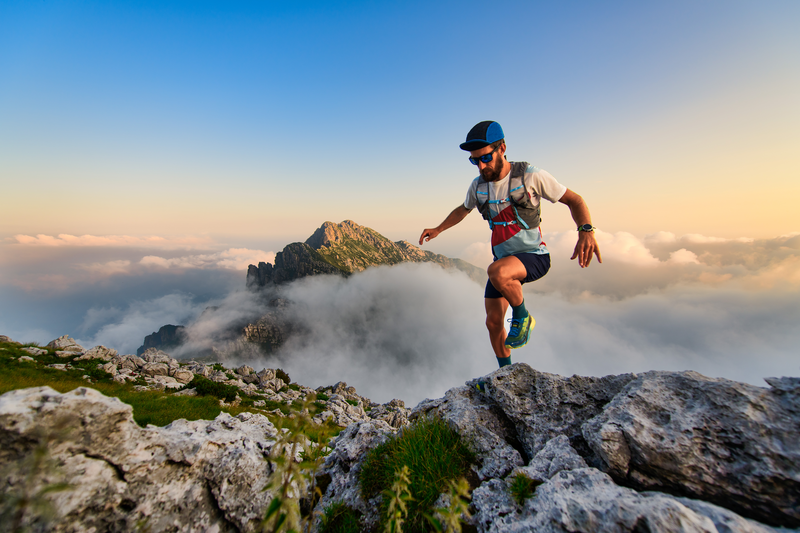
95% of researchers rate our articles as excellent or good
Learn more about the work of our research integrity team to safeguard the quality of each article we publish.
Find out more
ORIGINAL RESEARCH article
Front. Nutr. , 19 March 2025
Sec. Nutrition and Food Science Technology
Volume 12 - 2025 | https://doi.org/10.3389/fnut.2025.1538039
This article is part of the Research Topic Trends on Valorization of Agri-Food Waste Through Green Technologies View all 4 articles
Introduction: Current research primarily focuses on exploring and developing innovative substrates for cultivating Lentinus edodes, aiming to address substrate shortages and the continuous rise in production costs.
Methods: This study uses Quercus (oak) sawdust (OS) as a control to evaluate the potential of Korshinsk peashrub (KP), Hippophae rhamnoides (seabuckthorn) pruning (HRP), and Lycium barbarum (goji) pruning (LBP) in the cultivation of L. edodes by measuring parameters such as mycelium growth, yield, protein, fat, fiber, amino acids, soluble sugars, and organic acids. Furthermore, principal component analysis and official script function analysis were used to investigate the influence of the matrix formula ratio on the nutritional values of shiitake mushrooms.
Results: Results showed that the average duration to complete stages 2 and 3 of mycelial growth on 10% KP substrates significantly decreased compared to the OS group by 11.0 and 10.7 days, respectively. The weight of mushrooms produced from all agro-forest waste substrates was significantly lower than that of the control group, decreasing by 18.96 to 53.88%. The average mushroom weight for KP groups ranged from 235.37 g/kg to 252.27 g/kg, which was statistically higher than that of the LBP treatments, which ranged from 143.56 g/kg to 165.96 g/kg. However, the protein content in the 10% LBP and 10% HRP groups was significantly higher than that of the control, with increases of 4.69 and 12.89%, respectively, and fiber content also improved, increasing by 3.98 to 12.59%. Furthermore, the content of sweet-tasting amino acids in the 10% KP and 20% KP groups significantly increased compared to the OS group (by 34.86 and 144.92%, respectively). The 30% LBP and 10% KP-10% LBP-10% HRP groups exhibited higher glucose values compared to the OS (increased by 118.71 and 72.26%, respectively). Interestingly, the addition of LBP and KP to the substrates promoted the synthesis of acetic acid in shiitake mushrooms, while this organic acid was not detected in the OS. In summary, shiitake mushrooms cultured in 20% KP, 10% LBP, or 10% KP-10% LBP-10% HRP demonstrated significantly better overall performance.
Discussion: This approach not only reduces operational costs by at least 1,680 RMB but also contributes to environmental sustainability by diverting 2,400 kg of agro-forest waste from landfills. Consequently, the utilization of agroforestry waste serves as an effective strategy not only for environmental protection and cost reduction during mushroom production but also for enhancing the nutritional value of shiitake mushrooms. This, in turn, helps combat malnutrition and contributes to national food security.
Mushrooms are considered healthy foods due to their low-calorie content and various beneficial components, such as polysaccharides, phenolics, and triterpenes (1). Lentinus edodes is one of the most popular and commercially favored edible mushroom species (2, 3). China accounts for approximately 72% of global mushroom production, solidifying its position as the world’s leading mushroom producer. Notably, the cultivation of L. edodes (shiitake mushroom) represents approximately 25% of China’s total mushroom output (4). In 2022, China produced approximately 12.96 million tons of L. edodes, representing 98.3% of the total global production of shiitake mushrooms (5). In the wild, L. edodes are typically found growing on hardwood trees, particularly those from the Fagaceae family, including oak, beech, and chestnut (6). On an industrial scale, the conventional substrate for cultivating L. edodes typically consists of approximately 80% hardwood sawdust, primarily from oak, and 20% starch-rich additives, such as wheat bran or rice bran. In China, sawdust has emerged as one of the main substrates for cultivating L. edodes due to its excellent aeration, water retention, nutrient supply, and easy availability; its proportion usually accounts for approximately 50% of bagged substrates (7). With the rapid expansion of L. edodes cultivation areas, substrate shortages and rising production costs have become significant challenges for the industry. Numerous research and industry efforts are currently underway to address these issues.
The mycelium of L. edodes can secrete lignin-degrading enzymes, enabling it to produce fruiting bodies on wood or similar artificial materials. Various wood products and agricultural byproducts serve as substrates for the commercial production of L. edodes (8). Currently, L. edodes can be cultivated on a diverse range of lignocellulosic substrates, such as coffee grounds, sugarcane bagasse, grain straw, vineyard prunings, and sorghum stubble (9–16). These alternative substrates not only enhance the growth of L. edodes but also contribute to resource recycling and minimize the environmental impact of crop waste. Therefore, the research to explore low-cost and locally adapted alternative substrates for L. edodes will continue as the scale of its cultivation expands.
Cash tree production has rapidly increased in China over the last decade, making it one of the largest fruit producers in the world (17, 18). Due to its excellent salt tolerance, drought resistance, fast growth, and ability to produce fruit in the first year of planting, Lycium (Goji) is widely used for improving saline land and supporting rural economic development. As a result, the area devoted to Lycium cultivation has expanded in northern China over the last few decades (19). The estimated production areas of Lycium cover approximately 880 million m2, extending across the entire northwest to central China, including Xinjiang, Ningxia, Gansu, Qinghai, Shanxi, Inner Mongolia, and Hubei (20). As an ecological protection plant, the annual yield of Caragana korshinsk is estimated at approximately 550,000 tons and is widely distributed in northeastern, northwestern, and northern China, representing a significant lignocellulosic biomass resource (21, 22). Moreover, as one of the most effective species for soil and water conservation, the total area of Hippophae rhamnoides (seabuckthorn) in China is approximately 2.7 million m2, accounting for more than 90% of the world’s growth area (23). Notably, these shrub plants exhibit remarkable branching and regenerative abilities, requiring regular pruning every 4–5 years. The majority of Korshinsk peashrub (KP), Hippophae rhamnoides pruning (HRP), and Lycium barbarum pruning (LBP) are not handled properly, representing a substantial lignocellulosic biomass resource. These materials are often either burned or discarded along orchard edges. However, their high content of lignocellulose leads to poor feed quality, while incineration is not in line with the existing policies in China, making the stroma utilization of pruning waste a problem.
According to early studies, these three types of agro-forest wastes have the potential for mushroom cultivation. For instance, KP has been shown to accelerate the growth rate of Pleurotus tuoliensis (24) and enhance the fruit body yield of Pleurotus eryngii (22). When used at a proportion of 78% (by volume), KP improved the protein, crude fiber, crude fatty acid, polysaccharide, and total sugar contents of L. edodes (21). Additionally, the use of 20% HRP (by volume) significantly improved the mycelial biomass and total cellulose enzyme activity of L. edodes (25). Similarly, LBP also has the potential to be used for the cultivation of L. edodes due to its wide variety of phytonutrients, including proteins, minerals, vitamins, and functional components (26–29).
However, the use of these pruning waste materials for mushroom cultivation has not yet been investigated. Therefore, this study represents the first attempt to explore the potential of locally available KP, HRP, and LBP, either individually or in various combinations, for growing the shiitake strain “Qihe#1.” Furthermore, it examines alternative local substrate formulations to replace the conventional oak sawdust substrate used in L. edodes production.
