
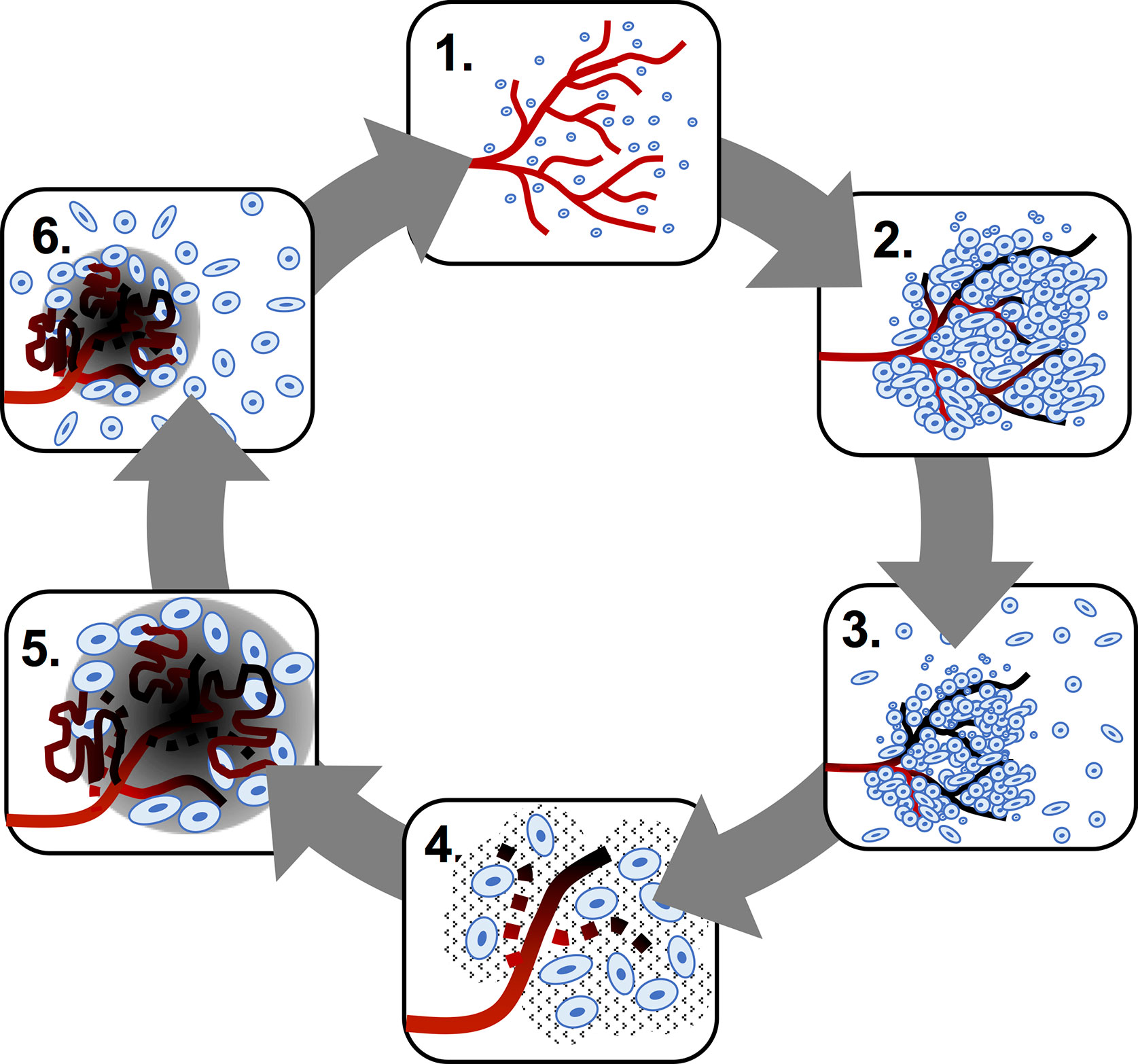
Figure 1 Hypoxia-neovascularization cycle in glioblastoma. The steps below are as follows: 1. Glioma cells consume oxygen provided by the functional vasculature. 2. Endothelial injury, prothrombotic factors and increased mechanical pressure in regions of high glioma cell density induce vaso-occlusion and necrosis. 3. Perivascular glioma cells switch to a “go” phenotype based on presence of hypoxia. 4. Pseudopalisading glioma cells secrete pro-angiogenic factors. 5. Pro-angiogenic factors stimulate the formation of aberrant, highly permeable neovasculature, which results in more hypoxia and accelerated progression. 6. Pseudopalisading cells migrate to a new vasculature where the cycle begins anew.
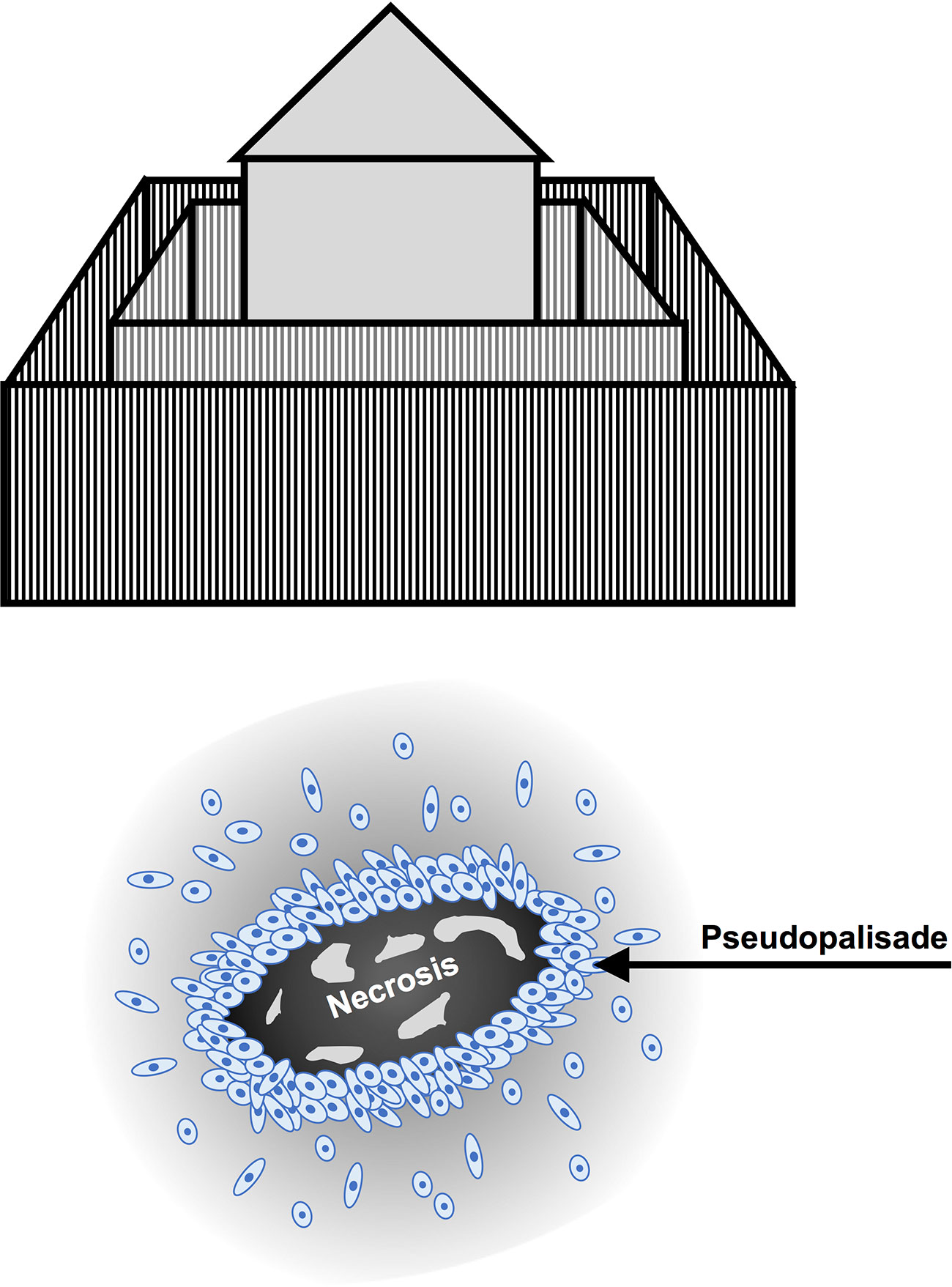
Figure 2 Palisades and pseudopalisading cells. Palisades are defined as a protective layer, similar to a fence or perimeter of wooden stakes or iron railings. (top figure) In glioblastoma, pseudopalisades or “false palisades” are dense migratory zones of cells in picket-fence or perimeter formation, which surround necrotic tissue; hence the term, “pseudopalisading necrosis”. The pervasive hypothesis is that pseudopalisading cells are “microenvironmental migrants” that co-localize in search of better oxygenated conditions due to the presence of vascular collapse and necrosis (40). (bottom figure)
According to the Go-or-Grow hypothesis, cells can either migrate or proliferate, but not do both, which accounts for the relative quiescence of pseudopalisading cells (36). Those cells that do not migrate undergo apoptosis, eventually leading to a zone of central necrosis. These repeated cycles of angiogenesis, vascular collapse and cellular migration/infiltration contribute to resistance, making treatment of these tumors difficult (37), as tumor cells that have spread far and wide from the main tumor in search of a more hospitable microenvironment around new vasculature make the tumor deeply infiltrative. Interestingly, hypoxia is only marginally present in low grade gliomas, where the vasculature remains largely intact (38), which suggests that low oxygenation created by the tumor itself maybe a driving force responsible for progression and aggressiveness (39).
