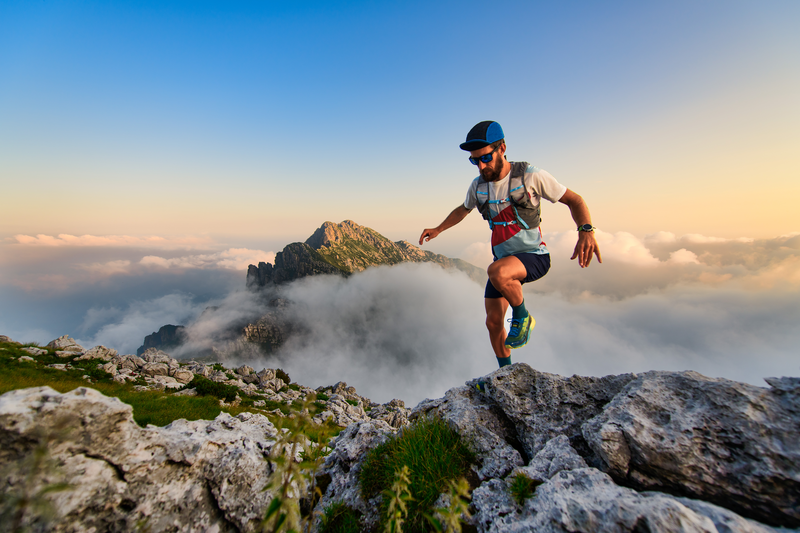
95% of researchers rate our articles as excellent or good
Learn more about the work of our research integrity team to safeguard the quality of each article we publish.
Find out more
ORIGINAL RESEARCH article
Front. Nutr. , 14 March 2025
Sec. Nutritional Epidemiology
Volume 12 - 2025 | https://doi.org/10.3389/fnut.2025.1526879
Background: Sarcopenia, the accelerated loss of muscle mass and function, is commonly associated with aging, especially in older adults. While low serum calcium has been linked to muscle loss in individuals over 50, its relationship with sarcopenia in younger adults (20–60 years) is unclear. This study examines this association using data from the National Health and Nutrition Examination Survey (NHANES) from 2011 to 2018.
Methods: This population-based, cross-sectional study analyzed participants aged 20–60 from NHANES 2011–2018. Individuals with missing data were excluded. Sarcopenia was assessed using appendicular skeletal muscle (ASM) measured by dual-energy X-ray absorptiometry, defined by ASM/BMI or ASM/Weight. Multiple logistic regression and stratified analyses were used to explore the correlation between serum calcium levels and sarcopenia prevalence.
Results: Among 7,309 adults, 578 (7.91%) had sarcopenia by ASM/BMI and 1,363 (18.65%) by ASM/Weight. Higher serum calcium levels were significantly associated with a lower risk of sarcopenia (ASM/BMI: OR 0.07, 95% CI 0.02–0.20; ASM/Weight: OR 0.09, 95% CI 0.04–0.19). The trend was consistent across age, gender, education, poverty income ratio, and race, with some exceptions.
Conclusion: This study highlights a negative association between serum calcium and sarcopenia risk in young and middle-aged adults, suggesting that calcium interventions could be beneficial in preventing sarcopenia in this population. However, the cross-sectional design precludes any inference of causality, and further longitudinal studies are warranted to confirm these findings.
Sarcopenia is a condition characterized by the accelerated loss of muscle mass and function, occurring in the context of a progressive and widespread skeletal muscle disorder (1). The prevalence of sarcopenia is expected to quadruple from 50 to over 200 million cases in the next 40 years (2). This condition is linked to increased risks of mortality, fractures, hypertension, diabetes mellitus (DM), disability, and the need for hospitalization or institutionalization (1, 3–6), imposing a significant burden on healthcare finances.
Recent studies further indicate that the onset of sarcopenia is influenced by diverse factors, including demographic variables, diabetes mellitus, hypertension, and chronic kidney disease (7–10). Additionally, obesity and systemic inflammation have been implicated in exacerbating muscle deterioration and elevating metabolic risks. Although sarcopenia has traditionally been viewed as predominantly affecting older adults (1), emerging evidence shows that it can manifest earlier in life (11). For example, a notably high prevalence of sarcopenia has been documented among young and middle-aged South Korean individuals (12, 13). Moreover, hospitalization costs linked to sarcopenia are often more significant in younger adults compared to those aged 65 and older (14). Young and middle-aged adults with sarcopenia face a heightened risk of developing metabolic disorders, experiencing reduced physical function, and enduring long-term disability, highlighting the need for early intervention (15, 16). Despite this, the underlying factors contributing to sarcopenia in this population remain poorly understood and warrant further investigation. Therefore, this study focuses on identifying risk factors for sarcopenia in young and middle-aged adults (20–60 years) to develop early interventions that may reduce the long-term health impacts of muscle loss, including metabolic disorders and disability.
In young adults and middle aged adults, skeletal muscle displays higher plasticity compared to older individuals, which relies on the potential of muscle fibers to undergo structural changes and the composition of muscle protein isoforms (17). Within muscle fibers, calcium plays a pivotal role in multiple processes critical for muscle health: it not only facilitates excitation-contraction coupling by binding to troponin and regulating the actin-myosin cross-bridge cycle, but also acts as a critical signaling molecule in pathways such as mTOR and CaMK, which regulate muscle protein synthesis and degradation (18–22). Furthermore, calcium is indispensable for the proper function of calpains, which are calcium-dependent proteases involved in myogenesis and the turnover of muscle proteins (19, 21). Insufficient calcium levels may therefore disrupt these processes, leading to impaired muscle repair and regeneration, and potentially accelerating protein degradation pathways, thereby contributing to sarcopenic outcomes (23, 24). Previous studies have linked low serum calcium levels to skeletal muscle loss in older adults (≥50 years), demonstrating that reduced calcium intake predicts muscle loss (25, 26). However, limited research has examined this relationship in young and middle-aged adults.
This study collected data from NHANES 2011–2018, employing multiple logistic regression and stratified analysis to examine the correlation between serum calcium levels and the sarcopenia among adults aged 20–60 in the United States.
