- 1Radiology Division, Istituto Nazionale Tumori, IRCCS, Fondazione G. Pascale, Naples, Italy
- 2Radiology Division, Universita’ Degli Studi Della Campania Luigi Vanvitelli, Naples, Italy
- 3Hepatobiliary Surgical Oncology Division, Istituto Nazionale Tumori, IRCCS, Fondazione G. Pascale, Naples, Italy
- 4Department of Radiology, Careggi University Hospital, Florence, Italy
- 5Department of Medicine and Health Sciences “V. Tiberio”, University of Molise, Campobasso, Italy
This article provides an overview of imaging assessment of ablated pancreatic cancer. Only studies reporting radiological assessment on pancreatic ablated cancer were retained. We found 16 clinical studies that satisfied the inclusion criteria. Radiofrequency ablation and irreversible electroporation have become established treatment modalities because of their efficacy, low complication rates, and availability. Microwave Ablation (MWA) has several advantages over radiofrequency ablation (RFA), which may make it more attractive to treat pancreatic cancer. Electrochemotherapy (ECT) is a very interesting emerging technique, characterized by low complication rate and safety profile. According to the literature, the assessment of the effectiveness of ablative therapies is difficult by means of the Response Evaluation Criteria in Solid Tumors (RECIST) criteria that are not suitable to evaluate the treatment response considering that are related to technique used, the timing of reassessment, and the imaging procedure being used to evaluate the efficacy. RFA causes various appearances on imaging in the ablated zone, correlating to the different effects, such as interstitial edema, hemorrhage, carbonization, necrosis, and fibrosis. Irreversible electroporation (IRE) causes the creation of pores within the cell membrane causing cell death. Experimental studies showed that Diffusion Weigthed Imaging (DWI) extracted parameters could be used to detect therapy effects. No data about functional assessment post MWA is available in literature. Morphologic data extracted by Computed Tomography (CT) or Magnetic Resonance Imaging (MRI) do not allow to differentiate partial, complete, or incomplete response after ECT conversely to functional parameters, obtained with Position Emission Tomography (PET), MRI, and CT.
Introduction
Oncology disease is the second principal cause of death in both men and women. Incidence continues to increase for pancreatic cancer, with an estimated death rate of 81.7% among new cases of 2020 and a 5-year relative survival rate of the 9% (1). The decision regarding resectability status of pancreatic cancer should be made by the multidisciplinary meetings consensus following the acquisition of pancreatic imaging including complete staging. In fact, most patients had locally advanced or metastatic disease at diagnosis, and systemic chemotherapy is usually the main treatment (2–6). Most patients experience relapse after treatment. Furthermore, the “cure rate” for this disease is only 9%, and without treatment, the median survival of patients with metastatic disease is only 3 months. First-line treatment regimens consists of FOLFIRINOX and gemcitabine/albumin-bound nab-paclitaxel, and for patients with BRCA1/2 and PALB2 mutations, gemcitabine/cisplatin. Compared with nab-paclitaxel/gemcitabine, FOLFIRINOX may be associated with a somewhat better response rate and progression-free and overall survival (OS), but it is a difficult regimen that is best reserved for fit patients (3). Despite the latest introduction of new treatment schemes, chemotherapy in advanced pancreatic cancers still correlates to an unfortunate long-term survival and considerable ad interim complications (6, 7). The resectability assessment of Locally Advanced Pancreatic Cancer (LAPC) after neoadjuvant therapy is still challenging. In dedicated cancer centers, patients with persistent LAPC after chemotherapy should be subjected to local treatment if they are in good clinical condition (WHO Performance Status 0–1) and according to Response Evaluation Criteria in Solid Tumors (RECIST) in stable disease after 2–4 months chemotherapy. However, randomized trials to assess the ablative therapies additional value to chemotherapy-alone are lacking and currently there are no completed trials comparing multiple ablative approaches (8). Additionally, there is increasing suggestion that local ablative therapies can induce a systemic anti-tumor response (8).
Today ablative therapies should be used as consolidative treatment in stable disease (9). Assessment after ablative treatment is complicated and is related to the type of treatment used (10–15). Radiofrequency ablation (RFA) and microwave ablation (MWA) are hyperthermic tools that use energy to heat the target area to at least 60°C (16, 17). Although the technological features of RFA and MWA are comparable, the differences occur from the physical phenomenon used to create heat. In fact, RFA is based on thermocoagulation necrosis, while MWA causes cellular death thanks to dielectric heating (16, 17).
The cell membrane permeability changes induced by the application of an external electric field is called electroporation. Electroporation can be applied in either an irreversible (IRE) (14, 18–28) or a reversible manner (11–13, 29, 30), depending on the electrical field strength and duration. IRE is based on alteration of the transmembrane potential, causing the disruption of the lipid bilayer by the creation of small pores (“nanopores”), thus driving the cells toward apoptosis (23). Reversible electroporation can be used in combination with administration of a chemotherapeutic drug (ECT) or also gene therapy and vaccination (Electrogenetransfer, EGT). ECT is based on the electroporation of cells and the associated administration of low doses of a chemotherapeutic agent, especially bleomycin (BLM). An external electrical field is applied to the cell membrane inducing a transient and reversible orientation of its polar molecules, consequently there is an increase in cell permeability with a higher dose of chemotherapeutic agent that can penetrate (11). ECT determines a direct toxic phenomenon and an anti-vascular effect. “This so called ‘vascular lock’ effect retains the chemotherapeutic agent in the treatment area thereby increasing the treatment effect further” (31). “Furthermore, the type of cell death that is mediated is dependent on the number of intracellular BLM molecules. A few hundred to few thousand molecules lead to a slow mitotic cell death and more internalized molecules lead to a faster pseudoapoptotic cell death” (32).
Several therapies both thermal and non-thermal have the ability to stimulate anti-tumor immunity. The immune-modulatory response evidence is currently the strongest related to radiotherapy, although data is accumulating for high-intensity focused ultrasound, radiofrequency ablation, reversible and irreversible electroporation (33–35).
RECIST are inappropriate to assess locoregional therapies, since existing morphologic response criteria do not offer the sufficient data to assess the efficacy of treatment. Therefore, establishment of response evaluation criteria devoted to ablation therapies is needed in clinical practice, as well as in clinical trials. According to García-Figueiras et al. functional features could predict treatment success before size changes become evident (15).
Our purpose is reporting an overview and update of imaging techniques in the response assessment to ablative therapies in pancreatic cancer.
Methods
This overview is the result of a self-study without protocol and registration number.
Search Criterion
We assessed several electronic databases: PubMed (US National Library of Medicine, http://www.ncbi.nlm.nih.gov/pubmed), Scopus (Elsevier, http://www.scopus.com/), Web of Science (Thomson Reuters, http://apps.webofknowledge.com/), and Google Scholar (https://scholar.goo-gle.it/). The following search criteria have been used: “Pancreatic Cancer” AND “Ablative Therapies” AND “Imaging Assessment", “Pancreatic Cancer” AND “RFA” AND “Imaging Assessment, “Pancreatic Cancer” AND “MWA” AND “Imaging Assessment, “Pancreatic Cancer” AND “IRE” AND “Imaging Assessment, “Pancreatic Cancer” AND “ECT” AND “Imaging Assessment.” According to our personal decision to assess functional imaging in evaluating ablation treatment, and since only in the last 10 years these diagnostic tool have reached their applicability, the search covered the years from January 2010 to May 2020. Moreover, the references of the found papers were evaluated for papers not indexed in the electronic database. We analyzed all titles and abstracts. The inclusion criteria was: clinical study evaluating radiological assessment of pancreatic cancer after ablative therapies. Articles published in the English language from January 2010 to May 2020 were included. Exclusion criteria were studies with no sufficient reported data, case report, review or editorial letter.
Results
We recognized 140 studies that assessed ablation treatment in pancreatic cancer from January 2010 to May 2020. Ninety-one studies have different topic in respect to the radiological assessment; 5 did not have sufficient data and 8 are case report, review, or letter to editors; so 36 articles were included at the end (Figure 1). We included 18 papers for RFA, 3 paper for MWA, 11 paper for IRE, and 4 paper for ECT. Table 1 reports the mean value and the range of overall survival and the mean value of major complication rates, minor complication rates, mortality rate, and imaging analysis in pancreatic cancer treated with ablation therapies. For IRE and ECT we reported the data of the researches that have assessed significant study population, while for RFA and MWA less patients have been treated so we reported mean value considering each included study.