The experiment was conducted at the Center for Mushroom Studies in Qinghai Province, under the Qinghai Academy of Agricultural and Forestry Sciences, China (E101°49′17″, N36°34′03″). In this experiment, 10 treatments (substrate formulations) were established, each repeated 12 times (120 bags of 1,000 g fresh weight) using a completely randomized design method. The 10 tested substrate formulations consisted of a mixture of OS (oak sawdust), Korshinsk peashrub (KP), Hippophae rhamnoides pruning (HRP), and Lycium barbarum pruning (LBP) in varying ratios (Table 1). It is important to note that the maximum amount of alternative sawdust was limited to 30%, based on findings from our pilot study (unpublished). Subsequently, oak sawdust was added to the mixture to ensure that the total proportion of sawdust reached 80%. Similarly, 180 g of wheat bran, 10 g of sugar, and 10 g of calcium carbonate were evenly incorporated into all substrates, which were prepared on a dry weight basis. The pruned branches of Goji and Seabuck were collected from Nomuhong Town in Golmud City, Qinghai Province, while Korshinsk peashrub was gathered in Ledu District, Haidong City, Qinghai Province. Oak sawdust was collected from a local oak forest located in Ankang City, Shaanxi Province. A sawdust crusher was used to reduce the size of the Lycium, Hippophae, and Korshinsk branches to sawdust measuring 3–5 cm in size.
The strain “Qihe#1” was deposited and is maintained at the Edible Mushroom Research Center of the Qinghai Academy of Agricultural and Forestry Sciences. The isolates are preserved in a potato-dextrose-agar (PDA) medium at 4°C.
A wheat grain culture medium (85% wheat, 10% sawdust, 3% bran, 1% gypsum, 1% sucrose) was prepared for culturing the mycelium of L. edodes (21, 30). The wheat grains were steamed until the interior of the grains had no white core, after which they were filtered and mixed with the other ingredients. Each mixture (250 g fresh weight) was placed in a polypropylene bag and autoclaved at 121°C for 4 using a horizontal autoclave pot (Runjin, Shandong, China) RJA-1200 (hereafter referred to as “seed bag”). After cooling, the mycelium, pre-prepared in PDA medium, was inoculated into each seed bag. The inoculated bags were then placed under room temperature conditions to cultivate the mycelium for 3 weeks.
The conventional production formula for L. edodes (78% oak sawdust, 20% wheat bran, 1% sugar, and 1% light calcium carbonate) was used as the control. Different proportions of seabuckthorn, goji berry, and C. intermedia sawdust were added to replace the sawdust in the control formula, serving as the experimental treatment groups. The specific treatment formulas are detailed in Table 1. The materials were prepared in accordance with the specified formula proportions, ensuring uniformity through mixing. Subsequently, water was added to achieve a moisture content of 55–60%. Finally, lime or calcium carbonate was added to adjust the pH to a range of 7.0–7.5. Each mixture (1,000 g fresh weight) was placed in a polypropylene bag measuring 18 cm × 36 cm × 50 cm and sterilized at normal pressure for 18–20 h at 100°C using the YXQWF-C horizontal rectangular pressure steam sterilizer (Yixiang, Hubei, China; hereafter referred to as “stick’”). After sterilization, a 2% (W/W) spawn, previously prepared in a seed bag, was inoculated into the cooled stick and incubated at room temperature in the dark for 1 month to allow for colonization and hyphal growth.
The stick of L. edodes culture should be maintained in complete darkness at a temperature of 20–22°C and a relative humidity of 50–60%. Throughout the entire growth and development cycle of L. edodes, mycelium development is categorized into four consecutive stages based on the research by Song et al. (31), as shown in Table 2. The conclusion of each stage was recorded as the number of days after spawning. The polyethylene bags were removed to induce fruiting bodies at the end of stage 3. Twenty-four hours later, the sticks were placed on shelves inside the fruiting room, which was set to a temperature of 16°C and a relative humidity of 90%, with scattered light provided. The number of days to harvest (HT) was also documented. Mushrooms were harvested in total two times (1st: F1 and 2nd: F2). At the conclusion of F1, the sticks were incubated again in a dark environment with a fruiting room temperature of 20–22°C and a relative humidity of 60%. During each mushroom harvest, the quantity and weight of fresh mushrooms in each stick (NM) were recorded. Additionally, the yield was assessed as the total weight of mushrooms harvested per stick (WM) during the two flushes. In the first flush, a sliding caliper was used to measure the mushroom pileus diameter.
Table 2. The four consecutive stages of shiitake mycelium development (31).
Fresh and representative mushroom samples from each treatment of the first flush were selected to evaluate their nutritional composition. Each analysis was conducted three times. The ash content was determined by taking 5.0 g samples of mushrooms, placing them in a crucible, and heating them at 550°C in a muffle furnace for 24 h. The ash content was calculated as shown in Equation 1:
M2: weight of the crucible containing ash.
M1: weight of an empty crucible.
The fat content (%) was assessed using the Soxhlet apparatus technique (32) by weighing 5.0 g of mushroom samples and placing them in the pre-weighed Soxhlet extractor flask (M1). A volume of 500 mL of petroleum ether was added to the flask, and the system was then heated for 10 h. Subsequently, the residue in the bottom flask was dried in a bain-marie at 100°C ± 5°C for 1 h, after which the fat remaining in the bottom flask was weighed (M2). The fat content was calculated as shown in Equation 2:
M2: weight of the flask containing fat.
M1: weight of the empty flask of the extractor.
Crude fiber content (%) was determined according to NFSS (33) as the loss on ignition of the dried residue remaining after digesting a 5.0 g dry mushroom sample in 1.25% (w/v) H2SO4 and 1.25% (w/v) KOH. Crude protein content (N*6.25; %) was determined using the Kjeldahl method based on NFSS (34).
Amino acid content (%) was determined according to NFSS (35). First, 2.0 g of the sample was prepared and placed in a hydrolysis tube, followed by the addition of 10 mL hydrochloric acid (6 mol/L). The system was then frozen, vacuum-sealed, and filled before being hydrolyzed at 110°C for 22 h. After hydrolysis, the tubes were rinsed with water, and the rinsing waste liquid (V1) and hydrolysate (V2) were combined and diluted to a final volume of 50 mL. Subsequently, 1.0 mL of the hydrolysate was accurately measured. Following thorough sample preparation, which included concentration, washing, and drying, the processed hydrolysate was supplemented with 2.0 mL of sodium citrate solution. Amino acid content was analyzed using an amino acid analyzer, and concentrations of amino acids in the sample were calculated using the external standard method based on peak area. Amino acid content was calculated using the following Equation 3:
Ci: The concentration in the sample solution (nmol/mL).
F: The dilution factor.
V: The volume of the hydrolysate transferred and diluted (mL).
M: The molar mass of the amino acid (g/mol).
The extraction and analysis of organic acids were conducted as follows: a 1.0 g sample was weighed in a centrifuge tube, and 10 mL of water was added and vortexed for 30 s. After ultrasonic extraction at room temperature for 30 min, the sample was centrifuged at 5,000 r/min for 5 min. The supernatant was mixed with 10 mL of ethanol and vortexed for another 30 s, then centrifuged again at 5,000 r/min for 5 min. The resulting supernatant was transferred to a chicken heart flask before being evaporated to dryness using rotary evaporation, redissolved in 5 mL of water, centrifuged at 10000 r/min for 5 min, filtered through a 0.22 μm membrane, and prepared for testing. The HPLC system utilized an LC-2030C Plus Athena-C18 column (4.6 mm × 250 mm, 5 μm) sourced from Shimadzu, based in Shanghai, China. To identify and quantify the organic acids, their retention times were compared to those of authentic standards supplied by Yuanye Bio-Technology Co., Ltd., also in Shanghai, China. Furthermore, the quantification of each sample was achieved by comparing its peak area against the calibration curve of the corresponding standard compound.
The extraction process for polyols and soluble sugars from the samples was conducted according to previously established methods. The LC-20AT HPLC system utilized an Athena NH2-RP (II) column (4.6 mm × 250 mm, 5 μm) paired with a 20A RID. The primary soluble sugars in the samples were fully resolved within a 40-min isocratic elution. The column was maintained at 40°C, with a mobile phase of acetonitrile: water (70: 30) at a flow rate of 1.0 mL/min, and an injection volume of 10 μL was employed. Each analyte was assessed against a reference sample from Yuanye (Shanghai) and quantified using the calibration curve of the respective authentic compounds.