National Health and Nutrition Examination Survey (NHANES) is a cross-sectional survey conducted by the National Center for Health Statistics, which selects participants using a complex 4-stage probability sampling design. It gathers information on the health and nutritional status of the civilian, noninstitutionalized population in the United States, excluding those in correctional facilities or nursing homes. The NHANES (National Health and Nutrition Examination Survey) data utilized in this study were collected by the National Center for Health Statistics (NCHS). During data collection, informed consent was obtained from all participants, and the study protocols received approval from the NCHS Ethics Review Board. As this research involves secondary analysis of publicly available, de-identified data, it is exempt from additional ethical review or participant consent requirements.
Starting in 1999, NHANES collected and released data in 2-year cycles. Since 2007, several changes have been made to the over-sampled domains (27). Notably, the entire Hispanic population, rather than just Mexican Americans (MA), was oversampled. In addition, similar to the approach taken with those aged 60 and over in the previous cycle, Black individuals and low-income populations were oversampled to ensure accurate estimates for these specific groups (28). Due to the absence of skeletal muscle mass data between 2007 and 2010, our study selected the 2011–2012 cycle as the starting point for data inclusion. Furthermore, considering the potential unknown impacts of the COVID-19 pandemic on the data results, our research only incorporated NHANES data from 2011 to 2018.
Overall, between 2011 and 2018, a total of 39,156 subjects were initially included in the NHANES study. Among these, 17,879 subjects possessed complete data on skeletal muscle mass, of which 8,757 were aged 20–60 years old and were either male or non-pregnant females. Data were excluded if individuals were missing certain values, including 679 subjects lacking data on the ratio of family income to poverty (PIR), 423 subjects without data on alanine aminotransferase (ALT, U/L), and 432 subjects without data on aspartate aminotransferase (AST, U/L). Additionally, 422 subjects lacked data on cholesterol (refrigerated serum, mmol/L), 427 subjects were missing data on triglycerides (refrigerated serum, mmol/L), 470 subjects lacked data on diet calcium and diet phosphorus, 423 subjects had no data on serum phosphorus, and 439 subjects were without data on serum calcium (mmol/L). Some individuals had multiple missing data points. Ultimately, our study included 7,309 participants (Figure 1).
The NHANES database utilized Dual-energy X-ray absorptiometry (DXA) whole-body scans to assess appendicular skeletal muscle mass (ASM) (29). DXA scan was performed using Hologic QDR 4500A fan beam X-ray bone densitometer (Hologic, Inc., Bedford, MA). Original scan results were analyzed using Hologic Discovery software (version 12.1, Hologic, Inc.) to derive fat mass and lean mass. Body regions (head, arms, trunk, and legs) were delineated manually using tools provided by the software. Trunk region was defined as the area from the inferior edge of the chin as the upper borders to the oblique lines that cross the femoral necks and converge below the pubic symphysis as the lower perimeter, with vertical borders lateral to the ribs. The area below the lower borders of the trunk was defined as leg region (30).The calculation of ASM involved summing the lean soft tissue masses of both the arms and legs. Sarcopenia lacks a universally established definition (12, 31), and research has shown that different methodologies used to define sarcopenia can significantly impact study outcomes (32).
This study adopted two distinct criteria for defining sarcopenia: 1. According to the international consensus meeting of the National Institutes of Health (FNIH) (31), sarcopenia was determined by assessing the ASM/BMI ratio, with values less than 0.789 for men and less than 0.512 for women considered indicative of sarcopenia. 2. As an alternative definition, sarcopenia was identified based on an ASM/weight (in kilograms) ratio that fell 1 standard deviation below the sex-specific mean of a younger reference group aged 20–29 years. The cutoff values for this criterion were set at less than 0.32 for men and less than 0.25 for women (33).
Although functional assessments such as grip strength and gait speed are widely used in defining sarcopenia (34, 35), they were not included in this study due to data availability constraints. Specifically, grip strength data were only collected in the NHANES 2011–2014 cycles, and gait speed was not assessed in NHANES during the study period (2011–2018). To ensure consistency across the entire dataset, we used ASM adjusted for BMI and weight as proxies for sarcopenia (31, 33). While this approach effectively evaluates muscle mass-related sarcopenia, it may not fully capture the functional aspects of the condition. Future studies incorporating functional parameters would provide a more comprehensive assessment of sarcopenia.
In this study, our primary objective was to explore the correlation between serum calcium levels and the prevalence of sarcopenia. Given the recognized influence of dietary intake on blood calcium levels (36), we conducted a comprehensive evaluation of these components as part of our analysis. Furthermore, acknowledging the complex etiology of sarcopenia, we conducted an extensive review of the existing literature to identify several potential variables that could contribute to the occurrence of sarcopenia or calcium metabolism (29, 37–43). These variables, including Demographic Variables, DM, hypertension (HBP) and chronic kidney disease (CKD) were incorporated into our study. Detailed data collection methods for all these variables are provided below.
Age, sex, race, and education level were self-reported by participants (44, 45). BMI was calculated as a person’s weight in kilograms divided by the square of height in meters. The PIR serves as an indicator of family income in relation to the poverty threshold. It categorizes income levels into three groups: low-income (PIR ≤ 1.3), middle-income (PIR > 1.3–3.5), and high-income (PIR > 3.5) (42). Smoking status was defined based on self-report of having smoked at least 100 cigarettes in a participant’s lifetime (46). One is considered to be a drinking status if they meet any of the following criteria: 1. This includes individuals who consume at least two drinks per day for females or at least three drinks per day for males. 2. Individuals who engage in binge drinking on at least 2 days per month.3. Those with a history of daily binge drinking (47).
A diagnosis of DM is established if any of the following conditions are met: 1. Participants who responded affirmatively to the NHANES question, “Has a doctor or other health professional ever told you that you had DM?.” 2. DM was diagnosed if participants had fasting plasma glucose levels ≥7 mmol/L (equivalent to 125 mg/dL), reported a physician diagnosis of DM. 3. Participants who are currently using medication to lower blood glucose levels (28).
Hypertension diagnosis was established using three criteria: 1. Participants who responded affirmatively to the NHANES question, “Has a doctor ever told you that you have hypertension?.” 2. Participants with SBP greater than 140 mmHg or diastolic blood pressure (DBP) higher than 90 mmHg were classified as hypertensive. If multiple blood pressure readings were available, the average reading was used for diagnosis. 3. Participants were deemed hypertensive if they were currently taking medications such as calcium channel blockers (CCBs), beta blockers, diuretics, angiotensin-converting enzyme inhibitors, and/or angiotensin II receptor blockers (ACEIs/ARBs) (48).
