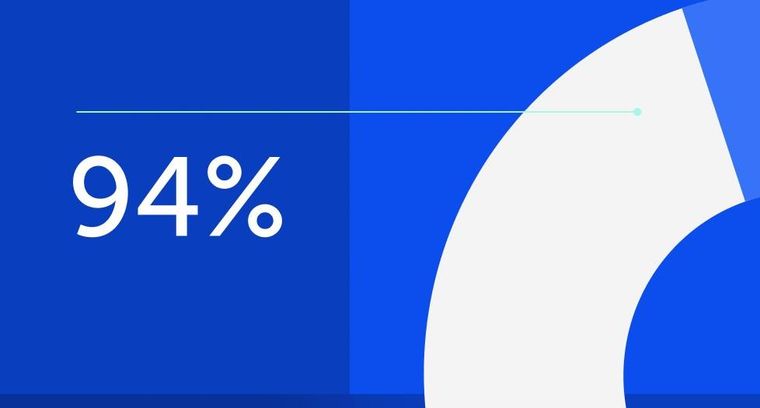
94% of researchers rate our articles as excellent or good
Learn more about the work of our research integrity team to safeguard the quality of each article we publish.
Find out more
ORIGINAL RESEARCH article
Front. Nutr., 10 April 2025
Sec. Nutrition and Metabolism
Volume 12 - 2025 | https://doi.org/10.3389/fnut.2025.1572189
This article is part of the Research TopicPotential Preventive and Therapeutic Effects of Phytochemicals on Metabolic Disorders and Cardiovascular DiseaseView all articles
Background: Metabolic syndrome (MetS) is a global health concern linked to increased mortality. Diets rich in plant-derived compounds, such as polyphenols, have shown potential health benefits for MetS. Among these, flavan-3-ols, a class of commonly occurring polyphenolic compounds, are known for their antioxidant and anti-inflammatory properties. Therefore, we hypothesize that flavan-3-ols intake is negatively associated with mortality risk in MetS population.
Methods: This study analyzed NHANES data (2007–2008, 2009–2010, and 2017–2018). Flavan-3-ol and monomer intake were obtained from the USDA Flavonoid and FNDDS databases. Associations with mortality were assessed using Cox regression, survival differences were compared using Kaplan–Meier curves, and non-linear trends were examined using restricted cubic splines. Subgroup analyses were conducted to explore potential effect modifications.
Results: Over a median follow-up period of 114 months, 1,856 participants survived, while 329 deaths were recorded. In Model 3, participants in the highest tertile (T3) of flavan-3-ol intake exhibited a 33% lower risk of all-cause mortality compared to those in the lowest tertile (T1) (HR = 0.67, 95%CI: 0.49–0.92). For monomers, the hazard ratios ranged from 0.55 for higher levels of epigallocatechin to 0.71 for higher levels of gallocatechin. Kaplan–Meier curves indicated significant differences in survival status across dietary flavan-3-ol intake groups. However, no association was found between flavan-3-ol intake and cardiovascular mortality risk. Additionally, restricted cubic spline (RCS) analysis did not reveal any non-linear relationship, and no significant interaction effects were observed in the subgroup analysis.
Conclusion: Higher dietary intake of flavan-3-ols is negatively associated with mortality risk in MetS population.
Metabolic Syndrome (MetS) is defined by a cluster of metabolic risk factors, including dyslipidemia, hypertension, central obesity, and insulin resistance (1). It has become a major global health concern, affecting approximately 20–25% of the world’s population, with a particularly high prevalence in high-income countries (2). According to the National Health and Nutrition Examination Survey (NHANES, 2011–2016), the prevalence of MetS in the U.S. population is estimated to be 34.7% (3). Additionally, MetS is frequently associated with a state of low-grade systemic inflammation, which further exacerbates insulin resistance and increases mortality risk (4, 5). The global burden of MetS-related mortality continues to rise annually, making it one of the leading causes of death worldwide (6, 7). Current treatment strategies primarily involve pharmacological interventions, including lipid-lowering, glucose-lowering, and antihypertensive medications. However, long-term use of these agents imposes a substantial burden on hepatic and renal function (8–11). As all components of MetS are sensitive to lifestyle changes, it remains the effective and safe strategy for managing MetS and preventing its complications.
To present, a few dietary patterns with anti-inflammatory qualities have made headway in the treatment of MetS (12, 13). In this context, the role of bioactive compounds from diet has been repeatedly emphasized (14). Flavonoids, a class of polyphenolic compounds abundantly found in plants, are well-known for their anti-inflammatory, antioxidant, and antidiabetic properties (15). They contain the following six components: Isoflavones, Anthocyanins, Flavan-3-ols, Flavanones, Flavonoids, and Flavanols. Flavan-3-ols, a major contributor to polyphenol intake, are predominantly found in green tea, cocoa, fruits, and red wine (16, 17). And flavan-3-ols are playing an increasingly important role in the development of dietary recommendations and reference intake values (18–20). They consist of six main monomers: Epicatechin 3-gallate (ECG), Epigallocatechin 3-gallate (EGCG), Epicatechin (EC), Epigallocatechin (EGC), Gallocatechin (GC), and Catechin (C). Recent epidemiological studies have confirmed that flavan-3-ols can help prevent the onset of chronic metabolic diseases and improve metabolic function to some extent (21–23). Extensive in vitro studies have demonstrated that flavan-3-ols and their monomers exhibit anti-inflammatory and antioxidant properties (24, 25). However, while these monomers show promising effects in cell and animal models, excessive intake of monomeric supplements in clinical settings may impose additional hepatic and renal burdens and cause gastrointestinal distress (26, 27). Current public health guidelines emphasize obtaining nutrients from diverse dietary sources rather than relying on isolated supplements (28). Additionally, flavan-3-ols have been associated with improvements in metabolic parameters, including blood pressure, glucose, and lipid levels. However, the relationship between their dietary intake and mortality risk remains inconclusive in survival cohort studies (29–31). The relationship between flavan-3-ols and mortality risk in MetS population is still under investigation.