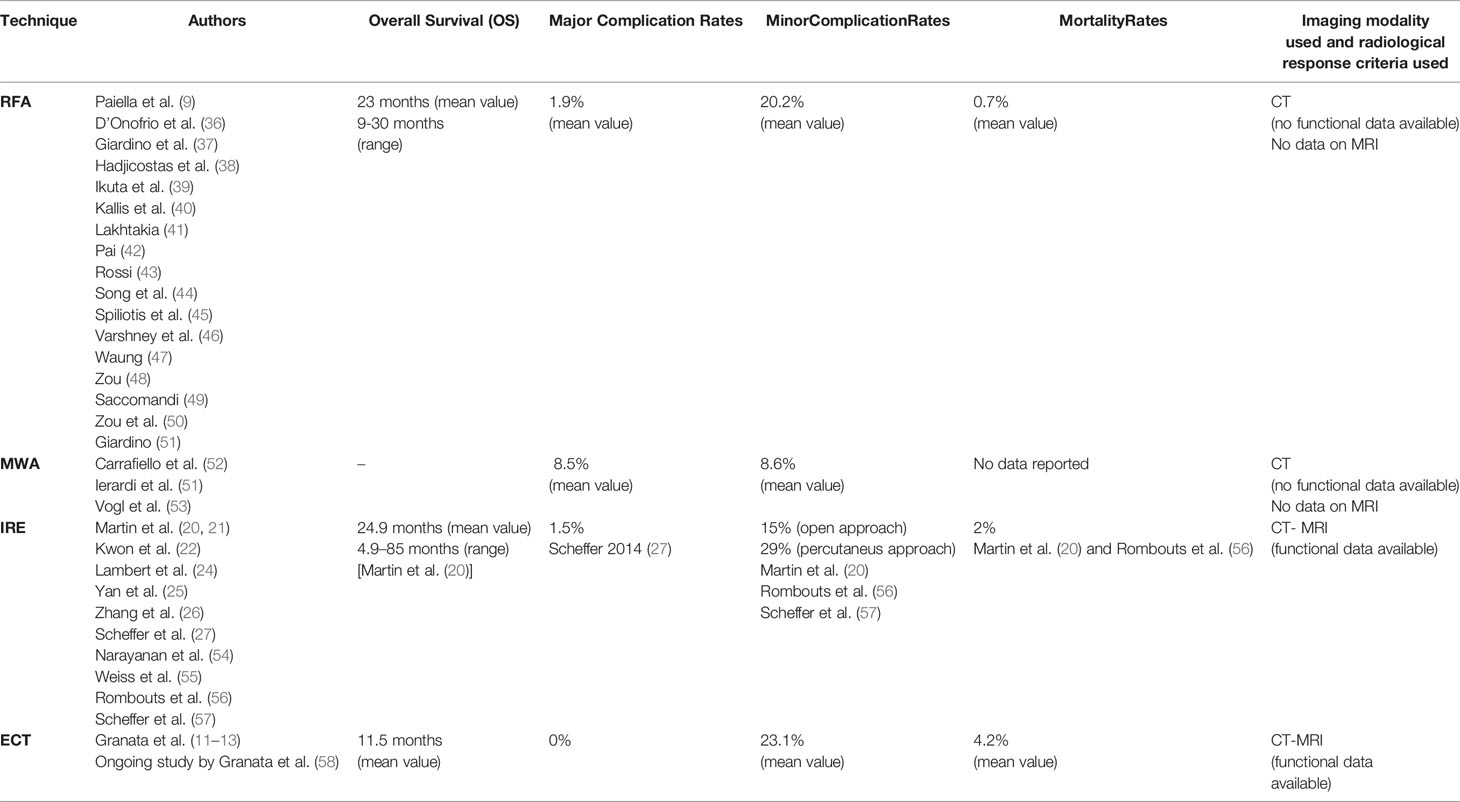
Table 1 Mean value and the range of overall survival and the mean value of major complication rates, minor complication rates, mortality rate, and imaging analysis in pancreatic cancer treated with ablation therapies.
Radiofrequency Ablation
During RFA, the zone of active tissue heating is restricted to a few millimeters around the active needle, with the remainder of the ablation zone being heated via thermal conduction (16). The treatment effectiveness is related to the target size, with the best result for lesions with a size smaller than 3.5 cm (16, 17). Also, some structural characteristics of biological tissues, such as electrical and thermal conductivity, dielectric permittivity, and blood perfusion rate, have effect on the growth of ablation area. The coagulation necrosis extent is linked to the energy deposited correlated to the hepatic blood flow that with its cooling properties affected tumor ablation; this phenomenon is commonly termed “heat sink effect” (20). The heat-sink effect limits the all thermal ablation method’s effectiveness since the combined effect of electrical and thermal sink increases the incomplete necrosis risk (17–20, 59).
Today the most application of RFA on pancreatic cancer is the treatment of patients with stage-III, in case of no further systemic therapies response. However, some studies included also stage-IV patients (9). At the best of our knowledge, 18 papers assessed RFA in clinical setting (Table 1) (9, 36–51). In most patients RFA was reserved to stages III–IV, and in lower stage in 22 unfit-for-surgery patients. RFA was performed in 158/279 (56.6%) head lesions, in 68/279 (24.4%) body-tail lesions, and in 2 uncinate process lesions. Computed Tomography (CT) scan was the diagnostic tool mostly used to assess the treatment. Fifty-two complications were reported (Table 1). The most frequent were pancreatic fistula (12 cases), portal thrombosis (10 cases), and pancreatitis (8 cases). In three patients was reported duodenal injury, and in two patients abdominal bleeding. Two deaths were registered due to hepatic failure (49). In a recent review Paiella et al. reported a good oncological outcome obtained with the use of RFA on pancreatic cancers with a median OS of 30 months for patients treated with RFA, median OS of 25.6 months in the group treated RFA plus systemic therapy (Table 1) (9).
Recently, RFA is used as an upfront option, justified on the basis of an immunological antitumoral stimulation (50, 60).
Microwave Ablation
MWA determines a larger zone of active heating (up to 2 cm surrounding the antenna) obtaining more uniform necrosis of the lesion. MWA benefits compared to RFA are: lesion size can be larger for larger area of necrosis determined by MWA; the treatment time is shorter (16). Carrafiello et al. (52) assessed MWA in 10 patients in stage IV, with lesion located in the head of the pancreas (Table 1). During the follow-up (mean time 9.2 months, range 3–16 months), the major complications rate was 30% (3 patients). Two patients developed pancreatitis and one patient pseudoaneurysm of the gastroduodenal artery. CT scan was performed up to 15 months after the treatment (Table 1). No patients showed a complete response. At 1 month follow-up there were found 1 progressive disease (PD), 1 partial response (PR), and 8 stable diseases (SD). Ierardi et al. (51) assessed feasibility and safety of MWA in LAPC using a new technology of MW with high power (100 W) and frequency of 2,450 MH. They treated five patients with pancreatic head cancer. Follow-up was performed by CT after 1, 3, 6, and, when possible, 12 months. The treatment was feasible in all patients (100%), observing no major complications. Minor complications resolved during the hospital stay (4 days) (Table 1). An improvement in Quality of Life (QoL) was observed in all patients (51). Vogl et al. (53) treated 20 pancreatic cancer patients. Seventeen lesions (77.3%) of pancreatic head cancer and 5 (22.7%) of body-tail. The efficacy reported was 100%, without major complications. Minor complications were found in 2 patients (9.1%) (severe local pain correlated to the treatment). PD was documented in one case (10%) of the 10/22 accessible 3-month follow-up MR examinations (Table 1).
Altogether, MWA shows promising results, however, it needs further data to improve the knowledge about the efficacy, the safety, and the oncological outcome.
Irreversible Electroporation
IRE induces an electric field across cells in order to alter the cellular transmembrane potential. When a sufficiently high voltage is reached, the cell membrane phospholipid bilayer structure is disrupted, inducing cell apoptosis. The evidence suggests that IRE “leaves supporting tissue largely unaffected, preserving the structure of large blood vessels and bile ducts” (16, 17, 19). Since IRE efficacy is linked to electrical energy delivered; therefore its efficacy is not influenced by the heat-sink effect (16, 17, 19). This suggests safer and more effective ablation of neoplasms adjacent to large vessels or fragile structures (9–18, 20–22, 24–27, 54–56, 59).
Considering this, IRE preserves surrounding tissues and protect the vessels; this characteristic would be an essential feature when the lesion encases the major peripancreatic vessels, in which the use of thermal treatment could be unsafe and inefficacious (9–18, 20–22, 24–27, 54–56, 59).
Currently, IRE is used on stage-III LAPC (18, 27). Narayanan et al. reported three cases of IRE on stage IV (45). Also, several researchers reported the possibility to use IRE, as a technique to reduce R1 resections rate (20, 22, 54, 55). For IRE, two to six electrodes are typically placed around the tumor, with a maximum spacing of 2.0–2.5 cm. IRE has the disadvantage of necessity of general anesthesia. Rombouts et al., in a systematic review, reported complication rate of 13%, and a mortality of 2% (56). The complication rate increases with percutaneous approach (29 vs 15%) (20, 56, 57). Martin et al., assessing 200 treated patients, showed an overall rate of adverse events of 37% and a mortality rate of 2% (Table 1) (20). The most common complications described are pancreatitis, abdominal pain, bile leakage, pancreatic leakage, duodenal leakage, duodenal ulcer, pneumothorax, hematoma, and deep vein thrombosis (Table 1) (6). MR and CT were the diagnostic tool mostly used to assess the treatment. Despite the large number of studies on IRE in pancreatic cancer, only Martin et al. (20) reported an outstanding median Overall Survival (OS) of 24.9 months (range 12.4–85 months). Consequently, there is a need for a greater number of studies that evaluate efficacy in terms of oncological outcomes (Table 1).
