- Department of Pediatrics, Child and Adolescent Psychiatric Center of Jiangbei Campus, The First Affiliated Hospital of Army Medical University (Army 958th Hospital), Chongqing, China
Attention deficit hyperactivity disorder (ADHD) is a common neurodevelopmental disorder, which is characterized by inattention, impulsivity and hyperactivity. Although the etiology and pathogenesis of ADHD are not fully understood, existing studies have shown that it may be related to genetic factors, environmental factors, abnormal brain development, and psychosocial factors. In recent years, with the concept of microbioa-gut-brain axis (MGBA), more and more studies have begun to pay attention to the effect of gut microbiota on ADHD. Dietary structure can significantly change the diversity and abundance of gut microbiota. Therefore, dietary supplements or food additives to regulate gut microbiota have become one of the potential ways to treat ADHD. Peppers, as an important dietary component, have potential value in regulating gut microbiota. Among them, capsaicin (8-methyl N-vanillyl-6-noneamide, CAP), as a key active component of peppers, has been shown to have potential therapeutic effects on central nervous system (CNS) diseases such as Parkinson’s disease, epilepsy, and depression. In addition, much attention has been paid to the beneficial effects of CAP on gut microbiota. Chili peppers contain not only CAP, but also rich in vitamin C and fatty acids, all of which may ameliorate ADHD by modulating the gut microbiota. This finding not only provides a potential treatment for ADHD, but also provides a new perspective to expand the research and clinical treatment of ADHD pathogenesis. Although current research on the potential therapeutic effects of chili peppers on ADHD is still at an early stage and requires further verification through larger-scale and more rigorous controlled studies, its potential clinical value cannot be ignored.
1 Introduction
Attention deficit hyperactivity disorder (ADHD) is one of the most common neurodevelopmental disorders in children and adolescents, which is characterized by excessive activity, attention deficit and impulsive behavior. ADHD seriously affects patients’ academic performance, interpersonal relationships, and reduces their quality of life. Studies show that about 30–50% of children continue to show symptoms into adulthood (1). The etiology of ADHD is multifactorial and includes genetic predisposition, environmental influences, abnormal brain development, and psychosocial factors. Currently, methylphenidate and atomoxetine are the most commonly used drugs, but these drugs are often accompanied by adverse reactions, such as nausea, vomiting, loss of appetite, mood instability and sleep difficulty. In recent years, a large number of studies have shown a bidirectional interaction between the gut and the brain, a mechanism known as the microbiome-gut-brain axis (MGBA) (2). The gut microbiota is a key player in the gut-brain axis (2), and its composition and function can influence the onset and progression of ADHD (3). Diet is one of the important factors shaping the composition and metabolic activity of the gut microbiota (4). Therefore, interventions through diet may have a positive impact on the management of ADHD. Researchers have begun to pay attention to the potential role of dietary intervention and gut microbiota in the treatment of ADHD.
Studies have demonstrated the association between diet and ADHD, and a balanced diet or nutritional supplements may be a potential method to control ADHD. As a common dietary ingredient, chili peppers are rich in vitamin C, capsaicin (8-methyl N-vanillyl-6-noneamide, CAP), fatty acids, and other active ingredients, which have anti-inflammatory, anti-oxidative, anti-cancer, and adjusting gut microbiota (5–9). Recent studies have shown that CAP has beneficial effects on central nervous system (CNS) diseases such as Parkinson’s disease, epilepsy, stroke, and depression by reducing oxidative stress and improving neuroinflammation (10–14). Vitamin C has neuroprotective effects and also serves as an adjunct in the redox chain reaction required for neurotransmitter synthesis. This has proven beneficial for brain diseases such as stroke and epilepsy (15). In addition, disorders of fatty acid metabolism are closely related to the pathogenesis of neuropsychiatric disorders (16). Next, this study will explore the relationship between diet, gut microbiota, and ADHD, and analyze the effects of peppers and their components on ADHD through the gut microbiota and brain-gut axis. This provides a theoretical basis for further human clinical studies to verify the potential benefits of long-term consumption of peppers on ADHD.
2 Diet can affect the gut microbiota
Gut microbiota refers to the entire microbial ecosystem that inhabits the gastrointestinal tract of mammals, including bacteria, fungi, viruses, and protozoa (17). This complex and dynamic system plays a critical role in human health and disease. The initial colonization of microbiota begins at birth, and the mode of delivery, feeding pattern, dietary structure, and environmental factors all have significant effects on the formation of early gut microbiota (18). During the early stages of life, the gut microbiota rapidly diversifies and plays a key role in the development of the immune system (19, 20). Into adulthood, although the microbiota remains generally stable, its composition and function can still be affected by external factors, among which diet is one of the most important factors (21, 22).
Diet can alter the composition and diversity of the gut microbiota through MGBA, which in turn affects the interactions between the brain, gut, and microbiota, which has important implications for brain health and a variety of neurological diseases (23). Healthy diet (rich in dietary fiber and multiple sources of viable bacteria) is capable of promoting not only an increase in gut microbial diversity but also the production of short-chain fatty acids (SCFAs) and other beneficial bioactive compounds that contribute to gastrointestinal health, metabolic health, and brain function (24, 25). In contrast, western diet dominated by processed foods leads to unhealthy changes in the gut microbiota and is associated with disorders such as psychiatric disorders, gastrointestinal disorders, metabolic disorders (26), and obesity (27). A prospective randomized controlled trial in healthy individuals showed that diet rich in fermented foods was able to enhance the diversity of the microbiota and significantly reduce the levels of proinflammatory cytokines such as serum interleukin (IL-6) (28). High-fiber diet significantly alters the composition of the gut microbiota, leading to a large increase in the number of beneficial bacteria such as Lactobacillus and Bifidobacterium (29). Two studies have established an association between the Mediterranean diet and specific microbial communities, finding increased populations of beneficial bacteria such as Faecalibacterium prausnitzii and Roseburia spp. It also reduces the abundance of Collinsella aerofaciens, and Ruminococcus (30, 31). Diet-induced changes in the gut microbiota are associated with increased SCFAs and decreased metabolic by-products such as ethanol and carbon dioxide. The influence of diet is present throughout the life span, and modulation of MGBA is a promising strategy for the prevention and treatment of mental health disorders.
3 The association between the gut microbiota and ADHD
Numerous studies have demonstrated that gut microbiota plays a crucial role in diseases like ADHD, Alzheimer’s disease (AD), autism, epilepsy, etc. (3, 32, 33). The gut microbiota can regulate the levels of neurotransmitters, affect the function of the hypothalamic–pituitary–adrenal (HPA) axis, and alter the permeability of the blood–brain barrier. These mechanisms constitute the key links of the brain-gut axis in the pathophysiological process of ADHD. The gut microbiota of ADHD patients was transferred to mice by researchers and found that the structural integrity of white matter and gray matter regions (such as the internal capsule and hippocampus) in the mice was lower, and there was a significant correlation between the structural integrity of white matter and the differentially expressed microbial community (34). Tengeler et al. (34) demonstrated that the transfer of gut microbiota from individuals diagnosed with ADHD to mice could induce behavioral modifications, which were not evident when utilizing gut microbiota from individuals without ADHD. Individuals with ADHD and healthy controls have different gut microbiota compositions (35, 36). The Lactobacillus family has been shown to be consistently lower in those with ADHD than in their healthy counterparts (3). Prehn-Kristensen et al. (37) have proved that the α diversity of the gut microbiota in adolescent ADHD patients was lower, and the hyperactivity symptom score was negatively correlated with α diversity. Researchers (38) conducted a study on the gut microbiota while sequencing 16S rRNA genes from the V4 region, and demonstrated that children and adolescents diagnosed with ADHD have different gut microbiota signatures from unrelated controls.
4 Beneficial effects of diet on the gut microbiota in ADHD
Dietary therapy has significant positive effects on a variety of neurological disorders, including depression, Parkinson’s disease, autism, ADHD, and epilepsy (39–42). The Mediterranean diet has long been regarded as a positive contributor to health. Studies have shown that intervention with a Mediterranean diet in children with ADHD significantly improves impulsiveness and hyperactivity in 8 weeks (43). Liu et al. (44) used mice as an animal model to study the effect of ketogenic diet (KD) on ADHD, and found that compared with the normal diet group, the KD group had a significant increase in the abundance of Ruminococcus_gauvreauii_group, Bacteroides, Bifidobacterium, and Blautia. However, the abundance of Lactobacillus, Romboutsia, Facklamia, and Turicibacter was significantly reduced in the KD group. According to research, KD can help alleviate behavioral disorders associated with ADHD by modulating the gut microbiota. One study evaluated the effect of the elimination diet on ADHD symptoms in children and showed that the elimination diet resulted in significant improvements in ADHD symptoms and the effect lasted longer (45). In addition, dietary deficiency of essential nutrients affects changes in synapse number and myelin integrity, thereby affecting neurodevelopmental processes, including the formation of hippocampal learning and memory pathways and the maturation of executive functions, ultimately leading to negative consequences associated with ADHD (46). A systematic review of randomized clinical trials has shown that iron and zinc deficiencies are associated with the onset of ADHD, which can be ameliorated by dietary interventions (47). These studies provide evidence that ADHD can be improved by dietary interventions, which opens up the possibility of future clinical treatment of ADHD. The microbiome and its metabolites are influenced by changes in diet and host physiological function. Therefore, different dietary structures may contribute to changes in children with ADHD by regulating the gut microbiota. The microbiome and its metabolites are influenced by changes in diet and host physiological function. Therefore, different dietary structures may contribute to changes in children with ADHD by adjusting the gut microbiota.