The diagnosis of CKD was determined based on a combination of factors, which included: 1. Participants who were informed by a doctor or health professional that they had CKD. 2. Participants with an estimated glomerular filtration rate (eGFR) of less than 60 mL/min/1.73 m2. 3. Participants with a randomized urinary albumin/creatinine ratio (ACR) greater than 30 mg/g. The eGFR was calculated using the Modification of Diet in Renal Disease (MDRD) study equation, represented as follows: eGFR = 175 × standardized Scr^-1.154 × age^-0.203 × 1.212 [if Black] × 0.742 [if female], Where eGFR is expressed in mL/min/1.73 m2 of body surface area, and Scr (serum creatinine) is expressed in mg/dL (49).
Dietary calcium and phosphorus intake were assessed using a 24-h dietary recall interview, a well-established method for collecting detailed information on all foods and beverages consumed over the previous 24 h (50).
Blood samples were collected from participants after a fasting period of more than 8 h. These samples were processed on-site and subsequently shipped on dry ice to central laboratories, where they were stored at −70°C until analysis (51). Serum calcium levels, serum phosphorus levels, ALT and AST levels, cholesterol levels in refrigerated serum, and triglyceride levels in refrigerated serum were measured.
The study was conducted in accordance with the guidelines outlined in the Strengthening the Reporting of Observational Studies in Epidemiology (STROBE) (52).
Continuous variables were expressed as the mean (standard deviations), and categorical variables were presented as counts (percentages). Baseline characteristics among the various groups were compared using analysis of variance (ANOVA) for continuous variables and a χ2 test for categorical variables. Unadjusted and adjusted logistic regression models evaluated the relationship between serum calcium level as the primary predictor and probable sarcopenia status as the outcome. To explore potential interactions, the analysis was stratified by demographic variables. All data analyses were performed by using the Survey package in R software (version 4.3.0; R Foundation for Statistical Computing, Vienna, Austria). A two-sided p-value <0.05 indicated significance for all analyses.
The present study analyzed data from 7,309 participants aged 20 to 60 years who participated in NHANES from 2011 to 2018 (Figure 1). The prevalence of sarcopenia calculated using the ASM/BMI method was 7.91%, and using the ASM/weight method, it was 18.65%. Baseline characteristics of participants stratified by sarcopenia status according to the ASM/BMI (Table 1) and ASM/weight (Table 2) methods revealed consistent trends across both classification approaches. Individuals with sarcopenia were older and predominantly male. Racial disparities were observed, with a higher prevalence among Mexican Americans and Other Hispanics, while Non-Hispanic Black individuals had a significantly lower prevalence. Additionally, individuals identified as sarcopenia by both methods tended to be shorter stature, higher body weight and BMI, and lower PIR. This group also showed a higher prevalence of HBP, CKD, and DM. Moreover, sarcopenic individuals exhibited elevated blood levels of ALT, AST, cholesterol, and triglycerides, along with reduced dietary phosphorus intake, dietary calcium intake, serum calcium, and serum phosphorus.
Table 2. Characteristics of participants according to the status of sarcopenia, defined as ASM/Weight.
Significant differences in dietary calcium intake were noted in sarcopenic individuals identified by the ASM/BMI method, but not in those identified by the ASM/weight method. The ASM/BMI method revealed a higher proportion of individuals consuming alcohol, while the ASM/weight method showed no significant difference. Conversely, smoking was significantly more prevalent among individuals identified by the ASM/weight method, with no significant difference observed in the ASM/BMI group.
To investigate the relationship between various variables and the occurrence of sarcopenia, we conducted separate univariate logistic analyses. Under both definitions of sarcopenia, an increase in serum calcium was found to be negatively correlated with the risk of sarcopenia. Moreover, it was observed that age, gender, race, education, PIR, HBP, DM, CKD, ALT, AST, cholesterol, triglycerides, serum phosphorus, and dietary phosphorus were all identified as significant risk factors associated with the development of sarcopenia (Supplementary Tables 1, 2). After adjusting for all the covariates, it was consistently observed that higher Serum Calcium levels were associated with a decreased risk of sarcopenia (Table 3).
Table 3. Odds ratio of sarcopenia prevalence according to serum calcium category, sarcopenia defined by ASM/BMI and ASM/Weight.
To further elucidate the association between Serum Calcium and sarcopenia, we stratified Serum Calcium values into three tiers (Q1, Q2 and Q3). The result revealed that with increasing Serum Calcium levels, there was a gradual dose–response reduction in the risk of sarcopenia. Importantly, this trend remained consistent across different diagnostic criteria for sarcopenia (Figures 2A,B).
Figure 2. The prevalence of sarcopenia across different levels of serum calcium. (A) Sarcopenia defined using the appendicular skeletal muscle mass-to-body mass index (ASM/BMI) method. (B) Sarcopenia defined using the ASM-to-Weight (ASM/Weight) method. Q1, Q2, and Q3 represent tertiles of serum calcium levels.
We conducted stratification based on age, gender, race, education, and PIR. No significant interactions were identified between serum calcium and these stratifying variables. The relationship between serum calcium and the risk of sarcopenia remained consistent, except for specific racial groups (Sarcopenia defined by ASM/BMI: Other Hispanic, Non-Hispanic White, Non-Hispanic Asian, and Other Race; Sarcopenia defined by ASM/Weight, Non-Hispanic Black) (Figures 3, 4).
Figure 3. The association between serum calcium and sarcopenia within selected subgroups, with sarcopenia defined by appendicular skeletal muscle mass-to-body mass index (ASM/BMI). PIR refers to the Ratio of Family Income to Poverty.
Figure 4. The association between serum calcium and sarcopenia within selected subgroups, with sarcopenia defined by appendicular skeletal muscle mass-to-weight (ASM/Weight). PIR refers to the Ratio of Family Income to Poverty.
This study demonstrates a robust inverse association between serum calcium levels and sarcopenia risk in young and middle-aged adults (ages 20–60), irrespective of the definition criteria used. These associations persisted even after adjusting for potential confounding factors. Moreover, the observed dose–response trend across serum calcium tertiles further supports this association. Importantly, stratified analyses by demographic factors confirmed the consistency of this relationship, highlighting the potential relevance of serum calcium as a biomarker for sarcopenia risk assessment in this age group.