Electrochemotherapy
ECT is based on the electroporation of cells and the associated administration of low doses of chemotherapy. An external electric field to a cell induces a transient and reversible increase of cells transmembrane potential with a consequent increase of permeability (11–13, 30–32). Formation of the aqueous pores in the lipid bilayer is the widely recognized mechanism, but evidence is growing that individual membrane lipids and proteins changes also contribute at ECT cytotoxic effect (61). The increased accumulation of intracellular drug concentration has actually been shown both in vitro and in vivo (58, 62–65).
In the clinical setting few papers assessed the safety and efficacy of ECT in LAPC (11–13). Granata et al. (11) evaluated 13 patients with confirmed diagnosis of LAPC (stage III). In 53.8% (7/13) the lesion was on head and in 46.2% (6/13) the lesion was on body-tail. ECT was well tolerated with rapid resolution (4–8 days) of the abdominal pain. No serious adverse events occurred. No heart abnormalities were reported. No clinically significant hemodynamic or serum biologic changes were noted during or following ECT (Table 1). CT and MR were employed for the follow-up at 1, 3, 6, and 12 months. In an ongoing study, Granata et al. (58) showed that median OS was 11.5 months with range values of 73 months. At 1 month after ECT 76.0% of patients were in PR and 20.0% were in SD. Today, ECT is recommended during clinical studies in dedicated centres (11–13).
Imaging Analysis
The precise detection and characterization of pancreatic lesion is still difficult. CT and MRI are the main used modalities to assess pancreatic lesions and CT has become the modality of choice in the preoperative setting and staging, so as in treatment planning and follow-up (5). However, approximately 11% of ductal adenocarcinomas are undetected at CT (10). Morever, the pancreatic cancer assessment after neoadjuvant therapy is particularly difficult and as suggested by White et al., CT would miscalculate the resectability, since diagnostic performance seems to be reduced after therapy. Therefore, there are not radiological criteria in order to assess treatment response correlated to histological response (66). The situation becomes more complicated when evaluating effectiveness of ablative therapies, considering that RECIST criteria were not suitable to assess the response (67).
Dimensional Criteria
RECIST 1.1, based on the variation of largest diameter, do not allow to stratify the patients in responders or non-responders after ablation treatment, since after these therapies it is expected that there is an increase in the size of the ablated area. In fact, the primary endpoint of ablation therapy is to obtain a complete necrosis (similar to R0 resection) of liver tumors that is linked to create a safety margin of at least 10 mm round the external margin of the lesion (16, 68). Moreover, the nature of the pancreatic cancer, consisting of a more or less great quantity of cells fixed within a dense and fibrous stroma, reduce diagnostic accuracy when the treated area is measured (69). After effective therapy, it is difficult the differentiation between neoplastic cells and fibrosis and then it is difficult the evaluation with morphological criteria of therapy response. Moreover, a possible locoregional edema induced by treatment or inflammatory changes secondary to biliary drainage could be observed. Therefore, the treatment response evaluation for this cancer type is a serious challenge and the dimensional criteria are unsuitable (69).
Perfusional Assessment
Perfusion CT (CTp) can provide images and quantitative measurements of hemodynamic parameters based on the linear relationship between CT enhancement and iodinated contrast agent concentration (10). Several studies evaluated perfusion CT parameters to characterize and to evaluate the treatment in patient affected by pancreatic cancer; these studies demonstrates that CTp is more able to differentiate the pancreatic disorder respect to density measurements alone. However, no significant differences in the perfusion parameters values were found between acute-chronic pancreatitis and pancreatic adenocarcinoma, then the differential diagnosis by CTp data remains difficult (10).
Dynamic contrast-enhanced (DCE)-MRI allows the calculation of quantitative parameters linked to tumor perfusion, vessel permeability and extracellular-extravascular space composition by the post processing with pharmacokinetic models of the changes in signal intensity over time after the paramagnetic contrast medium injection (69). DCE-MRI can be analyzed by qualitative, semi-quantitative, and quantitative methods (69). DCE-MRI accuracy in the evaluation of pancreatic cancer remains unclear, probably due to the fact that in pancreatic cancer, “poorly represented microvascular components could be clarified by vessel functional impairment often observed in tumors, and by the presence of a prominent stromal matrix that embeds vessels. In addition, activated pancreatic stellate cells yield increasing fibrous stroma in tumor central areas, compressing blood vessels, leading to changes in vascularity and perfusion” (69).
Diffusion Weighted Imaging Assessment
The opportunity to obtain functional parameters by Diffusion Weighted Imaging (DWI) has facilitated the spread of this technique into clinical practice, increasing clinical confidence and decreasing false positives in the detection and characterization of lesions. DW data analysis can be done qualitatively and quantitatively, through the apparent diffusion coefficient (ADC) evaluation using a mono-exponential model at the signal intensity decay over the diffusion b values. DWI signal is linked to water mobility that related to tissue density (69). ADC can be used in the differentiation between benign and malignant tissue. Instead, the Intravoxel incoherent motion (IVIM) method used a more sophisticated process, a bi-exponential model to separately calculate the macroscopic mobility of water movement (contribution to diffusion), and microscopic movement of blood in capillaries (contribution of perfusion). Also IVIM parameters can be analyzed qualitatively and quantitatively (69). Moreover, according to the presence of microstructures, water molecules within biologic tissues exhibit a non-Gaussian phenomenon known as Diffusion Kurtosis Imaging (DKI) (69). Therefore, is possible the calculation of the kurtosis coefficient (K) linked to the deviance of diffusion from a Gaussian approach, and the diffusion coefficient (D) with the correction of non-Gaussian bias.
Since necrosis and perfusion modifications can happen before changes in size during therapy, DWI may aid as an early biomarker of treatment effectiveness (69).
Granata et al. showed that the perfusion-related factors extracted by DWI of pancreatic cancer, perfusion fraction (fp) and pseudo-diffusion coefficient (Dp) (linked to tumoral perfusion), mean diffusivity (MD) (linked to heterogeneous diffusion motion of water molecules in cells interstitial space) values are different from those found in normal pancreatic parenchyma and in peritumoral tissue; in addition these parameters showed better diagnostic performance than ADC (linked both perfusion and diffusion effects). The significantly different of perfusion-related factors value between cancer tissue and normal pancreatic parenchyma might be helpful for determining the most accurate diagnosis. Increased fp and MD values in peritumoral inflammation seem to suggest that DWI-derived parameters fit in the anticipated physiologic phenomena. These findings support the hypothesis that the kurtosis effect could have a better performance to differentiate pancreatic tumors, peritumoral inflammatory tissue, and normal pancreatic parenchyma (69).
Radiomics
The extraction of innumerable quantitative features by biomedical images such as CT, MR, or positron emission tomography (PET) images is known as Radiomics. These features provide data on tumor phenotype as well as cancer microenvironment. The main challenge is the collection and optimal combination of different multimodal data sources in a quantitative method that provides unambiguous clinical parameters that allow in a precise and robust way the prediction of the results according to the upcoming decisions (70). The central hypothesis of radiomics is that individual quantitative voxel-based variables are more sensitively associated with various clinical endpoints than the more qualitative radiological and clinical data more commonly used today (70).
Findings on Radiological Therapeutic Responses to Treatments
It is clear that, considering therapeutic responses to treatments, imaging data are sometimes complicated to understand because it depend on anatomic location, on the method of act of given therapy, on the morphological and functional criteria that are used for each imaging modality (15). In this setting, imaging observations depend highly on the type and method of therapy delivery, the timing of treatment, and the imaging technique being used to observe the effects.
RFA causes heterogeneous appearances on imaging in the ablated areas, correlated to the therapy effects, such as interstitial edema, hemorrhage, carbonization, necrosis, and fibrosis (Figure 2).