5 Chili peppers may be beneficial for ADHD
As a widely used vegetable and spice, peppers are rich in bioactive compounds, such as CAP, vitamin C, and fatty acids (24, 25). Since the 20th century, the production and consumption of chili peppers have gradually increased worldwide, making them an indispensable ingredient in numerous dishes (48, 49). Recent studies have shown that the active components in chili peppers exhibit a variety of biological properties in vitro and in vivo experiments, such as lipid-reducing, antioxidant, and anti-inflammatory effects (50–52). However, current research on the relationship between peppers and ADHD is still in its preliminary stage. Our study aimed to investigate the effects of chili peppers and its main components on ADHD. The prevalence of ADHD in China is lower than the global average, which may be related to dietary habits, cultural differences and economic development level. In China, especially Yunnan, Guizhou and Sichuan, the proportion of people who like spicy food is high, and the trend is steadily growing. Major components in chili peppers, such as CAP, vitamin C, and unsaturated fatty acids, have been shown to have the potential to modulate the gut microbiota. Based on the MGBA theory, a bidirectional interaction between the gut and the brain, we considered that chili peppers might also improve ADHD symptoms by modulating the gut microbiota.
5.1 CAP may ameliorate ADHD through the brain-gut axis
CAP is a vanillic acid derivative found mainly in the genus Capsicum and is a key contributor to its pungent taste. The mechanism of CAP activity covers both receptor-dependent and non-receptor-dependent pathways, but its protective effect against neurodegeneration is mainly through transient receptor potential vanilloid 1 (TRPV1). In the human CNS, TRPV1 is mainly found in the cerebral cortex, striatum and hippocampus. CAP can cross the blood–brain barrier and directly act on the nervous system via TRPV1 (53), participating in the neural pathways of the brain-gut axis. CAP also affects brain neurons and glial cells through TRPV1-dependent and independent mechanisms and plays a role in regulating autophagy, synaptic transmission and plasticity, and cognitive function (54, 55). CAP primarily reduces oxidative stress, alleviates pain and cognitive impairment, protects against cardiovascular disease, stroke, obesity, hypertension, and cancer via TRPV1 (56, 57). In addition, intestines are richly innervated by afferent sensory nerves expressing TRPV1 channels, and their activation plays an important role in the gut function regulation (58–60). CAP can stimulate TRPV1 receptors and induces local release of neuropeptides such as Substance P or gene-related peptide (58–61). These neuropeptides may regulate the composition and structure of the gut microbiota by altering the inflammatory and immune environment of the gut (62).
There has been an increasing amount of evidence suggesting that CAP can affect the composition, abundance, and function of the gut microbiota. It reduced hippocampal cell damage and improved brain tissue metabolism by enhancing the function of the gut-brain axis (63). Studies have shown that CAP increases the ratio of Firmicutes/Bacteroidetes bacteria and the number of Clostridium bacteria, which leads to an increase in peripheral 5-hydroxytryptamine (5-HT; serotonin) levels through microbial transfer (64). Mahalak et al. (5) found that adding CAP to an in vitro culture model of the human gut microbiota increased microbial diversity and increased the abundance of certain SCFAs, particularly butyric acid. But the study was conducted in vitro and did not consider the influence of host functions on the study results. In two separate in vivo studies conducted on mice, the inclusion of dietary CAP led to a decrease in weight gain and food consumption. Additionally, it enhanced the abundance of important intestinal microbial genera like Bacteroidetes, Akkermansia, and Prevotella, while simultaneously lowering the levels of Escherichia and Salmonella genera (65, 66). Ryu et al. (63) explored how Korean chili paste (kochujang) containing an appropriate amount of CAP could alleviate memory impairment in rats through the enteric-brain axis, and they found that compared to the control group, kochujang (Medium-CPS) intake decreased Clostridium and Solibaculum and increased Blautia and Lachnoclostridium belonging to the family Lachinospraceae compared to the Control group. These changes affected the metagenome function related to brain function and inflammation. The consumption of red peppers in kochujang has been shown to improve inflammation in rats with dextran sulfate sodium-induced colitis, modulating gut microbiota by decreasing harmful Enterococcus faecalis and Staphylococcus sciuri (67). An animal study has shown that CAP can alleviate Lipopolysaccharid (LPS) -induced depressive-like behavior and reduce TNF-α levels by enhancing the relative abundance of certain gut microbiota, such as Ruminococcus and Prevotella (14). CAP can increase beneficial bacteria such as Firmicutes and reduce harmful bacteria such as Enterococcus faecalis and Staphylococcus sciuri by changing the composition and abundance of intestinal flora. This can increase neurotransmitters (5-HT, DA), metabolites (SCFAs), etc. This affects the various pathways of the brain-gut axis and acts as an anti-inflammatory and neuroprotective agent to improve ADHD. But more molecular studies are needed to elucidate the underlying subtle mechanisms of gut-capsaicin interactions.
CAP has been shown to have potential neuroprotective properties, including improving cognitive function and preventing neurodegenerative diseases (67–70). The beneficial effects of CAP in Parkinson’s disease and depression have been described (71). CAP is also capable of enhancing the neural function of stroke patients (71). A study of the diet and cognitive status of 338 middle-aged and older adults shows that long-term intake of CAP can effectively improve cognitive performance in AD patients. This suggests that CAP could be a potential drug option for prevention and treatment of AD (72). In a crossover randomized controlled trial, patients with amyotrophic lateral sclerosis (ALS) experienced significant relief of ALS-related dysphagia after the administration of CAP (73). These researches suggest that CAP, as a common food additive, may have potential benefits for neurological diseases. It is currently believed that an imbalance in the gut microbiota may be associated with the onset of ADHD (35, 36). We believe CAP may be an emerging potential treatment for ADHD patients.
5.2 Beneficial effects of vitamin C on gut microbiota
The initial response of many individuals to chili peppers is that they are hot, but beyond this characteristic, they also hold a remarkable title - they are the top source of vitamin C among vegetables. The amount of vitamin C in different types of chili peppers varies. The amount of vitamin C found in different peppers genotypes varies between 43 and 247 mg for every 100 g of fresh fruit (74). The highest is sweet peppers, whose vitamin C content is four times that of oranges and 43 times that of apples. Vitamin C is a very important antioxidant and free radical scavenger that can promote iron ion absorption (75), participate in collagen synthesis, hormone synthesis, and carnitine synthesis (76). Additionally, it plays an important role in the function and regulation of the immune system (77). The health benefits of vitamin C have been widely proven, and the strong correlation between the gut microbiota and host health or various diseases has been widely reported, so exploring the known regulation of vitamin C on the gut microbiota is crucial.
Research indicates that after a two-week regimen of high-dose vitamin C supplementation, there is a notable rise in the relative abundance of the bacterial groups Lachnospiraceae (other) among healthy individuals. In contrast, both the Bacteroidetes phylum and Enterococcus genus exhibit a significant reduction (78). The bacterial groups Lachnospiraceae belongs to the Firmicutes phylum, and is a major bacterial group in the gut microbiota of healthy human subjects (79, 80). Lachnospiraceae is one of the main producers of SCFAs. These are known bacterial metabolites with multiple anti-inflammatory and antioxidant effects (81). Another study used a mouse model of rheumatoid arthritis to validate the regulatory role of vitamin C in modulating gut microbiota balance, which improved the alpha and beta diversity of the bacterial community, especially increasing the abundance of the genus Lachnospira and increasing the production of SCFAs (82). Vitamin C reconfigures the gut microbiota, improves the intestinal environment, reduces inflammatory responses and oxidative stress in the paraventricular nucleus of the hypothalamus, thereby weakening sympathetic nervous activity in hypertensive rats and lowering blood pressure (83).
Vitamin C increases the abundance of potential ADHD-related probiotics in the gut, such as Lachnospiraceae, and boosts the production of SCFAs (79, 84). This increases the number of related metabolites and neurotransmitters, which affect the brain-gut axis and thus play a beneficial role in ADHD. Additionally, higher intakes of vitamin C can enhance collagen synthesis within the intestinal tract, which strengthens the intestinal barrier and indirectly impacts gastrointestinal health and the microbiome (79, 80). Furthermore, vitamin C is known to have a protective effect against inflammation, oxidative stress, and certain neurochemical factors and neurotransmitters, which are implicated in the pathophysiology of ADHD (85, 86). This evidence suggests that by modulating the gut microbiota, vitamin C may exert a positive influence on ADHD, a condition often associated with oxidative stress and neuroinflammations (81–83, 87, 88).
5.3 Beneficial effects of omega-3 polyunsaturated fatty acids on gut microbiota
Chili peppers also contain Polyunsaturated Fatty Acids (PUFAs) (87). These PUFAs are in the whole fruit and seeds, but their seeds show even greater concentration per gram (88). The main types of PUFAs include omega-3 PUFAs and omega-6 PUFAs, which are considered very important for human health. Unsaturated fatty acids cannot be produced by the human body and must be obtained from food (86). It has been reported that Omega-3 PUFAs deficiency exists in people with various mental disorders, including ADHD, depression, and Autism Spectrum Disorder (ASD) (89). A randomized controlled trial found that supplementing with omega-3 PUFAs mixtures increased the concentrations of EPA and DHA in red blood cell membranes and improved memory function in children with ADHD (90). In male animal models with ADHD, a diet rich in omega-3 PUFAs promotes increased dopamine (DA) and 5-HT turnover in the striatum, which enhances attention and reduces impulsivity (91). Children afflicted with ADHD present significantly decreased total omega-3 PUFAs in contrast to the control group, and the omega-6:omega-3 fatty acid ratio is conspicuously higher in children with ADHD compared to the control group (92). Therefore, correcting the omega-3: omega-6 imbalance can improve ADHD symptoms by stabilizing neuronal membranes, dopaminergic neurotransmission, and reducing neuroinflammation.