Sarcopenia’s prevalence estimates vary significantly due to differences in diagnostic criteria and cut-off thresholds. For instance, an NHANES-based study defining sarcopenia by ASM/BMI reported prevalences of 4.25% (ages 18–39) and 8.85% (ages 40–64), with variations by ethnicity and sex (53). Meanwhile, a Korea National Health and Nutrition Examination Survey analysis, using ASM/Weight, found prevalences of 19.2 and 29.1% in the 20–39 and 40–64 age groups, respectively (54). In the large-scale Kangbuk Samsung Health Study (over 300,000 participants), sarcopenia was identified via skeletal muscle mass index; using −1 SD and − 2 SD from a younger reference group (ages 20–39), prevalence ranged from 12.7–26.7% in females and 10.3–13% in males at −1 SD, and 2.4–5.7% in females and 1.7–2.9% in males at −2 SD (13). Consistent with these epidemiological patterns, our findings showed a sarcopenia prevalence of 7.91% (ASM/BMI) and 18.65% (ASM/Weight) in adults aged 20–60, highlighting the importance of early prevention strategies in younger populations.
Notably, discrepancies in dietary calcium intake were observed among sarcopenic individuals when classified by ASM/BMI versus ASM/weight. This inconsistency may arise from differences in how each method accounts for body composition. ASM/BMI focuses on muscle mass relative to body size, while ASM/Weight considers total body weight, which can be influenced by factors like adiposity. Increased body fat may impact calcium absorption and metabolism, as well as alter dietary habits, potentially leading to variations in calcium intake and metabolism (55–58). Additionally, altered hormonal regulation due to higher adiposity, such as changes in insulin sensitivity or vitamin D metabolism, may also play a role in modulating calcium dynamics in the body (59, 60). Given these interactions, variations in body fat distribution may partly explain the inconsistencies observed in dietary calcium intake across different sarcopenia classification methods.
Furthermore, racial disparities in sarcopenia prevalence were evident in our findings. Consistent with prior NHANES data (38), our study revealed that sarcopenia prevalence was significantly higher among Mexican Americans and Other Hispanics, whereas Non-Hispanic Black individuals exhibited a markedly lower prevalence. Several factors may contribute to these differences. Genetic predispositions influencing muscle mass and bone density likely play a role, as Non-Hispanic Black individuals typically have greater skeletal muscle mass and bone mineral density (61–64), which may confer resistance to sarcopenia. Additionally, disparities in lifestyle factors—including physical activity levels, dietary patterns, socioeconomic conditions, and healthcare accessibility—could further influence sarcopenia risk across racial and ethnic groups (61–63, 65, 66). These findings underscore the importance of considering racial and ethnic heterogeneity when developing targeted sarcopenia prevention strategies.
Previous research suggests that dietary and metabolic factors influence sarcopenia risk, with higher energy intake and metabolic syndrome components linked to increased susceptibility (12, 54, 67). Moreover, elevated levels of glycated hemoglobin and subclinical hyperthyroidism have been correlated to a higher risk of sarcopenia in individuals with type 2 DM (68, 69). Conversely, adequate protein intake (70, 71), sufficient vitamin D levels (72), and the administration of Lactobacillus plantarum probiotics (73) have demonstrated an association with a reduced risk. In this study, multiple logistic regression analysis revealed that a significant inverse relationship between serum calcium levels and sarcopenia risk (ASM/BMI: OR 0.07, 95% CI 0.02–0.20; ASM/Weight: OR 0.09, 95% CI 0.04–0.19). Specifically, each unit increase in serum calcium corresponded to a 93–95% reduction in sarcopenia likelihood. These findings align with calcium’s well-established physiological roles in muscle function. For instance, Calcium ions (Ca2+) regulate excitation–contraction coupling and act as signaling molecules in critical pathways such as CaMK and calcineurin, thereby influencing muscle growth and fiber type composition (18, 22). Moreover, appropriate mitochondrial Ca2+ uptake is essential for ATP production, although excessive Ca2+ can lead to mitochondrial dysfunction and muscle atrophy (74). Consequently, maintaining calcium homeostasis is crucial for preserving muscle integrity and preventing sarcopenic progression.
Subgroup analyses confirmed that this inverse association remained significant even after controlling for variables potentially affecting calcium metabolism (PIR, DM, CKD, HBP, and serum phosphorus). While most demographic factors did not modify this relationship, certain racial groups showed distinct patterns. These observations corroborate earlier findings that documented substantial racial and ethnic differences in sarcopenia prevalence and characteristics (38), potentially reflecting variations in body composition, genetic factors, dietary habits, physical activity levels, and broader socioeconomic factors (62, 65, 75–78). Future research should thus incorporate racial and ethnic diversity to clarify the underlying mechanisms and explore how differing sarcopenia definitions impact these populations.
Several limitations should be noted in this study. First, cross-sectional design restricts causal inferences, making it challenging to determine whether low serum calcium levels are a cause or consequence of sarcopenia. Second, due to limited grip strength data in NHANES, our study diagnosed sarcopenia based solely on muscle mass without considering grip strength. Third, while NHANES collected physical activity data during the 2011–2014 cycles, the absence of this information in subsequent cycles prevented us from adjusting for physical activity levels, a critical confounder in muscle health studies. Fourth, dietary calcium intake was assessed using a single 24-h dietary recall, which may not accurately reflect habitual intake and is subject to recall bias (79).
Fifth, some participants lacked data on key variables in this study. These missing values have likely resulted from participants not providing the necessary information or not undergoing specific examinations. To address this, we employed listwise deletion, excluding any records with missing values. However, this approach can reduce statistical power and may introduce selection bias, potentially affecting the generalizability of our findings. Future studies should consider advanced statistical methods, such as multiple imputation, to handle missing data more effectively and minimize potential biases. Finally, our subgroup analysis indicated that many factors related to serum calcium were not sources of heterogeneity in its association with sarcopenia, underscoring the need for more empirical evidence to elucidate how serum calcium influences sarcopenia development. Therefore, further prospective studies are warranted to validate these findings.
In summary, this study identified a significant inverse association between serum calcium levels and sarcopenia risk in young and middle-aged adults, consistent across demographic subgroups. These findings highlight the potential role of calcium in muscle health, suggesting that adequate dietary calcium intake and supplementation may help prevent sarcopenia. Given the cross-sectional design, future longitudinal studies are needed to establish causality and assess the effectiveness of calcium-based interventions.
The original contributions presented in the study are included in the article/Supplementary material, further inquiries can be directed to the corresponding authors.
