- 1Department of Thyroid Surgery, The Affiliated Cancer Hospital of Xiangya School of Medicine, Central South University/Hunan Cancer Hospital, Changsha, Hunan, China
- 2Department of Thyroid Surgery, The Second Xiangya Hospital, Central South University, Changsha, Hunan, China
- 3Department of Surgery, University Hospital, Central South University, Changsha, Hunan, China
Objective: While previous studies have explored the relationship between obesity and levels of thyroid autoantibodies, research using novel indicators such as weight-adjusted waist index (WWI) remains limited. This study aimed to evaluate the potential relationship between WWI and thyroid autoantibody levels, with the objective of improving our understanding of the links between central obesity and Hashimoto’s thyroiditis (HT).
Methods: We conducted a cross-sectional study using data from the National Health and Nutrition Examination Survey (NHANES) cycles from 2007 to 2012. We analyzed the relationship between WWI and levels of thyroid peroxidase antibodies (TPOAb) and thyroglobulin antibodies (TgAb) through multivariate linear regression and subgroup analyses.
Results: The study included 7,056 participants with an average age of 49.71 ± 17.66 years, comprising 49.18% females. Mean WWI across the cohort was 11.04 ± 0.84. Analysis revealed a significant positive association between WWI and TPOAb levels (β: 4.78, 95% CI: 1.52, 8.05, p = 0.0041), which remained consistent across all multivariate linear regression models. In contrast, no significant correlation was found between WWI and TgAb levels after adjusting for covariates. Subgroup analysis stratified by gender demonstrated a notable gender-specific effect, where the positive correlation between WWI and TPOAb levels was evident only in females (β: 8.13, 95% CI: 4.14, 12.12, p < 0.0001).
Conclusion: This study used WWI as a novel indicator of central obesity and identified a strong association with HT, particularly notable in females. However, further high-quality studies are needed to confirm these findings and explore the underlying biological mechanisms.
Introduction
Thyroid autoantibodies, including thyroid peroxidase antibodies (TPOAb) and thyroglobulin antibodies (TgAb), are crucial markers for diagnosing and managing Hashimoto’s thyroiditis (HT) (1). TPOAb targets thyroid peroxidase, an enzyme essential for thyroid hormone production, while TgAb targets thyroglobulin, the protein precursor to these hormones. Elevated levels of these antibodies indicate an autoimmune response against the thyroid gland and are commonly observed in HT (2). This autoimmune attack on thyroid tissue can ultimately lead to irreversible hypothyroidism. Furthermore, chronic inflammation and immune dysregulation create a conducive environment for carcinogenesis within thyroid tissue (3). Consequently, HT has emerged as a significant public health concern. Understanding the factors that influence TPOAb and TgAb levels can offer valuable insights into the pathogenesis of these disorders and aid in developing effective treatment strategies.
In recent years, obesity has been recognized as a systemic chronic inflammatory disease, with adipocytes playing a critical role in chronic inflammation and immune regulation (4–6). Various studies have reported a link between obesity and HT, but this relationship remains controversial (7). Traditional obesity assessment tools, such as Body Mass Index (BMI) and Waist Circumference (WC), have limitations (8). For example, BMI cannot distinguish between central and peripheral obesity and varies with age, gender, and other factors (9, 10). In 2018, a new anthropometric index, the weight-adjusted-waist index (WWI), was introduced to assess obesity (11).
The WWI offers a more precise assessment of central obesity, which is crucial for understanding associated health risks, including metabolic and cardiovascular health (11). Using this novel indicator to investigate the correlation between obesity and HT may reveal a more accurate relationship, thereby contributing to the effective management of HT. Thus, our aim is to evaluate the potential relationship between WWI and thyroid autoantibody levels using data from the National Health and Nutrition Examination Survey (NHANES) covering the years 2007 to 2012, with the objective of improving our understanding of the links between central obesity and HT.
Materials and methods
Study design
This cross-sectional study adhered to the guidelines outlined in the Strengthening the Reporting of Observational Studies in Epidemiology (STROBE) statement (12).
Study population
This cross-sectional study utilized data from the National Health and Nutrition Examination Survey (NHANES) cycles from 2007 to 2012. NHANES is a national survey that evaluates the health and nutritional status of the U.S. population using a representative sample of non-hospitalized residents (13). Detailed information about the NHANES survey design can be found on the CDC website at https://www.cdc.gov/nchs/nhanes/index.htm.
While data related to WWI (weight and waist measurements) are present in all NHANES datasets, only the dataset spanning 2007 to 2012 included comprehensive data on thyroid function. Hence, this analysis utilized data from the 2007–2012 dataset. In this cohort, 30,442 individuals were initially considered. Exclusions were applied for participants lacking data on thyroid autoantibodies (a total of 15,700 individuals, comprising 15,646 without TPOAb data and 54 without TgAb data), WWI (a total of 4,751 individuals, including 4,727 without waist circumference data and 24 without weight data), and covariates (a total of 2,935 individuals). Ultimately, the study enrolled a total of 7,056 participants, as depicted in Figure 1.
