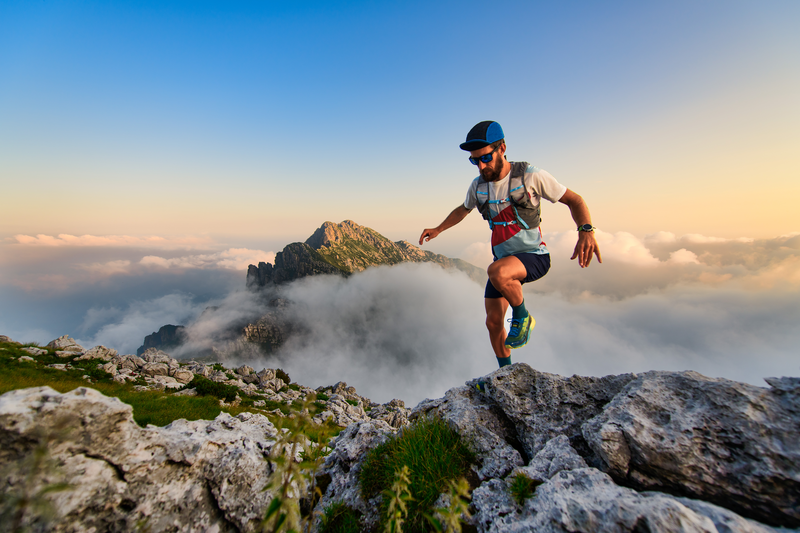
94% of researchers rate our articles as excellent or good
Learn more about the work of our research integrity team to safeguard the quality of each article we publish.
Find out more
REVIEW article
Front. Ocean Sustain. , 29 November 2024
Sec. Blue Food Provisions
Volume 2 - 2024 | https://doi.org/10.3389/focsu.2024.1504689
The development and management of our future aquatic food systems play a pivotal role in achieving the UN sustainable development goals (SDGs). While expanding aquatic food systems align with the ambitions of national 'blue economy' strategies, understanding challenges and opportunities is essential for successful expansion. Three globally relevant case studies—seaweed farming and harvesting, bivalve farming and harvesting, and tuna fisheries—were identified. A literature review revealed challenges and opportunities linked to UN SDGs related to poverty (1), hunger (2), health (3), gender equality (5), responsible consumption (12), climate action (13), and life below water (14). Although no single solution addresses all challenges, the cases emphasize that adapted spatial and ecosystem-based management offers pathways to address major challenges and capitalize on key opportunities.
Advancements to meet the Agenda of Sustainable Development by 2030 remain off-target. Bold actions are necessary to accelerate progress (UN, 2022) as extreme poverty and hunger are increasing in many regions (Lakner et al., 2022; FAO et al., 2022). This inflicts continuous pressure on finite resources (Searchinger et al., 2019), requiring smart establishment and maintenance of food systems. Aquatic food systems can contribute a unique nutritional benefit, complementary to other food systems (Golden et al., 2021).
The connections between food production by calories or mass, food security, and nutrition are not linear (Haddad et al., 2016), and our current food systems are facing multiple stressors (e.g., pollution, climate change, habitat degradation), highlighting a need for transformation (Costello et al., 2020; Blue Food Assessment, 2021). To establish equitable and sustainable food systems, a holistic approach, using a “food systems” lens, is instrumental to nourish the world by encompassing all actors and interactions along the food supply chain (HLPE, 2020). Important drivers that are considered through the food system lens include biophysical and environmental factors, technology, innovation, infrastructure, the economy and market, political and institutional, as well as socio-cultural and demographic conditions (Figure 1) (FAO, 2018; HLPE, 2020). This underscores that the success of our food systems should not only be measured in economic or caloric yield, but also by value generated across multiple dimensions of food security: availability, accessibility, utilization, stability, agency, and sustainability (FAO, 2018).
Figure 1. Future aquatic food systems, highlighting the pathway from production to consumption where at all steps targeted management and strategic decisions will allow the system to most successfully and equitably contribute to the seven SDGs shown at the bottom related to poverty (1), hunger (2), health (3), gender equality (5), responsible consumption (12), climate action (13), and life below water (14).
The UN Decades on Nutrition and Ocean Science offers a chance to align efforts on the potential of aquatic food systems for providing sufficient and affordable essential micronutrients (UN, 2015, 2021; FAO, 2022a). Today, harvested aquatic food resources are focused on relatively few species. Diversification of such food resources, including finfish, crustaceans, mollusks, aquatic plants including algae, and mammals can increase food security (Hicks et al., 2019; Golden et al., 2021). Aquatic foods also pose potential food safety hazards, including contaminants, viral and bacterial pathogens, as well as biotoxins and parasites (van der Oost et al., 2003; Fung et al., 2018; Novoslavskij et al., 2016). However, the intrinsic interrelation of food safety and nutrient provision remains under-studied and under-communicated in policy and research (Nordhagen et al., 2022). Food safety hazards may additionally arise from improper food handling, processing, transportation, and storage (Fung et al., 2018), further exacerbating potential food safety risks. Therefore, food risk and benefit assessments need to be extended in the process of diversification.
Aquatic food systems have a lower environmental impact compared to terrestrial food systems (Gephart et al., 2021; Koehn et al., 2022a) but are also affected by climate change which disproportionately impacts vulnerable populations (Maire et al., 2021; IPCC, 2022). Adaptive strategies incorporating both environmental and socioeconomic factors are therefore needed to strengthen resilience in aquatic food systems (FAO, 2021; Tigchelaar et al., 2021). Additional improvements can be expected from implementing increased nutrient circularity, reducing nutrient losses and increasing upcycling of food residue streams (Harder et al., 2021). This approach can benefit both aquatic and terrestrial food systems by highlighting synergies and reducing siloed and sector-specific practices. Furthermore, a dietary shift from reducing consumption of red meat to increasing consumption of aquatic foods would lower carbon emissions and the risk of diet-related non-communicable diseases (Golden et al., 2021).
Decline in food quantity or quality throughout the supply chain poses a major challenge to food security in terms of post-harvest loss. It is estimated that 14% of globally produced food is lost during this stage, but the true extent remains uncertain (FAO, 2019). Aquatic foods are particularly susceptible to spoilage, and up to 40% of fish and fisheries products are lost due to poor management, natural disasters, and lack of market access (Prodhan et al., 2022). Reducing post-harvest loss across the value chain would improve food and nutrition security and environmental sustainability, effectively contributing to achieving the SDGs (FAO, 2019; Cattaneo et al., 2021).
Despite its potential, aquatic food systems are underrepresented in food security and nutrition policy (Fisher et al., 2017; Tlusty et al., 2019; Bennett et al., 2021; Farmery et al., 2021b). Maximizing biomass yield and revenue remains the main policy focus (Bennett et al., 2021; Robinson et al., 2022), with only 77 out of 158 national fisheries policies identifying nutrition as a key objective (Koehn et al., 2022b). Criticism has arisen due to the misalignment between fisheries policy and small-scale actors (Short et al., 2021), necessitating policy adjustments to empower local control over food systems for sustenance and food sovereignty (Wittman, 2011). Although the need for food systems transformation is increasing globally, sustainable food systems including aquatic foods and key elements of food security outcomes (i.e., sufficient, safe and nutritious food that meets dietary needs and food preferences), are often discussed separately from food system yield (El Bilali et al., 2019).
This paper aims to delineate challenges and opportunities in sustainable aquatic food systems, illustrated through three case studies which we encourage to use as a starting point for management of such food systems. The case studies, developed during an international scientific workshop aboard the Norwegian tall ship “Statsraad Lehmkuhl” as part of the One Ocean Expedition in 2021, a UN Decade for Ocean Science activity (Huse et al., 2023; UN, 2021), are intended to serve as a foundation for crafting paths to achieve sustainability in all aquatic food systems. These studies, centered on seaweed aquaculture and harvest, bivalve aquaculture and harvest, and tuna fisheries, exemplify the concept of aquatic food systems (Figure 2).
Figure 2. Overview of the three case studies: Seaweed food systems, bivalve food systems and tuna food systems. Text adapted from FAO (2022b). Mt, million tons. Photos by Institute of Marine Research.
We conducted a scoping review on sustainable management of the three case studies (Figure 2), focusing on four key concepts: safe and nutritious food, policy and social equity, environment and climate change, and circularity (Figure 3). The literature is presented in a narrative form, with additional details on our approach provided in the Supplementary material.
Figure 3. Food system drivers and outcomes, and the key concepts highlighted in this paper. Adapted from HLPE (2020).
Following the PRISMA ScR screening process, 82 articles from across the world were included in the narrative synthesis (see Supplementary material for the PRISMA ScR flow diagram). Of these, 23 pertained to the seaweed case study, 41 to bivalves, and 29 to tuna. The selected articles spanned from 2014 marking the publication of the HLPE food systems report, to 2022, with most (58%) published in or after 2020.
The nutritional value of seaweed (macroalgae) has been recognized in several Asian and South American countries for centuries, and a growing global appreciation for utilizing seaweed as food has emerged since the turn of the millennium (Cavallo et al., 2021; Naylor et al., 2021). Seaweed are low-fat food sources containing heterogeneous levels of nutrients (Leandro et al., 2020; Slegers et al., 2021). Although the lipid levels in seaweed are generally low, unlike some terrestrial plants, they contain the highly unsaturated omega-3 fatty acids eicosapentaenoic acid (EPA) and docosahexaenoic acid (DHA) (Cavallo et al., 2021).
An evaluation of under-exploited edible seaweed (Ganesan et al., 2019) found substantial intra- and interspecies variation in dried samples of key nutrients including iron (14.8–72 mg/100 g) and zinc (1.5–5.2 μg/100 g). Origin, geographic position, seasonality, species, and processing need to be considered when investigating bioavailability, metabolic processes, and optimal use of seaweed products (Cavallo et al., 2021; Naylor et al., 2021). Due to the high levels of iodine in seaweed (Leandro et al., 2020) a diet including seaweed may lead to excessive iodine exposure. Therefore, to enhance awareness and ensure consumer safety, iodine concentrations in seaweed should be disclosed. Additionally, nutrient and contaminant levels in some seaweeds require further study to evaluate their potential as healthy and sustainable foods (Grebe et al., 2019).
With their role as primary producers in the aquatic ecosystem, some seaweeds have a high susceptibility to accumulate heavy metals from their surroundings (Leandro et al., 2020). High concentrations of inorganic arsenic, up to 117 mg/kg, have been determined in Hijiki (Sargassum fusiforme), which by far exceed the maximum limit of 3 mg/kg set by international food authorities (Leandro et al., 2020).
Microplastics are another emerging concern, as they can sorb to seaweeds such as bladder wrack (Fucus vesiculosus), commonly utilized for health supplements (Walkinshaw et al., 2020). Intensive cultivation of seaweed also makes it prone to disease outbreaks (Grebe et al., 2019). Current reports estimate that disease management of bacterial and viral outbreaks alone account for up to 50% of farming costs in high-intensive farms (Naylor et al., 2021). Potentially connecting the two hazards, marine plastic may also carry pathogenic bacteria (Walkinshaw et al., 2020).
Seaweed have diverse uses, including fresh consumption, cooked, or dried, as snacks and as an additive in various products like beverages, dairy items, pasta, vegetarian burgers, and salt (Cavallo et al., 2021; Slegers et al., 2021). Consumer acceptance is a major challenge for introducing seaweed products into new markets. Unfamiliar taste and appearance are key reasons for seaweed avoidance in the Global North (Slegers et al., 2021), while the perception of seaweed as healthy and sustainable drives their adoption (van den Burg et al., 2021). In Pacific Island nations like Samoa and Kiribati, sea grapes (Caulerpa lentillifera) are integral to local food culture, exemplifying a sustainable solution to health challenges and enhancing dietary diversity while benefiting communities (Butcher et al., 2020).