Epidemiology and Causative Factors
Glioblastoma, which accounts for about 15% of all CNS cancers (41), is an orphan disease, with less than 20,000 cases in the United States. Nearly half of patients with a new diagnosis of glioblastoma are over age 65, although glioblastoma can occur at any age including childhood (42). Of note, survival markedly decreases with advancing age (41). The tumor is twice as common in whites as in Africans and African-Americans, with a lower incidence in Asians and Native Americans, and slightly more males than females affected (43). No definitive links with smoking, diet, cellular phones, environmental exposures or electromagnetic fields have been identified (44). In 5% of cases a family history is reported and rare syndromes, such as Li-Fraumeni syndrome, neurofibromatosis I and II, Turcot’s syndrome, Ollier disease and Maffucci syndrome have been associated with elevated risk (45, 46). While ionizing radiation is a well-established risk factor, only a minority of presumed radiation-induced cranial tumors are glioblastomas (47). One possible driver of gliomagenesis that has been proposed is infection from CMV or EBV since both viruses have been detected in glioma samples, but it is presently unknown whether this is a causative or epiphenomenal association (48, 49).
Clinical Presentation and Diagnosis
The clinical presentation of glioblastoma varies depending on the size and location of the tumor as well as the degree of peritumoral edema (50). The most common general symptoms are new-onset headache and seizure, although seizures are more frequent in low grade glioma (51). Focal symptoms such as neurological deficits, and cognitive and personality changes are due to compression and infiltration of normal brain tissue (52). The presence and degree of peritumoral edema correlates with symptomatology. High dose steroids (10-20 mg IV dexamethasone loading dose followed by dexamethasone 4-24 mg in divided doses) (53) are typically administered to reduce tumor-associated vascular permeability and edema.
Gadolinium-enhanced magnetic resonance imaging (MRI) is the gold standard for glioblastoma diagnosis, due to the permeability of immature tumor vessels, which leads to extravascular accumulation of contrast agent with T1 shortening and signal enhancement on T1-weighted images (54). However, true delineation of the tumor is difficult because glioma cells invariably extend beyond the Gadolinium-enhanced abnormality (55).
Characteristically, with MRI, glioblastomas appear as a variegated or multiform contrast-enhancing mass(es), with thick, irregular rinds of enhancement surrounding hypointense, necrotic cores. (Figure 3) The appearance of necrosis, a hallmark of glioblastoma, is related both to the presence of thrombosed vessels and the rapid proliferative rate of the tumor cells, which leads to an extreme mismatch between accelerated oxygen consumption and the scarcity of blood supply (57). Surrounding vasogenic edema with mass effect, hemorrhage, and distortion or displacement of adjacent structures may also be visible (58).
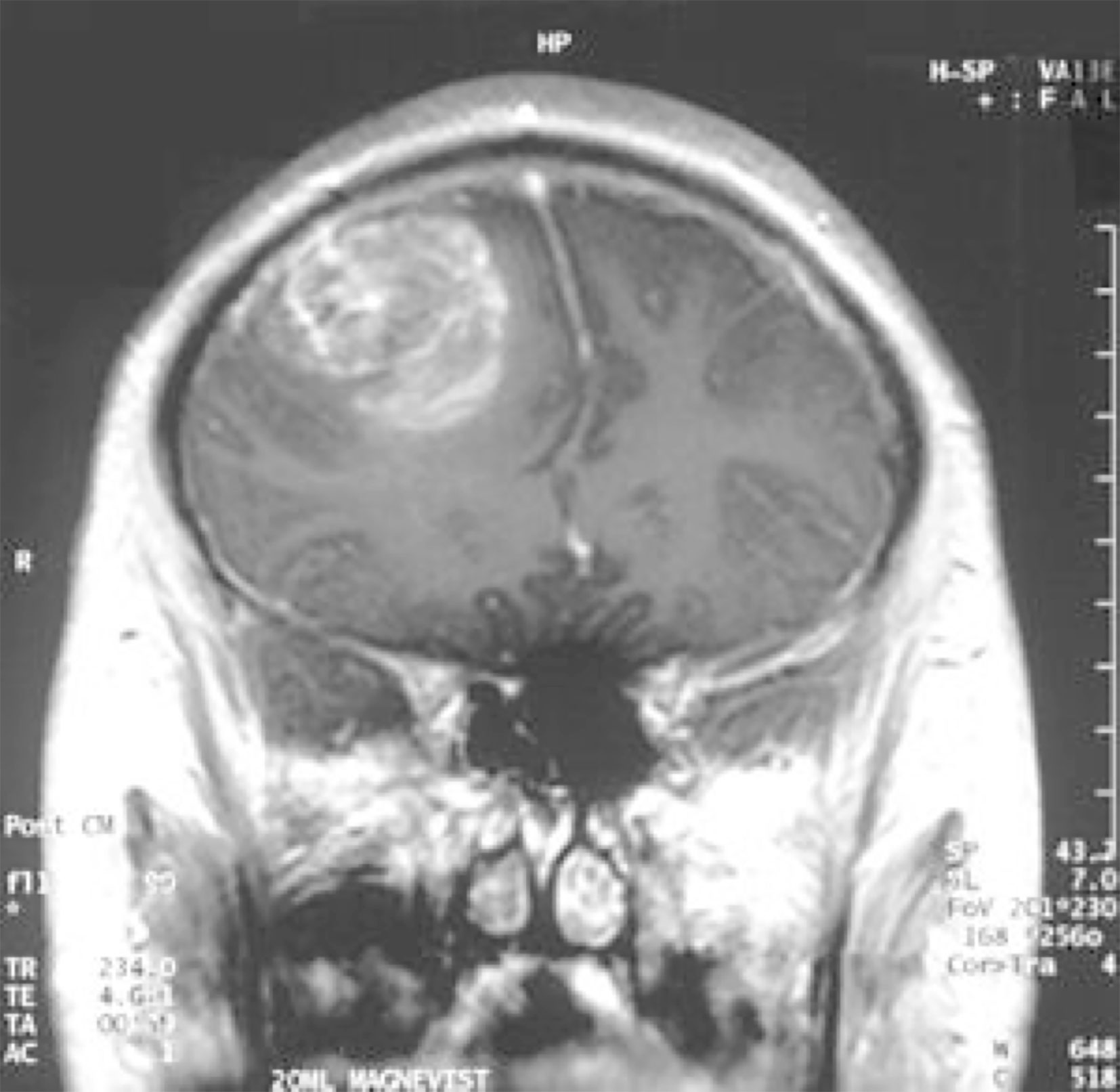
Figure 3 Magnetic resonance imaging (MRI) appearance of glioblastoma: contrast enhancing rind of tumor surrounding a necrotic core (56).