In this study, we hypothesize that higher dietary intake of flavan-3-ols is negatively associated with mortality risk in individuals with MetS. To test this hypothesis, we utilized data from the USDA Flavonoid Database to estimate flavan-3-ol intake from various foods and beverages. Mortality outcomes were obtained from the National Death Index (NDI) death certificate records. Using the NHANES dataset, we conducted a comprehensive analysis to examine the association.
The National Health and Nutrition Examination Survey (NHANES) is an important program that tracks nutrition and health trends in the U.S. population. All participants provide written consent, and the study undergoes strict ethical oversight (32). To ensure broad demographic representation, it employs a complex, multistage sampling framework. Comprehensive details on its methodology and publicly available datasets can be accessed through the official platform (32). We combined three cycles comprising 29,940 participants (2007–2008, 2009–2010, and 2017–2018). Participants with missing flavan-3-ols, ineligible death records, deaths within 1 year, pregnant women, and those with missing covariates were excluded. The final sample size was 2,185 participants. Figure 1 presents the survey flowchart.
The definition of MetS is based on the National Cholesterol Education Program (NCEP) (33). MetS is diagnosed if three of the following conditions: (1) waist circumference of ≥88 cm (women) and ≥102 cm (men), (2) hypertriglyceridemia (TG ≥150 mg/dL), (3) low high-density lipoprotein cholesterol (HDL < 40 mg/dL in men or < 50 mg/dL in women), (4) systolic blood pressure ≥ 130 mm Hg, diastolic ≥85 mm Hg or previous diagnosis of hypertension, or antihypertensive medication (5) Elevated fasting glucose (FPG) (FPG ≥ 5.6 mmol/L, or diagnosis of type 2 diabetes, or glycosylated hemoglobin >6.5%).
The USDA Flavonoid Database provides comprehensive information on dietary flavonoid values across various foods and beverages. Detailed data can be accessed at https://www.ars.usda.gov/ARSUserFiles/80400535/Data/Flav/Flav3.3.pdf. This database includes the flavonoid content (mg/100 g) of all foods and beverages listed in the USDA Food and Nutrient Database for Dietary Studies (FNDDS), corresponding to applicable the What We Eat in America (WWEIA) survey and NHANES dietary data releases (34). The NHANES dietary data are collected through two non-consecutive 24-h dietary recall interviews. In this study, we included the following flavonoids: Epicatechin 3-gallate (ECG), Epigallocatechin 3-gallate (EGCG), Epicatechin (EC), Epigallocatechin (EGC), Gallocatechin (GC), and Catechin (C).
Mortality data were determined using NHANES participant information matched to National Death Index (NDI) death certificate data dated through December 31, 2019 (35). Cardiovascular mortality is included with death code information for (I00-I09, I11, I13, I20-I51, and I60-I69).
Our analyses considered potential confounders, including age, race, gender, education, household income and poverty rate (PIR), BMI, depression, alcohol intake, smoking history, the Healthy Eating Index-2020 (HEI-2020) (36), history of cancer, and history of cardiovascular disease. Educational attainment was divided as </=/> High school. Race was categorized into five groups, including Mexican American, non-Hispanic Black and White, other Hispanic, or other race. PIR, an index measuring household socioeconomic status, was grouped as <1.5, 1.5–3.5, or >3.5. Depression was assessed using the Patient Health Questionnaire-9 (PHQ-9), a widely used screening tool for depressive symptoms, with a score of ≥10 indicating depression (37). Alcohol consumption history was categorized as never, moderate (<=2 drinks/day), and moderately heavy (>2 drinks/day). Smoking history was categorized as not smoking/quitting smoking and ongoing smoking. Cancer history was categorized as yes or no based on the patient’s questionnaire response “Have you ever been told by a doctor about any type of cancer.” Cardiovascular history included a history of stroke, angina, coronary heart disease, heart attack, and congestive heart failure as collected by the questionnaire. HEI-2020 assessed the diet of the U.S. population in 13 different areas (36): total vegetables, vegetables and legumes, total fruits, whole fruits, whole grains, seafood and plant proteins, dairy products, total protein foods, saturated fats, added sugars, fatty acids, refined grains, and sodium. The HEI-2020 is scored on a scale of 0–100, with higher scores reflecting healthier dietary patterns.
Dietary flavan-3-ols intake were stratified into tertiles, while individual monomers were categorized into tertiles or dichotomized based on their distributional characteristics to ensure robust and accurate analysis. To reduce outcome bias, death data within the first year of follow-up were excluded. Given the complex sampling design of the NHANES study, all analyses incorporated sample weights to maintain representativeness and validity.