There is inadequate policy and poor management of nascent seaweed farms, frequently attributed to a lack of connection of research efforts between the Global North and South (Krumhansl et al., 2017). In a study from Latin America only three out of twenty countries mention seaweed in their fisheries policy (Alemañ et al., 2019). Chile has pioneered seaweed policy and developed regulations to encourage diversification of the sector through large- and small-scale farming (Henriquez-Antipa and Carcamo, 2019), including multi-criteria decision analyses to assess perspectives of stakeholders and identifying current policy implementation gaps (Henriquez-Antipa and Carcamo, 2019). Furthermore, the compliance between the ecosystem approach to aquaculture developed by FAO and seaweed cultivation represents an opportunity to promote both social and ecological sustainability (Grebe et al., 2019).
While up to 85% of global seaweed production is used for human consumption in Asia (van den Burg et al., 2021), diverging utilization of seaweed in the Global North and South is reflected in market prices. Kelp produced in the US and Europe is retailed for over five times the price (US$ 944 t−1 wet weight) compared to kelp produced in Korea (US$ 177 t−1) (Grebe et al., 2019). A question arises as to how expansion of seaweed production in Western countries could fit in and compete in existing global markets. The high volume and low prices of commercialized seaweed in the Global South further entails its perception as a lower value source of income for small-scale producers and harvesters (Henriquez-Antipa and Carcamo, 2019). Activities such as small-scale kelp farming require little startup capital, but the missing standardization of cultivation techniques required to establish profitable businesses limit its expansion (Grebe et al., 2019; Farmery et al., 2020). Despite that most small-scale seaweed farmers in the Global South are women, their significant contributions to the fisheries remain undervalued, and their voices are not resonated in fisheries policy (Mirera et al., 2020; Thomas A. et al., 2021). The role of seaweed farming for achieving the SDGs and for stimulating the blue economic growth in Bangladesh has been assessed (Thomas J. B. E. et al., 2021), presenting key linkages between small-scale seaweed farming and 26 targets of 8 the SDGs. This included increased resource access for marginalized population groups and stimulation of the local economy, underpinned by the untapped opportunity of seaweed aquaculture expansion in tropical climates (Hossain et al., 2021). A Kenyan case study demonstrated that income generated from introduction of small-scale seaweed farming in rural villages improved food security and nutrition, and stimulated infrastructural development (Mirera et al., 2020). Dried seaweed for human consumption was the main study output, but it also included manufacturing of seaweed products including soaps, salad, and fish feed (Mirera et al., 2020).
The absence of feed and excreta from seaweed production, accompanied by a rapid increase in biomass, make it one of the least invasive and most productive aquatic food systems (Grebe et al., 2019; Hossain et al., 2021). Seaweed provides ecosystem services, like reducing local eutrophication via nutrient extraction and re-oxygenation, and could be used for amelioration near fish farms (van den Burg et al., 2021). For instance, large-scale cultivation of red algae Gracilaria lemaneiformis and Pyropia yezoensis has effectively mitigated the incidence of harmful algal blooms in China (Grebe et al., 2019). However, this ability may prove exhaustive in already nutrient-poor areas, and severe nutrient depletion has been documented in areas with intensive seaweed farming (Grebe et al., 2019).
Increasing evidence indicates that seaweed farming stimulates local biodiversity by providing valuable habitat structures and contributing as a direct food subsidy for herbivorous fish and invertebrates (Stentiford et al., 2020; Theuerkauf et al., 2021). Preliminary studies from Southeast Asia and East Africa also suggest that higher species richness and abundance are found in large scale seaweed farms compared with small-scale farms or wild kelp beds (Grebe et al., 2019; Theuerkauf et al., 2021). Structural additions from seaweed aquaculture may prevent seashore erosion (Hossain et al., 2021), while increased activity in this zone may compromise the foraging habitat of seabirds and other wildlife (Theuerkauf et al., 2021). A case study from Canada also points to the different ecosystem-level consequences of production yield and process, finding that small-scale harvest poses minimal impact on recovery rates, survival, and biomass dynamics of kelp (Krumhansl et al., 2017).
Notwithstanding its vast potential, disease outbreaks encouraged by seaweed farming are one of the leading causes of decreasing native seaweed stocks and loss of genetic diversity (van den Burg et al., 2021). Increased ship traffic has accelerated the spread of seaweed species such as wakame (Undaria pinnatifida), earning it a spot among the world's top invasive species (Grebe et al., 2019). A related challenge is that hypoxic effects on meiofaunal populations are caused by eutrophication and associated filamentous algae drifts (Thomas J. B. E. et al., 2021). Furthermore, shading from large-scale kelp farms and use of equipment such as moorings for securing longlines can have detrimental effects on benthic ecosystems and cause entanglement of marine mammals (Grebe et al., 2019). Poorly located seaweed farms may also decrease the heterogeneity of macrofaunal communities, for example through shading and trampling of subtidal seagrass habitats (Theuerkauf et al., 2021).
The synergistic effects of climate change and anthropogenic activity are affecting seaweed farming to a growing extent. Beas-Luna et al. (2020) and Krumhansl et al. (2017) found that kelp ecosystems appear vulnerable to changes in ocean temperature, and that both episodic heat waves and long-term warming induce pronounced effects on kelp formation. This trend is particularly challenging given the role of seaweed as a primary producer, provider of habitat structure, and as a carbon sink. Increased anthropogenic nutrient pollution may cause eutrophication and harmful algal blooms, which are recurring more frequently (Kumar and Sharma, 2020), and elevate contaminant levels in seaweed (Cavallo et al., 2021).
New frontiers in seaweed production may enhance sustainability through multisectoral use (van den Burg et al., 2021). The environmental benefits of seaweed may also be mediated via the protein transition, representing the shift from human diets rich in meat and dairy to utilizing more plant-based protein sources. In an evaluation of the environmental performance of different burgers, in terms of global warming potential and land use, a burger containing up to 60% sugar kelp (Saccharina latissima) scored better, compared to soy- or wheat-based vegetarian burgers (Slegers et al., 2021).
Globally, seaweed and their by-products are used in food products, animal feed, pharmaceuticals, cosmetics, agriculture and biofuels (Grebe et al., 2019). Little evidence supports the role of seaweed as a dietary substitute for macronutrients from terrestrial crops and animals, but its use as fertilizer and animal feed is increasingly bridging the marine-land loop in nutrient circularity (Naylor et al., 2021). As an added benefit, specific species of seaweed may reduce enteric fermentation in ruminants, reducing the climate footprint of beef or mutton production (van den Burg et al., 2021). Algal biomass has also been suggested as a cost-effective replacement for parts of the fish meal in aquaculture, both in terms of environmental output and to offset food security issues attached to the estimated 15.6 million tons of wild fish used in fish meal and oil production annually (Stentiford et al., 2020). However, the wide application of seaweed entails multiple processing routes, many of which are in their infancy. Naylor et al. (2021) proposes that adopting a “biorefinery” approach to seaweed processing, similar to that of agriculture, could add value and minimize waste. The environmental output of processing must also be considered in a circular model. The following steps were suggested: (i) Applying the full mass of seaweed in products and prevent waste. (ii) Taking fractionation steps in an order that yields the most valuable compounds and maintains their functionality. (iii) Investing energy and chemical resources only if it increases the product value. Combined, this may reduce the environmental impact of seaweed processing (Grebe et al., 2019).
To mitigate climate change, it has been estimated that the global seaweed population could sequester the equivalent amount of carbon as mangroves, salt marshes and seagrasses combined (Grebe et al., 2019). Still, to assess whether the absorption of carbon and nutrients during seaweed cultivation contributes to an overall positive environmental effect, full carbon and nutrient cycles need to be modeled (van den Burg et al., 2021). In a cradle-to-gate life cycle assessment, a study of the nutrient return from kelp aquaculture in Sweden (Lemasson et al., 2019) reported that kelp could capture finite nutrients to be recirculated, supporting the circular blue-green bioeconomy. Challenges of kelp aquaculture were also pointed to, identifying land-based seaweed hatcheries as energy intensive compared with cultivation of other low-trophic species such as bivalves (Thomas J. B. E. et al., 2021). Another opportunity is the inclusion of seaweed in integrated multi-trophic aquaculture. Studies with seaweed co-cultured with shrimp, mollusks or salmon have proven successful in terms of commercial yields, providing up to twice the profit compared with mono-cultured seaweed, while absorbing a part of the climatic impact of production (Alemañ et al., 2019).
Implementing a circular approach is also important in a market context. For instance, current strategies in European seaweed production follow a linear pattern, with an emphasis on quantitative yields and reduced-price levels (van den Burg et al., 2021). On the contrary, qualitative aspects are suggested as key drivers toward more circular food systems, and that the focus should be shifted to producing the optimal quantity of seaweed while balancing the carrying capacity of the oceans and keeping the preferences of end-users in mind (Grebe et al., 2019).
Endowed with key micronutrients including vitamin B12, iron, zinc, calcium, selenium, and omega-3 fatty acids, bivalves have a favorable nutritional profile for human health (Lemasson et al., 2019; Farmery et al., 2020). A substantial range in nutrient content has been recorded between species; for example, oysters (Crassostrea gigas) are known as a good source of dietary calcium while other species such as sici-shells (Polinicies aemingiana) have lower concentrations of calcium (Farmery et al., 2020). This variation extends to the marine omega-3 fatty acids where higher average concentrations of EPA and DHA have been determined in clams and oysters than in scallops (Tan et al., 2020). As formerly reviewed by Tan et al. (2021), marine habitats at high latitudes have been positively linked with increasing long-chain marine omega-3 content. The global supply of marine long-chain omega-3 for human consumption is under pressure, and currently meets 30% of global demand (Willer et al., 2021). On average, concentrations of EPA and DHA in bivalves range between 9.50 and 35.28 mg/g, which illustrates the capability of increased bivalve production to satiate this demand (Tan et al., 2020; Willer et al., 2021). This potential remains untapped in many of the world's fastest growing populations, including India and Kenya, which have some of the highest potential for bivalve aquaculture expansion (Gentry et al., 2017).
Possible changes in the nutritional properties of two commercially valuable oysters, Crassostrea gigas and Ostrea edulis, were modeled under climate-change scenarios. Mid- to end-of-century predictions suggest that increased ocean acidification and warming are likely to impair the nutritive composition of both species in terms of reduced concentration of essential minerals and lipids (Lemasson et al., 2019). Furthermore, Oliva et al. (2019) reported that ocean acidification may reduce consumer appeal to bivalve species due to altered color and texture. So far, this has been opposed in a sensory study by Lemasson et al. (2017), indicating that increasing levels of ocean acidification and warming do not significantly alter the sensory properties of the oyster C. gigas. Adapting practices toward safeguarding climate resilient species less prone to quality deterioration is a key to maximizing future nutrient yields from bivalve food systems (Lemasson et al., 2019).