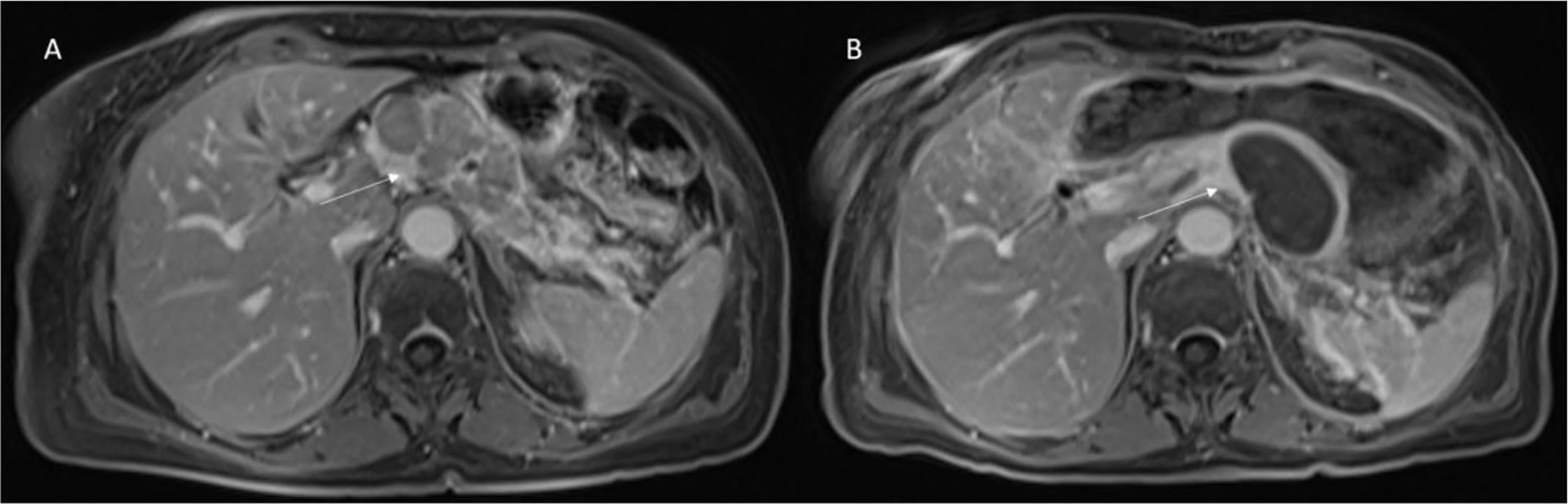
Figure 2 Patient 1 with Body-Tail Pancreatic Cancer. Morphological MRI assessment post-RFA treatment. In (A) (VIBE T1-W post-contrast sequence during portal phase in axial plane) pre-treatment evaluation of lesion (arrow). In (B) (VIBE T1-W post-contrast sequence during portal phase in axial plane) arrow shows ablated area. Qualitative assessment shows significant differences in SI in pre- and post-treatment sequences.
Experimental studies showed that DWI could be used to detect the efficacy of IRE treatment (71, 72).
The evaluation of the treatment response in terms of lesion dimensional reduction is not appropriate because not always a positive response to treatment is linked to a size reduction; furthermore dimensional criteria do not allow the differentiation of the fibrotic tissue from the residual tumor.
Therefore, an evaluation based only on dimensional data is not appropriate to assess the efficacy of such complex treatments. However, one of the major topics evaluated by papers that we analyzed regarding the ablative techniques is the short- and long- term efficacy based on the tumor dimension reduction. Regarding RFA papers, the results on the follow-up was reported in 12 out of 18 studies for a total of 214 patients (36–41, 43, 45–48). Assessment time was between 7 and 34 months and was mainly performed by means of CT-scan and MRI (seven studies), considering only dimensional criteria (36–41, 43, 45–48). According to Paiella et al. for RFA, and in general for “thermal techniques,” the gold standard of imaging is represented by CT with a post-ablative hypointense area observed as result of the treatment (9). However, also pancreatic tumor is hypointense so that a “qualitative assessment” based only human eyes could cause misdiagnosis. A quantitative evaluation based on perfusion evaluation or metabolic analysis allows a more objective reassessment and a more correct stratification of patients in responders and non-responders to treatment (73–77).
Regarding MWA studies, the follow-up was reported in all cases. Assessment time was between 1 and 12 months, performed by CT and MRI, considering dimensional criteria.
At the best of our knowledge no papers in literature reported findings on efficacy of ablation by RFA or MWA using functional radiological approaches such as DWI, DKI, PET. On the contrary in literature are present studies about the evaluation of efficacy by IRE and ECT using several functional radiological parameters in the assessment of the treatment.
Vroomen et al. (14) assessed specific imaging features after IRE for LAPC with contrast-enhanced (ce) MRI and ce-CT, and to explore the correlation of these features with the development of recurrence. They assessed pre and post IRE, for MRI, the Signal Intensity (SI) on T2-Weigthed sequences, on T1-Weigthed sequences (before and after ce, during arterial and venous phase), on DWI and on ADC map; and for CT attenuation in the arterial and portal venous phase. They found that the most remarkable signal alterations after IRE were shown by DWI-b800 and ceMRI. According to Vroomen et al., these features may be useful to establish technical success and predict treatment outcome. Granata et al. (12, 13) assessed morphological (Figures 3 and 4) and functional (Figure 5) diagnostic parameters to evaluate the efficacy of ECT (61, 78–81). The researchers showed that RECIST criteria were not able to discriminate partial, complete, or incomplete response after treatment, conversely using functional parameters, obtained with PET and MRI, it is possible.
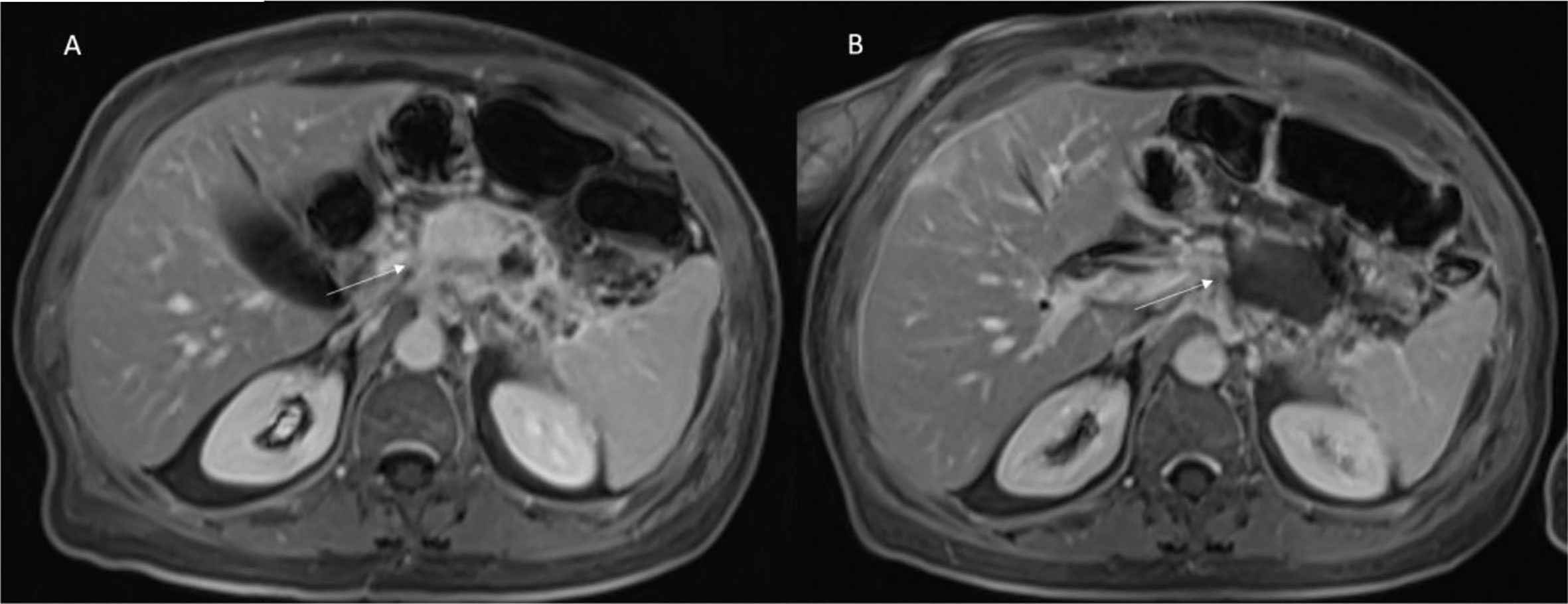
Figure 3 Patient 2 with Body-Tail Pancreatic Cancer. Morphological MRI assessment post-ECT treatment. In (A) (VIBE T1-W post-contrast sequence during portal phase in axial plane) pre-treatment evaluation of lesion (arrow). In (B) (VIBE T1-W post-contrast sequence during portal phase in axial plane) arrow shows ablated area. Qualitative assessment shows significant differences in SI in pre- and post-treatment sequences.