JJ: Conceptualization, Data curation, Funding acquisition, Investigation, Methodology, Validation, Writing – original draft, Writing – review & editing. GC: Conceptualization, Data curation, Formal analysis, Funding acquisition, Validation, Writing – original draft, Writing – review & editing. YL: Conceptualization, Investigation, Methodology, Resources, Writing – review & editing. QZ: Conceptualization, Data curation, Writing – review & editing. ZC: Conceptualization, Data curation, Formal analysis, Funding acquisition, Resources, Supervision, Writing – original draft, Writing – review & editing.
The author(s) declare that financial support was received for the research and/or publication of this article. This work was supported by the Yunnan Province 14th Five-Year Plan Provincial Clinical Key Specialty Construction Project (The Affiliated Hospital of Yunnan University, Trauma Surgery), the Yunnan Province Xingdian Talent Support Program (Chen Zhong), the Yunnan University Medical Research Fund Project (No. YDYXJJ2024-0020), the Yunnan Provincial Basic Research Program Project (202501AT070182), the Yunnan Provincial Science and Technology Department-Kunming Medical University Joint Fund Applied Basic Research Projects (No. 202401AY070001-172, 202401AY070001-174, 202401AY070001-268), the Yunnan Provincial Department of Education Science Research Fund Project (2024J043).
The authors declare that the research was conducted in the absence of any commercial or financial relationships that could be construed as a potential conflict of interest.
The author(s) declare that no Gen AI was used in the creation of this manuscript.
All claims expressed in this article are solely those of the authors and do not necessarily represent those of their affiliated organizations, or those of the publisher, the editors and the reviewers. Any product that may be evaluated in this article, or claim that may be made by its manufacturer, is not guaranteed or endorsed by the publisher.
The Supplementary material for this article can be found online at: https://www.frontiersin.org/articles/10.3389/fnut.2025.1526879/full#supplementary-material
1. Cruz-Jentoft, AJ, Bahat, G, Bauer, J, Boirie, Y, Bruyère, O, Cederholm, T, et al. Sarcopenia: revised European consensus on definition and diagnosis. Age Ageing. (2019) 48:16–31. doi: 10.1093/ageing/afy169
2. Cruz-Jentoft, AJ, Baeyens, JP, Bauer, JM, Boirie, Y, Cederholm, T, Landi, F, et al. Sarcopenia: European consensus on definition and diagnosis: report of the European working group on sarcopenia in older people. Age Ageing. (2010) 39:412–23. doi: 10.1093/ageing/afq034
3. Veronese, N, Smith, L, Barbagallo, M, Yang, L, Zou, L, Haro, JM, et al. Sarcopenia and fall-related injury among older adults in five low-and middle-income countries. Exp Gerontol. (2021) 147:111262. doi: 10.1016/j.exger.2021.111262
4. Kara, M, Kara, Ö, Ceran, Y, Kaymak, B, Kaya, TC, Çıtır, BN, et al. SARcopenia assessment in hypertension: the SARAH study. Am J Phys Med Rehabil. (2023) 102:130–6. doi: 10.1097/PHM.0000000000002045
5. Chung, SM, Moon, JS, and Chang, MC. Prevalence of sarcopenia and its association with diabetes: a meta-analysis of community-dwelling Asian population. Front Med. (2021) 8:681232. doi: 10.3389/fmed.2021.681232
6. Cruz-Jentoft, AJ, and Sayer, AA. Sarcopenia. Lancet. (2019) 393:2636–46. doi: 10.1016/S0140-6736(19)31138-9
7. Endo, T, Akai, K, Kijima, T, Kitahara, S, Abe, T, Takeda, M, et al. An association analysis between hypertension, dementia, and depression and the phases of pre-sarcopenia to sarcopenia: a cross-sectional analysis. PLoS One. (2021) 16:e0252784. doi: 10.1371/journal.pone.0252784
8. Xiao, J, Caan, BJ, Cespedes Feliciano, EM, Meyerhardt, JA, Kroenke, CH, Baracos, VE, et al. The association of medical and demographic characteristics with sarcopenia and low muscle radiodensity in patients with nonmetastatic colorectal cancer. Am J Clin Nutr. (2019) 109:615–25. doi: 10.1093/ajcn/nqy328
9. Mesinovic, J, Zengin, A, De Courten, B, Ebeling, PR, and Scott, D. Sarcopenia and type 2 diabetes mellitus: a bidirectional relationship. Diabetes Metab Syndr Obes. (2019) 12:1057–72. doi: 10.2147/DMSO.S186600
10. Yu, M-D, Zhang, HZ, Zhang, Y, Yang, SP, Lin, M, Zhang, YM, et al. Relationship between chronic kidney disease and sarcopenia. Sci Rep. (2021) 11:20523. doi: 10.1038/s41598-021-99592-3
11. Jung, HN, Jung, CH, and Hwang, YC. Sarcopenia in youth. Metabolism. (2023) 144:155557. doi: 10.1016/j.metabol.2023.155557
12. Hwang, YC, Cho, IJ, Jeong, IK, Ahn, KJ, and Chung, HY. Differential association between sarcopenia and metabolic phenotype in Korean young and older adults with and without obesity. Obesity. (2017) 25:244–51. doi: 10.1002/oby.21694
13. Park, CH, do, JG, Lee, YT, and Yoon, KJ. Sex difference in cutoff and prevalence of sarcopenia among 300,090 urban Korean population: association with metabolic syndrome. Medicina. (2022) 58:361. doi: 10.3390/medicina58101361
14. Sousa, AS, Guerra, RS, Fonseca, I, Pichel, F, Ferreira, S, and Amaral, TF. Financial impact of sarcopenia on hospitalization costs. Eur J Clin Nutr. (2016) 70:1046–51. doi: 10.1038/ejcn.2016.73