Data analysis was conducted using a one-way analysis of variance (ANOVA), and means were compared using Duncan’s multiple range test at a p-value of <0.05 in SPSS 25.0. To explore the relationships between indicators during the growth stage of L. edodes, Pearson correlation analysis was conducted using the Correlation Plot function in Origin 2021. To mitigate the adverse effects of different measurement scales, the original data for each index were standardized using SPSS 25.0. Principal component analysis (PCA) was then applied to reduce dimensionality and simplify the data. Additionally, membership function analysis was used to standardize the indexes obtained from the principal component analysis. The membership function values and the comprehensive evaluation value (36) were calculated using the following Equations 4–6:
Xj: The measured value of the jth index.
Xmax: The maximum value of the jth index.
Xmin: The minimum value of the jth index.
Wj: The importance degree of the jth comprehensive indicator in all comprehensive indicators.
Pj: The contribution rate of the jth comprehensive index.
D: The comprehensive evaluation of different tested substrates.
After the incubation period, the substrates showed dark-colored patches that eventually spread to cover the entire surface. According to the results of the one-way ANOVA (Table 3), there was no significant difference in the average number of days required to complete stages 1, 2, and 3 of mycelial growth on the tested substrates compared to the control, except for a significant reduction in stages 2 and 3 on 10%KP (reduced by 11.0 and 10.7 days, respectively). Conversely, the average number of days to reach stage 4 was significantly delayed in 20%KP and 30%KP compared to the OS (delayed by 34.67 and 35.17 days, respectively). Notably, the average number of days to reach stage 4 was reduced in the mixture 10%LBP-10%HRP compared to the OS (by 2 days). The duration was also significantly extended in the 20%KP and 30%KP treatments compared to OS treatment, with increases of 35.26 days and 35.88 days, respectively.
Table 3. The number of days to the mycelium development, fruit formation, and harvest in different experiments.
Results in Table 4 showed that the average mushroom number was significantly reduced compared to the control, except for 10% HRP, 20% KP, and the mixture of 10% KP-10% LBP-10% HRP (by 8.3, 7.9, and 6.9, respectively). The mixture treatment of 10% KP-10% LBP-10% HRP significantly improved the average mushroom number compared to LBP alone (by 9.2) and KP alone (by 7.5). The average mushroom number for LBP ranged from 16.8 to 24.1, showing a statistical difference from the KP substrates (10, 20, and 30%, respectively). On the other hand, the weight of mushrooms was significantly lower than the control in all substrates. The average mushroom weight in the KP groups ranged from 235.37 g/kg to 252.27 g/kg, demonstrating a statistically significant increase compared to the LBP treatments, where the average weight ranged from 143.56 g/kg to 165.96 g/kg. Mixing LBP and HRP improved the weight of mushrooms compared to LBP alone (by 35.38 g/kg) and HRP alone (by 48.5 g/kg). Simultaneously, the weight of mushrooms increased correspondingly (by 9.11 g/kg and 7.79 g/kg, respectively) with the addition of KP. However, mixing LBP, KP, and HRP reduced the weight of mushrooms compared to KP alone (by 14.26 g/kg).
Table 4. The number of days to the mycelium development, fruit formation, and harvest in different culture medium experiments.
Furthermore, both the average fresh weight of mushrooms and the total biological efficiency were significantly lower than that of OS across all substrates (Table 4). The average biological efficiency (BE) for KP ranged from 46.1 to 50.5%, which was statistically higher than that of LBP (ranging from 28.7 to 33.3%). Mixing LBP and HRP improved biological efficiency compared to LBP alone (an increase of 6.9%) and HRP alone (an increase of 9.6%). Notably, the comparison of productive indicators between flush 1 and flush 2 showed that 20% KP, 30% KP, and 10% KP-10% LBP-10% HRP resulted in significantly higher production of the second flush compared to other substrates (with second flush ratios of 5.06, 10.62, and 7.04%, respectively).
Moreover, mushrooms produced by the substrate inoculated with KP, HRP, and LBP were mainly from the G1 and G2 groups, except that 30% LBP produced 100% mushrooms from the G2 group and 30% KP produced 6.7% mushrooms from the G2 group. The supplemented 30% KP produced larger mushrooms (the pileus diameter 10–15 cm) than the other tested substrates.
The analysis of the nutritional composition of mushrooms (Table 5) showed that the protein content of the fruit body was significantly higher in 10% LBP and 10% HRP (24.53 and 26.45%, respectively) compared to OS. Additionally, it was significantly improved in treatments containing LBP (10% LBP, 20% LBP, 30% LBP, and 10% LBP-10% HRP) compared to those containing KP (10% KP, 20% KP, and 30% KP), with the highest value recorded in 10% LBP (24.53%). The fiber content of the mushrooms was enhanced in all tested substrates compared to OS, with the highest values found in 10% HRP and 30% KP (10.38 and 10.46%, respectively). Ash content was significantly lower in most substrates compared to OS. Fat content was lower in mushrooms from most substrates compared to OS, except in 20% LBP, 30% LBP, 10% LBP-10% HRP, and 10% KP-10% LBP-10% HRP (0.90, 0.79, 0.79, and 0.91%, respectively), where it was significantly higher.
Table 5. Nutritional components analysis of the used sawdust and harvest fresh L. edodes in 10 different substrates.
Table 6 shows the amino acid composition and content of shiitake mushroom fruiting bodies cultivated on various substrate formulations. Seventeen amino acids—aspartate, threonine, serine, valine, methionine, isoleucine, leucine, phenylalanine, lysine, glutamate, glycine, alanine, L-cysteine, tyrosine, histidine, arginine, and proline—were identified in shiitake mushrooms, while tryptophan was found at levels below the detection limit. The content of 15 amino acids across 8 substrates showed a significant decrease compared to the control. Specifically, the amino acid content in the 20% KP treatment group increased from 0.529 to 14.527, whereas it ranged from 0.032 to 3.854 in the 10% KP treatment. Conversely, the glutamate content of all test substrates was significantly lower than that of the OS, while the methionine levels in the 10% LBP, 20% LBP, and 30% LBP treatments were significantly higher compared to the control (1.411, 0.98, and 0.857, respectively).
Furthermore, the content of sweet-tasting amino acids significantly increased in 10%KP and 20%KP compared to the OS (by 5.023 and 20.884, respectively). The content of bitter-tasting amino acids and aromatic amino acids also showed significant increases in 10%KP (by 1.194 and 0.366, respectively) and 20%KP (by 6.435 and 1.962, respectively) when compared to the OS. In contrast, the MSG-like amino acid content significantly decreased in all tested substrates compared to the OS.
Moreover, the total content of essential amino acids in the 10% KP and 20% KP treatments was significantly greater compared to the OS (by 2.711 and 14.166, respectively). Similarly, the total content of nonessential amino acids in the 10% KP and 20% KP treatments was also significantly greater compared to the OS (by 3.241 and 14.26, respectively). The EAA/TAA ratio of the LBP group improved significantly by 23.66 to 30.43% compared with the OS, and the EAA/NEAA ratio also rose by 38.66 to 54.86%.
The contents of soluble sugars and organic acids in the fruiting bodies of L. edodes cultivated on different substrates are shown in Table 7. Arabitol content was higher in mushrooms cultivated on substrates with a higher proportion of 10% LBP, 20% KP, and the mixture of 10% KP-10% LBP-10% HRP, showing a significant difference compared to the other groups (p < 0.05). The 30% LBP group and the mixture of 10% KP-10% LBP-10% HRP exhibited higher glucose values compared to the OS (p < 0.05). The addition of 10% LBP to the substrate also resulted in a significantly increased trehalose content compared to OS (p < 0.05). In total, nine organic acids were detected in all samples. Statistical analysis showed that the content of mushrooms in the tested substrates was significantly different (Table 7). The tartaric acid content in mushrooms cultured in the 30% LBP treatment group was the highest (0.25 mg/g), whereas the OS group had the lowest (0.04 mg/g). The 20% LBP treatment provided the least amount of formic acid (0.13 mg/g), while the 20% KP treatment yielded the highest content (5.59 mg/g). The malic acid content in shiitake mushrooms ranged from 0.89 to 1.31 mg/g. Interestingly, the addition of LBP and KP to the substrate enhanced the synthesis of acetic acid in shiitake mushrooms, which was not detected in the OS. The citric acid content in the samples from the 10% LBP treatment group was the highest (12.66 mg/g), followed by the 10% KP-10% LBP-10% HRP treatment group (11.33 mg/g) and the 10% LBP-10% HRP treatment group (10.32 mg/g). For the fumaric acid content in L. edodes fruiting bodies, the 10% LBP-10% HRP treatment group showed the highest level (3.88 mg/g), followed by the 10% LBP group (3.88 mg/g) and the 30% LBP group (2.86 mg/g). Additionally, it was suggested that the addition of 30% LBP and 20%KP had a significant influence on the contents of succinic acid and butyric acid in L. edodes.