Radiographic patterns of disease include: local (unifocal disease), distant (second lesion noncontiguous with the primary lesion), multifocal (>2 non-contiguous lesions and/or cerebral spinal fluid dissemination) and diffuse (> 3 cm beyond the primary site) (59).
Advanced neuroimaging techniques, including assessment of perfusion, diffusion and spectroscopy, are very useful for purposes of characterizing glioblastomas at the time of diagnosis and for monitoring response to therapy and recurrence post treatment (60–64). Magnetic resonance spectroscopy (MRS) can be used to study metabolic changes in brain tumors. For example, use of proton MRS for biochemical profiling and measurement of phosphocholine, creatine and N-acetylaspartate, as well as associated relevant concentration ratios, have a role in the diagnosis of glioblastoma (63). Clinical applications include use of 1H-MRS for the differentiation of glioma recurrence from radiation necrosis (65). This approach is not only applicable to Hydrogen, but also to many other nuclei or isotopes (66). In diffusion-weighted imaging, water molecule diffusion is characterized by an apparent diffusion coefficient (ADC) (64). In glioblastoma, ADC is useful for early characterization of tumor, as there is an inverse correlation between ADC and grade (67), and may also help to detect early recurrence, especially in non-enhancing regions of the brain (68). Another imaging technology, diffusion tensor imaging, generates a mathematical model of diffusion in 3-D (66), and can be useful for the detection of tumor infiltration of adjacent normal appearing white matter (69). Perfusion weighted imaging allows for imaging of neovascularization, with assessment of blood flow, volume and leakage (70), and has been useful for prognostication in glioblastoma (71), assessment of treatment response, and differentiation of recurrence/progression from stable disease (72). Lastly, a new imaging approach, chemical exchange saturation transfer (64) allows for detection of specific molecules present at very low concentrations.
Therapeutic Management
Treatment
Treatment of glioblastoma is quadripartite: 1) surgery 2) adjuvant radiotherapy and TMZ 3) maintenance TMZ and 4) alternating electric fields
Surgery
The bedrock of glioblastoma treatment is gross total resection or subtotal resection to immediately relieve the tumor-associated mass effect, delay recurrence, and obtain tissue for molecular testing (73). Complete resection is currently impossible due to the diffuse infiltrative nature of the disease and the potential for iatrogenic damage to intact brain tissue as a result, especially when the tumor is located in or near the eloquent cortex, critical areas whose removal permanently compromises sensory processing, linguistic ability, and/or motor function (74). The presence of residual disease is responsible for the inevitable subsequent disease progression or recurrence, usually six months after surgery (75).
Nevertheless, aggressive resection is warranted on the basis of several studies, which have demonstrated that the smaller the residual tumor burden, the longer the progression-free survival (PFS) and OS, which makes the extent of resection a crucial prognostic factor (76–78). However, despite the introduction of several new surgical techniques and innovations (79), including neuronavigation, fluorescence, and intraoperative imaging (MRI, CT, ultrasound) to maximize visualization of tumor borders and optimize the extent of resection, while minimizing damage to adjacent normal brain, the prognosis and the quality of life for glioblastoma patients remains poor (80).
Chemoradiation Therapy
After maximal surgical resection, the standard of care is post-operative radiotherapy (81) to a field encompassing the primary tumor with a 2-3 cm margin. Radiation is commonly given over six to weeks with three-dimensional conformal beam or intensity-modulated radiotherapy (RT) (2 Gy per daily fraction (Monday to Friday) for a total dose of 60 Gy) with concomitant TMZ chemotherapy at a dose of 75 mg per square meter of body-surface area per day, 7 days per week followed by maintenance TMZ at a dose of 150-200 mg per square meter for 5 days during each 28-day cycle for up to 6 adjuvant chemotherapy cycles. Because the addition of TMZ to surgery and radiotherapy for newly diagnosed glioblastoma resulted in a clinically meaningful and statistically significant survival benefit with minimal additional toxicity, concomitant TMZ and radiotherapy followed by 6 cycles of adjuvant TMZ was adopted as the new standard of care for newly diagnosed glioblastoma (8). This practice change was based on a Phase 3 EORTC 26981-22981/NCIC CTG trial in patients with WHO performance status of 2 or more. In this study median PFS was 6.9 months in the TMZ arm vs. 5.0 months with radiation therapy arm, and a median survival of 14.6 months vs. 12.1 months, respectively. Of note, in the United States, non-progressive patients have been treated with TMZ for up to 12 cycles or more (82). Importantly, a recent phase II trial of continuing TMZ beyond 6 adjuvant cycles was associated with more toxicity, without any additional benefit in 6 month PFS (83).
The Stupp protocol (8), based on the EORTC-NCIC trial paper discussed above, established the primacy of TMZ and RT to RT alone. The analysis of this trial also correlated outcomes with MGMT (O6-methylguanine-DNA methyltransferase) methylation status. As an alkylating agent, temozolomide methylates DNA at the O6, N3, and N7 positions, which, if unrepaired, leads to DNA strand breaks (84). MGMT is a DNA repair enzyme located on chromosome 10q26 whose methylation is associated with loss of MGMT expression (85) and, hence, enhanced sensitivity to TMZ as a result of reduced DNA-repair activity (86). Conversely, an unmethylated MGMT enzyme results in high activity of MGMT and reduced activity of TMZ. Therefore, methylated MGMT is a favorable prognostic factor (87). More recently, in an effort to improve upon the standard of care regimen using TMZ, a phase 3 trial was performed comparing the combination of lomustine and TMZ vs. standard TMZ in patients with newly diagnosed glioblastoma with methylated MGMT promoter, with a median survival of 31.4 m vs. 48.1m (88).
In elderly or poor performance patients, hypofractionated radiotherapy (e.g., 40 Gy/15 Fractions) has emerged as a viable alternative to standard radiation therapy (e.g., 60 Gy/30 Fractions) on the basis of several studies, which collectively indicate that while both confer a similar survival benefit, hypofractionated radiation therapy is associated with less neurotoxicity and steroid administration (89).
Given the potential role of hypoxia in the biology of glioblastoma, there has been considerable interest in anti-angiogenesis therapies, but concern that long term treatment with agents such as bevacizumab might increase tumor hypoxia and result in a more infiltrative phenotype (90). Two Phase 3 trials of bevacizumab in the first line setting (91, 92), demonstrated improvement of PFS without a corresponding improvement in OS and, on this basis, bevacizumab is not administered in the first line therapy. Moreover, bevacizumab was associated with pseudoresponse, a term which refers to a rapid regression of enhancement due to vascular regression with a corresponding increase of invasiveness due to induction of hypoxia (93).
A number of new combination regimens incorporating immunotherapy have been conducted in the newly diagnosed setting. Immunotherapy is a potentially promising strategy due to the presence of a functional CNS lymphatic system and an altered blood brain barrier, which facilitate immune cell trafficking in and out of the CNS (94). Of note, glioblastoma is an “immune cold tumor”, meaning that it is not very immunogenic, with a very low mutational burden, and a highly immunosuppressive microenvironment, characterized by a low level of T cell infiltration (95). Nevertheless, several studies have shown that neoadjuvant PD1 blockade can modulate the glioblastoma microenvironment resulting in enhanced anti-tumor immune responses, elevated expression of chemokine transcripts, increased immune cell infiltration into tumors, and greater T cell receptor clonal diversity (96–98). The question remains whether or not these biological effects will translate into clinical benefit. Recent and ongoing trials combining immunotherapy with standard of care therapy are addressing this question. Unfortunately, Phase 3 clinical trials with nivolumab in newly diagnosed glioblastoma patients with unmethylated MGMT promoter status (CheckMate-493; NCT02617589) combined with radiation therapy (99), and in patients with methylated MGMT promoter status (CheckMate-548; NCT02667587) (100) combined with standard of care radiation therapy and TMZ (vs. standard of care treatment) failed to demonstrate a significant improvement in OS in CheckMate-498, and failed to meet the PFS primary endpoint in CheckMate-548. However, CheckMate-548 will be continued to allow the other primary endpoint, OS, to mature. Another immunotherapy combination trial, PERGOLA (NCT03899857) is evaluating the efficacy and toxicity of adding pembrolizumab to concurrent radiation therapy and TMZ in newly diagnosed glioblastoma patients. One caveat with immunotherapy in this setting is that the frequent use of high-dose corticosteroids to attenuate cerebral edema may serve to dampen anti-tumor immune responses.