15. Nishikawa, H, Asai, A, Fukunishi, S, Nishiguchi, S, and Higuchi, K. Metabolic syndrome and sarcopenia. Nutrients. (2021) 13:3519. doi: 10.3390/nu13103519
16. Lim, S, Kim, JH, Yoon, JW, Kang, SM, Choi, SH, Park, YJ, et al. Sarcopenic obesity: prevalence and association with metabolic syndrome in the Korean longitudinal study on health and aging (KLoSHA). Diabetes Care. (2010) 33:1652–4. doi: 10.2337/dc10-0107
17. Brook, MS, Wilkinson, DJ, Phillips, BE, Perez-Schindler, J, Philp, A, Smith, K, et al. Skeletal muscle homeostasis and plasticity in youth and ageing: impact of nutrition and exercise. Acta Physiol. (2016) 216:15–41. doi: 10.1111/apha.12532
18. Berchtold, MW, Brinkmeier, H, and Muntener, M. Calcium ion in skeletal muscle: its crucial role for muscle function, plasticity, and disease. Physiol Rev. (2000) 80:1215–65. doi: 10.1152/physrev.2000.80.3.1215
20. Bers, DM. Cardiac excitation–contraction coupling. Nature. (2002) 415:198–205. doi: 10.1038/415198a
21. Goll, DE, Carragher, NO, Frame, MC, Parr, T, and Martin, SG. The calpain system. Nat Rev Cancer. (2003) 83:731–801. doi: 10.1152/physrev.00029.2002
22. Gehlert, S, Bloch, W, and Suhr, F. Ca2+−dependent regulations and signaling in skeletal muscle: from electro-mechanical coupling to adaptation. Int J Mol Sci. (2015) 16:1066–95. doi: 10.3390/ijms16011066
23. Yin, L, Li, N, Jia, W, Wang, N, Liang, M, Yang, X, et al. Skeletal muscle atrophy: from mechanisms to treatments. Pharmacol Res. (2021) 172:105807. doi: 10.1016/j.phrs.2021.105807
24. Ganapathy, A, and Nieves, JW. Nutrition and sarcopenia—what do we know? Nutrients. (2020) 12:1755. doi: 10.3390/nu12061755
25. Petermann-Rocha, F, Chen, M, Gray, SR, Ho, FK, Pell, JP, and Celis-Morales, C. Factors associated with sarcopenia: a cross-sectional analysis using UK biobank. Maturitas. (2020) 133:60–7. doi: 10.1016/j.maturitas.2020.01.004
26. Kim, YS, Hong, KW, Han, K, Park, YC, Park, JM, Kim, K, et al. Longitudinal observation of muscle mass over 10 years according to serum calcium levels and calcium intake among Korean adults aged 50 and older: the Korean genome and epidemiology study. Nutrients. (2020) 12:856. doi: 10.3390/nu12092856
27. Ostrominski, JW, Arnold, SV, Butler, J, Fonarow, GC, Hirsch, JS, Palli, SR, et al. Prevalence and overlap of cardiac, renal, and metabolic conditions in US adults, 1999-2020. JAMA Cardiol. (2023) 8:1050–60. doi: 10.1001/jamacardio.2023.3241
28. Fang, L, Sheng, H, Tan, Y, and Zhang, Q. Prevalence of diabetes in the USA from the perspective of demographic characteristics, physical indicators and living habits based on NHANES 2009-2018. Front Endocrinol. (2023) 14:1088882. doi: 10.3389/fendo.2023.1088882
29. Tu, J, Shi, S, Liu, Y, Xiu, J, Zhang, Y, Wu, B, et al. Dietary inflammatory potential is associated with sarcopenia in patients with hypertension: national health and nutrition examination study. Front Nutr. (2023) 10:1176607. doi: 10.3389/fnut.2023.1176607
30. Zong, G, Zhang, Z, Yang, Q, Wu, H, Hu, FB, and Sun, Q. Total and regional adiposity measured by dual-energy X-ray absorptiometry and mortality in NHANES 1999-2006. Obesity. (2016) 24:2414–21. doi: 10.1002/oby.21659
31. Chun, HS, Kim, MN, Lee, JS, Lee, HW, Kim, BK, Park, JY, et al. Risk stratification using sarcopenia status among subjects with metabolic dysfunction-associated fatty liver disease. J Cachexia Sarcopenia Muscle. (2021) 12:1168–78. doi: 10.1002/jcsm.12754
32. Kinoshita, K, Satake, S, Matsui, Y, and Arai, H. Quantifying muscle mass by adjusting for body mass index is the best for discriminating low strength and function in Japanese older outpatients. J Nutr Health Aging. (2021) 25:501–6. doi: 10.1007/s12603-020-1557-3
33. Han, E, Lee, YH, Kim, G, Kim, SR, Lee, BW, Kang, ES, et al. Sarcopenia is associated with albuminuria independently of hypertension and diabetes: KNHANES 2008-2011. Metabolism. (2016) 65:1531–40. doi: 10.1016/j.metabol.2016.07.003
34. Moreira, VG, Perez, M, and Lourenço, RAJC. Prevalence of sarcopenia and its associated factors: the impact of muscle mass, gait speed, and handgrip strength reference values on reported frequencies. Clinics. (2019) 74:e477. doi: 10.6061/clinics/2019/e477
35. Masanés, F, Rojano i Luque, X, Salvà, A, Serra-Rexach, JA, Artaza, I, Formiga, F, et al. Cut-off points for muscle mass — not grip strength or gait speed — determine variations in sarcopenia prevalence. J Nutr Health Aging. (2017) 21:825–9. doi: 10.1007/s12603-016-0844-5
36. Hill, KM, Martin, BR, Wastney, ME, McCabe, GP, Moe, SM, Weaver, CM, et al. Oral calcium carbonate affects calcium but not phosphorus balance in stage 3–4 chronic kidney disease. Kidney Int. (2013) 83:959–66. doi: 10.1038/ki.2012.403
37. Yang, L, Smith, L, and Hamer, M. Gender-specific risk factors for incident sarcopenia: 8-year follow-up of the English longitudinal study of ageing. J Epidemiol Community Health. (2019) 73:86–8. doi: 10.1136/jech-2018-211258
38. Bigman, G, and Ryan, A. Implications of race and ethnicity in sarcopenia US national prevalence of sarcopenia by muscle mass, strength, and function indices. Gerontol Geriatr Res. (2021) 4:126.