Table 7. The contents of soluble sugars and organic acids in L. edodes samples of different cultivation substrates.
The results of the correlation analysis (Figure 1) showed that the substrates were positively correlated with seven growth indicators, whereas DAS1 and DAS3 exhibited a negative correlation. The weight of the mushroom (WM) was significantly influenced by DAS2, DAS4, HT, SM, and BE (p < 0.01). A negative correlation was found between NM and SM (p < 0.05). The harvest time was significantly impacted by DAS2 and DAS4 (p < 0.01). Conversely, there was a significant negative correlation between the cultivation (p < 0.05) substrate and glucose, tartaric acid, acetic acid, citric acid, fumaric acid, succinic acid, and butyric acid (Supplementary Figure 1). Among the three soluble sugars, a highly significant and strong positive correlation existed between arabiol and trehalose (p < 0.01). Regarding the nine organic acid components, there were extremely significant strong positive correlations between tartaric acid and butyric acid, succinic acid and tartaric acid, and succinic acid and butyric acid (with correlation coefficients of 0.95, 0.66, and 0.66, respectively; p < 0.01). Furthermore, glucose showed extremely significant positive correlations with tartaric acid and butyric acid, respectively.
Considering that there are more than 16 indicators and varying degrees of correlation among them, principal component analysis (PCA) was used to simplify and reduce the dimensionality of each indicator. The original 16 indicators were transformed into six independent, comprehensive indicators based on the principle that the characteristic eigenvalue of a principal component is greater than 1.00 and the cumulative contribution rate exceeds 80% (Table 8). The cumulative contribution rate of these six principal components was 88.16%, indicating that the six components can retain 88.16% of the information from the original index, which adequately explains the quality of shiitake mushrooms. The quality indicators for the principal components of L. edodes are shown in Figure 2. The factors NEAA, SAA, and ARAA significantly influenced the first principal component (PC1) with loading coefficients of 0.369, 0.364, and 0.356, respectively, suggesting that PC1 primarily reflects amino acid-related information. The second principal component (PC2) was predominantly influenced by trehalose, citric acid, arabinol, and glucose, with loading coefficients of 0.415, 0.410, and 0.311, indicating that PC2 is mainly associated with soluble sugar content. The third principal component (PC3) was significantly affected by tartaric acid, acetic acid, and propionic acid. Among these, tartaric acid and acetic acid exhibited larger positive loading values, while propionic acid showed a larger negative loading value (loading values were 0. 376, 0. 343, and-0.430, respectively), meaning that PC3 primarily reflects organic acid content. Subsequently, the membership function values (μxj) and comprehensive evaluation values (D) for the six principal component indicators were calculated, as detailed in Table 9. The results indicated that the comprehensive evaluation values for the groups 20% KP, 10% LBP, and 10% KP-10% LBP-10% HRP ranked among the top three, with D values of 0.67, 0.60, and 0.54, respectively. These findings suggest that shiitake mushrooms cultivated using these three substrates exhibit a higher nutritional value. Furthermore, among the 10 substrates evaluated, the groups 20% KP, 10% LBP, and 10% KP-10% LBP-10% HRP were identified as the optimal substrates for mushroom cultivation.
Table 9. Membership function analysis of quality indicators of L. edodes with different cultivation substrates.
Cultivating edible mushrooms has proven to be an effective biological method for transforming agricultural and forestry byproducts. This study comprehensively evaluates the feasibility of using fruit tree pruning residues as substrates for cultivating L. edodes. Additionally, we analyzed and summarized the effects of different addition ratios of Korshinsk peashrub (KP), Hippophae rhamnoides pruning (HRP), and Lycium barbarum pruning (LBP) on the yield, amino acid, and protein contents of Lentinus edodes. Our research results demonstrate that utilizing sawdust derived from discarded branches for shiitake mushroom cultivation is a practical alternative. Both sawdust obtained solely from forest waste and partially mixed sawdust can be effectively employed in shiitake mushroom cultivation. However, it should be noted that the proportions of these additives can significantly influence mycelium growth and the development of fruiting bodies (Tables 3, 4). During the growth process of shiitake mycelium, extracellular enzymes such as cellulase, hemicellulase, and ligninase are secreted, playing a crucial role in degrading the lignocellulosic components present in the substrate (37). Lignin is tightly bound within the cell wall of lignocellulose materials, serving as a protective shield for cellulose and hemicellulose, making them less vulnerable to degradation by enzymes (38). Therefore, mushrooms must first decompose lignin before proceeding to degrade cellulose (including hemicellulose and cellulose) (39, 40). The higher the proportion of lignin in the initial substrate mixture, the lower the bioavailability of that substrate (41, 42).
In light of the previous remarks, the study demonstrated that a 10% proportion of KP led to the most rapid mycelium development compared to OS. Moreover, both quicker mycelial spread and fruiting body formation were achieved on the substrate containing 10% KP, 10% LBP, and 10% HRP, in contrast to those on substrates with only 10% LBP or 10% HRP. This phenomenon may be attributed to the presence of 10% KP in these substrates. Particularly, compared to the substrate containing 10% KP, the browning of mycelium and the formation of primordium of Lentinus edodes were delayed by 10 days and 10 days, respectively, on the substrate with 10% LBP. Moreover, relative to the substrate with 10% KP, these processes were delayed by 6 days for mycelium browning and 10 days for primordium formation on the substrate with 10% HRP. It is evident that the physical environment for mycelial growth is dependent on the specific characteristics of the substrate. Previous studies have highlighted the significant role that KP plays as a substrate component in mushroom cultivation (22, 24, 43). In the context of KP substrates, their structural characteristics mitigate the compaction effect that occurs during mycelial growth compared to substrates composed of sawdust. This reduction in compaction facilitates the hydration process of the KP substrates through regular irrigation (44). Furthermore, in contrast to oak sawdust commonly employed in commercial production, the fruiting bodies of shiitake mushrooms could be harvested 2.1 days earlier from the substrate composed of 10% LBP and 10% HRP. This earlier harvest shortened the production cycle of shiitake mushrooms and consequently brought substantial economic benefits.
Generally, growers can harvest 0.3 kg to 0.5 kg of fresh shiitake mushrooms (with a biological efficiency ranging from 30 to 50%) from 1 kg of dry substrate (45). Except for the 10% LBP and 10% HRP, the biomass yields of the other tested substrates were similar, ranging from 201.34 g/kg for the substrate with 10% LBP and 10% HRP to 311.28 g/kg for oak sawdust. The corresponding bioefficiency fell within the range of 40.2 to 62.2% (Table 4). At the experimental scale, Yu et al. (39) documented a higher bioefficiency of 80.8% using oak sawdust. However, Leifa et al. (46) reported diverse bioefficiencies that varied from 78.4 to 85.8% across different lignocellulosic substrates. An important factor affecting the growth and fruiting of edible fungi is the carbon-to-nitrogen (C/N) ratio of the growth substrate (47, 48). It should be noted that the minimum C/N ratio necessary for the growth of shiitake mushrooms is 25:1, while the maximum C/N ratio is 55:1 (49). Desisa et al. (9) found that the total yield of shiitake mushrooms was relatively higher (20.96 mg/g) on substrates characterized by low carbon-to-nitrogen ratios. However, in the current experiment, higher total yields were obtained on substrates with higher C/N ratios. These basic findings align with the results of Sassine’s (50) research on growing shiitake mushrooms by utilizing oak acorns, vineyard pruning, and living pruning.
The nutritional content of fruiting bodies is strongly influenced by both the composition of the cultivation substrate (51–54) and the specific cultivated strain (55). The protein content of Qihe #1 shiitake mushrooms cultivated in the tested cultivation media ranged from 17.77 to 26.45% (Table 5).
This range was higher than the 13.7–19.6% and 12.4–17.2% reported by Desisa et al. (9) and Gaitan-Hernandez et al. (9), respectively, for shiitake mushrooms grown on agricultural waste, but lower than the 20–23% reported by Rahman and Choudhury (56). This range was higher than the 13.7–19.6% and 12.4–17.2% reported by Desisa et al. (9) and Gaitan-Hernandez et al. (9) for shiitake mushrooms cultivated on agricultural waste and lower than the 20–23% reported by Rahman and Choudhury (56). The protein content in this study was similar to that of milk (25.2%) (57) and significantly higher than that of vegetables (58).