Tumor Treating Fields
In October 2015, Optune®, a noninvasive portable device, which delivers alternating electric fields (TTFs) to disrupt cell division (101), was approved by the U.S. Food and Drug Administration (FDA) as an adjunct to TMZ in first-line glioblastoma on the basis of a Phase 3 trial called EF-14 in which the median OS and PFS at interim analysis (n=315) was 19.6 months for TTFs/TMZ vs. 16.6 months for TMZ alone (HR 0.75; log-rank p = 0.034) and 7.1 months for TTFs/TMZ vs. 4.0 months for TMZ alone(HR 0.6; log-rank p = 0.0014), respectively (9). In addition, an increase in 5-year survival from 5% to 13% was observed (102). Moreover, TTFs was safe with skin reactions from the application of electrodes to the head as the main adverse event (103). Nevertheless, use has been limited due to cost, patient stigmatization/inconvenience and the novelty of the mechanism (104).
Recent and Anticipated Results From Clinical Trials
Among some of the most eagerly anticipated results are those from a Phase 3 trial with DCVax-L, an autologous dendritic cell vaccine, with radiation therapy and TMZ. While final results are pending, an interim analysis demonstrated a mean OS of 23.1 months, with 2 and 3 year survival of 46.2% and 25.4%, respectively, with a higher OS in patients with methylated MGMT of 34.7 months vs. 19.8 months. To date DCVax has been safe and well tolerated with only 2.1% SAEs (105). Other promising vaccine approaches include SurVaxM, a peptide vaccine to survivin, with a single arm Phase II trial showing benefit in terms of PFS and OS (106). A prospective randomized trial is planned and expected to open to accrual in 2020.
There was also considerable interest in a Phase 3 trial using the antibody-drug conjugate depatuxizumab mafodotin (ABT-414), which binds activated EGFR, in combination with standard-of-care treatment in patients with newly diagnosed, EGFR-amplified glioblastoma. Unfortunately, an interim analysis failed to show an OS benefit, and the study was stopped for futility (107). EGFR has been thought of as an attractive target, due to EGFR amplification and overexpression in approximately 50% of glioblastoma tumors (108), even though, to date, trials with EGFR-pathway inhibitors have been disappointing (109), likely due to poor BBB permeability with subtherapeutic drug levels. Unfortunately, so far there is no evidence to date of an OS benefit with the addition of anti-EGFR treatments in first line or recurrent glioblastoma (110).
A large number of targeted agents are currently under investigation in early clinical trials (111). An example is the use of poly-(ADP-Ribose)-DNA Polymerase (PARP) inhibitors to enhance the efficacy of standard of care chemoradiation. There is considerable interest, in particular, in the use of veliparib. However, preliminary results with veliparib in the VERTU trial, a randomized Phase II trial in MGMT-unmethylated glioblastoma patients found that the combination of veliparib with chemoradiation was well tolerated, but did not improve outcomes (112). This combination is now being evaluated in a Phase III trial in MGMT-methylated newly diagnosed glioblastoma patients with adjuvant temozolomide, with results expected soon. Another active area of investigation is the use of targeted agents in place of TMZ, While trials replacing TMZ with targeted agents in unselected patients without MGMT promoter hypermethylation have also shown no OS benefit, an important new Phase 1/11a NCT Neuro Master Match umbrella trial is ongoing in newly diagnosed glioblastoma patients with IDH-wild type, without MGMT promoter hypermethylation, with a variety of promising agents in combination with standard radiation therapy (113). In addition, there are a number of promising new therapies and new agents in the pipeline (114, 115) in early preclinical/clinical development, such as TORC ½ inhibitors (116), tumor targeting peptides (117), immunotherapies (118, 119); combination therapies with anti-CD47 (120), and approaches targeting cancer cell metabolism (121).
Conclusion
Despite decades of research, glioblastoma remains one of the deadliest and most feared of all cancers. Treatment for it has reached a relative impasse with the use of surgery, radiation, TMZ, and TTFs, since the addition of other targeted agents such as angiogenesis and EGFR inhibitors have failed to date to improve survival, and there is no standard treatment for recurrence, which is inevitable. New therapies are desperately needed for first line treatment, with a number of promising therapeutic agent currently in development.
Author Contributions
BO and SK contributed to the study concept, design, and are guarantor of integrity of the entire study. BO, TR, AO, NS, and SK all contributed to literature search, manuscript preparation, and manuscript editing. All authors contributed to the article and approved the submitted version.
Conflict of Interest
Author BO is employed by the company EpicentRx. AO is currently employed by InterWest Partners.
The remaining authors declare that the research was conducted in the absence of any commercial or financial relationships that could be construed as a potential conflict of interest.
References
1. Stupp R, Tonn JC, Brada M, Pentheroudakis G. High-grade malignant glioma: ESMO Clinical Practice Guidelines for diagnosis, treatment and follow-up. Ann Oncol (2010) 21 Suppl 5:v190–3. doi: 10.1093/annonc/mdu05
2. Holland EC. Glioblastoma multiforme: The terminator. Proc Natl Acad Sci (2000) 97(12):6242–4. doi: 10.1073/pnas.97.12.6242
3. Konecne SM. Central nervous system neoplasms. In: Goodman C, Boissonnault W, editors. Pathology: Implications for the Physical Therapist. Philadelphia, Pa: WB Saunders Co (1998). p. 702–22.
4. Ohgaki H, Kleihues P. Genetic pathways to primary and secondary glioblastoma. Am J Pathol (2007) 170(5):1445–53. doi: 10.2352/ajpath.2007.070011
5. Siegel R, Ma J, Zou Z, Jemal A. Cancer Statistics. CA Cancer J Clin (2014) 64:9–29. doi: 10.3322/caac.21208
6. Burnet NG, Jefferies SJ, Benson RJ, Hunt DP, Treasure FP. Years of life lost (YLL) from cancer is an important measure of population burden–and should be considered when allocating research funds. Br J Cancer (2005) 92(2):241–5. doi: 10.1038/sj.bjc.6602321
7. Kleihues P, Burger PC, Collins VP, Newcomb EW, Ohgaki H, Cavenee WK. Glioblastoma. In: Kleihues P, Cavenee WK, editors. Pathology and genetics of tumors of the nervous system. Lyon, France: IARC (2000). p. 29–39.