39. Park, SW, Goodpaster, BH, Lee, JS, Kuller, LH, Boudreau, R, de Rekeneire, N, et al. Excessive loss of skeletal muscle mass in older adults with type 2 diabetes. Diabetes Care. (2009) 32:1993–7. doi: 10.2337/dc09-0264
40. Lee, N, and Choi, C-J. Smoking and diabetes as predictive factors of accelerated loss of muscle mass in middle-aged and older women: a six-year retrospective cohort study. J Women's Health. (2019) 28:1391–8. doi: 10.1089/jwh.2018.7527
41. Sabatino, A, Cuppari, L, Stenvinkel, P, Lindholm, B, and Avesani, CM. Sarcopenia in chronic kidney disease: what have we learned so far? J Nephrol. (2021) 34:1347–72. doi: 10.1007/s40620-020-00840-y
42. Gangrade, N, Figueroa, J, and Leak, T. Income disparities in snacking characteristics among US adolescents 12 to 19 years: National Health and nutrition examination survey 2005–2018. Curr Dev Nutr. (2021) 5:406–6. doi: 10.1093/cdn/nzab038_018
43. Goodman, WG. Calcium and phosphorus metabolism in patients who have chronic kidney disease. Med Clin. (2005) 89:631–47. doi: 10.1016/j.mcna.2004.12.005
44. Stommel, M, and Schoenborn, A. Accuracy and usefulness of BMI measures based on self-reported weight and height: findings from the NHANES & NHIS 2001-2006. BMC Public Health. (2009) 9:421. doi: 10.1186/1471-2458-9-421
45. Gandhi, K, Lim, E, Davis, J, and Chen, JJ. Racial-ethnic disparities in self-reported health status among US adults adjusted for sociodemographics and multimorbidities, National Health and nutrition examination survey 2011–2014. Ethn Health. (2020) 25:65–78. doi: 10.1080/13557858.2017.1395812
46. Aggarwal, R, Yeh, RW, Joynt Maddox, KE, and Wadhera, RK. Cardiovascular risk factor prevalence, treatment, and control in US adults aged 20 to 44 years, 2009 to march 2020. JAMA. (2023) 329:899–909. doi: 10.1001/jama.2023.2307
47. Rattan, P, Penrice, DD, Ahn, JC, Ferrer, A, Patnaik, M, Shah, VH, et al. Inverse association of telomere length with liver disease and mortality in the US population. Hepatol Communic. (2022) 6:399–410. doi: 10.1002/hep4.1803
48. Li, C, and Shang, S. Relationship between sleep and hypertension: findings from the NHANES (2007–2014). Int J Environ Res Public Health. (2021) 18:7867. doi: 10.3390/ijerph18157867
49. Levey, AS, Stevens, LA, Schmid, CH, Zhang, Y, Castro, AF, Feldman, HI, et al. A new equation to estimate glomerular filtration rate. Ann Intern Med. (2009) 150:604–12. doi: 10.7326/0003-4819-150-9-200905050-00006
50. Moshfegh, AJ, Rhodes, DG, Baer, DJ, Murayi, T, Clemens, JC, Rumpler, WV, et al. The US Department of Agriculture Automated Multiple-Pass Method reduces bias in the collection of energy intakes. Am J Clin Nutr. (2008) 88:324–32. doi: 10.1093/ajcn/88.2.324
51. Centers for Disease Control and Prevention. The third National Health and nutrition examination survey (NHANES III 1988–94) reference manuals and reports [CD-ROM]. Bethesda, MD: Centers for Disease Control and Prevention (1996).
52. Von Elm, E, Altman, DG, Egger, M, Pocock, SJ, Gøtzsche, PC, and Vandenbroucke, JP. The strengthening the reporting of observational studies in epidemiology (STROBE) statement: guidelines for reporting observational studies. Lancet. (2007) 370:1453–7. doi: 10.1016/S0140-6736(07)61602-X
53. Du, K, Goates, S, Arensberg, MB, Pereira, S, Gaillard, T, et al. Prevalence of sarcopenia and sarcopenic obesity vary with race/ethnicity and advancing age. Divers Equal Health Care. (2018) 15:173. doi: 10.21767/2049-5471.1000173
54. Bae, E-J, and Kim, Y-H. Factors affecting sarcopenia in Korean adults by age groups. Osong Public Health Res Perspect. (2017) 8:169–78. doi: 10.24171/j.phrp.2017.8.3.03
55. Janssen, I, Heymsfield, SB, Wang, ZM, and Ross, R. Skeletal muscle mass and distribution in 468 men and women aged 18–88 yr. J Appl Physiol. (2000) 89:81–8. doi: 10.1152/jappl.2000.89.1.81
56. Bredariol, AN, Rossato, LT, FMS, B, Nahas, PC, Orsatti, FL, and de Oliveira, EP. Calcium intake is inversely associated with body fat in postmenopausal women. Clin Nutr ESPEN. (2020) 39:206–9. doi: 10.1016/j.clnesp.2020.06.012
57. Jacqmain, M, Doucet, E, Després, JP, Bouchard, C, and Tremblay, A. Calcium intake, body composition, and lipoprotein-lipid concentrations in adults. Am J Clin Nutr. (2003) 77:1448–52. doi: 10.1093/ajcn/77.6.1448
58. Rivera-Villegas, A, Carrillo-Muro, O, Rodríguez-Cordero, D, Hernández-Briano, P, Sánchez-Barbosa, OY, Lazalde-Cruz, R, et al. Effects of supplemental calcium propionate and concentrate level: growth performance, body fat reserves, and health of high-risk beef calves. Vet Sci. (2024) 11:336. doi: 10.3390/vetsci11080336
59. Pourshahidi, LK. Vitamin D and obesity: current perspectives and future directions. Proc Nutr Soc. (2015) 74:115–24. doi: 10.1017/S0029665114001578
60. Hagström, E, Hellman, P, Lundgren, E, Lind, L, and Ärnlöv, J. Serum calcium is independently associated with insulin sensitivity measured with euglycaemic–hyperinsulinaemic clamp in a community-based cohort. Diabetologia. (2007) 50:317–24. doi: 10.1007/s00125-006-0532-9
61. Auyeung, TW, Lee, SWJ, Leung, J, Kwok, T, and Woo, J. Age‐associated decline of muscle mass, grip strength and gait speed: a 4‐year longitudinal study of 3018 community‐dwelling older Chinese. Geriatr Gerontol Int. (2014) 14:76–84. doi: 10.1111/ggi.12213
62. Shaw, S, Dennison, E, and Cooper, C. Epidemiology of sarcopenia: determinants throughout the lifecourse. Calcif Tissue Int. (2017) 101:229–47. doi: 10.1007/s00223-017-0277-0
63. Araujo, AB, Chiu, GR, Kupelian, V, Hall, SA, Williams, RE, Clark, RV, et al. Lean mass, muscle strength, and physical function in a diverse population of men: a population-based cross-sectional study. BMC Public Health. (2010) 10:1–8. doi: 10.1186/1471-2458-10-508