Elkanah et al. (59) reported that the high protein content of shiitake mushrooms cultivated in a culture medium supplemented with wheat bran may be due to the rich carbon and nitrogen levels in the medium. From a nutritional perspective, mushrooms are considered a valuable source of protein (53). This aligns with the hypothesis that mushrooms could serve as an effective substitute for meat, given that their nutritional value is comparable to that of numerous plant species (60, 61).
Currently, efforts are being made to explore protein sources that can satisfy the nutritional requirements of the world’s growing population (62). To date, a fitting approach has been suggested in which various wastes can be used to cultivate protein-rich mushrooms, thus enhancing the value of agro-forestry byproducts.
Mushroom fiber is mostly soluble and can be absorbed by the human intestine, while plant fiber mainly consists of cellulose or hemicellulose, which the human body cannot utilize (63). These fibers are beneficial to human health as they not only aid digestion but also act as prebiotics for gut microbes, helping to prevent disease (64). Furthermore, mushroom fibers such as chitin and β-glucan possess anti-obesity, anti-diabetes, and anti-hypertension properties. As a result, they are utilized in biomedicine for anti-inflammatory, anti-allergic, anti-cancer, and immunomodulatory applications (65, 66). The fiber content in mushrooms was enhanced in the tested substrates compared to OS, peaking at 10.26% in the substrate with 30% KP (Table 5). Moreover, this component was significantly higher in the substrate with 10% HRP compared to those with 30% LBP, 10% LBP-10% HRP, and 10% KP-10% LBP-10% HRP, which had fiber contents of 9.97, 9.66, and 9.83%, respectively. In contrast, shiitake mushrooms grown exclusively on rice straw exhibited the lowest crude fiber content at 1.5% (13). Producing fiber-rich mushrooms can meet the health and nutritional needs of various consumers, allowing them to be transformed into numerous innovative food products within the industry, thereby broadening the application range of mushroom products. The fat content of shiitake mushrooms cultivated in the 10 tested substrates was significantly lower, ranging from 0.45 to 0.91%, which is well below the 3–4% levels previously reported by Rahman and Choudhury (56). This suggests that shiitake mushrooms grown in these substrates are a low-calorie food source. The role of fat in the human body includes generating energy for muscles and bodily functions, as well as aiding in the digestion and absorption of nutrients (59). Therefore, the high nutritional value of shiitake mushrooms, which contain very little fat (less than 1.22%) and no cholesterol, has attracted global interest in this macro fungus (12, 67).
One of the most notable characteristics of shiitake mushrooms is their remarkable ability to enhance flavor, a quality attributed to the presence of volatile and non-volatile components in the fruiting bodies (68). The levels of sweet and aromatic amino acids in the 20%KP group increased significantly compared to the control group, with an increase ranging from 0.529 to 14.527 (Table 6). The presence of amino acids such as glutamic acid (Glu), aspartic acid (Asp), and monosodium glutamate (MSG) contributes to the perception of a salty flavor, while specific amino acid sequences such as Cys-Met, Glu-Pro-Glu, and Gly-Cys-Gly are crucial for the umami flavor of shiitake mushrooms (69–72). After LBP was added to the culture medium, the methionine content in the fruiting body increased remarkably. In particular, the content with the addition of 10% LBP was 5.79 times that of the control group. Methionine is a sulfur-containing amino acid that serves as a vital raw material in synthesizing the flavor of cooked meat through the Maillard reaction (73). These amino acids contribute to the overall taste experience and selectivity of shiitake mushrooms, making them a valuable ingredient in various culinary applications and food preparations.
Soluble sugar is one of the key substances that contribute to the flavor of shiitake mushrooms. It can interact with compounds such as amino acids and influence the development of sweet and caramel flavors during processing (68). The results showed that trehalose, arabinose, and glucose were the primary soluble sugars in the 10 tested mushrooms (Table 7). However, the total soluble sugar levels (19.11–48.94 mg/g) of the tested shiitake mushrooms in this study were significantly lower than the 78.65–126.1 mg/g reported by Chen et al. (74) and the 82.98–127.30 mg/g reported by Li et al. (75). The reasons for this difference may include the different strains of shiitake mushrooms and variations in cultivation substrates. During the growth of edible fungi, organic acids are closely associated with the metabolic processes involved in synthesizing phenols, amino acids, esters, and aromatic compounds. To some extent, the types and amounts of organic acids influence the formation of the unique flavor of the fruiting body (68). It was found that the primary organic acids in the 10% LBP, 20% LBP, 10% KP, 10% LBP-10% HRP, and 10% KP-10% LBP-10% HRP groups were formic acid, citric acid, and fumaric acid. However, the main organic acids in the 20% KP, 30% KP, and control groups were formic acid, malic acid, and citric acid. Additionally, butyric acid was identified as one of the major organic acids in the 30% LBP group (Table 7). However, a previous study identified succinic acid as the main organic acid in shiitake mushrooms at different growth stages (75). Yang et al. reported that citric acid was the main organic acid in shiitake mushrooms, with a content of 24.45 mg/g (76). Gao et al. cultivated shiitake mushrooms using straw and found that malic acid was the main organic acid (12). Our results suggest that agro-forest waste used as a substitute for sawdust has an impact on the organic acid compounds present in cultivated shiitake mushrooms.
Two exploratory data analysis methods, including principal component analysis (PCA) and membership function methods, were used to comprehensively assess the similarities and differences in the nutritional profiles of shiitake mushrooms grown on different substrates. The data presented in Tables 8, 9 demonstrate that the nutritional value of shiitake mushrooms is attributed to macromolecular nutrients, as well as volatile and non-volatile flavor components and other small molecules. Given this function and the vast amount of data generated, the statistical analysis method was employed after standardizing the data to explore and reveal the hidden relationships between the samples (77). Through factor transformation, PC1 was identified as primarily related to amino acid content; thus, it has also been referred to as the amino acid high-sensing factor. PC2 was mainly associated with soluble sugar content and can be designated as the sweetness factor. However, PC3 was mainly associated with the organic acid content and can be termed the organic acid perception factor (Figure 2). The comprehensive evaluation of factors and their rankings was ultimately derived through regression estimation. The results demonstrated that the treatment groups of 20%KP, 10%LBP, and 10%KP-10%LBP-10%HRP ranked among the top three. The amino acid perception factor (PC1) was dominant, while the non-volatile taste perception characteristics (PC3) presented an unacceptable risk. This further underscores the importance of amino acids in enhancing the taste and flavor selectivity of shiitake mushrooms.
Generally speaking, the market price for each ton of OS is 700 RMB, translating to a sawdust cost of 0.546 RMB per stick for cultivating L. edodes with the CK formula. Typically, a greenhouse (667 m2) can accommodate up to 8,000 mushroom sticks, leading to a total sawdust cost of 4,368 RMB for the greenhouse. By substituting OS with agro-forest waste for mushroom cultivation, we can save nearly half the cost of OS. Specifically, the 10%KP-10%LBP-10%HRP formulation used in mushroom cultivation results in a significant cost reduction, with sawdust for this formulation priced at 2,688 RMB. Notably, this method results in a saving of 1,680 RMB per greenhouse compared to the control. Furthermore, using the 10%KP-10%LBP-10%HRP formula for cultivating L. edodes in a greenhouse requires 2,400 kg of agro-forest waste, decreasing environmental pollution from these forest wastes. At the same time, the 2,400 kg of agro-forest waste can yield 3,500 kg of fresh shiitake mushrooms, expected to generate 35,000 RMB in economic benefits (calculated with 44% biological efficiency). Therefore, utilizing forest waste for mushroom cultivation and converting waste into economic value not only reduces environmental impact but also enhances resource recycling. Additionally, mushroom cultivation can generate employment opportunities in rural areas, increase farmers’ income levels, improve living conditions, and promote local economic development.