8. Stupp R, Mason WP, van den bent MJ, Weller M, Fisher B, Taphoorn MJ, et al. Radiotherapy plus concomitant and adjuvant temozolomide for glioblastoma. N Engl J Med (2005) 352(10):987–96. doi: 10.1371/journal.pone.0078943
9. Stupp R, Taillibert S, Kanner AA, Kesari S, Steinberg DM, Toms SA, et al. Maintenance Therapy with Tumor-Treating Fields Plus Temozolomide vs Temozolomide Alone for Glioblastoma: A Randomized Clinical Trial. JAMA (2015) 314:2535–43. doi: 10.1001/jama.2015.16669
10. Sherriff J, Tamangani J, Senthil L, Cruickshank G, Spooner D, Jones B, et al. Patterns of relapse in glioblastoma multiforme following concomitant chemoradiotherapy with temozolomide. Br J Radiol (2013) 86(1022):20120414. doi: 10.1259/bjr.20120414
11. Hou LC, Veeravagu A, Hsu AR, Tse VC. Recurrent glioblastoma multiforme: a review of natural history and management options. Neurosurg Focus (2006) 20(4):E5. doi: 10.3171/foc.2006.20.4.2
12. Ray A, Manjila S, Hdeib AM, Radhakrishnan A, Nock CJ, Cohen ML, et al. Extracranial metastasis of gliobastoma: Three illustrative cases and current review of the molecular pathology and management strategies. Mol Clin Oncol (2015) 3(3):479–86. doi: 10.3892/mco.2015.494
13. Koul R, Dubey A, Torri V, Kakumanu A, Goyal K. Glioblastoma Multiforme In Elderly Population. Int J Neurosurg (2012) 8(1). doi: 10.5580/2b2b
14. Louis DN, Perry A, Reifenberger G, Deimling A, Figarella-Branger D, Webster K, et al. The 2016 World Health Organization classification of tumors of the central nervous system: a summary. Acta Neuropathol (2016) 131:803–20. doi: 10.1007/s00401-016-1545-1
15. Sidaway P. CNS cancer: Glioblastoma subtypes revisited. Nat Rev Clin Oncol (2017) 14(10):587. doi: 10.1038/nrclinonc.2017.122
16. Verhaak RG, Hoadley KA, Purdom E, Wang V, Qi Y, Wilkerson MD, et al. Integrated genomic analysis identifies clinically relevant subtypes of glioblastoma characterized by abnormalities in PDGFRA, IDH1, EGFR, and NF1. Cancer Cell (2010) 17(1):98–110. doi: 10.1016/j.ccr.2009.12.020
17. Hegi ME, Diserens AC, Gorlia T, Hamou MF, Tribolet ND, Weller M, et al. MGMT gene silencing and benefit from temozolomide in glioblastoma. N Engl J Med (2005) 352(10):997–1003. doi: 10.1056/NEJMoa043331
18. Mansouri A, Hachem LD, Mansouri S, Nassiri F, Laperriere NJ, Xia D, et al. MGMT promoter methylation status testing to guide therapy for glioblastoma: refining the approach based on emerging evidence and current challenges. Neuro Oncol (2019) 21(2):167–78. doi: 10.1093/neuonc/noy132
19. Nie E, Miao F, Jin X, Wu W, Zhou X, Zeng A, et al. Fstl1/DIP2A/MGMT signaling pathway plays important roles in temozolomide resistance in glioblastoma. Oncogene (2019) 38(15):2706–21. doi: 10.1038/s41388-018-0596-2
20. Guo G, Sun Y, Hong R, Xiong J, Lu Y, Liu Y, et al. IKBKE enhances TMZ-chemoresistance through up regulations of MGMT expression in glioblastoma. Clin Trans Oncol (2019) 22(8):1252–62. doi: 10.1007/s12094-019-02251-3
21. Kirstein A, Schmid TE, Combs SE. The Role of miRNA for the Treatment of MGMT Unmethylated Glioblastoma Multiforme. Cancers (Basel) (2020) 12(5):1099. doi: 10.3390/cancers12051099
22. Guo C, Pirozzi CJ, Lopez GY, Yan H. Isocitrate dehydrogenase mutations in gliomas: mechanisms, biomarkers and therapeutic target. Curr Opin Neurol (2011) 24(6):648–52. doi: 10.1097/WCO.0b013e32834cd415
23. Brat DJ, Aldape K, Colman H, Holland EC, Louis DN, Jenkins RB, et al. cIMPACT-NOW update 3: recommended diagnostic criteria for “Diffuse astrocytic glioma, IDH-wildtype, with molecular features of glioblastoma, WHO grade IV”. Acta Neuropathol (2018) 136(5):805–10. doi: 10.1007/s00401-018-1913-0
24. Louis DN, Ellison DW, Brat DJ, Aldape K, Capper D, Hawkins C, et al. cIMPACT-NOW: a practical summary of diagnostic points from Round 1 updates. Brain Pathol (2019) 29(4):469–72. doi: 10.1111/bpa.12732
25. Louis DN, Wesseling P, Aldape K, Brat DJ, Capper D, Cree IA, et al. cIMPACT-NOW update 6: new entity and diagnostic principle recommendations of the cIMPACT-Utrecht meeting on future CNS tumor classification and grading. Brain Pathol (2020) 30(4):844–56. doi: 10.1111/bpa.12832
26. Kirsch M, De Groot H. NAD(P)H, a directly operating antioxidant? FASEB J (2001) 15(9):1569–74. doi: 10.1096/fj.00-0823hyp
27. Li T, Cox CD, Ozer BH, Nguyen HN, Lai TJ, Li S, et al. D-2-Hydroxyglutarate Is Necessary and Sufficient for Isocitrate Dehydrogenase 1 Mutant-Induced MIR148A Promote Methylation. Mol Cancer Res (2018) 16(6):947–60. doi: 10.1158/1541-7786.MCR-17-0367
28. Baldewpersad Tewarie NM, Burgers IA, Dawood Y, den Boon HC, den Brok MG, Klunder JH, et al. NADP+-dependent IDH1 R132 mutation and its relevance for glioma patient survival. Med Hypotheses (2013) 80:728–31. doi: 10.1016/j.mehy.2013.02.022
29. Wiestler B, Claus R, Hartlieb SA, Schliesser MG, Weiss EK, Hielscher T, et al. Malignant astrocytomas of elderly patients lack favorable molecular markers: an analysis of the NOA-08 study collective. Neuro Oncol (2013) 15(8):1017–26. doi: 10.1093/neuonc/not043
30. Reuss DE, Sahm F, Schrimpf D, Wiestler B, Capper D, Koelsche C, et al. ATRX and IDH1-R132H immunohistochemistry with subsequent copy number analysis and IDH sequencing as a basis for an “integrated” diagnostic approach for adult astrocytoma, oligodendroglioma and glioblastoma. Acta Neuropathol (2015) 129:133–46. doi: 10.1007/s00401-014-1370-3
31. Parsons DW, Jones S, Zhang X, Lin JC, Leary RJ, Angenendt P, et al. An integrated genomic analysis of human glioblastoma multiforme. Science (2008) 321:1807–12. doi: 10.1126/science.1164382
32. Brat DJ, van Meir EG. Vaso-occlusive and prothrombotic mechanisms associated with tumor hypoxia, necrosis, and accelerated growth in glioblastoma. Lab Invest (2004) 84:397–405. doi: 10.10138/labinvest.3700070
33. Navone SE, Guarnaccia L, Locatelli M, Rampini P, Caroli M, la Verde N, et al. Significance and Prognostic Value of The Coagulation Profile in Patients with Glioblastoma: Implications for Personalized Therapy. World Neurosurg (2019) 121:e621–9. doi: 10.1016/j.wneu.2018.09.177
34. Simanek R, Vormittag R, Hassler M, Roessler K, Schwarz M, Zielinski C, et al. Venous thromboembolism and survival in patients with high-grade glioma. Neuro Oncol (2007) 9:89–95. doi: 10.1215/15228517-2006-035
35. Martínez-González A, Calvo GF, Pérez Romasanta LA, Pérez-García VM. Hypoxic cell waves around necrotic cores in glioblastoma: a biomathematical model and its therapeutic implications. Bull Math Biol (2012) 74(12):2875–96. doi: 10.1007/s11538-012-9786-1
36. Giese A, Bjerkvig R, Berens ME, Westphal M. Cost of migration: invasion of malignant gliomas and implications for treatment. J Clin Oncol (2003) 21:1624–36. doi: 10.1200/JCO.2003.05.063
37. Nazzaro JM, Neuwelt EA. The role of surgery in the management of supratentorial intermediate and high-grade astrocytomas in adults. J Neurosurg (1990) 73:331–44. doi: 10.3171/jns.1990.73.3.0331
38. Zagzag D, Zhong H, Scalzitti JM, Laughner E, Simons JW, Semenza GL. Expression of hypoxia-inducible factor 1α in brain tumors: association with angiogenesis, invasion, and progression. Cancer (2000) 88:2606–18. doi: 10.1002/1097-0142(20000601)88:11<2606::AID-CNCR25>3.0.CO;2-W
39. Kalkan R. Hypoxia Is the Driving Force Behind GBM and Could Be a New Tool in GBM Treatment. Crit Rev Eukaryot Gene Expr (2015) 25(4):363–9. doi: 10.1615/critreveukaryotgeneexpr.2015015601
40. Huang WJ, Chen WW, Zhang X. Glioblastoma multiforme: Effect of hypoxia and hypoxia inducible factors on therapeutic approaches. Oncol Lett (2016) 12(4):2283–8. doi: 10.3892/ol.2016.4952
41. Ostrom QT, Gittleman H, Farah P, Ondracek A, Chen Y, Wolinsky Y, et al. CBTRUS statistical report: Primary brain and central nervous system tumors diagnosed in the United States in 2006-2010. Neuro Oncol (2013) 15 Suppl:2ii–56. doi: 10.1093/neuonc/not151
42. Ostrom QT, Gittleman H, Fulop J, Liu M, Blanda R, Kromer C, et al. CBTRUS statistical report: primary brain and central nervous system tumors diagnosed in the United States in 2008-2012. Neuro Oncol (2015) Suppl 4:iv1–iv62. doi: 10.1093/neuonc/nov189
43. Ostrom QT, Cioffi G, Gittleman H, Patil N, Waite K, Kruchko C, et al. CBTRUS Statistical Report: Primary Brain and Other Central Nervous System Tumors Diagnosed in the United States in 2012-2016. Neuro Oncol (2019) 21(Suppl 5):v1–v100. doi: 10.1093/neuonc/noz150
44. Lehrer S, Green S, Ramanathan L, Rosenzweig K, Labombardi V. No consistent relationship of glioblastoma incidence and cytomegalovirus seropositivity in whites, blacks, and Hispanics. Anticancer Res (2012) 32(3):1113–5.