The earlier conversation highlighted how the gut microbiota influences ADHD. Growing research suggests that omega-3 PUFAs may influence the gut microbiota and its byproducts, contributing to the improvement of gut dysbiosis (93–96). Omega-3 PUFAs can directly affect the gut microbiota, while the gut microbiota can directly or indirectly regulate the absorption, bioavailability, and bioconversion of omega-3 PUFAs (97). Omega-3 PUFAs derived from food consumption, undergo partial metabolism by anaerobic bacteria like Bifidobacteria and Lactobacilli in the distal intestine, consequently affecting the composition of the gut microbiota (98). Studies involving randomized trials on omega-3 PUFAs supplementation in humans have demonstrated that such supplementation leads to a temporary rise in the populations of various bacteria responsible for producing SCFAs (99). There have been demonstrated that the addition of omega-3 PUFAs to the diet can raise the abundance and percentage of Bifidobacterium in the intestines of male Sprague–Dawley rats (97). A study evaluated the cognitive ability and social behavior of mice fed high and low doses of omega-3 fatty acids (DHA + EPA), and their microbial composition was also sequenced using 16S rDNA. The results showed that those who ate a diet rich in omega-3 fatty acids showed stronger cognitive function and had higher levels of lactic acid bacteria and bifidobacteria (100).
Although there have been no clinical reports on the use of chili peppers in the treatment of ADHD, its active compounds (CAP, vitamin C, omega-3 PUFAs) have been shown to affect the gut microbiota and its metabolites (see Table 1). This interaction can provide a protective effect on the intestinal barrier, regulate the immune response and the production of neurotransmitters. It also provides anti-inflammatory and antioxidant properties. Peppers are a safe and relatively cheap compound that is commonly consumed in various countries. We suggest that regular addition of peppers to meals, such as bell peppers that can be eaten directly, may help improve symptoms in children with ADHD (Figure 1).
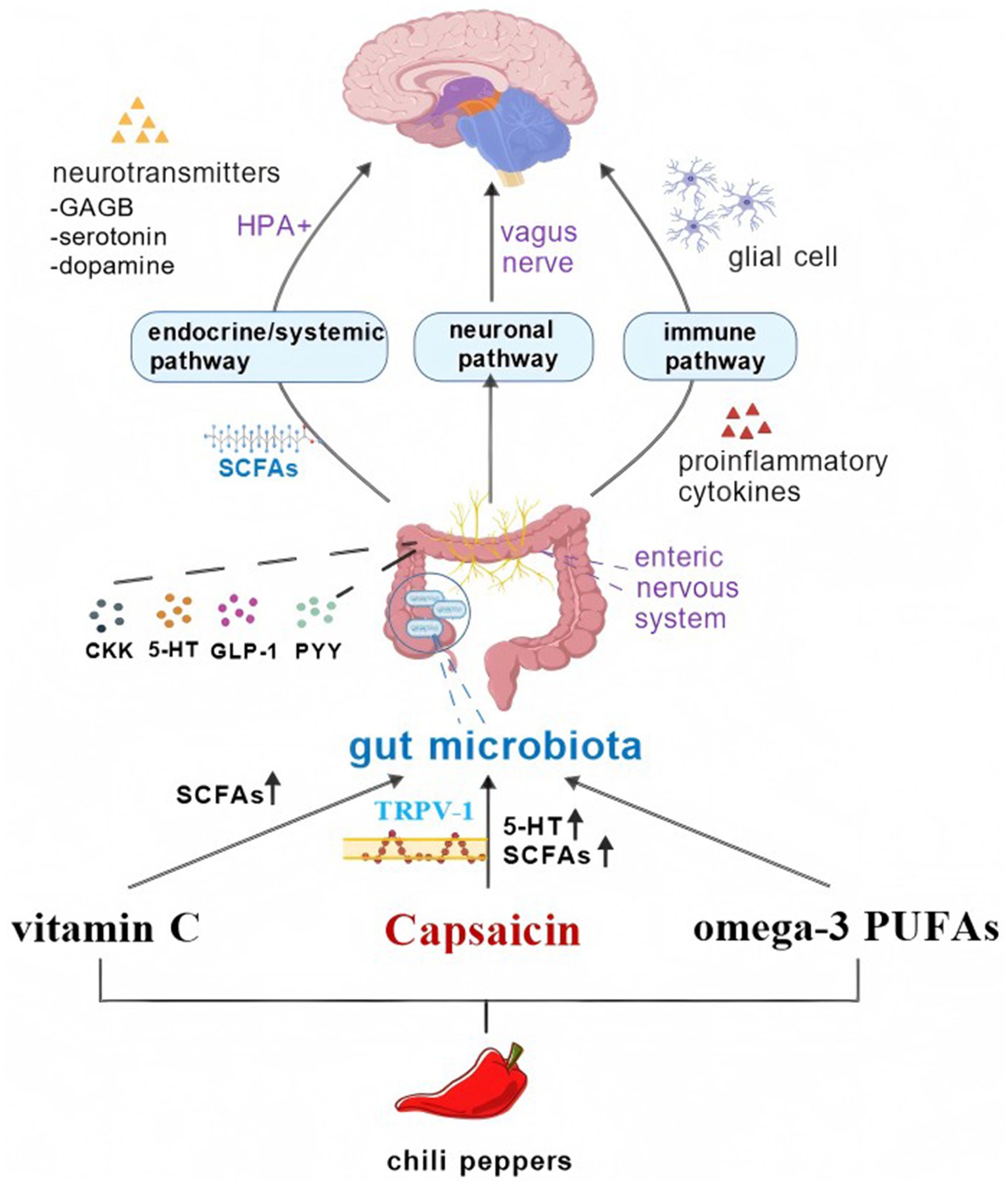
Figure 1. The active ingredient of chili peppers can affect the gut microbiota, thereby altering ADHD through the brain-gut axis. Figure created with BioGDP.com, licensed under CC BY-NC 4.0 (127).
5.4 Chili peppers regulate gut microbiota to improve ADHD pathway
The exact mechanism of ADHD onset is still not fully understood. At present, it is clearly proposed that the abnormal reduction of DA level and the involvement and dysfunction of the corresponding brain region triggered by nervous system inflammation may be the main cause of ADHD (101). Previous studies have found that the abundance of bacteria associated with the production of neurotransmitters (such as GABA and DA) and pathogenic and opportunistic bacteria (such as Enterococcus, Fusobacterium, and Eggerthella) increased in the gut microbiota of children with ADHD, while the number of bacteria that play an anti-inflammatory role (such as Bacillus faecalis) decreased (101). Therefore, improving the gut microbiota or supplementation with probiotics is expected to alleviate ADHD symptoms by increasing the production of neurotransmitters in the gut, reducing intestinal inflammation, and reducing the abundance of intestinal pathogens. As mentioned above, various components of chili can act on the gut microbiota, so ADHD patients can benefit from it. The gut microbiota was commonly believed to control neurodevelopment through three pathways: the immune pathway, the neuronal pathway, and the endocrine/systemic pathway, with connections and interactions among them.
5.4.1 Neural pathways
There are two neuroanatomical pathways that enable communication between the gut and the brain. First, direct interactions take place via the Vagus Nerve (VNS) and the autonomic nervous system (ANS) located in the spinal cord. VNS, which is the ninth cranial nerve, innervates the digestive tract by the hepatic and celiac branches. Siopi et al. (102) found that healthy mice receiving gut microbiota from chronic unpredictable mild stress (CUMS) mice showed vagus-mediated alterations in their 5-HT and DA pathways, which are associated with concurrent and persistent deficits in hippocampal neurogenesis and neuroinflammation. This suggests that the VNS can indeed mediate the effects of gut microbiota on brain function and behavior. In animal models, infections with pathogens such as Campylobacter jejuni and Citrobacter Amalonac induce anxiety-like behaviors (103), and supplementation with probiotics including Lactobacillus rhamnosus and Bifidobacterium longum alleviates these anxious/depression-like behaviors (104, 105). The gut microbiota has the ability to regulate host emotional and behavioral responses by acting on the vagus afferent nerve. Second, the enteric nervous system (ENS) of the gastrointestinal tract promotes bilateral interactions among these systems alongside ANS and VNS activity (106). The ENS, consisting of the myenteric and submucosal plexuses, communicates with the CNS via afferent neurons to transmit sensory information. This information travels through the spinal cord and vagal pathways, coordinating intestinal functions such as peristalsis and fluid flow (24). gut microbiota can also affect the maturation and function of ENS by activating pattern recognition receptors (PRRs), such as Toll-like receptors (TLRs) and 5-HT receptors (107).
In addition, the gut microbiota may influence the production of neurotransmitters. For example, certain Clostridium perfringens regulate the synthesis of 5-HT by expressing tryptophan hydroxylase 1 (TPH1) (108). The gut microbiota also influences DA levels in the frontal cortex and striatum of rodents, and perturbations of the dopaminergic system are associated with mood-related disorders and cognitive dysfunction. Besides, gut microbiota can regulate the expression of brain-derived neurotrophic factor (BDNF), which is closely related to neurogenesis, thereby affecting cognition and attention (109).