The ability of bivalves to improve water quality also entails absorption of viruses, bacteria, toxic algae, and other marine pollutants from the ocean (Littman et al., 2020; Tan et al., 2021; Naylor et al., 2021). While many pollutants can bioaccumulate in the aquatic food chain, contamination with microplastics is more likely to be found in benthic organisms toward the base of the food chain (Walkinshaw et al., 2020). The occurrence of microplastics in different bivalve species has been documented globally (Li et al., 2021; Andrade-Rivas et al., 2022), with higher concentrations of microplastics determined in areas with high anthropogenic activity. In samples from China and Taiwan, bivalves contained 4–57 and 10–62 pieces of microplastics/individual, respectively (Barboza et al., 2018; Chen et al., 2020), while Pacific razor clams from low-impact areas in rural Washington, USA, contained 4–12 pieces of microplastics/individual (Baechler et al., 2020). Consuming packaged or pre-cooked bivalves from markets and supermarkets may also entail higher exposure to microplastics compared with unprocessed bivalves and fresh fish (De-la-Torre, 2020; Gundogdu et al., 2020; Dawson et al., 2021).
Contaminants including pharmaceuticals, pesticides, and PAHs are also of concern, and are typically found in bivalves harvested close to point sources of pollution (Burket et al., 2018). Other harmful components may exhibit strong seasonal variations, including proliferation of paralytic shellfish toxins (PSTs) induced by harmful algal blooms (Harley et al., 2020). Toxin retention is known to vary among species, ranging from fast depuration rates in Mytilus edulis to long-term retention, of months to years, of PSTs in butter clams (Saxidomus gigantea) (Harley et al., 2020). Sanitary control has been enforced as a response to food safety concerns of bivalves in many countries, including a clean aquaculture environment and sanitary processing to secure safe products (Tan et al., 2021). While rigorous quality standards and hygiene control may be applicable for large-scale producers, this needs to be adapted to small-scale actors in the Global South who largely depend on wild catch.
Stentiford et al. (2020) reported that the rapid growth of the bivalve aquaculture industry has taken place under an absence of robust legal frameworks. This misreckoning of potential pitfalls and hazards has had severe impacts along the value chain, and currently limits exports for many countries in the Global South. Ninety percent of aquaculture output is currently not directed toward export, whereby certification has been proposed to increase both the market value and transparency of aquaculture products from low-income countries (Naylor et al., 2021). In the Global North, certification may also provide added value to bivalve products by ensuring the certainty of origin or the exclusivity of traditional production methods (Avdelas et al., 2021). Still, the low compliance between global certification schemes and small-scale actors has been scrutinized, listing insufficient finances, illiteracy, inadequate management skills, and low product demand as persistent barriers (Naylor et al., 2021). The tendency to focus on negative effects of aquaculture in certification schemes has also been critiqued, which, in the case of bivalve aquaculture, misses the mark on the potential provision of ecosystem services (Theuerkauf et al., 2021).
Low barriers of entry are facilitated by the modest need for farming infrastructure, particularly for small-scale production, which makes bivalve farming accessible to more people (Gentry et al., 2019; Tan et al., 2022). However, bivalve farming is also prone to mass mortality outbreaks, exemplified by recurrent mass mortality of juvenile oysters in France due to Ostreid herpes-virus infection. Annual economic losses due to bivalve mass mortalities have been estimated to US$270 million in Northwestern USA alone (Tan et al., 2021). While large farms may have the capacity to sustain such shocks, many small-scale actors do not have the financial flexibility to invest in disease diagnostics or quality seeds (Henriksson et al., 2021). The world's least developed counties are also predicted to have the lowest capacity for climate change adaptations in bivalve aquaculture, largely attributed to governance issues (Stewart-Sinclair et al., 2020). On the other hand, reduced adaptive capacity in bivalve aquaculture in high-income countries has been linked to limited species diversity (Stewart-Sinclair et al., 2020), highlighting the importance of developing policies and frameworks compliant with different socio-ecological settings.
Rebuilding of bivalve stocks in a context of poverty can only be achieved by addressing social challenges (Calvo-Ugarteburu et al., 2016). By including goals to support local management institutions and food security in a South African study, the mussel surface cover increased from 1 to 80% in an exploited area over a 10-year period (Calvo-Ugarteburu et al., 2016). Compared with large-scale actors such as Chile, the fragmentation in European production has led to greater bargaining power allocated to the depurating and processing sectors rather than the producers (Avdelas et al., 2021). In many West African countries, bivalve harvesting is exclusively practiced by women. A study from Guinea-Bissau highlighted that this practice is not only important for household food security, but also for increased purchasing power and female empowerment (Giselle Alvarenga et al., 2022). A similar study in Fiji supported this outcome while adding that female actors in small-scale bivalve harvest are often marginalized and need greater participation in management decisions and policies (Thomas A. et al., 2021). This current tension leads to the potential loss of traditional ecological knowledge of native bivalve species.
Non-fed bivalve farming is considered one of the most environmentally sustainable food systems (Farmery et al., 2016; Avdelas et al., 2021; Henriksson et al., 2021; Koehn et al., 2022a). Bivalves are among the food products generating the lowest greenhouse gas footprints (Koehn et al., 2022a). The environmental sustainability of non-fed bivalve food systems is attributed to the superfluousness of feed, and the provision of diverse ecosystem services (Farmery et al., 2016; Avdelas et al., 2021; Henriksson et al., 2021). By filtering phytoplankton and accumulating nitrogen and phosphorous from the ambient environment, bivalves mitigate coastal eutrophication (Naylor et al., 2021). In coastal waters, eutrophication may enhance algal blooms, which in turn may deprive water bodies of oxygen or contribute to shellfish poisoning (Estevez et al., 2019). For instance, in 2016 algal blooms in Chile caused substantial mortalities in several bivalve operations which had to close for 2 years, generating extensive economic losses (Naylor et al., 2021). Other beneficial outcomes of bivalve aquaculture include increased abundance and species richness of wild mobile macrofauna (Theuerkauf et al., 2021) and the potential of bivalve farms as net carbon sequesters (Willer et al., 2021). While political incentives to reward measures to enhance species abundance are still in their infancy, farms in Italy are presently awarded “green” certificates for the CO2 uptake of shells during production (Avdelas et al., 2021). This suggests that monetizing ecosystem services and rewarding bivalve industry for sustainable innovations may be a key to stimulate their growth (Theuerkauf et al., 2021).
Nevertheless, the cultivation of bivalves is not exempt from environmental challenges. These include the introduction of non-native invasive species, cultivation gear which may entrap wildlife as well as the release of microplastics, and depleting effects on benthic ecosystems through increased deposition of excrement, increasing eutrophication followed by oxygen depletion (Farmery et al., 2016; Avdelas et al., 2021; Willer et al., 2021). Many of these challenges are attributed to unsustainable management of culturing systems and typically manifest in overstocked farms with inappropriate siting, as reported in case-studies from China (Naylor et al., 2021). One of the overarching challenges remains that expansion of bivalve aquaculture will require occupation of large areas of ocean space, which in many cases are in use for other forms of aquaculture, shoreside infrastructure, or as protected areas (Gentry et al., 2017), requiring shared spatial management. Lack of access to ocean space has been identified as a key barrier in many European countries, where bivalve production is currently experiencing a downward trend (Avdelas et al., 2021). Gentry et al. (2017) projected that bivalve aquaculture still has expansive potential, with areas in warm tropical regions displaying the highest growth potential.
Accounting for the detrimental effects of ocean warming and acidification on species growth (Oliva et al., 2019), end-of-century predictions estimate a global loss of 5–20% of suitable areas for bivalve aquaculture (Cubillo et al., 2021), even though bivalves as an inhabitant of naturally variable environments are considered relatively resilient to environmental change (Shalders et al., 2022). The projected reductions are not evenly dispersed between regions, nor within each exclusive economic zone (EEZ), but climate changes are expected to induce dramatic declines in production potential for major bivalve producers including China, Thailand, and Canada (50–100%) (Froehlich et al., 2018). Adaptive planning, sensitive to location, choice of species, and exploration of alternative initiatives such as offshore aquaculture (also in conjunction with wind farms), will therefore become increasingly important to alleviate the lack of suitable ocean space (Avdelas et al., 2021).
Climate changes have already resulted in increased migration of biotoxin producing algae, causing more frequent algal blooms and mass mortality outbreaks in bivalves (Estevez et al., 2019; Tan et al., 2021). To safeguard sustainability, developing bivalve seeds resistant to specific pathogens represents an opportunity to mitigate disease risk and stabilize production, particularly in large-scale farming systems (Henriksson et al., 2021; Tan et al., 2021). In Europe, economic uncertainty is expected to increase under both moderate and extreme end-of-century carbon emission scenarios, following decreasing trends in most productivity parameters across both species and regions (Cubillo et al., 2021). A study from Canada shows that the sensitivity of bivalves is species-specific, with oysters (Crassostrea virginica) predicted to generally outperform mussels (Mytilus edulis) in future ocean warming scenarios (Littman et al., 2020). They also point to how the continuous urbanization of coastal marine environments may further compromise food safety through micro-debris and human pathogen contamination. In this case, climatic shifts such as increased precipitation are also forecasted to enhance bacterial transportation offshore, thus adding to the causal nexus (Colaiuda et al., 2021).
The reliance on natural ecosystems for feed entails that including bivalve species in polyculture systems or integrated multitrophic aquaculture may allow for greater nutrient-circularity (Farmery et al., 2016). Co-culturing bivalves and fed monoculture species such as salmon, may be mutually beneficial, where by-products from one species act as a fertilizer or food for another species and vice versa (Avdelas et al., 2021). As a net result, both the use of feed and the environmental impact may be reduced while producing more aquatic foods to support healthy diets (Farmery et al., 2016). One of the challenges in bivalve cultivation is the low edible yield. Constituting as little as 10% for some species, aggregation of shell waste continues to be a barrier to sustainable growth (Henriksson et al., 2021). Shell valorization routes are facing multiple hinders, including cleaning and preparation, long distance transportation of shells to points of utilization, and an overall dearth of waste-management regulations (Morris et al., 2021). Regardless, utilization of shells is well-established in a number of products used in food production systems, including fertilizers and supplements for poultry and livestock (Naylor et al., 2021) as well as water treatment systems and biofilters (Summa et al., 2022).
Phosphorous is one of the cornerstones of global food security, and is essential for optimal growth of plants, aquatic foods and mammals. The current extraction rate of phosphorous is unsustainable and is expected to be depleted within the next 50–100 years, giving rise to a major sustainability challenge (Morris et al., 2021). Upscaling bivalve production and harvest shows great potential toward achieving a more circular phosphorous-cycle, by capturing excessive phosphorous in marine environments for use on land (Thomas J. B. E. et al., 2021). Calcium carbonate is commonly used as a liming agent and is currently being mined in large quantities. An added incongruity therefore exists in the circular valorization of bivalve shells, which are mainly composed of calcium carbonate, but in many cases are considered waste (Morris et al., 2021). The loss of shells as structural components, such as reef building oysters, have also been identified as detrimental to many ecosystems. In terms of environmental circularity, simply cleaning and returning shells to their marine environment may therefore be the most cost-effective solution to conserve vulnerable ecosystems (Morris et al., 2021).
Tuna, with its large stocks in the Indian and Pacific Oceans, represents a cornerstone in current aquatic food systems (Bell et al., 2015; Lucena-Frédou et al., 2021). Renowned for its high protein content (25/100 g), tuna is also a source of vitamin A, vitamin B12 and iron (Farmery et al., 2020). Tuna is an essential protein source for food security in the Pacific Island Countries and Territories (PICTs) (Syddall et al., 2022b,a) where 50–90% of animal protein stems from marine resources, mainly caught in small-scale fisheries (SSF) (Pilling et al., 2015; Bell et al., 2018). Population growth and climate-change induced shifts in tuna abundance are modeled and observed to challenge this resource base, and in the future other sources will need to complement tuna to maintain current protein consumption levels (Bell et al., 2015; Pilling et al., 2015; Erauskin-Extramiana et al., 2019; Andriamahefazafy et al., 2020; Nicol et al., 2022; Tran et al., 2022).