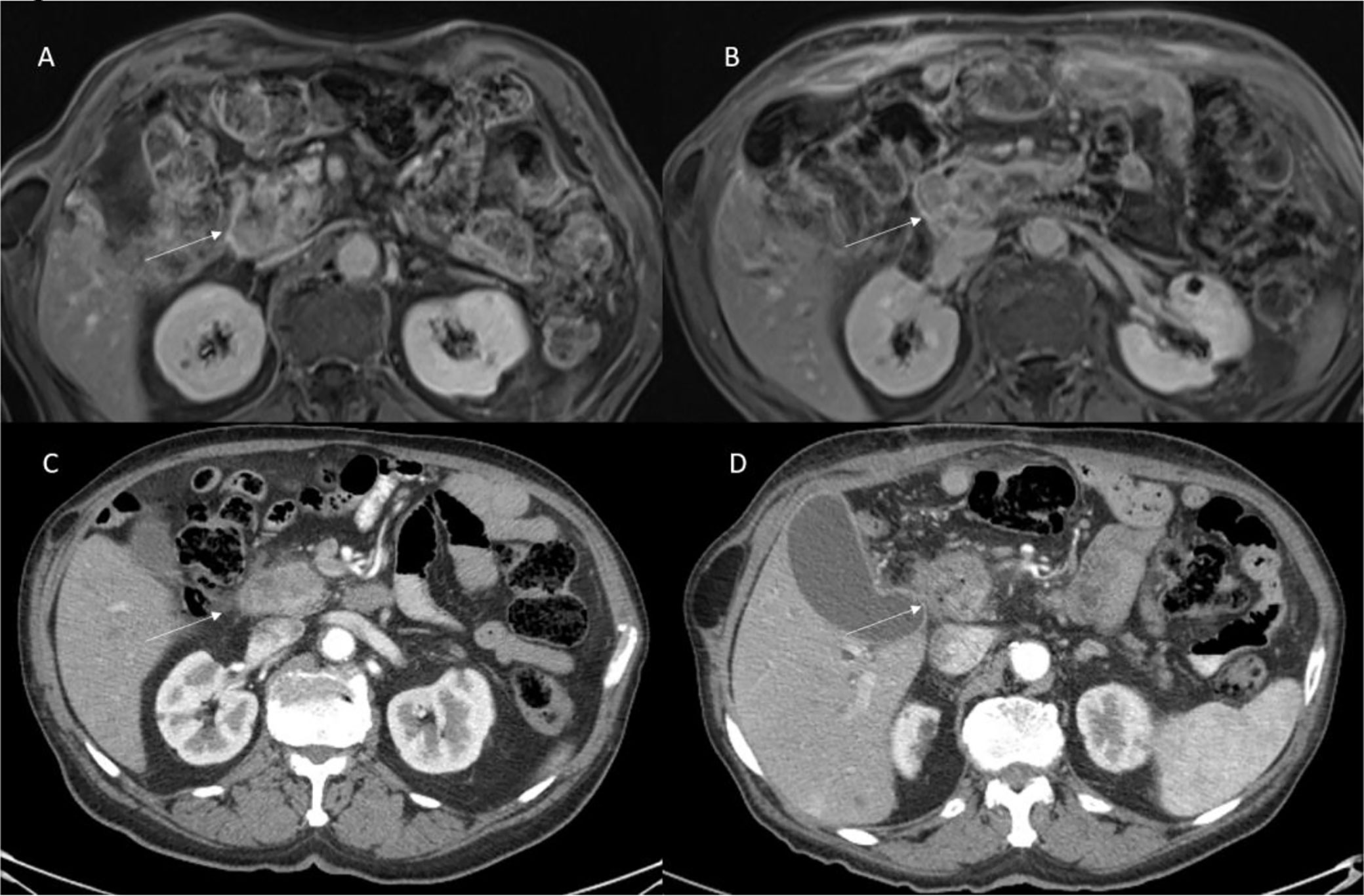
Figure 4 Patient 3 with head pancreatic cancer. Morphological MRI and CT assessment post-ECT treatment. In (A) (VIBE T1-W post-contrast sequence during portal phase in axial plane) and (C) (CT scan during pancreatic phase of contrast study) the arrow shows lesion. In (B) (VIBE T1-W post-contrast sequence during portal phase in axial plane) and (D) (CT scan during pancreatic phase of contrast study) the arrow shows ablated area. Qualitative assessment shows no significant differences in SI in pre- and post-treatment sequences and no significant differences in density in pre- and post-CT images.
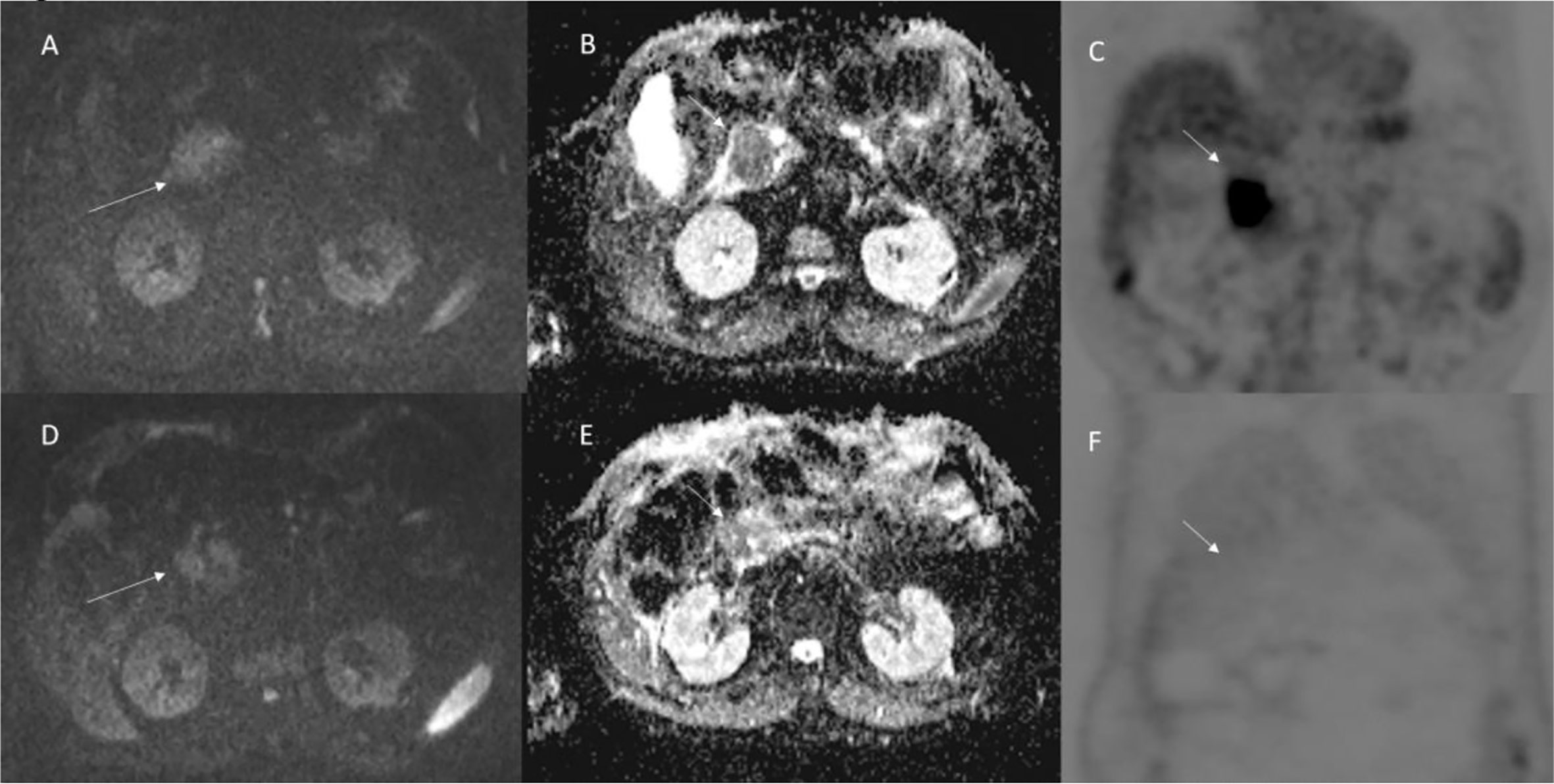
Figure 5 Patient 3 with head pancreatic cancer (the same of Figure 4). Functional (DW-MRI and PET) assessment post-ECT treatment. In (A) (pre treatment) and (D) (post treatment) (b 800 s/mm2) the lesion (arrow) shows restricted signal so as in ADC map (B: pre treatment; and E: post treatment) the lesion shows hypointense signal (arrow). This qualitative analysis is indicative of non-responder lesion. The PET (C: pre treatment; and F: post treatment) assessment shows (arrow) a responder lesion.
Conclusions
Although new chemotherapeutic schemes have been introduced, advanced pancreatic cancers still correlate with a poor long-term outcome. Local ablative therapies are used in some dedicated cancer centers in patients with LAPC. The assessment of a pancreatic cancer after neoadjuvant treatment is particularly complicated and the condition becomes more difficult when evaluating the effectiveness of ablative therapies, considering that RECIST criteria were not appropriate to assess the treatment. When considering therapy effects, imaging-derived parameters are sometimes complicated to understand, since they depend on anatomic location, on relations between specific tissue characteristics and the mechanism of action of therapy, and on the used techniques. In this setting, imaging features are correlated to the type and method of therapy delivery, the timing of treatment, and the imaging technique being used to observe the effects. A “qualitative assessment” based only human eyes should cause misdiagnosis. A quantitative evaluation based on perfusion evaluation or metabolic analysis allows a more objective reassessment and a more correct stratification of patients in responders and non-responders to treatment.