5.4.2 Endocrine/systemic pathways
Gut microbiota play a crucial role in the development and function of the HPA axis, a key regulatory system for neuroendocrine signaling and stress responses (110). The gut microbiota is capable of generating diverse metabolites, releasing neurotransmitters and neuromodulators, thereby influencing the HPA axis and exerting an effect in the pathogenesis of ADHD. When germ-free mice were exposed to constraint stress, they exhibited a stronger stress response compared to normal mice, with significant increases in adrenocorticoid and glucocorticoid levels. Adding Bifidobacterium can reduce the intensity of stress response in germ-free mice, suggesting that the gut microbiota plays a role in regulating the HPA axis (111). Studies have shown that the gut microbiota not only directly affects the production of glucocorticoids and immune mediators, such as tumor necrosis factor-α (TNF-α), interleukin-1β (IL-1β), and IL-6, but also further stimulates HPA axis activity through these mediators (112). The substances or metabolites mentioned encompass γ-aminobutyric acid (GABA), 5-HT, DA, norepinephrine, acetylcholine, histamine, SCFAs, and others (113, 114). It has been confirmed that bacilli and Bifidobacteria can produce GABA, Escherichia coli, Bacillus, and yeast can produce norepinephrine, Candida albicans, Streptococcus, Escherichia coli, and Enterococci can produce 5-HT, and Bacillus can also produce DA (115). However, metabolites such as 5-HT are unable to cross the blood–brain barrier without the involvement of VNS. VNS acts as a significant link between the endocrine/systemic pathway and the neuronal pathway. Furthermore, the gut microbiota interacts with the enteric endocrine system to regulate neurodevelopment. Enteric endocrine cells can produce glucagon-like peptide-1 (GLP-1), peptide YY (PYY), cholecystokinin (CCK), substance P, and 5-HT, which are involved in metabolic functions related to energy homeostasis (116). At the same time, enteric endocrine cells can sense various microbial signals and can also connect with VNS to communicate with the CNS. Gut microbiota is also capable of producing SCFAs, particularly butyrate, propionate, and acetate, which combat oxidative stress in inflammatory states by upregulating the activity of antioxidant enzymes such as glutathione peroxidase (GPX) and catalase (117).
5.4.3 Immune pathways
The gut microbiota plays a key role in regulating the differentiation and maturation of innate immune cells (such as macrophages, innate lymphocytes and dendritic cells), and is essential for the formation and integrity of the intestinal barrier and blood–brain barrier. Studies have shown that changes in the gut microbiota down-regulate the expression of tight junction (TJs) proteins, leading to impaired intestinal barrier and blood–brain barrier function, allowing biomacromolecules and microorganisms to penetrate the barrier, which in turn triggers neuroinflammatory responses (118). In addition, the gut microbiota was found to influence the process of monocyte migration from the periphery to the brain mediated by TNF-α, which was reversed by the use of probiotics (119). The development and maturation of the acquired immune system is also dependent on the presence of the gut microbiota. For example, intraepithelial lymphocytes require L. reuteri as well as a tryptophan-rich diet to reprogram intraepithelial CD4+T cells into immunomodulatory T cells (120). At the same time, intestinal microbiota dysbiosis leads to decreased levels of immunoglobulin A and G1 (IgA and IgG1) and increased levels of immunoglobulin E (IgE), thereby increasing the risk of developing a variety of diseases (121). SCFAs also have immune functions. They can reduce damage to the intestinal epithelial tissue, regulate tight junctions, and enhance the intestinal immune barrier. Other functions of SCFAs include regulating the production of cytokines by bone marrow cells, as well as differentiating T regulatory cells and promoting the differentiation of helper T cells (122). In addition to regulating the intestinal barrier, the gut microbiota is also involved in the transport of immune cells from the intestine to the brain. For example, the gut microbiota activates a group of IFNγ + NK cells, which then migrate to the CNS and induce the production of anti-inflammatory astrocytes.
It can be seen from this that gut microbiota can regulate brain immune responses and cells in this way, and changes in the composition of gut microbiota can lead to dysregulation of the MGBA, ultimately leading to ADHD. Chili peppers are an indispensable part of dietary structure, which contain CAP, vitamin C, and fatty acids that can alter the composition and function of the gut microbiota and affect the generation of fatty acids. These changes are transmitted through the brain-gut axis and subsequently affect ADHD.
6 Conclusion
Due to the long-term negative effects and side effects of medications and behavioral treatments, the search for effective treatments for ADHD has been ongoing in recent years. The gut microbiota maintains an interactive relationship with the host through neural, endocrine, and immune pathways, which is known as MGBA. Changes in MGBA are a factor in the development of neurodevelopmental disorders in children. Children with ADHD exhibit neuroinflammation and oxidative stress, which are considered part of its pathophysiological mechanisms. The MGBA may play a certain role in this process. According to MGBA theory, the effect of diet on the gut microbiota may be a promising therapeutic approach for children with ADHD. Peppers, as a common and inexpensive spice, are widely used around the world. Peppers not only are a unique flavor to food but also are rich in various beneficial components, such as CAP, vitamin C, and fatty acids. They can alter the composition of the gut microbiota and the secretion of metabolites, exert anti-inflammatory and immunomodulatory effects, and thus potentially have a positive impact on ADHD through the MGBA. Using small amounts of chili peppers in the diet as an adjunct to treating ADHD has fewer side effects and is more acceptable to parents compared to traditional medication. This review describes the effects of gut microbiota and dietary factors on ADHD, while exploring the potential therapeutic advantages of chili peppers in modulating gut microbiota through MGBA in children with ADHD. This potential mechanism and these effects are derived based on the aforementioned research and related theories; however, at present, there is still a lack of large-scale clinical research evidence to fully support this. This conclusion helps us further clarify future research directions, such as large-scale, randomized, double-blind, placebo-controlled human clinical trials, to validate the benefits and risks of chili peppers and CAP consumption for neurological disorders, especially ADHD.
CAP is widely used around the world, but the debate over its safety continues. A large number of studies have confirmed that CAP has anti-tumor, anti-inflammatory and analgesic effects (123). Contrary opinions suggest that long-term use of high doses of CAP may increase the risk of developing tumors (124, 125). Significantly increased consumption of chili peppers or CAP has the potential to increase the risk of gastric cancer, however, inconsistencies still exist in subgroup analysis between different regions (126). On the contrary, one study suggests that repeated ingestion of CAP over a prolonged period reduces symptoms in functional dyspepsia (128). The long-term safety of regular chili consumption has not yet been established, and further research is needed on the safety and potential chronic effects of long-term chili and CAP intake. Exploring the minimum effective dose required to achieve therapeutic effect and minimizing potential side effects is necessary to enhance the clinical effectiveness of capsaicin-based therapies. Chili peppers can also irritate human skin, and excessive intake can cause nausea, vomiting, abdominal pain and burning, diarrhea. Contact of CAP with eyes can cause severe tearing, pain, conjunctivitis, eyelid spasms. But combining CAP with other phytochemicals can reduce these adverse effects, such as vanillin, flavonoids, alkaloids, cannabinoids (129–131). However, there may be individual differences in tolerance to chili peppers, depending on existing health status or genetic susceptibility, etc. In the future, we will explore the factors that influence an individual’s response to chili peppers and CAP to develop personalized dietary guidelines.
There’s no doubt that the health effects of chili peppers are mostly positive. CAP, in particular, has potential benefits in treating ADHD. However, most of the available evidence comes from animal or in vitro studies, and these findings have not been consistently replicated in human clinical trials. When extrapolating the results of animal experiments to humans, the differences between animals and humans should be considered. In the future, more experimental studies and high-quality large-sample clinical trials are still needed to explore the effects of spicy components on mental disorder diseases through the microbiota-brain-gut axis. Most of the studies had short intervention periods, which does not shed light on the long-term effects of chili consumption on ADHD symptoms. The composition of gut microbiota is affected by race, region, diet, and other factors, and there are direct and significant differences among different individuals. Future studies need to analyze the characteristics of gut microbiota in different populations in more detail, and combine multi-dimensional data (such as genomics, metabolomics, etc.) to reveal the specific mechanisms of gut microbiota in mental disorders. Furthermore, for patients of different ages and races, the appropriate intake of chili peppers needs to be tested in future studies to explore the minimum effective dose required to achieve therapeutic effects and minimize potential side effects. In addition, the above-mentioned pungent components have the characteristics of low bioavailability and high liver and intestinal circulation intervals; therefore, looking for more efficient derivatives or complexes and choosing convenient and fast administration routes will bring new breakthrough drugs.
In the later stage, we will further improve the clinical and basic research to clarify the impact and mechanism of peppers on ADHD. Future studies should cover populations of different ages, genders, and regions to fully assess the range of safe doses of chili peppers in different populations and their ongoing effects on ADHD symptoms. This will provide a scientific basis for personalized treatment and promote the clinical application of Capsicum as an adjunct therapy. We should also consider that different types of chili peppers may produce different effects due to their intensity and active ingredient content. We also need to validate pharmacokinetic/safety studies of CAP in patients with psychiatric disorders such as ADHD. We can also improve the pharmacological properties of the compound by modifying the chemical structure of CAP, overcome some adverse reactions and increase the acceptability of peppers.
Author contributions
YL: Investigation, Visualization, Writing – original draft, Writing – review & editing. JF: Investigation, Writing – review & editing, Visualization, Writing – original draft. GD: Investigation, Writing – review & editing. LD: Investigation, Writing – review & editing. YH: Investigation, Writing – review & editing. QZ: Investigation, Writing – review & editing. JW: Writing – review & editing, Investigation. XC: Conceptualization, Project administration, Writing – review & editing.
Funding
The author(s) declare that financial support was received for the research, authorship, and/or publication of this article. This work was supported by the Chongqing Natural Science Foundation Project (No. CSTB2024NSCQ-MSX0619) and the Chongqing Science and Health Joint Project (No. 2025QNXM055) and Army 958 Hospital innovation talent fund (2024YGKT027).
Conflict of interest
The authors declare that the research was conducted in the absence of any commercial or financial relationships that could be construed as a potential conflict of interest.
Generative AI statement
The authors declare that no Generative AI was used in the creation of this manuscript.