Continuous data were presented as the mean (standard deviation), while binary variables were reported as unweighted frequencies (weighted frequencies). Between-group differences were assessed using the chi-square test for binary variables and the Wilcoxon rank-sum test for continuous variables. Kaplan–Meier curves were generated to evaluate survival across different levels of flavan-3-ols intake. The association between flavan-3-ols intake and mortality in MetS population was analyzed using a multivariate Cox regression model. To explore potential non-linear relationships, restricted cubic spline (RCS) regression was employed. Additionally, the analysis was stratified by age, sex, BMI, income, cancer history, and cardiovascular disease history, with interaction tests conducted to evaluate the presence of subgroup dependencies. All analyses were performed using R software version 4.2.3.
The study included 2,185 participants with MetS, representing approximately 27 million Americans. Over a median follow-up of 114 months, 1,856 participants survived, while 329 deaths were recorded. The mean age of the cohort was 54.2 years, with males comprising 49% of the population. The average BMI was 32.8 kg/m2. Notably, significant differences were observed between groups for C, EGC, EC, ECG, EGCG, and GC. Participants who died during the study period were generally older, had lower education levels, lower income, lower BMI, and lower alcohol consumption, and exhibited a higher prevalence of cancer and cardiovascular disease. Detailed cohort characteristics are presented in Table 1, while the distribution of flavan-3-ols and their monomers is summarized in Supplementary Tables 1, 2. Supplementary Table 3 presents liver and kidney conditions across different flavan-3-ols intake groups, showing that the T3 group had lower ALT and AST levels, but no significant differences in liver diseases (fatty liver/viral hepatitis), serum creatinine, or chronic kidney disease (CKD) prevalence. US-FLI was used to assess fatty liver (38), and eGFR was calculated based on the CKD Epidemiology Collaboration (CKD-EPI) equation (39). Additionally, Supplementary Figure 1 illustrates the Pearson correlation coefficients among the major monomers.
Table 2 presents the results of the Cox regression analysis. Supplementary Table 4 displays the VIF values for variables in Model 3, with all VIF values below 5, indicating no significant multicollinearity. In Model 3, the T3 group of flavan-3-ols demonstrated a 33% reduction in the risk of all-cause Mortality compared to the T1 group (HR = 0.67, 95% CI: 0.49–0.92, P for trend = 0.01). Similarly, the T3 group of C exhibited a 43% lower risk of mortality compared to the T1 group (HR = 0.57, 95% CI: 0.39–0.84), and the T3 group of EGC showed a 45% reduction in mortality risk compared to the T1 group (HR = 0.55, 95% CI: 0.38–0.81).
For ECG, participants in Group 2 (>0) had a 34% lower risk of mortality compared to Group 1 (=0) (HR = 0.66, 95% CI: 0.48–0.89). Similarly, Group 2 (>0) of EGCG was associated with a 30% reduction in mortality risk compared to Group 1 (=0) (HR = 0.70, 95% CI: 0.54–0.90), and Group 2 (>0) of GC had a 29% lower risk of mortality compared to Group 1 (=0) (HR = 0.71, 95% CI: 0.54–0.95). However, no significant differences in survival status were observed for EC.
Table 3 presents the results of the Cox regression analysis. In Model 1, participants in the T3 group of C had a 50% lower risk of mortality compared to those in the T1 group (HR = 0.50, 95% CI: 0.27–0.96). In Model 2, Group 2 of ECG showed a 40% lower risk of mortality compared to Group 1 (HR = 0.60, 95% CI: 0.41–0.88). However, in Model 3, no significant associations were observed between flavan-3-ols or monomers and cardiovascular mortality.
As shown in Figure 2, KM survival curves were plotted for different flavan-3-ol or monomers, including flavan-3-ols, C, EGC, EC, ECG, EGCG, and GC. For all-cause Mortality, the results indicated that the survival rate of the T3 group was significantly higher than that of the T1 group for flavan-3-ols, C, EGC, and EC (log-rank test p < 0.05). Additionally, the survival rate of Group 2 (>0) was higher than that of Group 1 (=0) for ECG, EGCG, and GC. The relationship between flavan-3-ols and their monomers with Cardiovascular Mortality risk is further illustrated in Supplementary Figure 2. However, no significant differences in cardiovascular mortality were observed across different dietary intake groups of flavan-3-ols.
Figure 2. Kaplan–Meier survival analysis of flavan-3-ols intake and all-cause mortality. (A) Flavan-3-ols and all-cause mortality; (B) C and all-cause mortality; (C) EGC and all-cause mortality; (D) EC and all-cause mortality; (E) ECG and all-cause mortality; (F) EGCG and all-cause mortality; (G) GC and all-cause mortality.
To further explore the potential dose–response relationship between flavan-3-ols and all-cause Mortality, we employed restricted cubic spline (RCS) analysis. As illustrated in Figure 3, the statistical tests did not reveal any significant non-linear association (p > 0.05). This could imply that the protective effect of flavan-3-ols against all-cause Mortality may be dose-dependent, with higher levels of intake potentially contributing to a reduced mortality risk.
Figure 3. Dose–response relationship between flavan-3-ols and all-cause mortality. (A) Flavan-3-ols; (B) C; (C) EGC; (D) EC; (E) ECG; (F) EGCG; (G) GC.