Data Availability Statement
The original contributions presented in the study are included in the article, further inquiries can be directed to the corresponding author.
Author Contributions
All authors listed have made a substantial, direct, and intellectual contribution to the work and approved it for publication.
Conflict of Interest
The authors declare that the research was conducted in the absence of any commercial or financial relationships that could be construed as a potential conflict of interest.
Acknowledgments
The authors are grateful to Alessandra Trocino, librarian at the National Cancer Institute of Naples, Italy. Moreover, the authors are grateful to Assunta Zazzaro for the collaboration.
Abbreviations
ADC, apparent diffusion coefficient; BLM, bleomycin; CT, computed tomography; DCE-MR, dynamic contrast-enhanced MRI; D, diffusion coefficient; Dp, pseudo-diffusion coefficient; DWI, diffusion weigthed imaging; DKI, diffusion kurtosis imaging; ECT, electrochemotherapy; EGT, electrogenetransfer; fp, perfusion fraction; IRE, irreversible electroporation; IVIM, intravoxel incoherent motion; K, kurtosis coefficient; OS, overall survival; LAPC, locally advanced pancreatic cancer; MD, mean diffusivity; MRI, magnetic resonance imaging; MWA, microwave ablation; PR, partial response; PD, progressive disease; PET, position emission tomography; QoL, quality of life; RFA, radiofrequency ablation; RECIST, Response Evaluation Criteria in Solid Tumors; SD, stable diseases.
References
1. Siegel RL, Miller KD, Jemal A. Cancer statistics, 2020. CA Cancer J Clin (2020) 70(1):7–30. doi: 10.3322/caac.21590
2. NCCN.org. NCCN Clinical Practice Guidelines in Oncology (NCCN Guidelines®) Pancreatic Adenocarcinoma Version 1.2020. (2020). Available at: https://www.nccn.org/professionals/physician_gls/default.aspx.
3. Tempero MA. NCCN Guidelines Updates: Pancreatic Cancer. J Natl Compr Canc Netw (2019) 17(5.5):603–5. doi: 10.6004/jnccn.2018.0043
4. Alistar A, Morris BB, Desnoyer R, Klepin HD, Hosseinzadeh K, Clark C, et al. Safety and tolerability of the first-in-class agent CPI-613 in combination with modified FOLFIRINOX in patients with metastatic pancreatic cancer: a single-centre, open-label, dose-escalation, phase 1 trial. Lancet Oncol (2017) 18(6):770–8. doi: 10.1016/S1470-2045(17)30314-5
5. Usón Junior PLS, Rother ET, Maluf FC, Bugano DDG. Meta-analysis of Modified FOLFIRINOX Regimens for Patients With Metastatic Pancreatic Cancer. Clin Colorectal Cancer (2018) 17(3):187–97. doi: 10.1016/j.clcc.2018.03.007
6. International Agency for Research on Cancer (IARC). http://www.iarc.fr/.
7. Chin V, Nagrial A, Sjoquist K, O’Connor CA, Chantrill L, Biankin AV, et al. Chemotherapy and radiotherapy for advanced pancreatic cancer. Cochrane Database Syst Rev (2018) 3(3):CD011044. doi: 10.1002/14651858.CD011044.pub2
8. van Veldhuisen E, van den Oord C, Brada LJ, Walma MS, Vogel JA, Wilmink JW, et al. Locally Advanced Pancreatic Cancer: Work-Up, Staging, and Local Intervention Strategies. Cancers (Basel) (2019) 11(7):E976. doi: 10.3390/cancers11070976
9. Paiella S, Salvia R, Ramera M, Girelli R, Frigerio I, Giardino A, et al. Local Ablative Strategies for Ductal Pancreatic Cancer (Radiofrequency Ablation, Irreversible Electroporation): A Review. Gastroenterol Res Pract (2016) 2016:4508376. doi: 10.1155/2016/4508376
10. Granata V, Fusco R, Catalano O, Setola SV, de Lutio di Castelguidone E, Piccirillo M, et al. Multidetector computer tomography in the pancreatic adenocarcinoma assessment: an update. Infect Agent Cancer (2016) 11:57. eCollection 2016. doi: 10.1186/s13027-016-0105-6
11. Granata V, Fusco R, Piccirillo M, Palaia R, Petrillo A, Lastoria S, et al. Electrochemotherapy in locally advanced pancreatic cancer: Preliminary results. Int J Surg (2015) 18:230–6. doi: 10.1016/j.ijsu.2015.04.055
12. Granata V, Fusco R, Setola SV, Piccirillo M, Leongito M, Palaia R, et al. Early radiological assessment of locally advanced pancreatic cancer treated with electrochemotherapy. World J Gastroenterol (2017) 23(26):4767–78. doi: 10.3748/wjg.v23.i26.4767
13. Granata V, Fusco R, Setola SV, Palaia R, Albino V, Piccirillo M, et al. Diffusion kurtosis imaging and conventional diffusion weighted imaging to assess electrochemotherapy response in locally advanced pancreatic cancer. Radiol Oncol (2019) 53(1):15–24. doi: 10.2478/raon-2019-0004
14. Vroomen LGPH, Scheffer HJ, Melenhorst MCAM, de Jong MC, van den Bergh JE, van Kuijk C, et al. MR and CT imaging characteristics and ablation zone volumetry of locally advanced pancreatic cancer treated with irreversible electroporation. Eur Radiol (2017) 27(6):2521–31. doi: 10.1007/s00330-016-4581-2
15. García-Figueiras R, Padhani AR, Baleato-González S. Therapy Monitoring with Functional and Molecular MR Imaging. Magn Reson Imaging Clin N Am (2016) 24:261–88. doi: 10.1016/j.mric.2015.08.003
16. Izzo F, Granata V, Grassi R, Fusco R, Palaia R, Delrio P, et al. Radiofrequency Ablation and Microwave Ablation in Liver Tumors: An Update. Oncologist (2019) 24(10):e990–1005. doi: 10.1634/theoncologist.2018-0337
17. Pillai K, Akhter J, Chua TC, Shehata M, Alzahrani N, Al-Alem I, et al. Heat sink effect on tumor ablation characteristics as observed in monopolar radiofrequency, bipolar radiofrequency, and microwave, using ex vivo calf liver model. Med (Baltimore) (2015) 94(9):e580. doi: 10.1097/MD.0000000000000580
18. Granata V, de Lutio di Castelguidone E, Fusco R, Catalano O, Piccirillo M, Palaia R, et al. Irreversible electroporation of hepatocellular carcinoma: preliminary report on the diagnostic accuracy of magnetic resonance, computer tomography, and contrast-enhanced ultrasound in evaluation of the ablated area. Radiol Med (2016) 121(2):122–31. doi: 10.1007/s11547-015-0582-5
19. Granata V, Fusco R, Catalano O, Piccirillo M, De Bellis M, Izzo F, et al. Percutaneous ablation therapy of hepatocellular carcinoma with irreversible electroporation: MRI findings. AJR Am J Roentgenol (2015) 204(5):1000–7. doi: 10.2214/AJR.14.12509
20. Martin RC2, Kwon D, Chalikonda S, Sellers M, Kotz E, Scoggins C, et al. Treatment of 200 locally advanced (stage III) pancreatic adenocarcinoma patients with irreversible electroporation: safety and efficacy. Ann Surg (2015) 262(3):486–94. discussion 492-4. doi: 10.1097/SLA.0000000000001441
21. Martin RC2, McFarland K, Ellis S. Velanovich V. Irreversible electroporation in locally advanced pancreatic cancer: potential improved overall survival. Ann Surg Oncol (2013) 20:S443–9. doi: 10.1245/s10434-012-2736-1
22. Kwon D, McFarland K, Velanovich V, Martin RC2. Borderline and locally advanced pancreatic adenocarcinoma margin accentuation with intraoperative irreversible electroporation. Surgery (2014) 156(4):910–20. doi: 10.1016/j.surg.2014.06.058
23. Ansari D, Kristoffersson S, Andersson R, Bergenfeldt M. The role of irreversible electroporation (IRE) for locally advanced pancreatic cancer: a systematic review of safety and efficacy. Scand J Gastroenterol (2017) 52(11):1165–71. doi: 10.1080/00365521.2017.1346705
24. Lambert L, Horejs J, Krska Z, Hoskovec D, Petruzelka L, Krechler T, et al. Treatment of locally advanced pancreatic cancer by percutaneous and intraoperative irreversible electroporation: general hospital cancer center experience. Neoplasma (2016) 63(2):269–73. doi: 10.4149/213_150611N326