Balancing revenues from foreign fishing fleets while ensuring local availability is one of the dilemmas in managing tuna fisheries in PICTs (James et al., 2018), highlighting a common challenge in the Global South where food insecurity is increasing because local aquatic resources are exported. Increased access to processed foods has spurred the Westernization of Pacific Islanders' diets, leading to the world's highest prevalence of obesity, and associated non-communicable diseases, co-occurring alongside micronutrient deficiencies (Farmery et al., 2020).
In Tuvalu (James et al., 2018), it was estimated that 266 Mt of edible fish are lost from the SFF due to foreign industrial fishing. Bycatch from global high-seas fisheries targeting tuna and tuna-like species amount to ~270,000 Mt annually, comprising small non-targeted tunas and more than 50 other fish species (Sardenne et al., 2020). Transshipping some of this bycatch to local ports has been presented as an opportunity to stimulate food availability (James et al., 2018). In analyses of 20 by-caught species, most were good sources of omega-3 fatty acids and protein, reinforcing their potential as a complementary nutrient supply (Sardenne et al., 2020). This approach has shown promise in Nauru and Tuvalu, where non-target species could provide an additional 20 kg protein per capita per annum (Pilling et al., 2015). Yet, the levels and stability of non-target catches are difficult to quantify and need further study. Further negative effects of transshipment in Tuvalu included lost employment days, reduced catches and potential losses in income due to reduced tuna availability (James et al., 2018). Potential benefits, such as spending in local businesses and by-catch offloads, were likely offset by a Pareto loss whereby benefits do not befall those who experience losses.
Canning tuna extends shelf life, boosting availability and affordability for low-income consumers (Bell et al., 2015). Newly established fish processing plants in Papua New Guinea are increasing the availability of tuna to the population and providing thousands of jobs (Pilling et al., 2015). However, it is difficult to compete with the cost-effective mass production in countries such as Thailand and Indonesia (Weng et al., 2015). The growing appreciation of sustainable seafood presents market potential through certification programs. Yet, a case study on Indonesian SFF of tuna reveals a disconnect between Northern consumer preferences and the infrastructure required in the Global South to obtain certifications (e.g., Fair Trade, MSC) (Duggan and Kochen, 2016).
Typical for top-predators, tunas are also a source of contaminants, including heavy metals, persistent organic pollutants, and microplastic (Farmery et al., 2020; Henriksson et al., 2021). Methylmercury levels in tunas can reach high concentrations, raising food safety concerns for populations with high intakes (Farmery et al., 2020). In a situation of decreased tuna exports and increased local consumption in PICTs, consumption limits need revision, and appropriate consumption limits for various tuna species and sizes must be established. Furthermore, the occurrence of microplastic in the gastrointestinal tract of tunas varies between species, ranging from 9.4% in skipjack tuna to 23.4% in yellowfin tuna (Walkinshaw et al., 2020). Currently, food safety monitoring for microplastics is in its early stages, as harmonized and standardized protocols have yet to be developed.
Tropical tunas are also exposed to ciguatoxins, which bioaccumulate and may cause Ciguatera fish poisoning (Farmery et al., 2020). Fish in the Scombridae family are also prone to post mortem bacterial formation of histamine, also known as scombrotoxin (Bell et al., 2018), possibly causing histamine intoxication of consumers. This does not only pertain to high-intensive processing, such as canning, but also to improving traditional tuna processing methods such as brining, smoke curing, and drying, particularly in areas with limited cooling facilities (Pilling et al., 2015).
The world's tuna fishery is facing many challenges as it seeks to conform to equitable and sustainable management (Weng et al., 2015). Tuna is the most important group of fishes by catch volume in the SSF of PICTs, and other countries such as Indonesia (Willis and Bailey, 2020). Almost half of households in PICTs acquire major parts of their income from SSF, from fishers and traders to processors and distributors (Weng et al., 2015). The exploitation of tuna also delivers important economic benefits to PICTs through the sale of fishing access rights to industrial distant water fleets. Effective management policy for tuna must therefore consider the socioeconomic importance of both small-scale and industrial sectors.
The trade-off between catching low-value juvenile tuna for maximum food availability vs. high-value adult tuna for export was identified as a major management tradeoff in the Western Central Pacific (Willis and Bailey, 2020). A disproportionate allocation of capacity-enhancing subsidies to the industrial sector was also pointed to, in addition to the skewness of workforce involved in industrial fisheries vs. SSF and the social benefits that follow suit (Willis and Bailey, 2020). A 13-year tagging experiment of skipjack and yellowfin tuna off eastern Papua New Guinea showed how industrial fishing activity may limit the availability and access of tuna to SSF (Leroy et al., 2016). Area closures for industrial fisheries may therefore improve access and increase catches for SSF.
Bell et al. (2015) emphasized the neglect of food security in tuna fisheries policy and suggested prioritizing the protection of economic benefits derived from selling licenses to distant water fishing nations in PICTs to improve local food security. By allowing distant water fishing in their EEZs, achieving sustainable fisheries in PICTs increasingly depends on decisions made by distant water fishing nations. Foregoing domestic fisheries for access payments from distant water fleets may result in negative trade-offs, where fishery resources are not equitably compensated through access fees and retention of local fleets (Nichols et al., 2015). A case study in Ghana, where tuna also represents the biggest seafood export, uncovered structural changes in tuna production from traditional SSF to Asia owned industrial purse-seine fishing (O'Neill et al., 2018).
In a review of tuna fisheries management documents in PICTs, Karcher et al. (2020) found that tuna fisheries have an established hard policy arena, with most legal instruments focused on offshore fisheries and illegal fishing. In contrast, food security, sustainability, and climate change tended to be covered in non-binding strategies. To maintain the contribution of SSF to food security in PICTs, policies should facilitate easier access to the nearshore tuna resources. This includes extending the use of Fish Attraction Devices (FAD), as a part of national infrastructure to safeguard food sovereignty. However, as Pilling et al. (2015) suggest, policy decisions in tuna fisheries should be tailored at the national level to account for local differences in tuna availability and access, cost efficiency, and social significance.
Climate change exacerbates the economic disruptions in tuna fisheries, making sustainable management of the sector crucial for achieving key development goals in PICTs and other dependent nations amidst growing uncertainties (Evans et al., 2015). Current SSF tuna catches are poorly documented and are managed at national and local levels in PICTs (Weng et al., 2015). Combined with the hesitancy of some distant water fishing nations to convey precise harvest numbers, increasing fishery monitoring, research, and capacity building through intersectoral collaboration and mutual transparency is essential to move forward. Evans et al. (2015) identified a wide range of research priorities to progress tuna fisheries' management under climate change scenarios, including refining climate models to more accurately forecast tuna distribution and abundance, in addition to assessing optimal access rights and the influence different scenarios of tuna distribution and abundance have on decision power.
Modeling long term economic consequences of supply shocks from climate change in four fish exporting PICTs, Dey et al. (2016b) reported that without policy advancements, several PICTs risk becoming net importers of fish by 2050. In a similar case study from India, responses in mackerel tuna (Euthynnus affinis) production under long-term climate change (4°C increase) showed a projected decrease in catch and food security, even under a sustainable management scenario (Das et al., 2020). Dueri et al. (2016) also investigated the interactions between climate change and socioeconomic development under different skipjack tuna (Katsuwonus pelamis) fishery management strategies. The study found that a maximum sustainable yield strategy may become increasingly profitable due to increased fishing efficiency from technological advancements, while the low catches and high prices resulting from a maximum economic yield strategy comes up short in terms of future food security. These results call for increased adaptive capacity in tuna fisheries, and to prioritize strengthening the resilience of coastal populations. However, future reduced tuna catches may still be sufficient to support local food security if policy is designed to reallocate tuna resources toward local consumption (Erauskin-Extramiana et al., 2019).
Harvesting tuna from the top of the food web releases lower tier groups from predation, which may impact the whole ecosystem through top-down and bottom-up processes (Weng et al., 2015). Tuna distributions are largely conditioned by ocean temperature, and their widespread populations may therefore be good indicators of the effects of climate change (Weng et al., 2015). Erauskin-Extramiana et al. (2019) estimated end-of-century changes in distribution and abundance of six tuna species under a high-greenhouse gas concentration scenario. They found that temperate tunas like albacore (Thunnus alalunga), Atlantic bluefin (Thunnus thynnus), southern bluefin (Thunnus maccoyii), and tropical bigeye tuna (Thunnus obesus) were expected to shift poleward and decline in the tropics. In contrast, skipjack and yellowfin (Thunnus albacares) tunas were projected to become more abundant in the tropics and EEZs of most PICTs (Erauskin-Extramiana et al., 2019). In a study by Dueri et al. (2016), projections for the global skipjack biomass showed an increase in scenarios for 2050 and 2095, with major changes in distribution patterns. Concurrent with Erauskin-Extramiana et al. (2019) and Dueri et al. (2016) found that the Pacific Ocean is likely to continue dominating the skipjack tuna fisheries, and that distribution in this area may shift eastward. This regional distribution shift is likely to impact the economy and food security of several PICTs, with a projected increase in tuna abundance in Fiji and Vanuatu, stable supply in the Solomon Islands and a decrease westward (Dey et al., 2016b). For mackerel tuna (Euthynnus affinis), end-of-century changes in ocean temperature and primary production may negatively impact the productivity of Indian tuna fisheries (Das et al., 2020). According to an ecosystem model, ocean warming will have the most pronounced effects leading to an eastward shift in the population by 2050 (Nicol et al., 2022). These projected changes will likely impact all types of fishing activities in the Pacific, suggesting marked losses (75,000–149,000 Mt) in the combined catches from Papua New Guinea, Solomon Islands, Nauru, Federated states of Micronesia, and the Republic of the Marshall Islands. For the SSF in the region, the catch reduction was estimated to 29,000 Mt. On a global scale, tuna fisheries are most vulnerable to climate change in the Global South (Erauskin-Extramiana et al., 2019).
Increasing the number of FADs has been proposed as a sustainable way of boosting tuna catches in SSF (Dey et al., 2016a; Bell et al., 2018). However, a related challenge is the associated reduction in tuna prices expected from increased use, estimated to increase toward the mid-century (Dey et al., 2016a). Tuna ranching represents another adaptive strategy, where wild bluefin juveniles are captured, fed, and raised to maturity. However, a systematic analysis of Atlantic bluefin tuna governance found that tuna ranching appears to promote unsustainable harvest (Epstein et al., 2014). Another challenge is attached to the high environmental footprint of niche species aimed for high-income consumers, including Pacific bluefin tuna (Thunnus orientalis), which has not benefited from efficiency improvements brought by the blue economy (Henriksson et al., 2021). In this context, a study by Andriamahefazafy et al. (2020) spotlights the paradox of the blue economy, where sustainability and economic growth are presented as jointly achievable. This is spurred by the geopolitical web of tuna access, combined with the stock dependency of local populations, which makes sustainable management measures difficult to implement (Andriamahefazafy et al., 2020).