Table 4 presents the results of the subgroup analysis on flavan-3-ols and all-cause mortality. The T3 group of flavan-3-ols was negatively associated with all-cause mortality in MetS individuals aged >60 years (HR = 0.65, 95% CI: 0.44–0.98), in the female MetS population (HR = 0.63, 95% CI: 0.42–0.92), and at the low-income level (HR = 0.63, 95% CI: 0.42–0.94). Supplementary Table 5 presents the association between flavan-3-ols and cardiovascular mortality across different subgroups. However, no significant interactions were found between flavan-3-ols and these subgroups in all interaction tests (p > 0.05 for interaction). Notably, no significant differences in prognosis were observed across different flavan-3-ols intake levels among individuals with varying liver and kidney conditions (p > 0.05 for interaction).
To address potential bias introduced by the discontinuity in the time series, we conducted a sensitivity analysis by excluding the 2017–2018 data and reanalyzing the dataset. The results in Supplementary Table 6 and Supplementary Figure 3 remained consistent with our primary findings, reinforcing the robustness of our conclusions.
In this study, we extracted data from the FNDDS and NHANES databases to investigate the relationship between flavan-3-ols intake and mortality risk, which included 2,185 MetS population. Our findings reveal that higher intake of flavan-3-ols are negatively associated with all-cause Mortality risk, and this protective effect may be a dose-dependent relationship. This negative correlation was consistent across most flavan-3-ol monomers. KM analysis further showed that individuals with higher flavan-3-ols intake had a significantly better survival rate compared to those with lower intake. However, no significant association was found between flavan-3-ol intake and the risk of cardiovascular mortality.
It is well known that the main sources of flavan-3-ols include red wine, green tea, cocoa, and fruits. Mei Chung and colleagues synthesized 39 prospective cohorts and found that an increase of one cup (236.6 mL) of tea (the main source of flavan-3-ol monomers) per day was related to a 1.5% lower risk of all-cause mortality and a 4% lower cardiovascular risk (40). A Nurses’ Health Study II (NHS II) also demonstrated that the consumption of red wine, tea, and fruits is inversely associated with the risk of all-cause mortality (29). Bondonno et al. (41) found that the protective effect of flavan-3-ols were not significant in the general population. However, they found a 25% reduction in risk of all-cause mortality in the highest tertile of flavan-3-ols compared with the lower tertile in an established subgroup of “high-risk individuals” (smokers, alcoholics, obese) (41). Our findings further support this observation, showing that even after adjusting for potential confounders such as alcohol consumption, smoking, and BMI, individuals in the T3 group of flavan-3-ol intake exhibited a 33% lower risk of all-cause mortality compared to those in the T1 group for MetS population. Notably, individuals with unhealthy habits or metabolic disorders often experience elevated levels of oxidative stress and inflammation, which predispose them to higher mortality risk. Given the potent antioxidant and anti-inflammatory properties of flavan-3-ols, their increased intake may provide substantial benefits to these at-risk populations. These results underscore the potential role of flavan-3-ols as a valuable dietary component for reducing mortality risk and improving health outcomes, particularly in metabolically compromised individuals.
To our knowledge, this is the first long-term survival cohort study about dietary flavan-3-ols in a MetS population. This study demonstrated that the protective effect of flavan-3-ols were significant in a high-risk population. Pro-inflammatory factors, including tumor necrosis factor-alpha (TNF-α), leptin, and interleukin-6 (IL-6), along with elevated levels of free fatty acids and adipokines, were markedly increased in individuals with MetS. These factors contributed to a heightened inflammatory state and oxidative stress, which elevated the risk of mortality (4, 5). In addition, macrophage polarization, thrombosis, monocyte adhesion, decreased nitric oxide utilization, vascular remodeling and stiffness, and oxidative stress levels have all been shown to play important roles in pathological mechanisms in metabolically disturbed populations (42–46). Flavan-3-ols can provide electrons to stop ROS production and chelate metal ions associated with free radical production (47). It also upregulate the activities of various ROS-scavenging enzymes, including glutathione peroxidase (GSH), superoxide dismutase (SOD), and catalase (CAT) (24, 25). They significantly reduced the secretion of inflammatory cytokines, including TNF-α, IL-6, and interleukin-1 beta (IL-1β), suppressing the activation of nuclear factor kappa B (NF-κB) in vivo and in vitro. Additionally, they exerted antioxidant effects through the activation of the nuclear factor erythroid 2-related factor 2 (Nrf2) pathway (48, 49).
Various monomers have also been extensively shown to improve the internal environment of MetS. EGCG has been widely shown to have preventive effects on various metabolic diseases (50). It modulates lipid absorption, increases glucose uptake and significantly improves symptoms of MetS. Supplementation with C in rats attenuated the expression of adipose inflammation (51). GC was shown to down-regulate 11 pro-inflammatory genes, including S100a8 and IL-1β, and up-regulate the expression of Gstm3 antioxidant genes in mouse experiments (52). Our analysis supplements the understanding of the long-term benefits of dietary flavan-3-ol monomers intake for MetS. In addition, we also found higher dietary intake including EGC, ECG, and EC were also protective against long-term survival states. It is important that although most monomers contribute positively to metabolic function, their strong correlation through dietary intake makes it difficult to pinpoint any single monomer as having a predominant effect.