An appropriate substrate formulation is indeed a prerequisite for achieving high yields of shiitake mushrooms. Various forestry waste branches have a significant impact on the growth of shiitake mushrooms. In areas planted with Lycium, Caragana korshinsk, and Hippophae rhamnoides, it is advisable to utilize a substrate matrix formed by mixing oak sawdust with waste forestry branch residues. This approach leverages locally available resources and can potentially provide a suitable environment for growing shiitake mushrooms. The optimal substrate formulation has been identified as containing 20% KP, 58% OS, 20% bran, 1% sucrose, and 1% light calcium carbonate. The second-best formulation consists of 10% LBP, 68% OS, 20% bran, 1% sucrose, and 1% light calcium carbonate. Furthermore, the substrate mixture comprising 10% KP, 10% LBP, 10% HRP, 48% OS, 20% bran, 1% sucrose, and 1% light calcium carbonate has demonstrated excellent performance regarding growth rate, yield, and nutritional quality of shiitake mushroom mycelium. This combination can offer a balanced mix of nutrients, proper moisture-holding capacity, and good aeration, which are all essential for the healthy development of mycelium and the subsequent fruiting of shiitake mushrooms. The findings of this study open up new possibilities for better utilizing these residues, which are often abundant in certain areas, enabling mushroom growers to potentially increase their productivity and improve the overall quality of the mushrooms they produce, thereby gaining a competitive edge in the market and realizing greater economic benefits. Further research into how to enhance the yield of shiitake mushrooms cultivated with waste, shorten the cultivation period, and conduct large-scale experiments across different regions and environments will be worthy pursuits based on existing research.
The raw data supporting the conclusions of this article will be made available by the authors without undue reservation.
HX: Conceptualization, Formal analysis, Funding acquisition, Methodology, Writing – original draft, Writing – review & editing. GH: Resources, Software, Visualization, Writing – review & editing. YL: Data curation, Formal analysis, Investigation, Methodology, Validation, Writing – original draft. QM: Conceptualization, Data curation, Investigation, Methodology, Writing – original draft. YZ: Investigation, Resources, Software, Validation, Visualization, Writing – original draft. YW: Conceptualization, Project administration, Resources, Supervision, Writing – review & editing. SL: Funding acquisition, Supervision, Writing – review & editing.
The author(s) declare that financial support was received for the research and/or publication of this article. This research was funded by Qinghai Natural Science Foundation Youth Fund Project (grant number 2023-ZJ-968Q).
We appreciate the support provided by the Qinghai Academy of Agriculture and Forestry Sciences.
GH was employed by the company Qinghai Water Conservancy and Hydropower Survey and Design Institute Co., Ltd.
The remaining authors declare that the research was conducted in the absence of any commercial or financial relationships that could be construed as a potential conflict of interest.
The author(s) declare that no Gen AI was used in the creation of this manuscript.
All claims expressed in this article are solely those of the authors and do not necessarily represent those of their affiliated organizations, or those of the publisher, the editors and the reviewers. Any product that may be evaluated in this article, or claim that may be made by its manufacturer, is not guaranteed or endorsed by the publisher.
The Supplementary material for this article can be found online at: https://www.frontiersin.org/articles/10.3389/fnut.2025.1538039/full#supplementary-material
SUPPLEMENTARY FIGURE S1 | Significant correlations analysis between cultivation substrates and quality indicators of L. edodes.
1. Bhambri, A, Srivastava, M, Mahale, VG, Mahale, S, and Karn, SK. Mushrooms as potential sources of active metabolites and medicines. Front Microbiol. (2022) 13:837266. doi: 10.3389/fmicb.2022.837266
2. Imam, KMSU, Xie, Y, Liu, Y, Wang, F, and Xin, F. Extraction, isolation, and identification of cytotoxic secondary metabolites from shiitake mushroom 808 Lentinula edodes (Berk.). ACS. Food Sci Technol. (2021) 1:551–8. doi: 10.1021/acsfoodscitech.0c00059
3. Royse, DJ, Baars, J, and Tan, Q. Current overview of mushroom production in the world In: DC Zield and A Pardo-Gimenez, editors. Edible and medicinal mushrooms: Technology and applications. Wiley. (2017). 5–13.
4. Chung, IM, Kim, SY, Han, JG, Kong, WS, Jung, MY, and Kim, SH. Fatty acids and stable isotope ratios in shiitake mushrooms (Lentinula edodes) indicate the origin of the cultivation substrate used: a preliminary case study in Korea. Food Secur. (2020) 9:9. doi: 10.3390/foods9091210
5. Cao, B, Zhang, Y, and Gao, B. Development history, current situation, and trends of global shiitake mushroom industry. Edible Fungi China. (2024) 31:1–20.
6. Menolli, M, Sanchez-Ramírez, S, Sanchez-García, M, and Wang, C. Global phylogeny of the shiitake mushroom and related Lentinula species uncovers novel diversity and suggests an origin in the Neotropics. Mol Phylogenet Evol. (2022) 173:107494. doi: 10.1016/j.ympev.2022.107494
7. Gong, D. The study on white Hypsizygus Marmoreus cultivation with waste vine stalks. Yangling City, Shaanxi province, China: Master's Thesis, Northwest A & F University. (2014).
8. Jeffries, TW. Biodegradation of lignin and hemicelluloses In: C Ratledge, editor. Biochemistry of microbial degradation. Springer, Dordrecht: The Netherlands (1994). 233–77.
9. Desisa, B, Muleta, D, Dejene, T, Jida, M, Goshu, A, and Martin-Pinto, P. Substrate optimization for shiitake (Lentinula edodes (Berk.) Pegler) mushroom production in Ethiopia. J Fungi (Basel). (2023) 9:811. doi: 10.3390/jof9080811
10. Avila, IAF, da Silva Alves, L, and Zied, DC. Bioconversion of rice straw by Lentinula edodes under different spawn formulations. Braz J Microbiol. (2023) 54:3137–46. doi: 10.1007/s42770-023-01116-w
11. Abdullah, MB, Abed, IA, and Alkobaisy, JS. Effect of different substrates and supplement with three types of spawn on Letinula edodes parameters for first production in Iraq. IOP Conf Ser Earth Environ Sci. (2022) 1060:012060. doi: 10.1088/1755-1315/1060/1/012060
12. Gao, S, Huang, Z, Feng, X, Bian, Y, Huang, W, and Liu, Y. Bioconversion of rice straw agro-residues by Lentinula edodes and evaluation of non-volatile taste compounds in mushrooms. Sci Rep. (1814) 10:1. doi: 10.1038/s41598-020-58778-x
13. Kumla, J, Suwannarach, N, Sujarit, K, Penkhrue, W, Kakumyan, P, Jatuwong, K, et al. Cultivation of mushrooms and their lignocellulolytic enzyme production through the utilization of agro-industrial waste. Molecules. (2020) 25:2811. doi: 10.3390/molecules25122811
14. Mou, C, Gong, Y, Chen, L, Martin, F, Kang, H, and Bian, Y. Comparative analysis of simulated in-situ colonization and degradation by Lentinula edodes on oak wafer and corn stalk. Front Microbiol. (2023) 14:1286064. doi: 10.3389/fmicb.2023.1286064
15. Zhang, Y, Li, Q, Duan, L, Ding, J, Li, Y, Wang, Y, et al. Effects of different orchard tree pruning residues on the yield and nutrient composition of Lentinus edodes. Front Nutr. (2024) 11:1477586. doi: 10.3389/fnut.2024.1477586
16. Baktemur, G, Kara, E, Yarar, M, Yilmaz, N, Agçam, E, Akyildiz, A, et al. Yield, quality, and enzyme activity of shiitake mushroom (Lentinula edodes) grown on different agricultural wastes. Not Bot Horti Agrobot Cluj-Napoca. (2022) 50:12553. doi: 10.15835/nbha50112553
17. Su, HR. A strategy investigation on sustainable development of China's deciduous fruit production. Deciduous Fruit. (2012) 1:1–4.
18. Li, W, Chen, WC, Wang, JB, Feng, J, Wu, D, Zhang, Z, et al. Effects of enzymatic reaction on the generation of key aroma volatiles in shiitake mushroom at different cultivation substrates. Food Sci Nutr. (2021) 9:2247–56. doi: 10.1002/fsn3.2198
19. Wang, Y, Ma, Q, Li, Y, Sun, T, Jin, H, Zhao, C, et al. Energy consumption, carbon emissions and global warming potential of wolfberry production in Jingtai oasis, Gansu province. China Environ Manage. (2019) 6:772–82. doi: 10.1007/s00267-019-01225-z
20. Wang, Y, Jin, H, Dong, X, Yang, S, Ma, S, and Ni, J. Quality evaluation of Lycium barbarum (wolfberry) from different regions in China based on polysaccharide structure, yield and bioactivities. Chin Med. (2019) 14:49. doi: 10.1186/s13020-019-0273-6
21. Zhao, Y, Yao, Y, Li, H, Han, Z, and Ma, X. Integrated transcriptome and metabolism unravel critical roles of carbon metabolism and oxidoreductase in mushroom with Korshinsk peashrub substrates. BMC Genomics. (2024) 25:1. doi: 10.1186/s12864-023-09893-2
22. Zou, Y, Du, F, Zhang, H, and Hu, Q. Evaluation of Korshinsk Peashrub (Caragana korshinskii Kom.) as a substrate for the cultivation of Pleurotus Eryngii. Waste Biomass Valor. (2019) 10:2879–85. doi: 10.1007/s12649-018-0301-2
23. Mei, D, Ma, X, Fu, F, and Cao, F. Research status and development prospects of sea buckthorn (Hippophae rhamnoides L.) resources in China. Forests. (2023) 14:2461. doi: 10.3390/f14122461
24. Du, F, Qu, J, Hu, Q, Yuan, X, Yin, G, Wang, L, et al. Maximizing the value of Korshinsk peashrub branches by the integration of Pleurotus tuoliensis cultivation and anaerobic digestion of spent mushroom substrate. Renew Energy. (2021) 179:679–86. doi: 10.1016/j.renene.2021.07.053
25. Qin, J. Effects of several forest and fruit plant waste and CaSO4 on mycelium growth of Lentinula edodes. Master's thesis, Nanjing Agricultural University, (2021).