Publisher’s note
All claims expressed in this article are solely those of the authors and do not necessarily represent those of their affiliated organizations, or those of the publisher, the editors and the reviewers. Any product that may be evaluated in this article, or claim that may be made by its manufacturer, is not guaranteed or endorsed by the publisher.
References
1. Wolraich, ML, Hagan, JF, Allan, C, Chan, E, Davison, D, Earls, M, et al. Clinical practice guideline for the diagnosis, evaluation, and treatment of attention-deficit/hyperactivity disorder in children and adolescents. Pediatrics. (2019) 144:e20192528. doi: 10.1542/peds.2019-2528
2. Wang, Q, Yang, Q, and Liu, X. The microbiota-gut-brain axis and neurodevelopmental disorders. Protein Cell. (2023) 14:762–75. doi: 10.1093/procel/pwad026
3. Wang, LJ, Yang, CY, Chou, WJ, Lee, MJ, Chou, MC, Kuo, HC, et al. Gut microbiota and dietary patterns in children with attention-deficit/hyperactivity disorder. Eur Child Adolesc Psychiatry. (2020) 29:287–97. doi: 10.1007/s00787-019-01352-2
4. Borrego-Ruiz, A, and Borrego, JJ. Human gut microbiome, diet, and mental disorders. Int Microbiol Off J Span Soc Microbiol. (2024) 27:1–15. doi: 10.1007/s10123-024-00518-6
5. Mahalak, KK, Bobokalonov, J, Firrman, J, Williams, R, Evans, B, Fanelli, B, et al. Analysis of the ability of capsaicin to modulate the human gut microbiota in vitro. Nutrients. (2022) 14:1283. doi: 10.3390/nu14061283
6. Jayapala, HPS, and Lim, SY. N-3 polyunsaturated fatty acids and gut microbiota. Comb Chem High Throughput Screen. (2023) 26:892–905. doi: 10.2174/1386207325666220701121025
7. Yang, Z, Zhou, DD, Huang, SY, Fang, AP, Li, HB, and Zhu, HL. Effects and mechanisms of natural products on Alzheimer’s disease. Crit Rev Food Sci Nutr. (2023) 63:3168–88. doi: 10.1080/10408398.2021.1985428
8. Zheng, J, Zhou, Y, Li, Y, Xu, DP, Li, S, and Li, HB. Spices for prevention and treatment of cancers. Nutrients. (2016) 8:495. doi: 10.3390/nu8080495
9. Azlan, A, Sultana, S, Huei, CS, and Razman, MR. Antioxidant, anti-obesity, nutritional and other beneficial effects of different chili pepper: a review. Molecules. (2022) 27:898. doi: 10.3390/molecules27030898
10. Pasierski, M, and Szulczyk, B. Capsaicin inhibits sodium currents and epileptiform activity in prefrontal cortex pyramidal neurons. Neurochem Int. (2020) 135:104709. doi: 10.1016/j.neuint.2020.104709
11. Wang, C, Huang, W, Lu, J, Chen, H, and Yu, Z. TRPV1-mediated microglial autophagy attenuates Alzheimer’s disease-associated pathology and cognitive decline. Front Pharmacol. (2021) 12:763866. doi: 10.3389/fphar.2021.763866
12. Baek, JY, Jeong, JY, Kim, KI, Won, SY, Chung, YC, Nam, JH, et al. Inhibition of microglia-derived oxidative stress by ciliary neurotrophic factor protects dopamine neurons in vivo from MPP+ neurotoxicity. Int J Mol Sci. (2018) 19:3543. doi: 10.3390/ijms19113543
13. Jittiwat, J, Suksamrarn, A, Tocharus, C, and Tocharus, J. Dihydrocapsaicin effectively mitigates cerebral ischemia-induced pathological changes in vivo, partly via antioxidant and anti-apoptotic pathways. Life Sci. (2021) 283:119842. doi: 10.1016/j.lfs.2021.119842
14. Xia, J, Gu, L, Guo, Y, Feng, H, Chen, S, Jurat, J, et al. Gut microbiota mediates the preventive effects of dietary capsaicin against depression-like behavior induced by lipopolysaccharide in mice. Front Cell Infect Microbiol. (2021) 11:627608. doi: 10.3389/fcimb.2021.627608
15. Ballaz, SJ, and Rebec, GV. Neurobiology of vitamin C: expanding the focus from antioxidant to endogenous neuromodulator. Pharmacol Res. (2019) 146:104321. doi: 10.1016/j.phrs.2019.104321
16. Grela, A, Rachel, W, Cole, M, Zyss, T, Zięba, A, and Piekoszewski, W. Application of fatty acid and lipid measurements in neuropsychiatry. Clin Chem Lab Med. (2016) 54:197–206. doi: 10.1515/cclm-2015-0394
17. Falony, G, Vandeputte, D, Caenepeel, C, Vieira-Silva, S, Daryoush, T, Vermeire, S, et al. The human microbiome in health and disease: hype or hope. Acta Clin Belg. (2019) 74:53–64. doi: 10.1080/17843286.2019.1583782
18. Penders, J, Thijs, C, Vink, C, Stelma, FF, Snijders, B, Kummeling, I, et al. Factors influencing the composition of the intestinal microbiota in early infancy. Pediatrics. (2006) 118:511–21. doi: 10.1542/peds.2005-2824
19. Roswall, J, Olsson, LM, Kovatcheva-Datchary, P, Nilsson, S, Tremaroli, V, Simon, MC, et al. Developmental trajectory of the healthy human gut microbiota during the first 5 years of life. Cell Host Microbe. (2021) 29:765–776.e3. doi: 10.1016/j.chom.2021.02.021
20. Yatsunenko, T, Rey, FE, Manary, MJ, Trehan, I, Dominguez-Bello, MG, Contreras, M, et al. Human gut microbiome viewed across age and geography. Nature. (2012) 486:222–7. doi: 10.1038/nature11053
21. Perler, BK, Friedman, ES, and Wu, GD. The role of the gut microbiota in the relationship between diet and human health. Annu Rev Physiol. (2023) 85:449–68. doi: 10.1146/annurev-physiol-031522-092054
22. Zhang, P. Influence of foods and nutrition on the gut microbiome and implications for intestinal health. Int J Mol Sci. (2022) 23:9588. doi: 10.3390/ijms23179588
23. Horn, J, Mayer, DE, Chen, S, and Mayer, EA. Role of diet and its effects on the gut microbiome in the pathophysiology of mental disorders. Transl Psychiatry. (2022) 12:164. doi: 10.1038/s41398-022-01922-0
24. Berding, K, Vlckova, K, Marx, W, Schellekens, H, Stanton, C, Clarke, G, et al. Diet and the microbiota-gut-brain Axis: sowing the seeds of good mental health. Adv Nutr. (2021) 12:1239–85. doi: 10.1093/advances/nmaa181
25. Karbownik, MS, Mokros, Ł, and Kowalczyk, E. Who benefits from fermented food consumption? A comparative analysis between psychiatrically ill and psychiatrically healthy medical students. Int J Environ Res Public Health. (2022) 19:3861. doi: 10.3390/ijerph19073861
26. Safadi, JM, Quinton, AMG, Lennox, BR, Burnet, PWJ, and Minichino, A. Gut dysbiosis in severe mental illness and chronic fatigue: a novel trans-diagnostic construct? A systematic review and meta-analysis. Mol Psychiatry. (2022) 27:141–53. doi: 10.1038/s41380-021-01032-1
27. Dong, TS, Guan, M, Mayer, EA, Stains, J, Liu, C, Vora, P, et al. Obesity is associated with a distinct brain-gut microbiome signature that connects Prevotella and Bacteroides to the brain’s reward center. Gut Microbes. (2022) 14:2051999. doi: 10.1080/19490976.2022.2051999
28. Wastyk, HC, Fragiadakis, GK, Perelman, D, Dahan, D, Merrill, BD, Yu, FB, et al. Gut-microbiota-targeted diets modulate human immune status. Cell. (2021) 184:4137–4153.e14. doi: 10.1016/j.cell.2021.06.019
29. Bootz-Maoz, H, Pearl, A, Melzer, E, Malnick, S, Sharon, E, Bennet, Y, et al. Diet-induced modifications to human microbiome reshape colonic homeostasis in irritable bowel syndrome. Cell Rep. (2022) 41:111657. doi: 10.1016/j.celrep.2022.111657
30. Ghosh, TS, Rampelli, S, Jeffery, IB, Santoro, A, Neto, M, Capri, M, et al. Mediterranean diet intervention alters the gut microbiome in older people reducing frailty and improving health status: the NU-AGE 1-year dietary intervention across five European countries. Gut. (2020) 69:1218–28. doi: 10.1136/gutjnl-2019-319654