64. Araujo, A, Zmuda, JM, Barrett-Connor, E, Sheu, Y, Patrick, AL, Leung, PC, et al. Race/ethnic differences in bone mineral density in men. Osteoporos Int. (2007) 18:943–53. doi: 10.1007/s00198-006-0321-9
65. Leon, CD, Barnes, LL, Bienias, JL, Skarupski, KA, Evans, DA, et al. Racial disparities in disability: recent evidence from self-reported and performance-based disability measures in a population-based study of older adults. J Gerontol B Psychol Sci Soc Sci. (2005) 60:S263–71. doi: 10.1093/geronb/60.5.s263
66. Al Snih, S, Kaushik, V, Eschbach, K, and Markides, K. Ethnic differences in physical performance in older Americans: data from the third National Health and nutrition examination survey (1988–1994). Aging Clin Exp Res. (2008) 20:139–44. doi: 10.1007/BF03324760
67. van Dronkelaar, C, van Velzen, A, Abdelrazek, M, van der Steen, A, Weijs, PJM, and Tieland, M. Minerals and sarcopenia; the role of calcium, Iron, magnesium, phosphorus, potassium, selenium, sodium, and zinc on muscle mass, muscle strength, and physical performance in older adults: a systematic review. J Am Med Dir Assoc. (2018) 19:6–11.e3. doi: 10.1016/j.jamda.2017.05.026
68. Li, P, Ding, L, Zhen, J, Hu, J, Fan, Y, and Liu, M. Association of subtle alterations in thyroid function with presarcopenia in patients with type 2 diabetes mellitus. J Diabetes Investig. (2022) 13:696–705. doi: 10.1111/jdi.13703
69. Sugimoto, K, Tabara, Y, Ikegami, H, Takata, Y, Kamide, K, Ikezoe, T, et al. Hyperglycemia in non-obese patients with type 2 diabetes is associated with low muscle mass: the multicenter study for clarifying evidence for sarcopenia in patients with diabetes mellitus. J Diabetes Investig. (2019) 10:1471–9. doi: 10.1111/jdi.13070
70. Kim, I, Son, K, Jeong, SJ, and Lim, H. Sex and diet-related disparities in low handgrip strength among Young and middle-aged Koreans: findings based on the Korea National Health and nutrition examination survey (KNHANES) from 2014 to 2017. Nutrients. (2022) 14:816. doi: 10.3390/nu14183816
71. Koopman, R, Verdijk, L, Manders, RJF, Gijsen, AP, Gorselink, M, Pijpers, E, et al. Co-ingestion of protein and leucine stimulates muscle protein synthesis rates to the same extent in young and elderly lean men. Am J Clin Nutr. (2006) 84:623–32. doi: 10.1093/ajcn/84.3.623
72. Kim, Y, Chang, Y, Ryu, S, Cho, IY, Kwon, MJ, Wild, SH, et al. Serum 25-hydroxy vitamin D and the risk of low muscle mass in young and middle-aged Korean adults. Eur J Endocrinol. (2022) 186:477–87. doi: 10.1530/EJE-21-1229
73. Huang, WC, Lee, MC, Lee, CC, Ng, KS, Hsu, YJ, Tsai, TY, et al. Effect of Lactobacillus plantarum TWK10 on exercise physiological adaptation, performance, and body composition in healthy humans. Nutrients. (2019) 11:836. doi: 10.3390/nu11112836
74. Terrell, K, Choi, S, and Choi, S. Calcium’s role and signaling in aging muscle, cellular senescence, and mineral. Int J Mol Sci. (2023) 24:17034. doi: 10.3390/ijms242317034
75. Newman, AB, Haggerty, CL, Goodpaster, B, Harris, T, Kritchevsky, S, Nevitt, M, et al. Strength and muscle quality in a well-functioning cohort of older adults: the health. Aging Body Composition Study. (2003) 51:323–30. doi: 10.1046/j.1532-5415.2003.51105.x
76. Fanelli Kuczmarski, M, Mason, MA, Beydoun, MA, Allegro, D, Zonderman, AB, and Evans, MK. Dietary patterns and sarcopenia in an urban African American and white population in the United States. J Nutr Gerontol Geriatr. (2013) 32:291–316. doi: 10.1080/21551197.2013.840255
77. Rolland, Y, Czerwinski, S, van Kan, GA, Morley, JE, Cesari, M, onder, G, et al. Sarcopenia: its assessment, etiology, pathogenesis, consequences and future perspectives. J Nutr Health Aging. (2008) 12:433–50. doi: 10.1007/BF02982704
78. Urzi, F, Pokorny, B, and Buzan, E. Pilot study on genetic associations with age-related sarcopenia. Front Genet. (2021) 11:615238. doi: 10.3389/fgene.2020.615238
Keywords: sarcopenia, serum calcium, young and middle-aged adults, NHANES, appendicular skeletal muscle mass
Citation: Jiang J, Chen G, Li Y, Zhao Q and Chen Z (2025) Serum calcium levels and the risk of sarcopenia in young adults: insights from NHANES 2011–2018. Front. Nutr. 12:1526879. doi: 10.3389/fnut.2025.1526879
Received: 03 December 2024; Accepted: 27 February 2025;
Published: 14 March 2025.
Edited by:
Sawsan A. Zaitone, University of Tabuk, Saudi ArabiaReviewed by:
Yasmina Mohammed Abd-Elhakim, Zagazig University, EgyptCopyright © 2025 Jiang, Chen, Li, Zhao and Chen. This is an open-access article distributed under the terms of the Creative Commons Attribution License (CC BY). The use, distribution or reproduction in other forums is permitted, provided the original author(s) and the copyright owner(s) are credited and that the original publication in this journal is cited, in accordance with accepted academic practice. No use, distribution or reproduction is permitted which does not comply with these terms.
*Correspondence: Qinggang Zhao, MzY4MjI4NzNAcXEuY29t; Zhong Chen, Y2hlbnpob25nZHJAeW51LmVkdS5jbg==
†These authors have contributed equally to this work
Disclaimer: All claims expressed in this article are solely those of the authors and do not necessarily represent those of their affiliated organizations, or those of the publisher, the editors and the reviewers. Any product that may be evaluated in this article or claim that may be made by its manufacturer is not guaranteed or endorsed by the publisher.
Research integrity at Frontiers
Learn more about the work of our research integrity team to safeguard the quality of each article we publish.