26. Ma, RH, Zhang, XX, Ni, ZJ, Thakur, K, Wang, W, Yan, YM, et al. Lycium barbarum (goji) as functional food: a review of its nutrition, phytochemical structure, biological features, and food industry prospects. Crit Rev Food Sci Nutr. (2023) 30:10621–35. doi: 10.1080/10408398.2022.2078788
27. Miranda, MR, Vestuto, V, Amodio, G, Manfra, M, Pepe, G, and Campiglia, P. Antitumor mechanisms of Lycium barbarum fruit: an overview of in vitro and in vivo potential. Life (Basel). (2024) 14:420. doi: 10.3390/life14030420
28. Qiang, X, Xia, T, Geng, B, Zhao, M, Li, X, Zheng, Y, et al. Bioactive components of Lycium barbarum and deep-processing fermentation products. Molecules. (2023) 28:8044. doi: 10.3390/molecules28248044
29. Teixeira, F, Silva, AM, Delerue-Matos, C, and Rodrigues, F. Lycium barbarum berries (Solanaceae) as source of bioactive compounds for healthy purposes: a review. Int J Mol Sci. (2023) 5:4777. doi: 10.3390/ijms24054777
30. Ren, A, Bao, Y, Han, A, Li, T, Liu, M, Wang, X, et al. Optimization of Lentinula edodes spawn medium formula based on D-optimal method. J Cold-Arid Agricul Sci. (2024) 3:724–33. doi: 10.3969/j.issn.2097-2172.2024.08.006
31. Song, TT, Shen, YY, Xu, F, Fan, LJ, and Cai, WM. Hub gene of Lentinula edodes at different developmental stages identified by weighted gene coexpression network analysis. Mycosystema. (2021) 7:1761–75. doi: 10.13346/j.mycosystema.200411
32. National health and family planning commission of the People's Republic of China. National food safety standard: determination of fat in foods. GB5009.6–2016. China: Standardization Administration of China (2016).
33. Ministry of health of the People's Republic of China. Determination of crude fiber in vegetable foods. GB/T 5009.10-2003. China: Standardization Administration of China (2003).
34. National health and family planning commission of the People's Republic of China. National food safety standard: determination of protein in foods. GB5009.5–2016. China: Standardization Administration of China (2016).
35. National health and family planning commission of the People's Republic of China. National food safety standard: fetermination of amino acids in foods. GB5009.124–2016. China: Standardization Administration of China (2016).
36. Chen, W, Li, W, Chen, H, Zhang, J, Tang, L, Wu, D, et al. Analysis and comprehensive evaluation of nutritionaland amino acid characteristics of Morchella cultivatedin Qinghai plateau. Acta Edulis Fungi. (2024) 31:64–75. doi: 10.16488/j.cnki.1005-9873.2024.02.008
37. Luz, JMR, Souza Lopes, L, Silva, MCS, Vieira, NA, Cardoso, WS, and Kasuya, MCM. Lentinula edodes lignocellulolases and lipases produced in Macaúba residue and use of the enzymatic extract in the degradation of textile dyes. 3 Biotech. (2023) 13:406. doi: 10.1007/s13205-023-03827-1
38. Badu, M, Twumasi, S, and Boadi, N. Effects of lignocellulosic in wood used as substrate on the quality and yield of mushrooms. FNS. (2011) 2:2780–4. doi: 10.4236/fns.2011.27107
39. Yu, G, Li, X, Zhao, S, Sun, S, Yu, Y, Chen, J, et al. Waste apple wood: a safe and economical alternative substrate for the cultivation of Pleurotus ostreatus and Lentinula edodes. Folia Hortic. (2022) 34:1–13. doi: 10.2478/fhort-2022-0014
40. Li, Z, Zhao, C, Zhou, Y, Zheng, S, Hu, Q, and Zou, Y. Label-free comparative proteomic analysis of Pleurotus eryngii grown on sawdust, bagasse, and peanut shell substrates. J Proteome. (2024) 294:105074. doi: 10.1016/j.jprot.2024.105074
41. Abou Fayssal, S, El Sebaaly, Z, Alsanad, MA, Najjar, R, Ohme, MB, Yordanova, MH, et al. Combined effect of olive pruning residues and spent coffee grounds on Pleurotus ostreatus production, composition, and nutritional value. PLoS One. (2021) 16:e0255794. doi: 10.1371/journal.pone.0255794
42. Haug, RT. Chapter 1: introduction In: Haug RT, Editor. The practical handbook of compost engineering. New York: Lewis Publishers (1993). 1–19.
43. Zhang, J, Ren, A, Chen, H, Zhao, M, Shi, L, Chen, M, et al. Transcriptome analysis and its application in identifying genes associated with fruiting body development in basidiomycete Hypsizygus Marmoreus. PLoS One. (2015) 10:e0123025. doi: 10.1371/journal.pone.0123025
44. Zied, DC, Silva Freitas, MA, Almeida Moreira, BR, Silva Alves, L, and Pardo-Giménez, AA. Comparative analysis of biodegradation and bioconversion of Lentinula edodes and other exotic mushrooms. Microorganisms. (2023) 11:897. doi: 10.3390/microorganisms11040897
45. Chen, AW. Shiitake bag cultivation, MushWorld In: Christopher H, Editor. Mushroom growers’ handbook 2: Shiitake cultivation. Seoul, Korea: Mush World (2005). 60–86.
46. Leifa, F, Pandey, A, and Soccol, CR. Solid state cultivation-an efficient method to use toxic agro-industrial residues. J Basic Microbiol. (2000) 40:187–97. doi: 10.1002/1521-4028(200007)40:3<187::AID-JOBM187>3.0.CO;2-Q
47. Valenzuela-Cobos, JD, Guevara-Viejó, F, Grijalva-Endara, A, Vicente-Galindo, P, and Galindo-Villardón, P. Multivariate statistical techniques: a new approach to identify the commercial properties of mixtures of flours of Lentinula edodes and cocoa pod husk. J Fungi (Basel). (2023) 9:991. doi: 10.3390/jof9100991
48. Kumar, P, Eid, EM, Al-Huqail, AA, Širić, I, Adelodun, B, Abou Fayssal, S, et al. Kinetic studies on delignification and heavy metals uptake by shiitake (Lentinula edodes) mushroom cultivated on agro-industrial wastes. Horticulturae. (2022) 8:316. doi: 10.3390/horticulturae8040316
49. Zied, D.C., Savoie, J.M., and Pardo-Gimenez, A. Soybean the main nitrogen source in cultivation substrates of edible and medicinal mushrooms, in: H. ShamyEl (Ed.), Soybean and nutrition. InTech. (2011), 12–22.