31. Meslier, V, Laiola, M, Roager, HM, De Filippis, F, Roume, H, Quinquis, B, et al. Mediterranean diet intervention in overweight and obese subjects lowers plasma cholesterol and causes changes in the gut microbiome and metabolome independently of energy intake. Gut. (2020) 69:1258–68. doi: 10.1136/gutjnl-2019-320438
32. Fan, Y, Wang, H, Liu, X, Zhang, J, and Liu, G. Crosstalk between the ketogenic diet and epilepsy: from the perspective of gut microbiota. Mediat Inflamm. (2019) 2019:8373060. doi: 10.1155/2019/8373060
33. Zhang, M, Zhao, D, Zhou, G, and Li, C. Dietary pattern, gut microbiota, and Alzheimer’s disease. J Agric Food Chem. (2020) 68:12800–9. doi: 10.1021/acs.jafc.9b08309
34. Tengeler, AC, Dam, SA, Wiesmann, M, Naaijen, J, van Bodegom, M, Belzer, C, et al. Gut microbiota from persons with attention-deficit/hyperactivity disorder affects the brain in mice. Microbiome. (2020) 8:44. doi: 10.1186/s40168-020-00816-x
35. Aarts, E, Ederveen, THA, Naaijen, J, Zwiers, MP, Boekhorst, J, Timmerman, HM, et al. Gut microbiome in ADHD and its relation to neural reward anticipation. PLoS One. (2017) 12:e0183509. doi: 10.1371/journal.pone.0183509
36. Jiang, HY, Zhou, YY, Zhou, GL, Li, YC, Yuan, J, Li, XH, et al. Gut microbiota profiles in treatment-naïve children with attention deficit hyperactivity disorder. Behav Brain Res. (2018) 347:408–13. doi: 10.1016/j.bbr.2018.03.036
37. Prehn-Kristensen, A, Zimmermann, A, Tittmann, L, Lieb, W, Schreiber, S, Baving, L, et al. Reduced microbiome alpha diversity in young patients with ADHD. PLoS One. (2018) 13:e0200728. doi: 10.1371/journal.pone.0200728
38. Bundgaard-Nielsen, C, Lauritsen, MB, Knudsen, JK, Rold, LS, Larsen, MH, Hindersson, P, et al. Children and adolescents with attention deficit hyperactivity disorder and autism spectrum disorder share distinct microbiota compositions. Gut Microbes. (2023) 15:2211923. doi: 10.1080/19490976.2023.2211923
39. Jacka, FN, O’Neil, A, Opie, R, Itsiopoulos, C, Cotton, S, Mohebbi, M, et al. A randomised controlled trial of dietary improvement for adults with major depression (the “SMILES” trial). BMC Med. (2017) 15:23. doi: 10.1186/s12916-017-0791-y
40. Parletta, N, Zarnowiecki, D, Cho, J, Wilson, A, Bogomolova, S, Villani, A, et al. A Mediterranean-style dietary intervention supplemented with fish oil improves diet quality and mental health in people with depression: a randomized controlled trial (HELFIMED). Nutr Neurosci. (2019) 22:474–87. doi: 10.1080/1028415X.2017.1411320
41. Adams, JB, Audhya, T, Geis, E, Gehn, E, Fimbres, V, Pollard, EL, et al. Comprehensive nutritional and dietary intervention for autism Spectrum disorder-a randomized, controlled 12-month trial. Nutrients. (2018) 10:369. doi: 10.3390/nu10030369
42. Ota, M, Matsuo, J, Ishida, I, Takano, H, Yokoi, Y, Hori, H, et al. Effects of a medium-chain triglyceride-based ketogenic formula on cognitive function in patients with mild-to-moderate Alzheimer’s disease. Neurosci Lett. (2019) 690:232–6. doi: 10.1016/j.neulet.2018.10.048
43. San Mauro Martin, I, Sanz Rojo, S, González Cosano, L, Conty de la Campa, R, Garicano Vilar, E, and Blumenfeld Olivares, JA. Impulsiveness in children with attention-deficit/hyperactivity disorder after an 8-week intervention with the Mediterranean diet and/or omega-3 fatty acids: a randomised clinical trial. Neurologia. (2022) 37:513–23. doi: 10.1016/j.nrl.2019.09.007
44. Liu, Y, Yang, C, Meng, Y, Dang, Y, and Yang, L. Ketogenic diet ameliorates attention deficit hyperactivity disorder in rats via regulating gut microbiota. PLoS One. (2023) 18:e0289133. doi: 10.1371/journal.pone.0289133
45. Walz, G, Blazynski, N, Frey, L, Schneider-Momm, K, Clement, H-W, Rauh, R, et al. Long-term effects of an Oligoantigenic diet in children with attention-deficit/hyperactivity disorder (ADHD) on Core symptomatology. Nutrients. (2022) 14:5111. doi: 10.3390/nu14235111
46. Black, MM. Impact of nutrition on growth, brain, and cognition. Nestle Nutr Inst Workshop Ser. (2018) 89:185–95. doi: 10.1159/000486502
47. Pardo-Garrido, A, Carpio-Toro, IL, Ramírez-Coronel, AA, Martínez-Suárez, PC, and Reivan-Ortiz, GG. The role of Iron and zinc in the treatment of ADHD among children and adolescents: a systematic review of randomized clinical trials. Nutrients. (2021) 13:4059. doi: 10.3390/nu13114059
48. Penella, C, and Calatayud, A. Pepper crop under climate change: Grafting as an environmental friendly strategy In: CS Rao, AK Shanker, and C Shanker, editors. Climate Resilient Agriculture - Strategies and Perspectives. London, UK: InTech (2018)
49. Delelegne, S, Belew, D, Mohammed, A, and Getachew, Y. Evaluation of elite hot pepper varieties (Capsicum spp.) for growth, dry pod yield and quality under Jimma Condition, South West Ethiopia. Int J Agricult Res. 9:364–74. doi: 10.3923/ijar.2014.364.374
50. Srinivasan, K. Biological activities of red pepper (Capsicum annuum) and its pungent principle capsaicin: a review. Crit Rev Food Sci Nutr. (2016) 56:1488–500. doi: 10.1080/10408398.2013.772090
51. Li, R, Lan, Y, Chen, C, Cao, Y, Huang, Q, Ho, CT, et al. Anti-obesity effects of capsaicin and the underlying mechanisms: a review. Food Funct. (2020) 11:7356–70. doi: 10.1039/d0fo01467b
52. Ghiasi, Z, Esmaeli, F, Aghajani, M, Ghazi-Khansari, M, Faramarzi, MA, and Amani, A. Enhancing analgesic and anti-inflammatory effects of capsaicin when loaded into olive oil nanoemulsion: an in vivo study. Int J Pharm. (2019) 559:341–7. doi: 10.1016/j.ijpharm.2019.01.043
53. Saria, A, Skofitsch, G, and Lembeck, F. Distribution of capsaicin in rat tissues after systemic administration. J Pharm Pharmacol. (1982) 34:273–5. doi: 10.1111/j.2042-7158.1982.tb04245.x
54. Wang, C, Lu, J, Sha, X, Qiu, Y, Chen, H, and Yu, Z. TRPV1 regulates ApoE4-disrupted intracellular lipid homeostasis and decreases synaptic phagocytosis by microglia. Exp Mol Med. (2023) 55:347–63. doi: 10.1038/s12276-023-00935-z
55. Çakır, M, Yüksel, F, Mustafa Özkut, M, Durhan, M, Kaymak, E, Tekin, S, et al. Neuroprotective effect of transient receptor potential Vanilloid 1 agonist capsaicin in Alzheimer’s disease model induced with okadaic acid. Int Immunopharmacol. (2023) 118:109925. doi: 10.1016/j.intimp.2023.109925
56. Jiang, X, Jia, LW, Li, XH, Cheng, XS, Xie, JZ, Ma, ZW, et al. Capsaicin ameliorates stress-induced Alzheimer’s disease-like pathological and cognitive impairments in rats. J Alzheimers Dis. (2013) 35:91–105. doi: 10.3233/JAD-121837
57. Wang, F, Xue, Y, Fu, L, Wang, Y, He, M, Zhao, L, et al. Extraction, purification, bioactivity and pharmacological effects of capsaicin: a review. Crit Rev Food Sci Nutr. (2022) 62:5322–48. doi: 10.1080/10408398.2021.1884840
58. Nagpal, R, Mishra, SK, Deep, G, and Yadav, H. Role of trp channels in shaping the gut microbiome. Pathog Basel Switz. (2020) 9:753. doi: 10.3390/pathogens9090753
59. Allais, L, De Smet, R, Verschuere, S, Talavera, K, Cuvelier, CA, and Maes, T. Transient receptor potential channels in intestinal inflammation: what is the impact of cigarette smoking? Pathobiol J Immunopathol Mol Cell Biol. (2017) 84:1–15. doi: 10.1159/000446568
60. Clapham, DE. TRP channels as cellular sensors. Nature. (2003) 426:517–24. doi: 10.1038/nature02196