Tuna fisheries generate food waste from by-catch, on-board waste, home waste, and during processing, which is limiting its circular stance (Sardenne et al., 2020). To increase production value in PICTs, tuna bycatch and discards are required by law to be offloaded at port (James et al., 2018). Retention of bycatch in a single port in Tuvalu amounted to 10–30 Mt in 1 year. While being labeled as an untapped resource, local access, unstable supply, and local food preferences are obstacles for the utilization of bycatch (Pilling et al., 2015). However, retaining non-targeted tunas that are considered too small for canning remains a potential way of reducing food waste in the tuna fishery. Also, tuna trimmings are used for fish meal in countries such as Thailand, which has both lowered the ratio of wild fish inputs in aquafeed and reduced waste (Naylor et al., 2021).
To achieve sustainable trade-offs between the multiple objectives of aquatic food systems requires a thorough understanding of how challenges and opportunities are linked with the SDGs and each other. The challenges and opportunities identified in the three cases were categorized in broader groups (see Supplementary material) and then mapped to the relevant SDGs. This allowed a clearer understanding of their interconnection and impact on sustainability.
In the seaweed food system (Figure 4) the opportunities (19) show more linkages (95) to the SDGs than the challenges (17; 45). Five opportunities, including “Fertilizer,” “Feed,” “Multitrophic aquaculture,” “Food security and nutrition,” and “Economy,” were linked to all relevant SDGs, while challenges lacked such comprehensive connections with links to five or fewer SDGs. Some challenges and opportunities shared the same SDG, and their success was interdependent, requiring addressing the linked challenge to seize the opportunity (e.g., challenges “Species variation, nutrients” and “Species variation, contaminants” linked with opportunities “Proteins & Nutrients” and “Food security and nutrition”).
Figure 4. Challenges and opportunities of future seaweed food systems in relation to achieving the SDGs (1: No Poverty, 2: Zero Hunger, 3: Good Health and Well-being, 5: Gender equality, 12: Responsible Consumption and Production, 13: Climate Action, 14: Life Below Water). Opportunities and challenges identified in the review were aggregated in categories and linked to the SDGs they impact (one category can impact several SDGs, and the number of links from the SDGs to categories are indicated indicated in black font to the bottom right of the SDG icon and reflected by the thickness of the connecting line). Seaweed image by Nasir Udin from Noun Project (CCBY3.0).
Unlike the seaweed case study, challenges (16) in bivalve aquaculture had more linkages (44) with the SDGs than opportunities (13; 29) (Figure 5). The scope of SDG links to challenges and opportunities for bivalves was narrower than that for seaweed, with challenges having a maximum of four SDGs linked and opportunities with three. More challenges than opportunities were linked to SDGs 2, 3, 12, and 14, whereas for SDG 13, there were more opportunities linked than challenges. Like seaweed, various opportunities and challenges were linked, such as the challenges “Biproduct valorization” and the opportunities “Shells as fertilizer” and “Recycle shell.”
Figure 5. Challenges and opportunities of future bivalve farming and harvesting food systems in relation to achieving the SDGs (1: No Poverty, 2: Zero Hunger, 3: Good Health and Well-being, 5: Gender equality, 12: Responsible Consumption and Production, 13: Climate Action, 14: Life Below Water). Opportunities and challenges identified in the review were aggregated in categories and linked to the SDGs they impact (one category can impact several SDGs, and the number of links from the SDGs to categories are indicated in black font to the bottom right of the SDG icon and reflected by the thickness of the connecting line). Seashell image by Jooyun Lee from Noun Project (CCBY3.0).
In the tuna case study (Figure 6), there was a notable difference in the number of challenges (17) vs. opportunities (12), with challenges having more linkages (66) to the SDGs than opportunities (42). Three challenges were linked to all SDGs (“Achieve both sustainability and economic growth,” “Management trade-offs between sectors,” and “Profit takes priority in policy”), while the maximum number of linked SDGs to an opportunity was five (“Tuna canning”). Similar as for seaweed and bivalves, several challenges and opportunities were linked, such as the challenge “Profit takes priority in policy” and the opportunity “Reallocate tuna resources to boost local food security.”
Figure 6. Challenges and opportunities of future small-scale tuna food systems in relation to achieving the SDGs (1: No Poverty, 2: Zero Hunger, 3: Good Health and Well-being, 5: Gender equality, 12: Responsible Consumption and Production, 13: Climate Action, 14: Life Below Water). Opportunities and challenges identified in the review were aggregated in categories and linked to the SDGs they impact (one category can impact several SDGs, and the number of links from the SDGs to categories are indicated in black font to the bottom right of the SDG icon and reflected by the thickness of the connecting line). DWN, Distant Water Nations; IUU, illegal, unregulated and unreported; FS, food security; FAD, fish attracting device. Tuna fish image by Vallone Design from Noun Project (CCBY3.0).
The significance of marine ecosystem health was evident in all three cases. SDG 14 exhibited the highest number of linkages in each case. However, the SDG with the fewest total links varied by case, with SDG 5 “Gender equality”, SDG 1 “No poverty”, and SDG 12 “Responsible consumption and production” having the fewest links in the seaweed, bivalve, and tuna cases, respectively. SDG 14 “Life below water” is crucial for realizing the potential of aquatic food systems, not only due to its fundamental role in food production but also because anthropogenic impacts such as pollution and emerging threats like microplastics can significantly affect the entire ecosystem.
Our linkage analysis distinctly reveals the intricate interconnections between challenges and opportunities related to the SDGs. Many of these challenges and opportunities are intertwined with multiple SDGs, highlighting the interdependency among them. Furthermore, the analysis demonstrates that resolving linked challenges is essential for realizing numerous opportunities. Collectively, this underscores the overarching theme of navigating intricate and multi-level tradeoffs to attain sustainable future seafood systems.
The present paper outlines actionable steps for global engagement, offering a realistic prospect for change by 2030 being aligned with recent studies (Farmery et al., 2022; Tigchelaar et al., 2022). The three case studies represent examples of aquatic food systems that must evolve to align with future blue economy goals (Farmery et al., 2021a). Other relevant aquatic food systems could have been analyzed, but our scope was to provide relevant examples without being comprehensive.
Our analysis reveals a spectrum of challenges and opportunities interlinked to the SDGs, emphasizing the absence of easy solutions for achieving sustainability. Instead of a straightforward path, navigating a complex terrain of multi-level and interconnected tradeoffs is essential for aquatic food systems to fully realize their potential. At its essence, this presents a wicked problem, requiring a delicate balance between conflicting objectives to achieve a sustainable compromise. Tackling such wicked problems lies at the heart of humanity's efforts to confront overarching challenges like climate change and biodiversity loss, all the while ensuring present and future health, equity, and livelihoods for individuals, the private sector, and nations. One potential solution, as evident in our cases, involves the development of enhanced and tailored spatial management strategies to support the achievement of SDGs while addressing challenges within seaweed, bivalve, and tuna systems. Such spatial management should build on the international momentum for sustainable ocean plans championed by the UN Ocean Decade and the High Level Panel for A Sustainable Ocean Economy (Ocean Panel, 2021), as well as the FAO Blue Transformation Roadmap 2022–2030 (FAO, 2022a) encompassing its targets and priority actions to be used as checklists for actions to be taken to achieve sustainability of future seafood systems.
Implementing such a proposed multi-sectoral spatial management may appear intricate, with trade-offs among multiple objectives. Nevertheless, there are conceptual and computational tools that can guide us toward balanced management approaches for sustainability. Fuzzy cognitive mapping (FCM), a qualitative approach, has proven valuable in engaging stakeholders to explore various management options in complex settings (Jetter and Kok, 2014). Integrated ecological-economic models can also aid in developing management actions that address diverse objectives (Briton et al., 2019). These approaches, already employed by fisheries management advisory groups such as the International Council for the Exploration of the Sea (ICES) (Olsen et al., 2023) and National Oceanic and Atmospheric Administration (NOAA) (DePiper et al., 2021), can be easily adapted for broader applications in aquatic food systems settings. Shared among all these approaches is the capacity to systematically explore diverse potential management solutions for the identified tradeoffs. This involves a comprehensive analysis of strengths, weaknesses, opportunities, and challenges, which must be presented clearly for decision-makers to forge informed resolutions that prioritize balanced sustainability. With the impending demographic changes, as the global population approaches 9.6 billion, with a significant increase in the Global South, it becomes crucial to have a well-established and validated toolbox for integrated spatial management. This toolbox should be readily accessible and tested, facilitating its easy implementation in countries with expansive strategies for developing their Blue Economies.
Leveraging the current momentum for location-based marine management, marine spatial planning (Ehler, 2021) and sustainability focus of the UN Decade for Ocean Science, provides a unique chance to design future strategies that enable aquatic food systems to expand, contributing to SDGs, particularly benefiting activities in SSF and aquaculture.
AH: Conceptualization, Data curation, Formal analysis, Investigation, Methodology, Visualization, Writing – original draft, Writing – review & editing. LF: Conceptualization, Formal analysis, Funding acquisition, Investigation, Methodology, Project administration, Resources, Supervision, Writing – original draft, Writing – review & editing. TK: Conceptualization, Investigation, Methodology, Writing – original draft, Writing – review & editing. MM: Conceptualization, Data curation, Formal analysis, Investigation, Methodology, Visualization, Writing – original draft, Writing – review & editing. JP: Conceptualization, Investigation, Writing – original draft, Writing – review & editing. TM: Conceptualization, Formal analysis, Investigation, Methodology, Visualization, Writing – original draft, Writing – review & editing. ES: Conceptualization, Investigation, Methodology, Visualization, Writing – original draft, Writing – review & editing. MS: Conceptualization, Investigation, Writing – original draft, Writing – review & editing. BL: Formal analysis, Investigation, Methodology, Writing – original draft, Writing – review & editing. RH: Conceptualization, Formal analysis, Investigation, Methodology, Visualization, Writing – original draft, Writing – review & editing. EO: Conceptualization, Data curation, Funding acquisition, Investigation, Methodology, Project administration, Supervision, Visualization, Writing – original draft, Writing – review & editing.
The author(s) declare financial support was received for the research, authorship, and/or publication of this article. We wish to acknowledge support from the IMR research program for Safe and Healthy Seafood (project number 3680_15387) for this work and support of the UN Ocean Decade Project “ClimeFOOD”, the tall ship S/S “Statsraad Lehmkuhl” foundation and its crew, and the One Ocean Expedition for providing a stimulating venue for the workshop where the case studies were identified.
Even Fjære is thanked for help with the literature review.
The authors declare that the research was conducted in the absence of any commercial or financial relationships that could be construed as a potential conflict of interest.
The author(s) declare that Generative AI was used in the creation of this manuscript. During the preparation of this work the authors used Chat GPT to improve the language and grammar of parts of the manuscript. After using this tool, the authors reviewed and edited the content as needed and take full responsibility for the content of the publication.
All claims expressed in this article are solely those of the authors and do not necessarily represent those of their affiliated organizations, or those of the publisher, the editors and the reviewers. Any product that may be evaluated in this article, or claim that may be made by its manufacturer, is not guaranteed or endorsed by the publisher.