25. Yan L, Chen YL, Su M, Liu T, Xu K, Liang F, et al. Single-institution Experience with Open Irreversible Electroporation for Locally Advanced Pancreatic Carcinoma. Chin Med J (Engl) (2016) 129(24):2920–5. doi: 10.4103/0366-6999.195476
26. Zhang Y, Shi J, Zeng J, Alnagger M, Zhou L, Fang G, et al. Percutaneous Irreversible Electroporation for Ablation of Locally Advanced Pancreatic Cancer: Experience From a Chinese Institution. Pancreas (2017) 46(2):e12–4. doi: 10.1097/MPA.0000000000000703
27. Scheffer HJ, Vroomen LG, de Jong MC, Melenhorst MC, Zonderhuis BM, Daams F, et al. Ablation of Locally Advanced Pancreatic Cancer with Percutaneous Irreversible Electroporation: Results of the Phase I/II PANFIRE Study. Radiology (2017) 282(2):585–97. doi: 10.1148/radiol.2016152835
28. Izzo F, Ionna F, Granata V, Albino V, Patrone R, Longo F, et al. New Deployable Expandable Electrodes in the Electroporation Treatment in a Pig Model: A Feasibility and Usability Preliminary Study. Cancers (Basel) (2020) 12(2):E515. doi: 10.3390/cancers12020515
29. Tafuto S, von Arx C, De Divitiis C, Maura CT, Palaia R, Albino V, et al. Electrochemotherapy as a new approach on pancreatic cancer and on liver metastases. Int J Surg (2015) 21:S78–82. doi: 10.1016/j.ijsu.2015.04.095
30. Miklavčič D, Mali B, Kos B, Heller R, Serša G. Electrochemotherapy: from the drawing board into medical practice. Biomed Eng Online (2014) 13:29. doi: 10.1186/1475-925X-13-29
31. Mir LM, Tounekti O, Orlowski S. Bleomycin: revival of an old drug. Gen Pharmacol (1996) 27(5):745–8. doi: 10.1016/0306-3623(95)02101-9
32. Gehl J, Geertsen PF. Efficient palliation of haemorrhaging malignant melanoma skin metastases by electrochemotherapy. Melanoma Res (2000) 10(6):585–9. doi: 10.1097/00008390-200012000-00011
33. Bastianpillai C, Petrides N, Shah T, Guillaumier S, Ahmed HU, Arya M. Harnessing the immunomodulatory effect of thermal and non-thermal ablative therapies for cancer treatment. Tumour Biol (2015) 36(12):9137–46. doi: 10.1007/s13277-015-4126-3
34. Neal RE2, Rossmeisl JH Jr, Robertson JL, Arena CB, Davis EM, Singh RN, et al. Improved local and systemic anti-tumor efficacy for irreversible electroporation in immunocompetent versus immunodeficient mice. PloS One (2013) 8(5):e64559. doi: 10.1371/journal.pone.0064559
35. Longo F, Perri F, Caponigro F, Della Vittoria Scarpati G, Guida A, Pavone E, et al. Boosting the Immune Response with the Combination of Electrochemotherapy and Immunotherapy: A New Weapon for Squamous Cell Carcinoma of the Head and Neck? Cancers (Basel) (2020) 12(10):E2781. doi: 10.3390/cancers12102781
36. D’Onofrio M, Barbi E, Girelli R, Tinazzi Martini P, De Robertis R, et al. Variation of tumoral marker after radiofrequency ablation of pancreatic adenocarcinoma. J Gastrointest Oncol (2016) 7(2):213–20. doi: 10.3978/j.issn.2078-6891.2015.085
37. Giardino A, Girelli R, Frigerio I, Regi P, Cantore M, Alessandra A, et al. Triple approach strategy for patients with locally advanced pancreatic carcinoma. HPB (Oxford) (2013) 15(8):623–7. doi: 10.1111/hpb.12027
38. Hadjicostas P, Malakounides N, Varianos C, Kitiris E, Lerni F, Symeonides P. Radiofrequency ablation in pancreatic cancer. HPB (Oxford) (2006) 8(1):61–4. doi: 10.1080/13651820500466673
39. Ikuta S, Kurimoto A, Iida H, Aihara T, Takechi M, Kamikonya N, et al. Optimal combination of radiofrequency ablation with chemoradiotherapy for locally advanced pancreatic cancer. World J Clin Oncol (2012) 3(1):12–4. doi: 10.5306/wjco.v3.i1.12
40. Kallis Y, Phillips N, Steel A, Kaltsidis H, Vlavianos P, Habib N, et al. Analysis of Endoscopic Radiofrequency Ablation of Biliary Malignant Strictures in Pancreatic Cancer Suggests Potential Survival Benefit. Dig Dis Sci (2015) 60(11):3449–55. doi: 10.1007/s10620-015-3731-8
41. Lakhtakia S, Ramchandani M, Galasso D, Gupta R, Venugopal S, Kalpala R, et al. EUS-guided radiofrequency ablation for management of pancreatic insulinoma by using a novel needle electrode (with videos). Gastrointest Endosc (2016) 83:234–9. doi: 10.1016/j.gie.2015.08.085
42. Pai M, Habib N, Senturk H, Lakhtakia S, Reddy N, Cicinnati VR, et al. Endoscopic ultrasound guided radiofrequency ablation, for pancreatic cystic neoplasms and neuroendocrine tumors. World J Gastrointest Surg (2015) 7(4):52–9. doi: 10.4240/wjgs.v7.i4.52
43. Rossi S, Viera FT, Ghittoni G, Cobianchi L, Rosa LL, Siciliani L, et al. Radiofrequency ablation of pancreatic neuroendocrine tumors: a pilot study of feasibility, efficacy, and safety. Pancreas (2014) 43(6):938–45. doi: 10.1097/MPA.0000000000000133
44. Song TJ, Seo DW, Lakhtakia S, Reddy N, Oh DW, Park DH, et al. Initial experience of EUS- guided radiofrequency ablation of unresectable pancreatic can- cer. Gastrointest Endosc (2016) 83(2):440–3. doi: 10.1016/j.gie.2015.08.048
45. Spiliotis JD, Datsis AC, Michalopoulos NV, Kekelos SP, Vaxevanidou A, Rogdakis AG, et al. Radiofrequency ablation combined with palliative surgery may prolong survival of patients with advanced cancer of the pancreas. Langenbecks Arch Surg (2007) 392(1):55–60. doi: 10.1007/s00423-006-0098-5
46. Varshney S, Sewkani A, Sharma S. Radiofrequency ablation ofunresectable pancreatic carcinoma: feasibility, efficacy and safety. JOP (2006) 7:74–8. doi: 10.4251/wjgo.v7.i2.6
47. Waung JA, Todd JF, Keane MG, Pereira SP. Successful management of a sporadic pancreatic insulinoma by endoscopic ultrasound- guided radiofrequency ablation. Endoscopy (2016) 48 Suppl 1:E144–5. doi: 10.1055/s-0042-104650
48. Zou YP, Li WM, Zheng F, Li FC, Huang H, Du JD, et al. Intraoperative radiofrequency ablation combined with 125 iodine seed implantation for unresectable pancreatic cancer. World J Gastroenterol (2010) 16 (40):5104–10. doi: 10.3748/wjg.v16.i40.5104
49. Saccomandi P, Lapergola A, Longo F, Schena E, Quero G. Thermal ablation of pancreatic cancer: A systematic literature review of clinical practice and pre-clinical studies. Int J Hyperthermia (2018) 35(1):398–418. doi: 10.1080/02656736.2018.1506165
50. Waitz R, Solomon SB. Can local radiofrequency ablation of tumors generate systemic immunity against metastatic disease? Radiology (2009) 251:1–2. doi: 10.1148/radiol.2511082215
51. Ierardi AM, Biondetti P, Coppola A, Fumarola EM, Biasina AM, Alessio Angileri S, et al. Percutaneous microwave thermosphere ablation of pancreatic tumours. Gland Surg (2018) 7(2):59–66. doi: 10.21037/gs.2017.11.05
52. Carrafiello G, Ierardi AM, Fontana F, Petrillo M, Floridi C, Lucchina N, et al. Microwave ablation of pancreatic head cancer: safety and efficacy. J Vasc Interv Radiol (2013) 24 (10):1513–20. doi: 10.1016/j.jvir.2013.07.005
53. Vogl TJ, Panahi B, Albrecht MH, Naguib NNN, Nour-Eldin NA, Gruber-Rouh T, et al. Microwave ablation of pancreatic tumors. Minim Invasive Ther Allied Technol (2018) 27(1):33–40. doi: 10.1080/13645706.2017.1420664
54. Narayanan G, Hosein PJ, Arora G, Barbery KJ, Froud T, Livingstone AS, et al. Percutaneous irreversible electroporation for downstaging and control of unresectable pancreatic adenocarcinoma. J Vasc Interventional Radiol (2012) 23 (12):1613–21. doi: 10.1016/j.jvir.2012.09.012
55. Weiss MJ, Wolfgang CL. Irreversible electroporation: a novel pancreatic cancer therapy. Curr Problems Cancer (2013) 37:262–5. doi: 10.1016/j.currproblcancer.2013.10.002