50. Sassine, YN, Nabhan, S, Rachkidy, E, and El Sebaaly, Z. Valorization of agro-forest wastes (oak acorns, vineyard pruning, and olive pruning) through the cultivation of shiitake (Lentinula edodes) mushrooms. Heliyon. (2024) 10:e32562. doi: 10.1016/j.heliyon.2024.e32562
51. Alsanad, MA, Sassine, YN, El Sebaaly, Z, and Abou Fayssal, S. Spent coffee grounds influence on Pleurotus ostreatus production, composition, fatty acid profile, and lignocellulose biodegradation capacity. CyTA-J Food. (2021) 19:11–20. doi: 10.1080/19476337.2020.1845243
52. Sassine, YN, Naim, L, El Sebaaly, Z, Abou Fayssal, S, and Alsanad, MA. Nano urea effects on Pleurotus ostreatus nutritional value depending on the dose and timing of application. Sci Rep. (2021) 11:e5588. doi: 10.1038/s41598-021-85191-9
53. Sassine, YN, Shuleva, N, and El Sebaaly, Z. Changes in Pleurotus ostreatus nutritional value and heavy metal profile as a result of supplementation with nanoadditive. Stud Fungi. (2022) 7:1–6. doi: 10.48130/SIF-2022-0012
54. Aubrey, MLL, Chin, CFS, Seelan, JSS, Chye, FY, Lee, HH, and Rakib, MRM. Metabolites profiling of protein enriched oyster mushroom (Pleurotus ostreatus (Jacq.) P. Kumm.) grown on oil palm empty fruit bunch substrate. LWT-Food Sci Technol. (2023) 181:114731. doi: 10.1016/j.lwt.2023.114731
55. Onyeka, EU, and Okehie, MA. Effect of substrate media on growth, yield, and nutritional composition of domestically grown oyster mushroom (Pleurotus ostreatus). African J Plant Sci. (2018) 12:141–7. doi: 10.5897/AJPS2016.1445
56. Rahman, T, and Choudhury, M. Shiitake mushroom: a tool of medicine. Bangladesh J Med Biochem. (2013) 5:24–32. doi: 10.3329/bjmb.v5i1.13428
57. Neeraj, A, Jarial, RS, Jarial, K, and Bhatia, JN. Comprehensive review on oyster mushroom species (Agaricomycetes): morphology, nutrition, cultivation and future aspects. Heliyon. (2024) 10:e26539. doi: 10.1016/j.heliyon.2024.e26539
58. Butnariu, M, and Butu, A. Chemical composition of vegetables and their products In: PCK Cheung, editor. Handbook of food chemistry. Berlin Heidelberg, Berlin, Heidelberg: Springer (2014)
59. Elkanah, FA, Oke, MA, and Adebayo, EA. Substrate composition effect on the nutritional quality of Pleurotus ostreatus (MK751847) fruiting body. Heliyon. (2022) 8:e11841. doi: 10.1016/j.heliyon.2022.e11841
60. Castellanos-Reyes, K, Villalobos-Carvajal, R, and Beldarrain-Iznaga, T. Fresh mushroom preservation techniques. Food Secur. (2021) 10:21–6. doi: 10.3390/foods10092126
61. Aubrey, MLL, Chin, CFS, Seelan, JSS, Chye, FY, Lee, HH, and Rakib, MRM. Conversion of oil palm by-products into value-added products through oyster mushroom (Pleurotus ostreatus) cultivation. Horticulturae. (2022) 8:1040. doi: 10.3390/horticulturae8111040
62. Avelar, Z, Rodrigues, RM, Ricardo, N, Pereira, RN, and Vicente, AA. Future food proteins-trends and perspectives In: R Bhat, editor. Future foods. Cambridge: Academic Press (2022). 267–85.
63. Cheung, PCK. Mini-review on edible mushrooms as source of dietary fiber: preparation and health benefits. Food Sci Human Wellness. (2013) 2:162–6. doi: 10.1016/j.fshw.2013.08.001
64. Li, S, Wang, A, Liu, L, Tian, G, Wei, S, and Xu, F. Evaluation of nutritional values of shiitake mushroom (Lentinus edodes) stipes. J Food Meas Charact. (2018) 12:2012–9. doi: 10.1007/s11694-018-9816-2
65. Mandaliya, D, Patel, S, and Seshadri, S. Fiber in our diet and its role in health and disease In: V Rani and U Yadav, editors. Functional food and human health. Singapore: Springer (2018)
66. Bhoite, R, Satyavrat, V, and Premasudha Sadananda, M. Clinical benefits of β-glucan supplementation in children: a review. Discov Food. (2022) 2:37–8. doi: 10.1007/s44187-022-00038-0
67. Bich Thuy Thi, N, Ve Van, L, Huyen Trang Thi, N, Luyen Thi, N, Thuy Trang Thi, T, and Nghien Xuan, N. Nutritional requirements for the enhanced mycelial growth and yield performance of Trametes versicolor. J Appl Biol Biotechnol. (2021) 1:1–7. doi: 10.7324/JABB.2021.9101
68. Chen, W, Li, W, Wu, D, Zhang, Z, Zhou, S, Yan, Y, et al. Determination of soluble sugar and organic acid components and evaluation of taste quality of 11 main cultivars of Lentinula edodes. Sci Technol Food Ind. (2022) 7:273–9. doi: 10.13386/j.issn1002-0306.2020110296
69. Li, Y, Han, J, Yarley, OPN, Wang, Y, Wang, Y, Zhang, A, et al. Effects of combined drying techniques and cellulase hydrolysis on the nutritional value and sensory properties of shiitake mushrooms (Lentinus edodes). Food Chem. (2024) 450:139387. doi: 10.1016/j.foodchem.2024.139387
70. Harada-Padermo, SDS, Dias-Faceto, LS, Selani, MM, Alvim, ID, Floh, EIS, Macedo, AF, et al. Umami ingredient: flavor enhancer from shiitake (Lentinula edodes) by products. Food Res Int. (2020) 137:109540. doi: 10.1016/j.foodres.2020.109540
71. Liu, Q, Hu, S, Song, Z, Cui, X, Kong, W, Song, K, et al. Relationship between flavor and energy status in shiitake mushroom (Lentinula edodes) harvested at different developmental stages. J Food Sci. (2021) 86:4288–302. doi: 10.1111/1750-3841.15904
72. Gao, J, Li, X, Jia, S, Zeng, H, and Zheng, B. Structural characterization and antioxidant activity of a glycoprotein isolated from shiitake mushrooms. Food Biosci. (2023) 53:102608. doi: 10.1016/j.fbio.2023.102608
73. Chen, D, Sheng, M, Wang, S, Chen, X, Leng, A, and Lin, S. Dynamic changes and formation of key contributing odorants with amino acids and reducing sugars as precursors in shiitake mushrooms during hot air drying. Food Chem. (2023) 30:136409. doi: 10.1016/j.foodchem.2023.136409
74. Chen, W, Li, W, Yang, Y, Yu, H, Zhou, S, Feng, J, et al. Analysis and evaluation of tasty components in the pileus and stipe of Lentinula edodes at different growth stages. J Agric Food Chem. (2015) 3:795–801. doi: 10.1021/jf505410a
75. Li, W, Chen, W, Yang, Y, Zhang, J, Feng, J, Yu, H, et al. Effects of culture substrates on taste component content and taste quality of Lentinula edodes. Int J Food Sci Technol. (2017) 52:981–91. doi: 10.1111/ijfs.13362
76. Yang, Y, Gu, Z, Liu, Y-F, Zhou, S, and Zhang, JS. Determination of seven organic acids in edible fungi by reversed-phase high performance liquid chromatography. Mycosystema. (2013) 32:1064–70.
Keywords: agro-forest waste, shiitake mushroom, alternative substrates, nutritional value, principal component analysis
Citation: Xu H, Han G, Li Y, Meng Q, Zhang Y, Wang Y and Li S (2025) Enhancing yield and quality: research and practice of agro-forest waste for Lentinus edodes (shiitake mushroom) cultivation. Front. Nutr. 12:1538039. doi: 10.3389/fnut.2025.1538039
Received: 02 December 2024; Accepted: 04 March 2025;
Published: 19 March 2025.
Edited by:
Jelena Vladic, NOVA University of Lisbon, PortugalReviewed by:
Tiziana Nardin, Fondazione Edmund Mach, ItalyCopyright © 2025 Xu, Han, Li, Meng, Zhang, Wang and Li. This is an open-access article distributed under the terms of the Creative Commons Attribution License (CC BY). The use, distribution or reproduction in other forums is permitted, provided the original author(s) and the copyright owner(s) are credited and that the original publication in this journal is cited, in accordance with accepted academic practice. No use, distribution or reproduction is permitted which does not comply with these terms.
*Correspondence: Hongyan Xu, aG9uZ3lhbnh1MjAyMEAxMjYuY29t; Songling Li, NDY1ODgzMTE0QHFxLmNvbQ==
†These authors have contributed equally to this work
Disclaimer: All claims expressed in this article are solely those of the authors and do not necessarily represent those of their affiliated organizations, or those of the publisher, the editors and the reviewers. Any product that may be evaluated in this article or claim that may be made by its manufacturer is not guaranteed or endorsed by the publisher.
Research integrity at Frontiers
Learn more about the work of our research integrity team to safeguard the quality of each article we publish.