45. Sadetzki S, Bruchim R, Oberman B, Armstrong G, Lau CC, Claus EB, et al. Description of selected characteristics of familial glioma patients - results from the Gliogene Consortium. Eur J Cancer (2013) 49(6):1335–45. doi: 10.1016/j.ejca.2012.11.009
46. Ellor SV, Pagano-Yo ung TA, Avgeropoulos NG. Glioblastoma: Background, standard treatment paradigms, and supportive care considerations. J Law Med Ethics (2014) 42:171–82. doi: 10.1111/jlme.12133
47. Fisher JL, Schwartzbaum JA, Wrensch M, Wiemels JL. Epidemiology of brain tumors. Neurol Clin (2007) 25:867–90, vii. doi: 10.1016/j.ncl.2007.07.002
48. Bhattacharjee B, Renzette N, Kowalik TF. Genetic analysis of cytomegalovirus in malignant gliomas. J Virol (2012) 86:6815–24. doi: 10.1128/JVI.00015-12
49. Akhtar S, Vranic S, Cyprian FS, Al Moustafa AE. Epstein-Barr Virus in Gliomas: Cause,Association, or Artifact? Front Oncol (2018) 8:123. doi: 10.3389/fonc.2018.00123
50. Clarke CRA. Neurological diseases in Kumar & Clark Clinical Medicine. 6th ed. Kumar P, Clark M, editors. Edinburgh: Elsevier Saunders (2005). p. 1244–45. doi: 10.1136/jnnp.2004.040360
51. Grant R. Overview: brain tumour diagnosis and management/Royal College of Physicians guidelines. J Neurol Neurosurg Psychiatry (2004) 75(suppl 2):ii18–23. doi: 10.1136/jnnp.2004.040360
52. Oberndorfer S, Lindeck-Pozza E, Lahrmann H, Struhal W, Hitzenberger P, Grisold W. The end-of-life hospital setting in patients with glioblastoma. J Palliat Med (2008) 11(1):26–30. doi: 10.1089/jpm.2007.0137
53. Miller JD, Leech P. Effects of mannitol and steroid therapy on intracranial volume- pressure relationships in patients. J Neurosurg (1975) 42(3):274–81. doi: 10.3171/jns.1975.42.3.2074
54. Wen PY, Macdonald DR, Reardon DA, Cloughesy TF, Sorensen AG, Galanis E, et al. Updated response assessment criteria for high-grade gliomas: Response assessment in neuro-oncology working group. J Clin Oncol (2010) 28:1963–72. doi: 10.21037/cco.2017.06.26
55. Brant-Zawadzki M, Berry I, Osaski L, Brasch R, Murovic J, Norman D. Gd-DTPA in clinical MR of the brain. 1. Intra-axial lesions. Am J Neuroradiol (1986) 7:781–8. doi: 10.2214/ajr.147.6.1223
56. Wikipedia. Glioblastoma. (2020). Available at: https://en.wikipedia.org/wiki/Glioblastoma [Accessed April 15, 2020].
57. Monteiro AR, Hill R, Pilkington GJ, Madureira PA. The Role of Hypoxia in Glioblastoma Invasion. Cells (2017) 6(4):45. doi: 10.3390/cells6040045
58. Johnson DR, Fogh SE, Giannini C, Kaufmann TJ, Raghunathan A, Theodosopoulos PV, et al. Case-based review: Newly diagnosed glioblastoma. Neuro Oncol Pract (2015) 2:106–21. doi: 10.1093/nop/npv020
59. Chamberlain MC. Radiographic patterns of relapse in glioblastoma. J Neurooncol (2011) 101:319–23. doi: 10.1007/s11060-010-0251-4
60. Tixier F, Um H, Bermudez D, Iyer A, Apte A, Graham MS, et al. Preoperative MRI-radiomics features improve prediction of survival in glioblastoma patients over MGMT methylation status alone. Oncotarget (2019) 10(6):660–72. doi: 10.18632/oncotarget.26578
61. Salama GR, Heier LA, Patel P, Ramakrishna R, Magge R, Tsiouris AJ. Diffusion Weighted/Tensor Imaging, Functional MRI and Perfusion Weighted Imaging in Glioblastome-Foundations and Future. Front Neurol (2018) 8:660. doi: 10.3389/fneur.2017.00660
62. Verma G, Chawla S, Mohan S, Wang S, Nasrallah M, Sheriff S, et al. Three-dimensional echo planar spectroscopic imaging for differentiation of true progression from pseudo progression in patients with glioblastoma. NRM BioMed (2019) 32(2):e4042. doi: 10.1002/nbm.4042
63. Strauss SB, Meng A, Ebani EJ, Chiang GC. Imaging Glioblastoma Posttreatment: Progression, Psuedoprogression, Pseudoresponse, Radiation Necrosis. Radiol Clin North Am (2019) 57(6):1199–216. doi: 10.1016/j.rcl.2019.07.003
64. Goncalves FG, Chawla C, Mohan S. Emerging MRI Techniques to Redefine Treatment Response in Patients with Glioblastoma. J Magn Reson Imaging (2020) 52:978–97. doi: 10.1002/jmri.27105
65. Crain ID, Elias PS, Chapple K, Scheck AC, Karis JP, Preul MC. Improving the Utility of 1 H-MRS for the differentiation of glioma recurrence from radiation necrosis. Neurooncol (2017) 133(1):97–105. doi: 10.1007/s11060-017-2407-y
66. Ulmer S, Backens M, Ahlhelm F. Basic Principles and Clinical Applications of Magnetic Resonance Spectroscopy in Neuroradiology. J Comput Assist Tomogr (2016) 40(1):1–13. doi: 10.1097/RCT.0000000000000322
67. Ellingson BM, Malkin MG, Rand SD, Connelly JM, Quinsey C, LaViolette PS, et al. Validation of functional diffusion maps (fDMs) as a biomarker for human glioma cellularity. J Magn Reson Imag (2010) 31(3):538–48. doi: 10.1002/jmri.22068
68. Chang PD, Chow DS, Yang PH, Filippi CG, Lignelli A. Predicting Glioblastoma Recurrence by Early Changes in the Apparent Diffusion Coefficient Value and Signal Intensity on FLAIR Images. Am J Roentgenol (2017) 208(1):57–65. doi: 10.2214/AJR.16.16234
69. Mohan S, Wang S, Coban G, Kural F, Chawla S, O’Rourke DM, et al. Detection of Occult Neoplastic Infiltration in the Corpus Callosum and Prediction of Overall Survival in Patients with glioblastoma Using Diffusion Tensor Imaging. Eur J Radiol (2019) 112:106–11. doi: 10.1016/j.ejrad.2019.01.015
70. Van Dijken BRJ, Van Laar PJ, Smits M, Dankbaar JW, Enting RH, van der Hoorn A. Perfusion MRI in treatment evaluation of glioblastomas: Clinical relevance of current and future techniques. J Magn Reson Imag (2019) 49(1):11–22. doi: 10.1002/jmri.26306
71. Bae S, Choi YS, Ahn SS, Chang JH, Kang SG, Kim EH, et al. Radiomic MRI Phenotyping of Glioblastoma. Radiology (2018) 289(3):797–806.