56. Rombouts SJE, Vogel JA, van Santvoort HC, van Lienden KP, van Hillegersberg R, Busch OR, et al. Systematic review of innovative ablative therapies for the treatment of locally advanced pancreatic cancer. Br J Surg (2015) 102(3):182–93. doi: 10.1002/bjs.9716
57. Scheffer HJ, Nielsen K, de Jong MC, van Tilborg AA, Vieveen JM, Bouwman AR, et al. Irreversible electroporation for nonthermal tumor ablation in the clinical setting: a systematic review of safety and efficacy. J Vasc Interventional Radiol (2014) 25(7):997–1011. doi: 10.1016/j.jvir.2014.01.028
58. Granata V, Fusco R, Palaia R, Belli A, Petrillo A, Izzo F. Comments on Electrochemotherapy with Irreversible Electroporation and FOLFIRINOX Improves Survival in Murine Models of Pancreatic Adenocarcinoma. Ann Surg Oncol (2020). doi: 10.1245/s10434-020-09183-66
59. Poulou LS, Botsa E, Thanou I, Ziakas PD, Thanos L. Percutaneous microwave ablation vs radiofrequency ablation in the treatment of hepatocellular carcinoma. World J Hepatol (2015) 7(8):1054–63. doi: 10.4254/wjh.v7.i8.1054
60. Giardino A, Innamorati G, Ugel S, Perbellini O, Girelli R, Frigerio I, et al. Immunomodulation after radiofrequency ablation of locally advanced pancreatic cancer by monitoring the immune response in 10 patients. Pancreatology (2017) 17(6):962–6. doi: 10.1016/j.pan.2017.09.008
61. Kotnik T, Rems L, Tarek M, Miklavčič D. Membrane Electroporation and Electropermeabilization: Mechanisms and Models. Annu Rev Biophys (2019) 48:63–91. doi: 10.1146/annurev-biophys-052118-115451
62. Dežman R, Čemažar M, Serša G, Seliškar A, Erjavec V, Trotovšek B, et al. Safety and Feasibility of Electrochemotherapy of the Pancreas in a Porcine Model. Pancreas (2020) 49(9):1168–73. doi: 10.1097/MPA.0000000000001642
63. Girelli R, Prejanò S, Cataldo I, Corbo V, Martini L, Scarpa A, et al. Feasibility and safety of electrochemotherapy (ECT) in the pancreas: a pre-clinical investigation. Radiol Oncol (2015) 49(2):147–54. doi: 10.1515/raon-2015-0013
64. Fiorentzis M, Viestenz A, Siebolts U, Seitz B, Coupland SE, Heinzelmann J. The Potential Use of Electrochemotherapy in the Treatment of Uveal Melanoma: In Vitro Results in 3D Tumor Cultures and In Vivo Results in a Chick Embryo Model. Cancers (Basel) (2019) 11(9):1344. doi: 10.3390/cancers11091344
65. Fernandes P, O’Donovan TR, McKenna SL, Forde PF. Electrochemotherapy Causes Caspase-Independent Necrotic-Like Death in Pancreatic Cancer Cells. Cancers (Basel) (2019) 11(8):1177. doi: 10.3390/cancers11081177
66. Lencioni R, Llovet JM. Modified RECIST (mRECIST) assessment for hepatocellular carcinoma. Semin Liver Dis (2010) 30:52–60. doi: 10.1055/s-0030-1247132
67. Kudo M, Ueshima K, Kubo S, Sakamoto M, Tanaka M, Ikai I, et al. Response Evaluation Criteria in Cancer of the Liver (RECICL) (2015 Revised version). Hepatol Res (2016) 46(1):3–9. doi: 10.1111/hepr.12542
68. Cassinotto C, Sa-Cunha A, Trillaud H. Radiological evaluation of response to neoadjuvant treatment in pancreatic cancer. Diagn Interv Imaging (2016) 97(12):1225–32. doi: 10.1016/j.diii.2016.07.011
69. Granata V, Fusco R, Sansone M, Grassi R, Maio F, Palaia R, et al. Magnetic Resonance Imaging in the assessment of pancreatic cancer with quantitative parameters extraction by means of Dynamic Contrast Enhanced Magnetic Resonance Imaging, Diffusion Kurtosis Imaging and Intravoxel Incoherent. Ther Adv Gastroenterol (2020) 13:1756284819885052. doi: 10.1177/1756284819885052
70. Fusco R, Granata V, Petrillo A. Introduction to Special Issue of Radiology and Imaging of Cancer. Cancers (Basel) (2020) 12(9):E2665. doi: 10.3390/cancers12092665
71. Mahmood F, Hansen RH, Agerholm-Larsen B, Gissel H, Ibsen P, Gehl J. Detection of electroporation-induced membrane permeabilization states in the brain using diffusion-weighted MRI. Acta Oncol (2015) 54(3):289–97. doi: 10.3109/0284186X.2014.991045
72. Zhang Z, Li W, Procissi D, Tyler P, Omary RA, Larson AC, et al. Rapid dramatic alterations to the tumor microstructure in pancreatic cancer following irreversible electroporation ablation. Nanomed (Lond) (2014) 9(8):1181–9. doi: 10.2217/nnm.13.72
73. Choi H. Response evaluation of gastrointestinal stromal tumors. Oncologist (2008) 13:4–7. doi: 10.1634/theoncologist.13-S2-4
74. Tirkes T, Hollar MA, Tann M, Kohli MD, Akisik F, Sandrasegaran K. Response criteria in oncologic imaging: review of traditional and new criteria. Radiographics (2013) 33:1323–41. doi: 10.1148/rg.335125214
75. van der Veldt AA, Meijerink MR, van den Eertwegh AJ, Haanen JB, Boven E. Choi response criteria for early prediction of clinical outcome in patients with metastatic renal cell cancer treated with sunitinib. Br J Cancer (2010) 102:803–9. doi: 10.1038/sj.bjc.6605567
76. Stacchiotti S, Collini P, Messina A, Morosi C, Barisella M, Bertulli R, et al. High-grade soft-tissue sarcomas: tumor response assessment–pilot study to assess the correlation between radiologic and pathologic response by using RECIST and Choi criteria. Radiology (2009) 251:447–56. doi: 10.1148/radiol.2512081403
77. Ding Q, Cheng X, Yang L, Zhang Q, Chen J, Li T, et al. PET/CT evaluation of response to chemotherapy in non-small cell lung cancer: PET response criteria in solid tumors (PERCIST) versus response evaluation criteria in solid tumors (RECIST). J Thorac Dis (2014) 6:677–83. doi: 10.3978/j.issn.2072-1439.2014.05.10
78. Cadossi R, Ronchetti M, Cadossi M. Locally enhanced chemotherapy by electroporation: clinical experiences and perspective of use of electrochemotherapy. Future Oncol (2014) 10(5):877–90. doi: 10.2217/fon.13.235
79. Aycock KN, Davalos RV. Irreversible Electroporation: Background, Theory, and Review of Recent Developments in Clinical Oncology. Bioelectricity (2019) 1:214–34. doi: 10.1089/bioe.2019.0029
80. Geboers B, Scheffer HJ, Graybill PM, Ruarus AH, Nieuwenhuizen S, Puijk RS, et al. High-Voltage Electrical pulses in oncology: Irreversible electroporation, electrochemotherapy, gene electrotransfer, electrofusion, and electroimmunotherapy. Radiology (2020) 295:254–72. doi: 10.1148/radiol.2020192190
Keywords: pancreatic cancer, ablation treatment, computed tomography, magnetic resonance imaging, functional imaging
Citation: Granata V, Grassi R, Fusco R, Setola SV, Palaia R, Belli A, Miele V, Brunese L, Grassi R, Petrillo A and Izzo F (2020) Assessment of Ablation Therapy in Pancreatic Cancer: The Radiologist’s Challenge. Front. Oncol. 10:560952. doi: 10.3389/fonc.2020.560952
Received: 11 May 2020; Accepted: 30 October 2020;
Published: 27 November 2020.
Edited by:
Mattia Squarcia, Hospital Clínic de Barcelona, SpainReviewed by:
Katsutoshi Sugimoto, Tokyo Medical University, JapanHiroyasu Fujiwara, Okayama City Hospital, Japan
Copyright © 2020 Granata, Grassi, Fusco, Setola, Palaia, Belli, Miele, Brunese, Grassi, Petrillo and Izzo. This is an open-access article distributed under the terms of the Creative Commons Attribution License (CC BY). The use, distribution or reproduction in other forums is permitted, provided the original author(s) and the copyright owner(s) are credited and that the original publication in this journal is cited, in accordance with accepted academic practice. No use, distribution or reproduction is permitted which does not comply with these terms.
*Correspondence: Roberta Fusco, ci5mdXNjb0Bpc3RpdHV0b3R1bW9yaS5uYS5pdA==