Although some studies have emphasized the protective effects of antioxidant supplementation in mitigating the cardiovascular disease pathology in MetS population (53), the United States Pharmacopeia has issued warnings regarding the potential hepatotoxicity and gastrointestinal side effects of common monomeric extracts such as C, EGC, EC, ECG, EGCG, and GC (54). These adverse effects exhibit considerable individual variability and are closely linked to extraction methods and storage conditions. In contrast, dietary intake offers a safer alternative, avoiding the potential toxicity and risks associated with isolated supplements while fulfilling daily nutritional requirements (28). Recent dietary guidelines on flavan-3-ols confirm that an intake of 400–600 mg/day can improve heart metabolism, including blood pressure, blood glucose, and lipid levels (18). Our study further supports the benefits of increased flavan-3-ols consumption through diet for MetS population. The dose–response relationship in this study showed a linear relationship, suggesting that the risk of all-cause mortality may be negatively associated with flavan-3-ols dietary intake in the MetS population. Nevertheless, the clinical significance of flavan-3-ols intake requires further investigation, as individual metabolic differences and dietary variations may influence its protective effects. Future research should aim to determine an optimal intake threshold, providing a foundation for more precise dietary guidelines tailored to the MetS population. Establishing such recommendations could help maximize flavan-3-ols’potential in improving long-term health outcomes.
The program has several additional strengths. We included patient data from 3 cycles and considered corresponding weights to represent the broader US population. In addition, we adjusted for multiple confounding variables and factors and designed a rigorous analytic process to make the results more reliable.
However, this study has some limitations. Dietary intake of flavan-3-ols was collected in a baseline data sheet, and dietary habits fluctuated during long-term follow-up. Additionally, 24-h dietary recall, although widely used in large-scale epidemiological studies, may introduce recall bias and measurement errors. Furthermore, there may be some variation in the dietary composition of different populations, making our results of limited representativeness.
Although we adjusted for several confounding variables, we could not exclude all confounding factors. In addition, for all observational studies, we were unable to derive causal effects. The MetS population is at a higher risk of cardiovascular events, yet our study did not identify a significant association between flavan-3-ol intake and cardiovascular mortality risk. This may be attributable to sample size limitations, underscoring the need for further large-scale studies to explore this relationship in greater depth. Moreover, our study primarily focused on the association between flavan-3-ol intake and mortality, without an in-depth investigation into the underlying biological mechanisms. Future research also should incorporate relevant metabolic and inflammatory biomarkers to explore their potential mediating effects on this association.
In this large-scale U.S. population study, higher dietary intake of flavan-3-ols is negatively associated with mortality risk in MetS population. We believe that further research into the optimal dietary flavan-3-ols intake could provide significant benefits in reducing mortality risk.
Publicly available datasets were analyzed in this study. This data can be found at: NHANES (https://www.cdc.gov/nchs/nhanes/index.htm).
The studies involving humans were approved by National Center for Health Statistics (NCHS) Research Ethics Review Board, which is part of the Centers for Disease Control and Prevention (CDC), United States. The studies were conducted in accordance with the local legislation and institutional requirements. The participants provided their written informed consent to participate in this study.
WZ: Conceptualization, Formal analysis, Writing – original draft. QZ: Data curation, Investigation, Writing – original draft. WY: Conceptualization, Software, Visualization, Writing – original draft. XT: Conceptualization, Validation, Writing – original draft. YX: Conceptualization, Funding acquisition, Supervision, Writing – review & editing. ZY: Conceptualization, Validation, Visualization, Writing – review & editing.
The author(s) declare that financial support was received for the research and/or publication of this article. This work was supported by the Hunan Provincial Natural Science Foundation Science and Health Joint Project Grant Number [2023JJ60044]; Hunan Province Traditional Chinese Medicine Research Program Project Grant Number [B2023079]; Hunan Province Clinical Medical Technology Innovation Guidance Project Grant Number [2021SK51413]; Excellent Youth Project of the Research Program of Hunan Provincial Department of Education Grant Number [21B0389]; Scientific Research Project of Education Department of Hunan Province [24A0274]; and the Domestic First-class Construction Discipline of Chinese Medicine in Hunan University of Chinese Medicine.
Thanks to all who contributed to the NHANES study.
The authors declare that the research was conducted in the absence of any commercial or financial relationships that could be construed as a potential conflict of interest.
The authors declare that no Gen AI was used in the creation of this manuscript.
All claims expressed in this article are solely those of the authors and do not necessarily represent those of their affiliated organizations, or those of the publisher, the editors and the reviewers. Any product that may be evaluated in this article, or claim that may be made by its manufacturer, is not guaranteed or endorsed by the publisher.
The Supplementary material for this article can be found online at: https://www.frontiersin.org/articles/10.3389/fnut.2025.1572189/full#supplementary-material
MetS, Metabolic syndrome; ECG, Epicatechin 3-gallate; EGCG, Epigallocatechin 3-gallate; EC, Epicatechin; EGC, Epigallocatechin; GC, Gallocatechin; C, Catechin; NHANES, National Health and Nutrition Examination Survey; USDA, United States Department of Agriculture; FNDDS, Food and Nutrient Database for Dietary Studies; WWEIA, What We Eat in America survey; RCS, Restricted cubic spline; HEI-2020, Healthy Eating Index-2020; PHQ-9, Patient Health Questionnaire-9; TNF-α, Tumor necrosis factor-alpha; IL-6, Interleukin-6; IL-1β, Interleukin-1 beta; NF-κB, Nuclear factor kappa B; Nrf2, Nuclear factor erythroid 2-related factor 2.