72. Seeger A, Braun C, Skardelly M, Paulsen F, Schittenhelm J, Ernemann U, et al. Comparison of Three Different MR Perfusion Techniques and MR Spectroscopy of Multiparametric Assessment in Distinguishing Recurrent High-Grade Gliomas from Stable Disease. Acad Radiol (2013) 20(12):1557–65. doi: 10.1016/j.acra.2013.09.003
73. Young RM, Jamshidi A, Davis G, Sherman JH. Current trends in the surgical management and treatment of adult glioblastoma. Ann Transl Med (2015) 3(9):121. doi: 10.3978/j.issn.2305-5839.2015.05.10
74. Duffau H. Long-term outcomes after supratotal resection of diffuse low-grade gliomas: a consecutive series with 11-year follow-up. Acta Neurochir (2016) 158:51–8. doi: 10.1007/s00701-015-2621-3
75. Wilson TA, Karajannis MA, Harter DH. Glioblastoma multiforme: State of the art and future therapeutics. Surg Neurol Int (2014) 5:64–2. doi: 10.4103/2152-7806.132138
76. Lacroix M, Abi-Said D, Fourney DR, Gokaslan ZL, Shi W, DeMonte F, et al. A multivariate analysis of 416 patients with glioblastoma multiforme: prognosis, extent of resection, and survival. J Neurosurg (2001) 95(2):190–8. doi: 10.3171/jns.2001.95.2.0190
77. Mukherjee D, Quiñones-Hinojosa A. Impact of extent of resection on outcomes in patients with high-grade gliomas. In: Hayat MA, editor. Tumors of the central nervous system, vol. 2 . San Francisco, CA: Springer (2011). p. 173–9. doi: 10.1007/978-94-007-0618-7_19
78. Orringer D, Lau D, Khatri S, Zamora-Berridi GJ, Zhang K, Wu C, et al. Extent of resection in patients with glioblastoma: limiting factors, perception of resectability, and effect on survival. J Neurosurg (2012) 117:851–9. doi: 10.3171/2012.8.JNS12234
79. Pino MA, Imperato A, Musca I, Maugeri R, Giammalva GR, Costantino G, et al. New Hope in Brain Glioma Surgery: The Role of Intraoperative Ultrasound. A Rev Brain Sci (2018) 8(11):202. doi: 10.3390/brainsci8110202
80. Davis ME. Glioblastoma: Overview of Disease and Treatment. Clin J Oncol Nurs (2016) 20(5 Suppl):S2–8. doi: 10.1188/16.CJON.S1.2-8
81. Laperriere N, Zuraw L, Cairncross G, Cancer Care Ontario Practice Guidelines Initiative Neuro-Oncology Disease Site Group. Radiotherapy for newly diagnosed malignant glioma in adults: a systemic review. Radiother Oncol (2002) 64:259–73. doi: 10.1016/s0167-8140(02)00078-6
82. Blumenthal DT, Gorlia T, Gilbert MR, Kim MM, Nabors LB, Mason WP, et al. Is more better? The impact of extended adjuvant temozolomide in newly diagnosed glioblastoma: a secondary analysis of EORTC and NRG Oncology/RTOG. Neuro Oncol (2017) 19(8):1119–26. doi: 10.1093/neuonc/nox025
83. Balana C, Vaz MA, Sepúlveda JM, Mesia C, Del Barco S, Pineda E, et al. A phase II randomized, multicenter, open-label trial of continuing adjuvant temozolomide beyond six cycles in patients with glioblastoma (GEINO 14-01). Neuro Oncol (2020) noaa107. doi: 10.1093/neuonc/noaa107
84. Fan CH, Liu WL, Cao H, Wen C, Chen L, Jiang G. O6-methylguanine DNA methyltransferase as a promising target for the treatment of temozolomide-resistant gliomas. Cell Death Dis (2013) 4(10):e876. doi: 10.1038/cddis.2013.388
85. Watts GS, Pieper RO, Costello JF, Peng YM, Dalton WS, Futscher BW. Methylation of discrete regions of the O6-methylguanine DNA methyltransferase (MGMT) CpG island is associated with heterochromatinization of the MGMT transcription start site and silencing of the gene. Mol Cell Biol (1997) 17:5612–9. doi: 10.1128/mcb.17.9.5612
86. Nakamura M, Watanabe T, Yonekawa Y, Kleihues P, Ohgaki H. Promoter methylation of the DNA repair gene MGMT in astrocytomas is frequently associated with G:C ->A: T mutations of the TP53 tumor suppressor gene. Carcinogenesis (2001) 22(10):1715–9. doi: 10.1093/carcin/22.10.1715
87. Pandith AA, Qasim I, Zahoor W, Shah P, Bhat AR, Sanadhya D, et al. Concordant association validates MGMT methylation and protein expression as favorable prognostic factors in glioma patients on alkylating chemotherapy (Temozolomide). Sci Rep (2018) 8(1):6704. doi: 10.1038/s41598-018-25169-2
88. Herrlinger U, Tzaridis T, Mack F, Steinbach JP, Schlegel U, Sabel M, et al. Lomustine-temozolomide combination therapy vs standard temozolomide therapy in patients with newly diagnosed glioblastoma with methylated MGMT promoter (CeTeG/NOA-09): a randomized, open-label, phase 3 trial. Lancet (2020) 393(10172):678–88. doi: 10.1016/S0140-6736(18)31791-4
89. Okada M, Miyake K, Tamiya T. Glioblastoma Treatment in the Elderly. Neurol Med Chir (Tokyo) (2017) 57(12):667–76. doi: 10.2176/nmc.ra.2017-0009
90. Iwamoto FM, Abrey LE, Beal K, Gutin PH, Rosenblum MK, Reuter VE, et al. Patterns of 92. relapse and prognosis after bevacizumab failure in recurrent glioblastoma. Neurology (2009) 73(15):1200–6. doi: 10.1212/WNL.0b013e3181bc0184
91. Chinot OL, Wolfgang W, Mason W, Henriksson R, Saran F, Nishikawa R, et al. Bevacizumab plus radiotherapy-temozolomide for glioblastoma multiforme. N Engl J Med (2014) 370:709–22. doi: 10.1056/NEJMoa1308345
92. Gilbert MR, Dignam JJ, Armstrong TS, Wefel JS, Blumenthal DT, Vogelbaum MA, et al. A randomized trial of bevacizumab for newly diagnosed glioblastoma. N Engl J Med (2014) 370:699–708. doi: 10.1056/NEJMoa1308573
93. Paez-Ribes M, Allen E, Hudock J, Takeda T, Okuyama H, Vinals F, et al. Antiangiogenic therapy elicits malignant progression of tumors to increased local invasion and distant metastasis. Cancer Cell (2009) 15(3):220–31. doi: 10.1016/j.ccr.2009.01.027
94. Kamath SD, Kumthekar PU. Immune Checkpoint Inhibitors for the Treatment of Central Nervous System (CNS) Metastatic Disease. Front Oncol (2018) 8:414. doi: 10.3389/fonc.2018.00414
95. Antunes AR, Scheyltjens I, Duerinck J, Neyns B, Movahedi K, Ginderachter JA. Understanding the Glioblastoma Immune Microenvironment on Basis for the Development of New Immunotherapeutic Strategies. Elife (2020) 9:e52176. doi: 10.7554/elife.52176
96. Cloughesy TF, Mochizuki AY, Orpilla JR, Hugo W, Lee AH, Davidson TB, et al. Neoadjuvant anti-PD-1 immunotherapy promotes a survival benefit with intratumoral and systemic immune responses in recurrent glioblastoma. Nat Med (2019) 25(3):477–86. doi: 10.1038/s41591-018-0337-7
97. Zhao J, Chen AX, Gartrell RD, Silverman AM, Aparicio L, Chu, et al. Immune and genomic correlates of response to anti-PD-1 immunotherapy in glioblastoma. Nat Med (2019) 25:462–9. doi: 10.1038/s41591-019-0349-y
98. Schalper KA, Rodriguez-Ruiz ME, Diez-Valle R, Lopez-Janeiro A, Porciuncula A, Idoate MA, et al. Neoadjuvant nivolumab modifies the tumor immune microenvironment in resectable glioblastoma. Nat Med (2019) 25(3):470–6. doi: 10.1038/s41591-018-0339-5
99. Bristol Myers Squibb. Bristol-Myers Squibb Announces Phase 3 CheckMate -498 Study Did Not Meet Primary Endpoint of Overall Survival with Opdivo (nivolumab) Plus Radiation in Patients with Newly Diagnosed MGMT-Unmethylated Glioblastoma Multiforme (2019). (Accessed 3 August 2020).