The Supplementary Material for this article can be found online at: https://www.frontiersin.org/articles/10.3389/focsu.2024.1504689/full#supplementary-material
Alemañ, A., Espi, R., Robledo, D., and Hayashi, L. (2019). Development of seaweed cultivation in Latin America: current trends and future prospects. Phycologia 58, 462–471. doi: 10.1080/00318884.2019.1640996
Andrade-Rivas, F., Afshari, R., Yassi, A., Mardani, A., Taft, S., Guttmann, M., et al. (2022). Industrialization and food safety for the Tsleil-Waututh Nation: an analysis of chemical levels in shellfish in Burrard Inlet. Environ. Res. 206:112575. doi: 10.1016/j.envres.2021.112575
Andriamahefazafy, M., Bailey, M., Sinan, H., and Kull, C. A. (2020). The paradox of sustainable tuna fisheries in the Western Indian Ocean: between visions of blue economy and realities of accumulation. Sustain. Sci. 15, 75–89. doi: 10.1007/s11625-019-00751-3
Avdelas, L., Edo, A. M., Borges Marques, A. C., Cano, S., Capelle, J. J., Carvalho, N., et al. (2021). The decline of mussel aquaculture in the European Union: causes, economic impacts and opportunities. Rev. Aquacult. 13, 91–118. doi: 10.1111/raq.12465
Baechler, B. R., Granek, E. F., Mazzone, S. J., Nielsen-Pincus, M., and Er, S. M. (2020). Microplastic exposure by razor clam recreational harvester-consumers along a sparsely populated coastline. Front. Mar. Sci. 7:588481. doi: 10.3389/fmars.2020.588481
Barboza, L. G. A., Vethaak, A. D., Lavorante, B., Lundebye, A. K., and Guilhermino, L. (2018). Marine microplastic debris: an emerging issue for food security, food safety and human health. Mar. Pollut. Bull. 133, 336–348. doi: 10.1016/j.marpolbul.2018.05.047
Beas-Luna, R., Micheli, F., Woodson, C. B., Carr, M., Malone, D., Torre, J., et al. (2020). Geographic variation in responses of kelp forest communities of the California Current to recent climatic changes. Glob. Chang. Biol. 26, 6457–6473. doi: 10.1111/gcb.15273
Bell, J. D., Allain, V., Allison, E. H., Andrefoueet, S., Andrew, N. L., Batty, M. J., et al. (2015). Diversifying the use of tuna to improve food security and public health in Pacific Island countries and territories. Mar. Policy 51, 584–591. doi: 10.1016/j.marpol.2014.10.005
Bell, J. D., Cisneros-Montemayor, A., Hanich, Q., Johnson, J. E., Lehodey, P., Moore, B. R., et al. (2018). Adaptations to maintain the contributions of small-scale fisheries to food security in the Pacific Islands. Mar. Policy 88, 303–314. doi: 10.1016/j.marpol.2017.05.019
Bennett, A., Basurto, X., Virdin, J., Lin, X., Betances, S. J., Smith, M. D., et al. (2021). Recognize fish as food in policy discourse and development funding. Ambio 50, 981–989. doi: 10.1007/s13280-020-01451-4
Blue Food Assessment (2021). Building Blue Food Futures for People and the Planet. The Report of the Blue Food Assessment. Stanford Center for Ocean Solutions & Center on Food Security and the Environment, USA. Available at: https://bluefood.earth/policy/
Briton, F., Macher, C., Merzeréaud, M., Le Grand, C., Fifas, S., and Thébaud, O. (2019). Providing integrated total catch advice for the management of mixed fisheries with an eco-viability approach. Environ. Model. Assess. 25, 307–325. doi: 10.1007/s10666-019-09685-7
Burket, S. R., Sapozhnikova, Y., Zheng, J. S., Chung, S. S., and Brooks, B. W. (2018). At the intersection of urbanization, water, and food security: determination of select contaminants of emerging concern in mussels and oysters from Hong Kong. J. Agric. Food Chem. 66, 5009–5017. doi: 10.1021/acs.jafc.7b05730
Butcher, H., Burkhart, S., Paul, N., Tiitii, U., Tamuera, K., Eria, T., et al. (2020). Role of seaweed in diets of Samoa and Kiribati: exploring key motivators for consumption. Sustainability 12:7356. doi: 10.3390/su12187356
Calvo-Ugarteburu, G., Raemaekers, S., and Halling, C. (2016). Rehabilitating mussel beds in Coffee Bay, South Africa: towards fostering cooperative small-scale fisheries governance and enabling community upliftment. Ambio 46, 214–226. doi: 10.1007/s13280-016-0823-4
Cattaneo, A., Sánchez, M. V., Torero, M., and Vos, R. (2021). Reducing food loss and waste: five challenges for policy and research. Food Policy 98:101974. doi: 10.1016/j.foodpol.2020.101974
Cavallo, G., Lorini, C., Garamella, G., and Bonaccorsi, G. (2021). Seaweeds as a “palatable” challenge between innovation and sustainability: a systematic review of food safety. Sustainability 13:7652. doi: 10.3390/su13147652
Chen, J. Y. S., Lee, Y. C., and Walther, B. A. (2020). Microplastic contamination of three commonly consumed seafood species from taiwan: a pilot study. Sustainability 12:9543. doi: 10.3390/su12229543
Colaiuda, V., Di Giacinto, F., Lombardi, A., Ippoliti, C., Giansante, C., Latini, M., et al. (2021). Evaluating the impact of hydrometeorological conditions on E. coli concentration in farmed mussels and clams: experience in Central Italy. J. Water Health 19, 512–533. doi: 10.2166/wh.2021.203
Costello, C., Cao, L., Gelcich, S., Cisneros-Mata, M. Á., Free, C. M., Froehlich, H. E., et al. (2020). The future of food from the sea. Nature 588, 95–100. doi: 10.1038/s41586-020-2616-y
Cubillo, A. M., Ferreira, J. G., Lencart-Silva, J., Taylor, N. G. H., Kennerley, A., Guilder, J., et al. (2021). Direct effects of climate change on productivity of European aquaculture. Aquac. Int. 29, 1561–1590. doi: 10.1007/s10499-021-00694-6
Das, I., Lauria, V., Kay, S., Cazcarro, I., Arto, I., Fern, J. A., et al. (2020). Effects of climate change and management policies on marine fisheries productivity in the north-east coast of India. Sci. Total Environ. 724:138082. doi: 10.1016/j.scitotenv.2020.138082
Dawson, A. L., Santana, M. F. M., Miller, M. E., and Kroon, F. J. (2021). Relevance and reliability of evidence for microplastic contamination in seafood: a critical review using Australian consumption patterns as a case study. Environ. Pollut. 276:116684. doi: 10.1016/j.envpol.2021.116684
De-la-Torre, G. E. (2020). Microplastics: an emerging threat to food security and human health. J. Food Sci. Technol. 57, 1601–1608. doi: 10.1007/s13197-019-04138-1
DePiper, G., Gaichas, S., Muffley, B., Ardini, G., Brust, J., Coakley, J., et al. (2021). Learning by doing: collaborative conceptual modelling as a path forward in ecosystem-based management. ICES J. Mar. Sci. 78, 1217–1228. doi: 10.1093/icesjms/fsab054
Dey, M. M., Gosh, K., Valmonte-Santos, R., Rosegrant, M. W., and Chen, O. L. (2016a). Economic impact of climate change and climate change adaptation strategies for fisheries sector in Solomon Islands: implication for food security. Mar. Policy 67, 171–178. doi: 10.1016/j.marpol.2016.01.004
Dey, M. M., Rosegrant, M. W., Gosh, K., Chen, O. L., and Valmonte-Santos, R. (2016b). Analysis of the economic impact of climate change and climate change adaptation strategies for fisheries sector in Pacific coral triangle countries: model, estimation strategy, and baseline results. Mar. Policy 67, 156–163. doi: 10.1016/j.marpol.2015.12.011
Dueri, S., Guillotreau, P., Jimenez-Toribio, R., Oliveros-Ramos, R., Bopp, L., and Maury, O. (2016). Food security or economic profitability? Projecting the effects of climate and socioeconomic changes on global skipjack tuna fisheries under three management strategies. Glob. Environ. Change 41, 1–12. doi: 10.1016/j.gloenvcha.2016.08.003
Duggan, D. E., and Kochen, M. (2016). Small in scale but big in potential: opportunities and challenges for fisheries certification of Indonesian small-scale tuna fisheries. Mar. Policy 67, 30–39. doi: 10.1016/j.marpol.2016.01.008
Ehler, C. N. (2021). Two decades of progress in Marine Spatial Planning. Mar. Policy 132:104134. doi: 10.1016/j.marpol.2020.104134
El Bilali, H., Callenius, C., Strassner, C., and Probst, L. (2019). Food and nutrition security and sustainability transitions in food systems. Food Energy Sec. 8:e00154. doi: 10.1002/fes3.154
Epstein, G., Nenadovic, M., and Boustany, A. (2014). Into the deep blue sea: commons theory and international governance of Atlantic Bluefin Tuna. Int. J. Commons 8, 277–303. doi: 10.18352/ijc.410
Erauskin-Extramiana, M., Arrizabalaga, H., Hobday, A. J., Cabre, A., Ibaibarriaga, L., Arregui, I., et al. (2019). Large-scale distribution of tuna species in a warming ocean. Glob. Chang. Biol. 25, 2043–2060. doi: 10.1111/gcb.14630
Estevez, P., Castro, D., Pequeño-Valtierra, A., Giraldez, J., and Gago-Martinez, A. (2019). Emerging marine biotoxins in seafood from european coasts: incidence and analytical challenges. Foods 8:149. doi: 10.3390/foods8050149
Evans, K., Young, J. W., Nicol, S., Kolody, D., Allain, V., Bell, J., et al. (2015). Optimising fisheries management in relation to tuna catches in the western central Pacific Ocean: a review of research priorities and opportunities. Mar. Policy 59, 94–104. doi: 10.1016/j.marpol.2015.05.003
FAO (2019). The State of Food and Agriculture 2019. Moving Forward on Food Loss and Waste Reduction. Rome: FAO.
FAO (2021). The State of Food Security and Nutrition in the World 2021. Transforming Food Systems for Food Security, Improved Nutrition and Affordable Healthy Diets for All. Rome: FAO.
FAO (2022a). Blue Transformation - Roadmap 2022–2030: A Vision for FAO's Work on Aquatic Food Systems. Rome.
FAO (2022b). The State of World Fisheries and Aquaculture 2022. Towards Blue Transformation. Rome: FAO.
FAO IFAD, UNICEF, WFP, and WHO. (2022). The State of Food Security and Nutrition in the World 2022. Repurposing Food and Agricultural Policies to Make Healthy Diets More Affordable. Rome, FAO.