1. Eckel, RH, Grundy, SM, and Zimmet, PZ. The metabolic syndrome. Lancet. (2005) 365:1415–28. doi: 10.1016/S0140-6736(05)66378-7
2. Adjei, NK, Samkange-Zeeb, F, Boakye, D, Saleem, M, Christianson, L, Kebede, MM, et al. Ethnic differences in metabolic syndrome in high-income countries: a systematic review and meta-analysis. Rev Endocr Metab Disord. (2024) 25:727–50. doi: 10.1007/s11154-024-09879-9
3. Li, M, Jiang, S, Dong, C, and Jiang, D. Association between fat-soluble vitamins and metabolic syndromes in US adults: a cross-section study from NHANES database. BMC Endocr Disord. (2024) 24:178. doi: 10.1186/s12902-024-01711-4
4. Lin, Z, and Sun, L. Research advances in the therapy of metabolic syndrome. Front Pharmacol. (2024) 15:1364881. doi: 10.3389/fphar.2024.1364881
5. Koh, KK, Han, SH, and Quon, MJ. Inflammatory markers and the metabolic syndrome: insights from therapeutic interventions. J Am Coll Cardiol. (2005) 46:1978–85. doi: 10.1016/j.jacc.2005.06.082
6. Saklayen, MG. The global epidemic of the metabolic syndrome. Curr Hypertens Rep. (2018) 20:12. doi: 10.1007/s11906-018-0812-z
7. Li, W, Qiu, X, Ma, H, and Geng, Q. Incidence and long-term specific mortality trends of metabolic syndrome in the United States. Front Endocrinol. (2023) 13:1029736. doi: 10.3389/fendo.2022.1029736
8. Orchard, TJ, Temprosa, M, Goldberg, R, Haffner, S, Ratner, R, Marcovina, S, et al. The effect of metformin and intensive lifestyle intervention on the metabolic syndrome: the diabetes prevention program randomized trial. Ann Intern Med. (2005) 142:611–9. doi: 10.7326/0003-4819-142-8-200504190-00009
9. Katsimardou, A, Imprialos, K, Stavropoulos, K, Sachinidis, A, Doumas, M, and Athyros, V. Hypertension in metabolic syndrome: novel insights. Curr Hypertens Rev. (2020) 16:12–8. doi: 10.2174/1573402115666190415161813
10. Kraja, AT, Province, MA, Straka, RJ, Ordovas, JM, Borecki, IB, and Arnett, DK. Fenofibrate and metabolic syndrome. Endocr Metab Immune Disord Drug Targets. (2010) 10:138–48. doi: 10.2174/187153010791213047
11. Grundy, SM. Drug therapy of the metabolic syndrome: minimizing the emerging crisis in polypharmacy. Nat Rev Drug Discov. (2006) 5:295–309. doi: 10.1038/nrd2005
12. Farhadnejad, H, Parastouei, K, Rostami, H, Mirmiran, P, and Azizi, F. Dietary and lifestyle inflammatory scores are associated with increased risk of metabolic syndrome in Iranian adults. Diabetol Metab Syndr. (2021) 13:30. doi: 10.1186/s13098-021-00648-1
13. Finicelli, M, Squillaro, T, Di Cristo, F, Di Salle, A, Melone, MAB, Galderisi, U, et al. Metabolic syndrome, Mediterranean diet, and polyphenols: evidence and perspectives. J Cell Physiol. (2019) 234:5807–26. doi: 10.1002/jcp.27506
14. Poulsen, NB, Lambert, MNT, and Jeppesen, PB. The effect of plant derived bioactive compounds on inflammation: a systematic review and Meta-analysis. Mol Nutr Food Res. (2020) 64:e2000473. doi: 10.1002/mnfr.202000473
15. Shen, N, Wang, T, Gan, Q, Liu, S, Wang, L, and Jin, B. Plant flavonoids: classification, distribution, biosynthesis, and antioxidant activity. Food Chem. (2022) 383:132531. doi: 10.1016/j.foodchem.2022.132531
16. Sebastian, RS, Wilkinson Enns, C, Goldman, JD, Martin, CL, Steinfeldt, LC, Murayi, T, et al. A new database facilitates characterization of flavonoid intake, sources, and positive associations with diet quality among US Adults12. J Nutr. (2015) 145:1239–48. doi: 10.3945/jn.115.213025
17. Bai, W, Wang, C, and Ren, C. Intakes of total and individual flavonoids by US adults. Int J Food Sci Nutr. (2014) 65:9–20. doi: 10.3109/09637486.2013.832170
18. Crowe-White, KM, Evans, LW, Kuhnle, GGC, Milenkovic, D, Stote, K, Wallace, T, et al. Flavan-3-ols and Cardiometabolic health: first ever dietary bioactive guideline. Adv Nutr. (2022) 13:2070–83. doi: 10.1093/advances/nmac105
19. Kuhnle, GGC. Nutrition epidemiology of flavan-3-ols: the known unknowns. Mol Asp Med. (2018) 61:2–11. doi: 10.1016/j.mam.2017.10.003
20. Lupton, JR, Atkinson, SA, Chang, N, Fraga, CG, Levy, J, Messina, M, et al. Exploring the benefits and challenges of establishing a DRI-like process for bioactives. Eur J Nutr. (2014) 53 Suppl 1:1–9. doi: 10.1007/s00394-014-0666-3
21. Zhang, S, Xu, M, Zhang, W, Liu, C, and Chen, S. Natural polyphenols in metabolic syndrome: protective mechanisms and clinical applications. Int J Mol Sci. (2021) 22:6110. doi: 10.3390/ijms22116110
22. Hejazi, J, Hosseinpour-Niazi, S, Yuzbashian, E, Mirmiran, P, and Azizi, F. The protective effects of dietary intake of flavonoids and its subclasses on metabolic syndrome incidence. Int J Food Sci Nutr. (2022) 73:116–26. doi: 10.1080/09637486.2021.1928008