61. Wiles, TJ, Jemielita, M, Baker, RP, Schlomann, BH, Logan, SL, Ganz, J, et al. Host gut motility promotes competitive exclusion within a model intestinal microbiota. PLoS Biol. (2016) 14:e1002517. doi: 10.1371/journal.pbio.1002517
62. Song, JX, Ren, H, Gao, YF, Lee, CY, Li, SF, Zhang, F, et al. Dietary capsaicin improves glucose homeostasis and alters the gut microbiota in obese diabetic Ob/Ob mice. Front Physiol. (2017) 8:602. doi: 10.3389/fphys.2017.00602
63. Ryu, MS, Yue, Y, Li, C, Yang, HJ, Zhang, T, Wu, X, et al. Moderate capsaicin-containing kochujang alleviates memory impairment through the gut-brain axis in rats with scopolamine-induced amnesia. Biomed Pharmacother Biomed Pharmacother. (2024) 178:117091. doi: 10.1016/j.biopha.2024.117091
64. Deng, R, Yu, S, Ruan, X, Liu, H, Zong, G, Cheng, P, et al. Capsaicin orchestrates metastasis in gastric cancer via modulating expression of TRPV1 channels and driving gut microbiota disorder. Cell Commun Signal. (2023) 21:364. doi: 10.1186/s12964-023-01265-3
65. Wang, Y, Tang, C, Tang, Y, Yin, H, and Liu, X. Capsaicin has an anti-obesity effect through alterations in gut microbiota populations and short-chain fatty acid concentrations. Food Nutr Res. (2020) 64:3525–39. doi: 10.29219/fnr.v64.3525
66. Baboota, RK, Murtaza, N, Jagtap, S, Singh, DP, Karmase, A, Kaur, J, et al. Capsaicin-induced transcriptional changes in hypothalamus and alterations in gut microbial count in high fat diet fed mice. J Nutr Biochem. (2014) 25:893–902. doi: 10.1016/j.jnutbio.2014.04.004
67. Mahoro, P, Moon, HJ, Yang, HJ, Kim, KA, and Cha, YS. Protective effect of gochujang on inflammation in a DSS-induced colitis rat model. Foods. (2021) 10:1072. doi: 10.3390/foods10051072
68. Inyang, D, Saumtally, T, Nnadi, CN, Devi, S, and So, PW. A systematic review of the effects of capsaicin on Alzheimer's disease. Int J Mol Sci. (2023) 24:10176. doi: 10.3390/ijms241210176
69. Thornton, T, Mills, D, and Bliss, E. Capsaicin: a potential treatment to improve cerebrovascular function and cognition in obesity and ageing. Nutrients. (2023) 15:1537. doi: 10.3390/nu15061537
70. Tyagi, S, Shekhar, N, and Thakur, AK. Protective role of capsaicin in neurological disorders: an overview. Neurochem Res. (2022) 47:1513–31. doi: 10.1007/s11064-022-03549-5
71. Pasierski, M, and Szulczyk, B. Beneficial effects of capsaicin in disorders of the central nervous system. Molecules. (2022) 27:2484. doi: 10.3390/molecules27082484
72. Liu, CH, Bu, XL, Wang, J, Zhang, T, Xiang, Y, Shen, LL, et al. The associations between a capsaicin-rich diet and blood amyloid-β levels and cognitive function. J Alzheimers Dis. (2016) 52:1081–8. doi: 10.3233/JAD-151079
73. Higashi, T, Murata, N, Fujimoto, M, Miyake, S, Egusa, M, Higuchi, H, et al. Capsaicin may improve swallowing impairment in patients with amyotrophic lateral sclerosis: a randomized controlled trial. Acta Med Okayama. (2022) 76:179–86. doi: 10.18926/AMO/63412
74. Saxena, A, Raghuwanshi, R, Gupta, VK, and Singh, HB. Chilli anthracnose: the epidemiology and management. Front Microbiol. (2016) 7:1527. doi: 10.3389/fmicb.2016.01527
75. Padayatty, SJ, Katz, A, Wang, Y, Eck, P, Kwon, O, Lee, J-H, et al. Vitamin C as an antioxidant: evaluation of its role in disease prevention. J Am Coll Nutr. (2003) 22:18–35. doi: 10.1080/07315724.2003.10719272
76. Doseděl, M, Jirkovský, E, Macáková, K, Krčmová, LK, Javorská, L, Pourová, J, et al. Vitamin C-sources, physiological role, kinetics, deficiency, use, toxicity, and determination. Nutrients. (2021) 13:615. doi: 10.3390/nu13020615
77. Milani, GP, Macchi, M, and Guz-Mark, A. Vitamin C in the treatment of COVID-19. Nutrients. (2021) 13:1172. doi: 10.3390/nu13041172
78. Otten, AT, Bourgonje, AR, Peters, V, Alizadeh, BZ, Dijkstra, G, and Harmsen, HJM. Vitamin C supplementation in healthy individuals leads to shifts of bacterial populations in the gut-a pilot study. Antioxidants (Basel). (2021) 10:1278. doi: 10.3390/antiox10081278
79. Vacca, M, Celano, G, Calabrese, FM, Portincasa, P, Gobbetti, M, and De Angelis, M. The controversial role of human gut Lachnospiraceae. Microorganisms. (2020) 8:573. doi: 10.3390/microorganisms8040573
80. Lozupone, CA, Stombaugh, JI, Gordon, JI, Jansson, JK, and Knight, R. Diversity, stability and resilience of the human gut microbiota. Nature. (2012) 489:220–30. doi: 10.1038/nature11550
81. Koh, A, De Vadder, F, Kovatcheva-Datchary, P, and Bäckhed, F. From dietary Fiber to host physiology: short-chain fatty acids as key bacterial metabolites. Cell. (2016) 165:1332–45. doi: 10.1016/j.cell.2016.05.041
82. Zhang, Y, Zhen, S, Xu, H, Sun, S, Wang, Z, Li, M, et al. Vitamin C alleviates rheumatoid arthritis by modulating gut microbiota balance. Biosci Trends. (2024) 18:187–94. doi: 10.5582/bst.2024.01037
83. Li, Y, Salih Ibrahim, RM, Chi, HL, Xiao, T, Xia, WJ, Li, HB, et al. Altered gut microbiota is involved in the anti-hypertensive effects of vitamin C in spontaneously hypertensive rat. Mol Nutr Food Res. (2021) 65:e2000885. doi: 10.1002/mnfr.202000885
84. Pham, VT, Dold, S, Rehman, A, Bird, JK, and Steinert, RE. Vitamins, the gut microbiome and gastrointestinal health in humans. Nutr Res. (2021) 95:35–53. doi: 10.1016/j.nutres.2021.09.001
85. Kwak, MJ, Kim, SH, Kim, HH, Tanpure, R, Kim, JI, Jeon, BH, et al. Psychobiotics and fecal microbial transplantation for autism and attention-deficit/hyperactivity disorder: microbiome modulation and therapeutic mechanisms. Front Cell Infect Microbiol. (2023) 13:1238005. doi: 10.3389/fcimb.2023.1238005
86. Wiktorowska-Owczarek, A, Berezińska, M, and Nowak, JZ. PUFAs: structures, metabolism and functions. Adv Clin Exp Med. (2015) 24:931–41. doi: 10.17219/acem/31243
87. Jarret, RL, Levy, IJ, Potter, TL, and Cermak, SC. Seed oil and fatty acid composition in Capsicum spp. J Food Compos Anal. (2013) 30:102–8. doi: 10.1016/j.jfca.2013.02.005
88. Chouaibi, M, Rezig, L, Hamdi, S, and Ferrari, G. Chemical characteristics and compositions of red pepper seed oils extracted by different methods. Ind Crop Prod. (2019) 128:363–70. doi: 10.1016/j.indcrop.2018.11.030
89. Agostoni, C, Nobile, M, Ciappolino, V, Delvecchio, G, Tesei, A, Turolo, S, et al. The role of Omega-3 fatty acids in developmental psychopathology: a systematic review on early psychosis, autism, and ADHD. Int J Mol Sci. (2017) 18:2608. doi: 10.3390/ijms18122608
90. Widenhorn-Müller, K, Schwanda, S, Scholz, E, Spitzer, M, and Bode, H. Effect of supplementation with long-chain ω-3 polyunsaturated fatty acids on behavior and cognition in children with attention deficit/hyperactivity disorder (ADHD): a randomized placebo-controlled intervention trial. Prostaglandins Leukot Essent Fatty Acids. (2014) 91:49–60. doi: 10.1016/j.plefa.2014.04.004
91. Dervola, KS, Roberg, BA, Wøien, G, Bogen, IL, Sandvik, TH, Sagvolden, T, et al. Marine Ο-3 polyunsaturated fatty acids induce sex-specific changes in reinforcer-controlled behaviour and neurotransmitter metabolism in a spontaneously hypertensive rat model of ADHD. Behav Brain Funct. (2012) 8:56. doi: 10.1186/1744-9081-8-56
92. LaChance, L, McKenzie, K, Taylor, VH, and Vigod, SN. Omega-6 to Omega-3 fatty acid ratio in patients with ADHD: a Meta-analysis. J Can Acad Child Adolesc Psychiatry. (2016) 25:87–96.