100. Bristol Myers Squibb. provides update on Phase 3 Opdivo (nivolumab) CheckMate-548 trial in patients with newly diagnosed MGMT-methylated glioblastoma multiforme (2019). (Accessed 3 August 2020).
101. Branter J, Basu S, Smith S. Tumour treating fields in a combinational therapeutic approach. Oncotarget (2018) 9(93):36631–44. doi: 10.18632/oncotarget.26344
102. Stupp R, Taillibert S, Kanner A, Read W, Steinberg D, Benoit L, et al. Effect of tumor-treating fields plus maintenance temozolomide vs maintenance temozolomide alone on survival in patients with glioblastoma: a randomized clinical trial. Jama (2017) 318:2306–16. doi: 10.1001/jama.2017.18718
103. Lukas RV, Ratermann KL, Wong ET, Villano JL. Skin toxicities associated with tumor treating fields: case-based review. J Neurooncol (2017) 135:593–99. doi: 10.1007/s11060-017-2612-8
104. Wick W. TTFields: where does all the skepticism come from? Neuro Oncol (2016) 18(3):303–5. doi: 10.1093/neuonc/now012
105. Liau LM, Ashkan K, Tran DD, Campian JL, Trusheim J, Cobbs CS, et al. First results on survival from a large Phase 3 clinical trial of an autologous dendritic cell vaccine in newly diagnosed glioblastoma. J Transl Med (2018) 16(1):142. doi: 10.1186/s12967-018-1507-6
106. Ahluwalia MS, Reardon DA, Abad AP, Curry WT, Wong ET, Belal A, et al. SurVaxM with standard therapy in newly diagnosed glioblastoma: Phase II trial update. J Clin Oncol (2019) 37(Suppl 15):2016. doi: 10.1200/JCO.2019.37.15_suppl.2016
107. Lassman A, Pugh S, Wang T, Aldape K, Gan H, Preusser M, et al. A randomized, double-blind placebo-controlled Phase 3 trial of Depatuxizumab mafodotin (ABT-414) in epidermal growth factor receptor (EGFR) amplified newly diagnosed glioblastoma. Neuro-Oncology (2019) 21(Suppl 6):vi17. doi: 10.1093/neuonc/noz175.064
108. van den Bent MJ, Gao Y, Kerkhof M, Kros JM, Gorlia T, van Zwieten K, et al. Epidermal growth factor receptor (EGFR) amplification rates observed in screening patients for randomized clinical trials in glioblastoma. Ann Oncol (2017) 28(suppl_5):v100. doi: 10.1007/s11060-019-03222-y
109. Wen PY, Chang SM, Lamborn KR, Kuhn JG, Norden AD, Cloughesy TF, et al. Phase I/II study of erlotinib and temsirolimus for patients with recurrent malignant gliomas: North American brain tumor consortium trial 04-02. Neuro-oncol (2014) 16:567–78. doi: 10.1093/neuonc/not247
110. Lee A, Arasaratnam M, Chan DL, Khasraw M, Howell VM, Wheeler H. Anti-epidermal growth receptor for glioblastoma in adults. Cochrane Database Syst Rev (2020) 5(5):CD013238. doi: 10.1002/14651858.CD013238.pub2
111. Wen P, Weller M, Lee E, Alexander B, Barnholtz-Sloan J, Barthel F, et al. Glioblastoma in adults: a Society for Neuro-Oncology (SNO) and European Society of Neuro-Oncology (EANO) Consensus Review on Current Management and Future Directions. Neuro Oncol (2020) 22(8):1073–113. doi: 10.1093/neuonc/noaa106
112. Khasraw M, McDonald KL, Rosenthal M, Lwin Z, Ashley DM, Wheeler H, et al. A randomized phase II trial of veliparib, radiotherapy and temozolomide in patients with unmethylated MGMT glioblastoma. J Clin Onc (2019) 37(Suppl 15):2011. doi: 10.1200/JCO.2019.37.15_suppl.2011
113. Wick W, Dettmer S, Berberich A, Kessler T, Karapanagiotou-Schenkel I, Wick A, et al. N2M2 (NOA-20) phase I/II trial of molecularly matched targeted therapies plus radiotherapy in patients with newly diagnosed non-MGMT hypermethylated glioblastoma. Neuro Oncol (2019) 21(1):95–105. doi: 10.1093/neuonc/noy161
114. Mann J, Ramakrishna R, Magge R, Wernicke AG. Advances in Radiotherapy for Glioblastoma. Front Neurol (2018) 8:748. doi: 10.3389/fneur.2017.00748
115. Liu EK, Sulman EP, Wen PY, Kurz C. Novel Therapies for Glioblastoma. Curr Neurol Neurosci Rep (2020) 20:19. doi: 10.1007/s11910-020-01042-6
116. Lapointe S, Mason W, MacNeil M, Harlos C, Tsang R, Sederias J, et al. A phase I study of vistusertib (dual mTORC1/2 inhibitor) in patients with previously treated glioblastoma multiforme: a CCTG study. Invest New Drugs (2020) 38:1137–44. doi: 10.1007/s10637-019-00875-4
117. Raucher D. Tumor targeting peptides: novel therapeutic strategies in glioblastoma. Currently Open Pharmacol (2019) 47:14–9. doi: 10.1016/j.coph.2019.01.1006
118. Jacques FH, Nicholas G, Lorimer I, Sikatifoko V, Prevost J. Avelumab in newly diagnosed glioblastoma multiform: The SEJ study. JCO (2019) 37:15–suppl. doi: 10.1200/JCO.2019.37.15_suppl.e13571
119. Shen SH, Woroniecka K, Barbour AB, Fecci PE, Sanchez-Perez L, Sampson JH. CAR T cells and checkpoint inhibition of the treatment of glioblastoma. Expert Opin Biol There (2020) 20:579–91. doi: 10.1080/14712598.2020.1727436
120. Gholamin S, Yossuef OA, Rafat M. Irradiation or temozolomide chemotherapy enhancesanti-CD47 treatment of glioblastoma. Innate Immune (2020) 26(2):130–7. doi: 10.1177/1753425919876690
Keywords: glioblastoma, radiation therapy, high-grade gliomas, cancer, brain tumors
Citation: Oronsky B, Reid TR, Oronsky A, Sandhu N and Knox SJ (2021) A Review of Newly Diagnosed Glioblastoma. Front. Oncol. 10:574012. doi: 10.3389/fonc.2020.574012
Received: 08 July 2020; Accepted: 28 September 2020;
Published: 05 February 2021.
Edited by:
Sandro M. Krieg, Technical University of Munich, GermanyReviewed by:
Antonio Silvani, Carlo Besta Neurological Institute (IRCCS), ItalyJennie W. Taylor, University of California, San Francisco, United States
Copyright © 2021 Oronsky, Reid, Oronsky, Sandhu and Knox. This is an open-access article distributed under the terms of the Creative Commons Attribution License (CC BY). The use, distribution or reproduction in other forums is permitted, provided the original author(s) and the copyright owner(s) are credited and that the original publication in this journal is cited, in accordance with accepted academic practice. No use, distribution or reproduction is permitted which does not comply with these terms.
*Correspondence: Susan J. Knox, sknox@stanford.edu