Farmery, A. K., Alexander, K., Anderson, K., Blanchard, J. L., Carter, C. G., Evans, K., et al. (2022). Food for all: designing sustainable and secure future seafood systems. Rev. Fish Biol. Fish 32, 101–121. doi: 10.1007/s11160-021-09663-x
Farmery, A. K., Allison, E. H., Andrew, N. L., Troell, M., Voyer, M., Campbell, B., et al. (2021a). Blind spots in visions of a “blue economy” could undermine the ocean's contribution to eliminating hunger and malnutrition. One Earth 4, 28–38. doi: 10.1016/j.oneear.2020.12.002
Farmery, A. K., Gardner, C., Jennings, S., Green, B. S., and Watson, R. A. (2016). Assessing the inclusion of seafood in the sustainable diet literature. Fish Fish. 18, 607–618. doi: 10.1111/faf.12205
Farmery, A. K., Scott, J. M., Brewer, T. D., Eriksson, H., Steenbergen, D. J., Albert, J., et al. (2020). Aquatic foods and nutrition in the pacific. Nutrients 12:3705. doi: 10.3390/nu12123705
Farmery, A. K., White, A., and Allison, E. H. (2021b). Identifying policy best-practices to support the contribution of aquatic foods to food and nutrition security. Foods 10:1589. doi: 10.3390/foods10071589
Fisher, B., Naidoo, R., Guernier, J., Johnson, K., Mullins, D., Robinson, D., et al. (2017). Integrating fisheries and agricultural programs for food security. Agric. Food Sec. 6:1. doi: 10.1186/s40066-016-0078-0
Froehlich, H. E., Gentry, R. R., and Halpern, B. S. (2018). Global change in marine aquaculture production potential under climate change. Nat. Ecol. Evol. 2, 1745–1750. doi: 10.1038/s41559-018-0669-1
Fung, F., Wang, H.-S., and Menon, S. (2018). Food safety in the 21st century. Biomed. J. 41, 88–95. doi: 10.1016/j.bj.2018.03.003
Ganesan, A. R., Subramani, K., Shanmugam, M., Seedevi, P., Park, S., Alfarhan, A. H., et al. (2019). A comparison of nutritional value of underexploited edible seaweeds with recommended dietary allowances. J. King Saud Univ. Sci. 32, 1206–1211. doi: 10.1016/j.jksus.2019.11.009
Gentry, R. R., Froehlich, H. E., Grimm, D., Kareiva, P., Parke, M., Rust, M., et al. (2017). Mapping the global potential for marine aquaculture. Nat. Ecol. Evol. 1, 1317–1324. doi: 10.1038/s41559-017-0257-9
Gentry, R. R., Ruff, E. O., and Lester, S. E. (2019). Temporal patterns of adoption of mariculture innovation globally. Nat. Sustain. 2, 949–956. doi: 10.1038/s41893-019-0395-y
Gephart, J. A., Henriksson, P. J. G., Parker, R. W. R., Shepon, A., Gorospe, K. D., Bergman, K., et al. (2021). Environmental performance of blue foods. Nature 597, 360–365. doi: 10.1038/s41586-021-03889-2
Giselle Alvarenga, D., Vasconcelos, M. J., and Catarino, L. (2022). Examining the socioeconomic benefits of oysters: a provisioning ecosystem service from the mangroves of Guinea-Bissau, West Africa. J. Coast. Res. 38, 355–360. doi: 10.2112/JCOASTRES-D-21-00055.1
Golden, C. D., Koehn, J. Z., Shepon, A., Passarelli, S., Free, C. M., Viana, D. F., et al. (2021). Aquatic foods to nourish nations. Nature 598, 315–320. doi: 10.1038/s41586-021-03917-1
Grebe, G. S., Byron, C. J., St Gelais, A., Kotowicz, D. M., and Olson, T. K. (2019). An ecosystem approach to kelp aquaculture in the Americas and Europe. Aquac. Rep. 15:100215. doi: 10.1016/j.aqrep.2019.100215
Gundogdu, S., Cevik, C., and Atas, N. T. (2020). Stuffed with microplastics: microplastic occurrence in traditional stuffed mussels sold in the Turkish market. Food Biosci. 37:100715. doi: 10.1016/j.fbio.2020.100715
Haddad, L., Hawkes, C., Webb, P., Thomas, S., Beddington, J., Waage, J., et al. (2016). A new global research agenda for food. Nature 540, 30–32. doi: 10.1038/540030a
Harder, R., Giampietro, M., and Smukler, S. (2021). Towards a circular nutrient economy. A novel way to analyze the circularity of nutrient flows in food systems. Resour. Conserv. Recycling 172:105693. doi: 10.1016/j.resconrec.2021.105693
Harley, J. R., Lanphier, K., Kennedy, E. G., Leighfield, T. A., Bidlack, A., Gribble, M. O., et al. (2020). The Southeast Alaska Tribal Ocean Research (SEATOR) Partnership: addressing data gaps in harmful algal bloom monitoring and shellfish safety in Southeast Alaska. Toxins 12:407. doi: 10.3390/toxins12060407
Henriksson, P. J. G., Troell, M., Banks, L. K., Belton, B., Beveridge, M. C. M., Klinger, D. H., et al. (2021). Interventions for improving the productivity and environmental performance of global aquaculture for future food security. One Earth 4, 1220–1232. doi: 10.1016/j.oneear.2021.08.009
Henriquez-Antipa, L. A., and Carcamo, F. (2019). Stakeholder's multidimensional perceptions on policy implementation gaps regarding the current status of Chilean small-scale seaweed aquaculture. Mar. Policy 103, 138–147. doi: 10.1016/j.marpol.2019.02.042
Hicks, C. C., Cohen, P. J., Graham, N. A. J., Nash, K. L., Allison, E. H., D'Lima, C., et al. (2019). Harnessing global fisheries to tackle micronutrient deficiencies. Nature 574, 95–98. doi: 10.1038/s41586-019-1592-6
HLPE (2020). Food Security and Nutrition- Building a Global Narative Towards 2030. Rome: A report by the High Level Panel of Experts on Food Security and Nutrition of the Committee on World Food Security.
Hossain, M. S., Sharifuzzaman, S. M., Nobi, M. N., Chowdhury, M. S. N., Sarker, S., Alamgir, M., et al. (2021). Seaweeds farming for sustainable development goals and blue economy in Bangladesh. Mar. Policy 128:04469. doi: 10.1016/j.marpol.2021.104469
Huse, G., Baussant, T., Becker, M., Biuw, M., Bødtker, G., Cook, J., et al. (2023). The one ocean expedition: science and sailing for the ocean we want. Rapport fra havforskningen.
IPCC (2022). “Climate change 2022: impacts, adaptation, and vulnerability,” in Contribution of Working Group II to the Sixth Assessment Report of the Intergovernmental Panel on Climate Change, eds. H.-O. Pörtner, D. C. Roberts, M. Tignor, E. S. Poloczanska, K. Mintenbeck, A. Alegría, et al. (Cambridge: Cambridge University Press).
James, P. A. S., Tidd, A., and Kaitu, L. P. (2018). The impact of industrial tuna fishing on small-scale fishers and economies in the Pacific. Mar. Policy 95, 189–198. doi: 10.1016/j.marpol.2018.03.021
Jetter, A. J., and Kok, K. (2014). Fuzzy Cognitive Maps for futures studies—A methodological assessment of concepts and methods. Futures 61, 45–57. doi: 10.1016/j.futures.2014.05.002
Karcher, D. B., Fache, E., Breckwoldt, A., Govan, H., Ilosvay, X. E. E., King, J. K. K., et al. (2020). Trends in South Pacific fisheries management. Mar. Policy 118:104021. doi: 10.1016/j.marpol.2020.104021
Koehn, J. Z., Allison, E. H., Golden, C. D., and Hilborn, R. (2022a). The role of seafood in sustainable diets. Environ. Res. Lett. 17:035003. doi: 10.1088/1748-9326/ac3954
Koehn, J. Z., Allison, E. H., Villeda, K., Chen, Z., Nixon, M., Crigler, E., et al. (2022b). Fishing for health: do the world's national policies for fisheries and aquaculture align with those for nutrition? Fish Fish. 23, 125–142. doi: 10.1111/faf.12603
Krumhansl, K. A., Bergman, J. N., and Salomon, A. K. (2017). Assessing the ecosystem-level consequences of a small-scale artisanal kelp fishery within the context of climate-change. Ecol. Appl. 27, 799–813. doi: 10.1002/eap.1484
Kumar, M. S., and Sharma, S. A. (2020). Toxicological effects of marine seaweeds: a cautious insight for human consumption. Crit. Rev. Food Sci. Nutr. 61, 500–521. doi: 10.1080/10408398.2020.1738334
Lakner, C., Mahler, D. G., Negre, M., and Prydz, E. B. (2022). How much does reducing inequality matter for global poverty? J. Econ. Inequal. 20, 559–585. doi: 10.1007/s10888-021-09510-w
Leandro, A., Pacheco, D., Cotas, J., Marques, J. C., Pereira, L., and Gonçalves, A. M. M. (2020). Seaweed's bioactive candidate compounds to food industry and global food security. Life 10:140. doi: 10.3390/life10080140
Lemasson, A. J., Hall-Spencer, J. M., Kuri, V., and Knights, A. M. (2019). Changes in the biochemical and nutrient composition of seafood due to ocean acidification and warming. Mar. Environ. Res. 143, 82–92. doi: 10.1016/j.marenvres.2018.11.006
Lemasson, A. J., Kuri, V., Hall-Spencer, J. M., Fletcher, S., Moate, R., and Knights, A. M. (2017). Sensory qualities of oysters unaltered by a short exposure to combined elevated pCO2 and temperature. Front. Mar. Sci. 4:352. doi: 10.3389/fmars.2017.00352
Leroy, B., Peatman, T., Usu, T., Caillot, S., Moore, B., Williams, A., et al. (2016). Interactions between artisanal and industrial tuna fisheries: Insights from a decade of tagging experiments. Mar. Policy 65, 11–19. doi: 10.1016/j.marpol.2015.12.001
Li, Q. P., Ma, C. Z., Zhang, Q., and Shi, H. H. (2021). Microplastics in shellfish and implications for food safety. Curr. Opin. Food Sci. 40, 192–197. doi: 10.1016/j.cofs.2021.04.017
Littman, R. A., Fiorenza, E. A., Wenger, A. S., Berry, K. L. E., van de Water, J., Nguyen, L., et al. (2020). Coastal urbanization influences human pathogens and microdebris contamination in seafood. Sci. Total Environ. 736:139081. doi: 10.1016/j.scitotenv.2020.139081
Lucena-Frédou, F., Mourato, B., Frédou, T., Lino, P. G., Muñoz-Lechuga, R., Palma, C., et al. (2021). Review of the life history, fisheries, and stock assessment for small tunas in the Atlantic Ocean. Rev. Fish Biol. Fish 31, 709–736. doi: 10.1007/s11160-021-09666-8
Maire, E., Graham, N. A. J., MacNeil, M. A., Lam, V. W. Y., Robinson, J. P. W., Cheung, W. W. L., et al. (2021). Micronutrient supply from global marine fisheries under climate change and overfishing. Curr. Biol. 31, 4132–4138.e4133. doi: 10.1016/j.cub.2021.06.067
Mirera, D. O., Kimathi, A., Ngarari, M. M., Magondu, E. W., Wainaina, M., and Ototo, A. (2020). Societal and environmental impacts of seaweed farming in relation to rural development: The case of Kibuyuni village, south coast, Kenya. Ocean Coast. Manag. 194:105253. doi: 10.1016/j.ocecoaman.2020.105253
Morris, J. P., Backeljau, T., and Chapelle, G. (2021). Shells from aquaculture: a valuable biomaterial, not a nuisance waste product. Rev. Aquacult. 11, 42–57. doi: 10.1111/raq.12225
Naylor, R. L., Hardy, R. W., Buschmann, A. H., Bush, S. R., Cao, L., Klinger, D. H., et al. (2021). A 20-year retrospective review of global aquaculture. Nature 591, 551–563. doi: 10.1038/s41586-021-03308-6
Nichols, R., Yamazaki, S., Jennings, S., and Watson, R. A. (2015). Fishing access agreements and harvesting decisions of host and distant water fishing nations. Mar. Policy 54, 77–85. doi: 10.1016/j.marpol.2014.12.019
Nicol, S., Lehodey, P., Senina, I., Bromhead, D., Frommel, A. Y., Hampton, J., et al. (2022). Ocean futures for the world's largest yellowfin tuna population under the combined effects of ocean warming and acidification. Front. Mar. Sci. 9:816772. doi: 10.3389/fmars.2022.816772
Nordhagen, S., Lambertini, E., DeWaal, C. S., McClafferty, B., and Neufeld, L. M. (2022). Integrating nutrition and food safety in food systems policy and programming. Glob. Food Sec. 32:100593. doi: 10.1016/j.gfs.2021.100593
Novoslavskij, A., Terentjeva, M., Eizenberga, I., Valcina, O., Bartkevičs, V., and Bērzinš, A. (2016). Major foodborne pathogens in fish and fish products: a review. Ann. Microbiol. 66, 1–15. doi: 10.1007/s13213-015-1102-5
Ocean Panel (2021). 100% Sustainable Ocean Management: An Introduction to Sustainable Ocean Plans. Washington, DC: High Level Panel for a Sustainable Ocean Economy (Ocean Panel), 27.