93. Costantini, L, Molinari, R, Farinon, B, and Merendino, N. Impact of Omega-3 fatty acids on the gut microbiota. Int J Mol Sci. (2017) 18:2645. doi: 10.3390/ijms18122645
94. Shahidi, F, and Ambigaipalan, P. Omega-3 polyunsaturated fatty acids and their health benefits. Annu Rev Food Sci Technol. (2018) 9:345–81. doi: 10.1146/annurev-food-111317-095850
95. Wolbrink, DRJ, Grundsell, JR, Witteman, B, van de Poll, M, van Santvoort, HC, Issa, E, et al. Are omega-3 fatty acids safe and effective in acute pancreatitis or sepsis? A systematic review and meta-analysis. Clin Nutr. (2020) 39:2686–94. doi: 10.1016/j.clnu.2019.12.006
96. Huang, Y, Liu, F, Lai, J, Jiang, S, Tan, X, Chen, L, et al. The adjuvant treatment role of ω-3 fatty acids by regulating gut microbiota positively in the acne vulgaris. J Dermatol Treat. (2024) 35:2299107. doi: 10.1080/09546634.2023.2299107
97. Guinane, CM, and Cotter, PD. Role of the gut microbiota in health and chronic gastrointestinal disease: understanding a hidden metabolic organ. Ther Adv Gastroenterol. (2013) 6:295–308. doi: 10.1177/1756283X13482996
98. Lauridsen, C. Effects of dietary fatty acids on gut health and function of pigs pre-and post-weaning. J Anim Sci. (2020) 98:skaa086. doi: 10.1093/jas/skaa086
99. Watson, H, Mitra, S, Croden, FC, Taylor, M, Wood, HM, Perry, SL, et al. A randomised trial of the effect of omega-3 polyunsaturated fatty acid supplements on the human intestinal microbiota. Gut. (2018) 67:1974–83. doi: 10.1136/gutjnl-2017-314968
100. Liu, P, Peng, G, Zhang, N, Wang, B, and Luo, B. Crosstalk between the gut microbiota and the brain: an update on neuroimaging findings. Front Neurol. (2019) 10:883. doi: 10.3389/fneur.2019.00883
101. Corona, JC. Role of oxidative stress and Neuroinflammation in attention-deficit/hyperactivity disorder. Antioxidants (Basel). (2020) 9:1039. doi: 10.3390/antiox9111039
102. Siopi, E, Galerne, M, Rivagorda, M, Saha, S, Moigneu, C, Moriceau, S, et al. Gut microbiota changes require vagus nerve integrity to promote depressive-like behaviors in mice. Mol Psychiatry. (2023) 28:3002–12. doi: 10.1038/s41380-023-02071-6
103. Lyte, M, Li, W, Opitz, N, Gaykema, RPA, and Goehler, LE. Induction of anxiety-like behavior in mice during the initial stages of infection with the agent of murine colonic hyperplasia Citrobacter rodentium. Physiol Behav. (2006) 89:350–7. doi: 10.1016/j.physbeh.2006.06.019
104. Bercik, P, Park, AJ, Sinclair, D, Khoshdel, A, Lu, J, Huang, X, et al. The anxiolytic effect of Bifidobacterium longum NCC3001 involves vagal pathways for gut-brain communication. Neurogastroenterol Motil. (2011) 23:1132–9. doi: 10.1111/j.1365-2982.2011.01796.x
105. Bravo, JA, Forsythe, P, Chew, MV, Escaravage, E, Savignac, HM, Dinan, TG, et al. Ingestion of Lactobacillus strain regulates emotional behavior and central GABA receptor expression in a mouse via the vagus nerve. Proc Natl Acad Sci USA. (2011) 108:16050–5. doi: 10.1073/pnas.1102999108
106. Sorboni, SG, Moghaddam, HS, Jafarzadeh-Esfehani, R, and Soleimanpour, S. A comprehensive review on the role of the gut microbiome in human neurological disorders. Clin Microbiol Rev. (2022) 35:e0033820. doi: 10.1128/CMR.00338-20
107. De Vadder, F, Grasset, E, Mannerås Holm, L, Karsenty, G, Macpherson, AJ, Olofsson, LE, et al. Gut microbiota regulates maturation of the adult enteric nervous system via enteric serotonin networks. Proc Natl Acad Sci USA. (2018) 115:6458–63. doi: 10.1073/pnas.1720017115
108. Yano, JM, Yu, K, Donaldson, GP, Shastri, GG, Ann, P, Ma, L, et al. Indigenous bacteria from the gut microbiota regulate host serotonin biosynthesis. Cell. (2015) 161:264–76. doi: 10.1016/j.cell.2015.02.047
109. Maqsood, R, and Stone, TW. The gut-brain Axis, BDNF, NMDA and CNS disorders. Neurochem Res. (2016) 41:2819–35. doi: 10.1007/s11064-016-2039-1
110. Han, W, Tellez, LA, Perkins, MH, Perez, IO, Qu, T, Ferreira, J, et al. A neural circuit for gut-induced reward. Cell. (2018) 175:665–678.e23. doi: 10.1016/j.cell.2018.08.049
111. Sudo, N. Microbiome, HPA axis and production of endocrine hormones in the gut. Adv Exp Med Biol. (2014) 817:177–94. doi: 10.1007/978-1-4939-0897-4_8
112. Cussotto, S, Sandhu, KV, Dinan, TG, and Cryan, JF. The neuroendocrinology of the microbiota-gut-brain Axis: a Behavioural perspective. Front Neuroendocrinol. (2018) 51:80–101. doi: 10.1016/j.yfrne.2018.04.002
113. Agirman, G, and Hsiao, EY. SnapShot: the microbiota-gut-brain axis. Cell. (2021) 184:2524–2524.e1. doi: 10.1016/j.cell.2021.03.022
114. Needham, BD, Funabashi, M, Adame, MD, Wang, Z, Boktor, JC, Haney, J, et al. A gut-derived metabolite alters brain activity and anxiety behaviour in mice. Nature. (2022) 602:647–53. doi: 10.1038/s41586-022-04396-8
115. Sandhu, KV, Sherwin, E, Schellekens, H, Stanton, C, Dinan, TG, and Cryan, JF. Feeding the microbiota-gut-brain axis: diet, microbiome, and neuropsychiatry. Transl Res J Lab Clin Med. (2017) 179:223–44. doi: 10.1016/j.trsl.2016.10.002
116. Fairley, A, Stewart, CJ, Cassidy, A, Woodside, JV, and McEvoy, CT. Diet patterns, the gut microbiome, and Alzheimer’s disease. J Alzheimers Dis. (2022) 88:933–41. doi: 10.3233/JAD-220205
117. Hsu, YJ, Chiu, CC, Li, YP, Huang, WC, Huang, YT, Huang, CC, et al. Effect of intestinal microbiota on exercise performance in mice. J Strength Cond Res. (2015) 29:552–8. doi: 10.1519/JSC.0000000000000644
118. Braniste, V, Al-Asmakh, M, Kowal, C, Anuar, F, Abbaspour, A, Tóth, M, et al. The gut microbiota influences blood-brain barrier permeability in mice. Sci Transl Med. (2014) 6:263ra158. doi: 10.1126/scitranslmed.3009759
119. D’Mello, C, Ronaghan, N, Zaheer, R, Dicay, M, Le, T, MacNaughton, WK, et al. Probiotics improve inflammation-associated sickness behavior by altering communication between the peripheral immune system and the brain. J Neurosci. (2015) 35:10821–30. doi: 10.1523/JNEUROSCI.0575-15.2015
120. Cervantes-Barragan, L, Chai, JN, Tianero, MD, Di Luccia, B, Ahern, PP, Merriman, J, et al. Lactobacillus reuteri induces gut intraepithelial CD4+CD8αα+ T cells. Science. (2017) 357:806–10. doi: 10.1126/science.aah5825
121. Gensollen, T, Iyer, SS, Kasper, DL, and Blumberg, RS. How colonization by microbiota in early life shapes the immune system. Science. (2016) 352:539–44. doi: 10.1126/science.aad9378
122. Mann, ER, Lam, YK, and Uhlig, HH. Short-chain fatty acids: linking diet, the microbiome and immunity. Nat Rev Immunol. (2024) 24:577–95. doi: 10.1038/s41577-024-01014-8
123. Zhang, W, Zhang, Y, Fan, J, Feng, Z, and Song, X. Pharmacological activity of capsaicin: mechanisms and controversies (review). Mol Med Rep. (2024) 29:38. doi: 10.3892/mmr.2024.13162
124. López-Carrillo, L, López-Cervantes, M, Robles-Díaz, G, Ramírez-Espitia, A, Mohar-Betancourt, A, Meneses-García, A, et al. Capsaicin consumption, Helicobacter pylori positivity and gastric cancer in Mexico. Int J Cancer. (2003) 106:277–82. doi: 10.1002/ijc.11195
125. Geng, S, Zheng, Y, Meng, M, Guo, Z, Cao, N, Ma, X, et al. Gingerol reverses the Cancer-promoting effect of capsaicin by increased TRPV1 level in a urethane-induced lung carcinogenic model. J Agric Food Chem. (2016) 64:6203–11. doi: 10.1021/acs.jafc.6b02480
126. Luo, L, Yan, J, Wang, X, and Sun, Z. The correlation between chili pepper consumption and gastric cancer risk: a meta-analysis. Asia Pac J Clin Nutr. (2021) 30:130–9. doi: 10.6133/apjcn.202103_30(1).0016
127. Jiang, S, Li, H, Zhang, L, Mu, W, Zhang, Y, Chen, T, et al. Generic Diagramming Platform (GDP): a comprehensive database of high-quality biomedical graphics. Nucleic Acids Research. (2024) gkae973. doi: 10.1093/nar/gkae973
128. Führer, M, and Hammer, J. Effect of repeated, long term capsaicin ingestion on intestinal chemo-and mechanosensation in healthy volunteers. Neurogastroenterol Motil. (2009) 21:e7. doi: 10.1111/j.1365-2982.2008.01227.x
129. Abbas, MA. Modulation of TRPV1 channel function by natural products in the treatment of pain. Chem Biol Interact. (2020) 330:109178. doi: 10.1016/j.cbi.2020.109178
130. Yeon, KY, Kim, SA, Kim, YH, Lee, MK, Ahn, DK, Kim, HJ, et al. Curcumin produces an antihyperalgesic effect via antagonism of TRPV1. J Dent Res. (2010) 89:170–4. doi: 10.1177/0022034509356169
Keywords: ADHD, gut microbiota, diet, MGBA, chili peppers
Citation: Li Y, Feng J, Ding G, Deng L, He Y, Zhang Q, Wang J and Chen X (2025) The possible effects of chili peppers on ADHD in relation to the gut microbiota. Front. Nutr. 12:1551650. doi: 10.3389/fnut.2025.1551650
Edited by:
Ke Chen, University of Electronic Science and Technology of China, ChinaReviewed by:
Song Cui, Children’s Hospital of Chongqing Medical University, ChinaLubna Al-Gailani, Cyprus Health and Social Sciences University, Cyprus
Kamalesh Dattaram Mumbrekar, Manipal Academy of Higher Education, India
Copyright © 2025 Li, Feng, Ding, Deng, He, Zhang, Wang and Chen. This is an open-access article distributed under the terms of the Creative Commons Attribution License (CC BY). The use, distribution or reproduction in other forums is permitted, provided the original author(s) and the copyright owner(s) are credited and that the original publication in this journal is cited, in accordance with accepted academic practice. No use, distribution or reproduction is permitted which does not comply with these terms.
*Correspondence: Xia Chen, MDZjaGVueGlhQDE2My5jb20=
†These authors have contributed equally to this work