23. Raman, G, Avendano, EE, Chen, S, Wang, J, Matson, J, Gayer, B, et al. Dietary intakes of flavan-3-ols and cardiometabolic health: systematic review and meta-analysis of randomized trials and prospective cohort studies. Am J Clin Nutr. (2019) 110:1067–78. doi: 10.1093/ajcn/nqz178
24. Rodríguez-Ramiro, I, Martín, MA, Ramos, S, Bravo, L, and Goya, L. Comparative effects of dietary flavanols on antioxidant defences and their response to oxidant-induced stress on Caco2 cells. Eur J Nutr. (2011) 50:313–22. doi: 10.1007/s00394-010-0139-2
25. Meng, Q, Velalar, CN, and Ruan, R. Effects of epigallocatechin-3-gallate on mitochondrial integrity and antioxidative enzyme activity in the aging process of human fibroblast. Free Radic Biol Med. (2008) 44:1032–41. doi: 10.1016/j.freeradbiomed.2007.11.023
26. Navarro, V, Khan, I, Björnsson, E, Seeff, LB, Serrano, J, and Hoofnagle, JH. Liver injury from herbal and dietary supplements. Hepatology. (2017) 65:363–73. doi: 10.1002/hep.28813
27. EFSA Panel on Food Additives and Nutrient Sources added to Food (ANS)Younes, M, Aggett, P, Aguilar, F, Crebelli, R, Dusemund, B, et al. Scientific opinion on the safety of green tea catechins. EFSA J. (2018) 16:e05239. doi: 10.2903/j.efsa.2018.5239
28. Lichtenstein, AH, and Russell, RM. Essential nutrients: food or supplements? Where should the emphasis be? JAMA. (2005) 294:351–8. doi: 10.1001/jama.294.3.351
29. Ivey, KL, Jensen, MK, Hodgson, JM, Eliassen, AH, Cassidy, A, and Rimm, EB. Association of flavonoid-rich foods and flavonoids with risk of all-cause mortality. Br J Nutr. (2017) 117:1470–7. doi: 10.1017/S0007114517001325
30. Hejazi, J, Ghanavati, M, Hejazi, E, Poustchi, H, Sepanlou, SG, Khoshnia, M, et al. Habitual dietary intake of flavonoids and all-cause and cause-specific mortality: Golestan cohort study. Nutr J. (2020) 19:108. doi: 10.1186/s12937-020-00627-8
31. Ponzo, V, Goitre, I, Fadda, M, Gambino, R, De Francesco, A, Soldati, L, et al. Dietary flavonoid intake and cardiovascular risk: a population-based cohort study. J Transl Med. (2015) 13:218. doi: 10.1186/s12967-015-0573-2
32. NHANES – National Health and nutrition examination survey homepage. Available online at: https://www.cdc.gov/nchs/nhanes/index.htm (accessed August 5, 2024)
33. Expert Panel on Detection, Evaluation, and Treatment of High Blood Cholesterol in Adults. Executive summary of the third report of the National Cholesterol Education Program (NCEP) expert panel on detection, evaluation, and treatment of high blood cholesterol in adults (adult treatment panel III). JAMA. (2001) 285:2486–97. doi: 10.1001/jama.285.19.2486
34. FNDDS Flavonoid database: USDA ARS. Available online at: https://www.ars.usda.gov/northeast-area/beltsville-md-bhnrc/beltsville-human-nutrition-research-center/food-surveys-research-group/docs/fndds-flavonoid-database/ (accessed January 3, 2025)
35. NCHS Data linkage – mortality data. (2024). Available online at: https://www.cdc.gov/nchs/data-linkage/mortality.htm (accessed August 5, 2024)
36. Shams-White, MM, Pannucci, TE, Lerman, JL, Herrick, KA, Zimmer, M, Meyers Mathieu, K, et al. Healthy eating Index-2020: review and update process to reflect the dietary guidelines for Americans, 2020-2025. J Acad Nutr Diet. (2023) 123:1280–8. doi: 10.1016/j.jand.2023.05.015
37. Liu, X, Liu, X, Wang, Y, Zeng, B, Zhu, B, and Dai, F. Association between depression and oxidative balance score: National Health and nutrition examination survey (NHANES) 2005-2018. J Affect Disord. (2023) 337:57–65. doi: 10.1016/j.jad.2023.05.071
38. Pan, J, Zhou, Y, Pang, N, and Yang, L. Dietary niacin intake and mortality among individuals with nonalcoholic fatty liver disease. JAMA Netw Open. (2024) 7:e2354277. doi: 10.1001/jamanetworkopen.2023.54277
39. Inker, LA, Eneanya, ND, Coresh, J, Tighiouart, H, Wang, D, Sang, Y, et al. New creatinine- and cystatin C-based equations to estimate GFR without race. N Engl J Med. (2021) 385:1737–49. doi: 10.1056/NEJMoa2102953
40. Chung, M, Zhao, N, Wang, D, Shams-White, M, Karlsen, M, Cassidy, A, et al. Dose-response relation between tea consumption and risk of cardiovascular disease and all-cause mortality: a systematic review and meta-analysis of population-based studies. Adv Nutr. (2020) 11:790–814. doi: 10.1093/advances/nmaa010