Oliva, R. D. P., Vasquez-Lavin, F., San Martin, V. A., Hernez, J. I., Vargas, C. A., Gonzalez, P. S., et al. (2019). Ocean acidification, consumers' preferences, and market adaptation strategies in the mussel aquaculture industry. Ecol. Econ. 158, 42–50. doi: 10.1016/j.ecolecon.2018.12.011
Olsen, E., Tomczak, M. T., Lynam, C. P., Belgrano, A., Kenny, A., and Hunsicker, M. (2023). Testing management scenarios for the North Sea ecosystem using qualitative and quantitative models. ICES J. Mar. Sci. 80, 218–234. doi: 10.1093/icesjms/fsac231
O'Neill, E. D., Asare, N. K., and Aheto, D. W. (2018). Socioeconomic dynamics of the Ghanaian tuna industry: a value-chain approach to understanding aspects of global fisheries. Afr. J. Mar. Sci. 40, 303–313. doi: 10.2989/1814232X.2018.1513866
Pilling, G. M., Harley, S. J., Nicol, S., Williams, P., and Hampton, J. (2015). Can the tropical Western and Central Pacific tuna purse seine fishery contribute to Pacific Island population food security? Food Sec. 7, 67–81. doi: 10.1007/s12571-014-0407-8
Prodhan, M. M. H., Khan, M. A., Palash, M. S., and Rahman, M. T. (2022). Nature, extent, and causes of post-harvest losses at fisher and farmer level: an in-depth study. Aquaculture 550:737856. doi: 10.1016/j.aquaculture.2021.737856
Robinson, J. P. W., Nash, K. L., Blanchard, J. L., Jacobsen, N. S., Maire, E., Graham, N. A. J., et al. (2022). Managing fisheries for maximum nutrient yield. Fish Fish. 23, 800–811. doi: 10.1111/faf.12649
Sardenne, F., Bodin, N., Medieu, A., Antha, M., Arrisol, R., Gr, L., et al. (2020). Benefit-risk associated with the consumption of fish bycatch from tropical tuna fisheries. Environ. Pollut. 267:115614. doi: 10.1016/j.envpol.2020.115614
Searchinger, T., Waite, R., Hanson, C., and Ranganathan, J. (2019). A Menu of Solutions to Feed Nearly 10 Billion People by 2050. World Resources Institute, Synthesis Report. Available at: https://files.wri.org/d8/s3fs-public/wrr-food-full-report.pdf
Shalders, T. C., Champion, C., Coleman, M. A., and Benkendorff, K. (2022). The nutritional and sensory quality of seafood in a changing climate. Mar. Environ. Res. 176:105590. doi: 10.1016/j.marenvres.2022.105590
Short, R. E., Gelcich, S., Little, D. C., Micheli, F., Allison, E. H., Basurto, X., et al. (2021). Harnessing the diversity of small-scale actors is key to the future of aquatic food systems. Nat. Food 2, 733–741. doi: 10.1038/s43016-021-00363-0
Slegers, P. M., Helmes, R. J. K., Draisma, M., Broekema, R., Vlottes, M., and Burg, S. (2021). Environmental impact and nutritional value of food products using the seaweed Saccharina latissima. J. Clean. Prod. 319:128689. doi: 10.1016/j.jclepro.2021.128689
Stentiford, G. D., Bateman, I. J., Hinchliffe, S. J., Bass, D., Hartnell, R., Santos, E. M., et al. (2020). Sustainable aquaculture through the One Health lens. Nat. Food 1, 468–474. doi: 10.1038/s43016-020-0127-5
Stewart-Sinclair, P. J., Last, K. S., Payne, B. L., and Wilding, T. A. (2020). A global assessment of the vulnerability of shellfish aquaculture to climate change and ocean acidification. Ecol. Evol. 10, 3518–3534. doi: 10.1002/ece3.6149
Summa, D., Lanzoni, M., Castaldelli, G., Fano, E. A., and Tamburini, E. (2022). Trends and opportunities of bivalve shells' waste valorization in a prospect of circular blue bioeconomy. Resources 11:48. doi: 10.3390/resources11050048
Syddall, V. M., Fisher, K., and Thrush, S. (2022a). Collaboration a solution for small island developing states to address food security and economic development in the face of climate change. Ocean Coast. Manag. 221:106132. doi: 10.1016/j.ocecoaman.2022.106132
Syddall, V. M., Fisher, K., and Thrush, S. (2022b). What does gender have to do with the price of tuna? Social-ecological systems view of women, gender, and governance in Fiji's tuna fishery. Marit. Stud. 21, 447–463. doi: 10.1007/s40152-022-00281-2
Tan, K., Ma, H., Li, S., and Zheng, H. (2020). Bivalves as future source of sustainable natural omega-3 polyunsaturated fatty acids. Food Chem. 311:125907. doi: 10.1016/j.foodchem.2019.125907
Tan, K., Zhang, H. K., Li, S. K., Ma, H. Y., and Zheng, H. P. (2021). Lipid nutritional quality of marine and freshwater bivalves and their aquaculture potential. Crit. Rev. Food Sci. Nutr. 62, 6990–7014. doi: 10.1080/10408398.2021.1909531
Tan, T. Y., Miraldo, M. C., Fontes, R. F. C., and Vannucchi, F. S. (2022). Assessing bivalve growth using bio-energetic models. Ecol. Modell. 473:110069. doi: 10.1016/j.ecolmodel.2022.110069
Theuerkauf, S. J., Barrett, L. T., Alleway, H. K., Costa-Pierce, B. A., St Gelais, A., and Jones, R. C. (2021). Habitat value of bivalve shellfish and seaweed aquaculture for fish and invertebrates: pathways, synthesis and next steps. Rev. Aquacult. 14, 54–72. doi: 10.1111/raq.12584
Thomas, A., Mangubhai, S., Fox, M., Meo, S., Miller, K., Naisilisili, W., et al. (2021). Why they must be counted: Significant contributions of Fijian women fishers to food security and livelihoods. Ocean Coast. Manag. 205:105571. doi: 10.1016/j.ocecoaman.2021.105571
Thomas, J. B. E., Sinha, R., Str, A., Soderqvist, T., Stadmark, J., Franzen, F., et al. (2021). Marine biomass for a circular blue-green bioeconomy?: A life cycle perspective on closing nitrogen and phosphorus land-marine loops. J. Ind. Ecol. 26, 2136–2153. doi: 10.1111/jiec.13177
Tigchelaar, M., Cheung, W. W. L., Mohammed, E. Y., Phillips, M. J., Payne, H. J., Selig, E. R., et al. (2021). Compound climate risks threaten aquatic food system benefits. Nat. Food 2, 673–682. doi: 10.1038/s43016-021-00368-9
Tigchelaar, M., Leape, J., Micheli, F., Allison, E. H., Basurto, X., Bennett, A., et al. (2022). The vital roles of blue foods in the global food system. Glob. Food Sec. 33:100637. doi: 10.1016/j.gfs.2022.100637
Tlusty, M. F., Tyedmers, P., Bailey, M., Ziegler, F., Henriksson, P. J. G., Béné, C., et al. (2019). Reframing the sustainable seafood narrative. Glob. Environ. Change 59:101991. doi: 10.1016/j.gloenvcha.2019.101991
Tran, N., Chan, C. Y., Aung, Y. M., Bailey, C., Akester, M., Cao, Q. L., et al. (2022). Foresighting future climate change impacts on fisheries and aquaculture in vietnam. Front. Sustain. Food Syst. 6:829157. doi: 10.3389/fsufs.2022.829157
UN (2015). United Nations Decade of Action on Nutrition. New York, NY. Available at: https://www.un.org/nutrition/
UN (2021). United Nations Decade of Ocean Science for Sustainable Development. Paris. Available at: https://oceandecade.org/
van den Burg, S. W. K., Dagevos, H., and Helmes, R. J. K. (2021). Towards sustainable European seaweed value chains: a triple P perspective. ICES J. Mar. Sci. 78, 443–450. doi: 10.1093/icesjms/fsz183
van der Oost, R., Beyer, J., and Vermeulen, N. P. E. (2003). Fish bioaccumulation and biomarkers in environmental risk assessment: a review. Environ. Toxicol. Pharmacol. 13, 57–149. doi: 10.1016/S1382-6689(02)00126-6
Walkinshaw, C., Lindeque, P. K., Thompson, R., Tolhurst, T., and Cole, M. (2020). Microplastics and seafood: lower trophic organisms at highest risk of contamination. Ecotoxicol. Environ. Saf. 190:110066. doi: 10.1016/j.ecoenv.2019.110066
Weng, K. C., Glazier, E., Nicol, S. J., and Hobday, A. J. (2015). Fishery management, development and food security in the Western and Central Pacific in the context of climate change. Deep Sea Res. 113, 301–311. doi: 10.1016/j.dsr2.2014.10.025
Willer, D. F., Nicholls, R. J., and Aldridge, D. C. (2021). Opportunities and challenges for upscaled global bivalve seafood production. Nat. Food 2, 935–943. doi: 10.1038/s43016-021-00423-5
Willis, C., and Bailey, M. (2020). Tuna trade-offs: balancing profit and social benefits in one of the world's largest fisheries. Fish Fish. 21, 740–759. doi: 10.1111/faf.12458
Keywords: aquatic food, sustainability, food systems, bivalve, seaweed, tuna
Citation: Hasselberg AE, Frøyland L, Kögel T, Markhus MW, Plata J, van der Meeren T, Sørhus E, Sanden M, Lunestad BT, Hannisdal R and Olsen E (2024) Maximizing the potential of sustainable aquatic food systems for global food security: key opportunities and challenges. Front. Ocean Sustain. 2:1504689. doi: 10.3389/focsu.2024.1504689
Received: 01 October 2024; Accepted: 05 November 2024;
Published: 29 November 2024.
Edited by:
Erlend Moksness, Blue Growth & Marine Services, NorwayReviewed by:
Aleksandar Vidakovic, Swedish University of Agricultural Sciences, SwedenCopyright © 2024 Hasselberg, Frøyland, Kögel, Markhus, Plata, van der Meeren, Sørhus, Sanden, Lunestad, Hannisdal and Olsen. This is an open-access article distributed under the terms of the Creative Commons Attribution License (CC BY). The use, distribution or reproduction in other forums is permitted, provided the original author(s) and the copyright owner(s) are credited and that the original publication in this journal is cited, in accordance with accepted academic practice. No use, distribution or reproduction is permitted which does not comply with these terms.
*Correspondence: Erik Olsen, ZXJpa29AaGkubm8=
Disclaimer: All claims expressed in this article are solely those of the authors and do not necessarily represent those of their affiliated organizations, or those of the publisher, the editors and the reviewers. Any product that may be evaluated in this article or claim that may be made by its manufacturer is not guaranteed or endorsed by the publisher.
Research integrity at Frontiers
Learn more about the work of our research integrity team to safeguard the quality of each article we publish.