41. Bondonno, NP, Lewis, JR, Blekkenhorst, LC, Bondonno, CP, Shin, JH, Croft, KD, et al. Association of flavonoids and flavonoid-rich foods with all-cause mortality: the Blue Mountains eye study. Clin Nutr. (2020) 39:141–50. doi: 10.1016/j.clnu.2019.01.004
42. Wang, J, Wu, Q, Wang, X, Liu, H, Chen, M, Xu, L, et al. Targeting macrophage phenotypes and metabolism as novel therapeutic approaches in atherosclerosis and related cardiovascular diseases. Curr Atheroscler Rep. (2024) 26:573–88. doi: 10.1007/s11883-024-01229-z
43. Slate-Romano, JJ, Yano, N, and Zhao, TC. Irisin reduces inflammatory signaling pathways in inflammation-mediated metabolic syndrome. Mol Cell Endocrinol. (2022) 552:111676. doi: 10.1016/j.mce.2022.111676
44. Alessi, M-C, and Juhan-Vague, I. Metabolic syndrome, haemostasis and thrombosis. Thromb Haemost. (2008) 99:995–1000. doi: 10.1160/TH07-11-0682
45. Ferreira, I, Beijers, HJ, Schouten, F, Smulders, YM, Twisk, JW, and Stehouwer, CD. Clustering of metabolic syndrome traits is associated with maladaptive carotid remodeling and stiffening: a 6-year longitudinal study. Hypertension. (2012) 60:542–9. doi: 10.1161/HYPERTENSIONAHA.112.194738
46. Saladini, F, and Palatini, P. Arterial distensibility, physical activity, and the metabolic syndrome. Curr Hypertens Rep. (2018) 20:39. doi: 10.1007/s11906-018-0837-3
47. Godos, J, Romano, GL, Laudani, S, Gozzo, L, Guerrera, I, Dominguez Azpíroz, I, et al. Flavan-3-ols and vascular health: clinical evidence and mechanisms of action. Nutrients. (2024) 16:2471. doi: 10.3390/nu16152471
48. Fraga, CG, Oteiza, PI, and Galleano, M. Plant bioactives and redox signaling: (−)-Epicatechin as a paradigm. Mol Asp Med. (2018) 61:31–40. doi: 10.1016/j.mam.2018.01.007
49. Zhao, D, Shen, S, Guo, Y, Wang, Y, Gu, C, Guo, B, et al. Flavan-3-ol monomers intake is associated with osteoarthritis risk in Americans over 40 years of age: results from the National Health and nutritional examination survey database. Food Funct. (2024) 15:6966–74. doi: 10.1039/d3fo04687g
50. Sudathip, S, Grove, KA, and Lambert, JD. Laboratory studies on weight control and prevention of metabolic syndrome by green tea. Pharmacol Res. (2011) 64:146–54. doi: 10.1016/j.phrs.2010.12.013
51. Vazquez Prieto, MA, Bettaieb, A, Rodriguez Lanzi, C, Soto, VC, Perdicaro, DJ, Galmarini, CR, et al. Catechin and quercetin attenuate adipose inflammation in fructose-fed rats and 3T3-L1 adipocytes. Mol Nutr Food Res. (2015) 59:622–33. doi: 10.1002/mnfr.201400631
52. Xiao, X, Ge, H, Wang, Y, Wan, X, Li, D, and Xie, Z. (−)-Gallocatechin Gallate mitigates metabolic syndrome-associated diabetic nephropathy in db/db mice. Food Secur. (2024) 13:1755. doi: 10.3390/foods13111755
53. Sharebiani, H, Mokaram, M, Mirghani, M, Fazeli, B, and Stanek, A. The effects of antioxidant supplementation on the pathologic mechanisms of metabolic syndrome and cardiovascular disease development. Nutrients. (2024) 16:1641. doi: 10.3390/nu16111641
Keywords: flavan-3-ols, metabolic syndrome, mortality, NHANES, cohort study, flavan-3-ol monomers
Citation: Zhang W, Zhou Q, Yang W, Tan X, Xu Y and Yi Z (2025) Relationship between dietary flavan-3-ols intake and mortality in metabolic syndrome population; a large cohort study. Front. Nutr. 12:1572189. doi: 10.3389/fnut.2025.1572189
Received: 06 February 2025; Accepted: 27 March 2025;
Published: 10 April 2025.
Edited by:
Dong Zheng, The First Affiliated Hospital of Soochow University, ChinaReviewed by:
Matteo Della Porta, University of Milan, ItalyCopyright © 2025 Zhang, Zhou, Yang, Tan, Xu and Yi. This is an open-access article distributed under the terms of the Creative Commons Attribution License (CC BY). The use, distribution or reproduction in other forums is permitted, provided the original author(s) and the copyright owner(s) are credited and that the original publication in this journal is cited, in accordance with accepted academic practice. No use, distribution or reproduction is permitted which does not comply with these terms.
*Correspondence: Yin Xu, MzExMTE4QGhudWNtLmVkdS5jbg==; Zhan Yi, MDAzOTgzQGhudWNtLmVkdS5jbg==
Disclaimer: All claims expressed in this article are solely those of the authors and do not necessarily represent those of their affiliated organizations, or those of the publisher, the editors and the reviewers. Any product that may be evaluated in this article or claim that may be made by its manufacturer is not guaranteed or endorsed by the publisher.
Research integrity at Frontiers
Learn more about the work of our research integrity team to safeguard the quality of each article we publish.