Evaluation of central obesity
The WWI is designed to assess central obesity, calculated by dividing waist circumference (in cm) by the square root of body weight (in kg) (14). In this study, WWI was treated as the exposure variable.
Evaluation of HT
The HT was assessed based on the levels of thyroid peroxidase antibodies (TPOAb) and thyroglobulin antibodies (TgAb) in individual serum samples. The magnitude of TPOAb and TgAb levels partly reflects the severity of autoimmune thyroid disease (15). Due to the lack of thyroid ultrasound imaging data in the NHANES dataset, this study could not evaluate HT at an imaging level.
Selection of covariates
To enhance the accuracy of this study, we included a variety of sociodemographic and behavioral factors, along with selected biochemical markers related to thyroid function and obesity, as potential confounding variables. The included covariates were: age (years), gender (Male/Female), racial/ethnic group (Mexican American, Other Hispanic, Non-Hispanic White, Non-Hispanic Black, or Other race/multiracial), education level (Less than high school, High school, or More than high school), Poverty Income Ratio (PIR), marital status (Married or living with partner, Divorced, separated, or widowed, Never married), smoking status (indicating if the participant has smoked at least 100 cigarettes in their life: Yes/No), alcohol intake (drank at least 12 alcoholic drinks in the past year: Yes/No), diabetes status (Yes/No), hypertension status (Yes/No), levels of FT3, FT4, TSH, Tg, and High-density lipoprotein cholesterol (HDL-C). The selection of covariates was based on their established associations with both obesity and thyroid autoimmunity, ensuring that potential confounding factors were adequately accounted for. Demographic variables such as age, gender, and racial/ethnic group were included due to their influence on thyroid function and obesity prevalence. Socioeconomic factors, represented by education level and Poverty Income Ratio (PIR), were considered for their impact on health behaviors and access to healthcare. Lifestyle factors, including smoking status and alcohol intake, were selected because of their known effects on metabolic health and immune regulation. Clinical variables, such as diabetes and hypertension status, were included given their associations with chronic inflammation and thyroid dysfunction. Additionally, thyroid-related parameters (FT3, FT4, TSH, Tg) and metabolic markers like HDL-C were incorporated to provide context for the relationship between WWI and thyroid autoantibodies. This comprehensive selection ensured a robust analysis and minimized bias in evaluating the association.
Statistical analysis
Statistical analysis adhered to survey methods and NHANES analysis guidelines. Continuous variables were presented as mean ± standard deviation (Mean ± SD), while categorical variables were expressed as percentages. The relationship between WWI and thyroid autoantibodies was evaluated using multivariate linear regression models with three levels of adjustment: unadjusted, minimally adjusted, and fully adjusted models. To further assess the association across different levels of central obesity, WWI was divided into quartiles, and separate linear regression analyses were conducted for each quartile (16). Additionally, smoothed curve fitting was employed to assess the relationship between WWI and thyroid autoantibodies, providing a graphical representation of the association. Subgroup analysis was conducted to evaluate effect modification across various subgroups, including gender, age, race, education level, smoking status, alcohol intake, diabetes status, and hypertension status. Interaction tests were applied to assess the consistency of these associations across the different subgroups. All statistical analyses were performed using R software (version 4.2) and EmpowerStats (v.2.01, X&Y Solutions, Inc., Boston, MA, United States), with a two-sided p-value of less than 0.05 considered statistically significant.
Ethics approval and consent to participate
All procedures conducted in studies involving human participants adhered to the ethical standards set by the institutional and/or national research committees, in line with the 1964 Helsinki Declaration and its subsequent amendments or equivalent ethical guidelines. The analyses were based on data from the National Health and Nutrition Examination Survey (NHANES). The study received approval from the Ethics Review Board of the National Center for Health Statistics. Detailed information is available on the NHANES website. Written informed consent was obtained from each participant prior to their inclusion in the NHANES database. Further details regarding the ethics application and informed consent process are also provided on the NHANES website.
Results
Population characteristics
Our study included 7,056 individuals with an average age of 49.71 ± 17.66 years, of whom 49.18% were female and 48.07% were Non-Hispanic White. Additional demographic data are presented in Table 1. The average WWI for all participants was 11.04 ± 0.84. The mean WWI values for each quartile (Q1–Q4) were as follows: Q1: 8.11–10.47, Q2: 10.47–11.05, Q3: 11.05–11.61, and Q4: 11.61–15.39. The mean TPOAb and TgAb values for all individuals were 20.48 ± 94.02 IU/mL and 9.94 ± 89.71 IU/mL, respectively. Both TPOAb and TgAb levels showed an upward trend across increasing WWI quartiles (TPOAb: Q1: 12.81 ± 64.43 IU/mL, Q2: 19.61 ± 86.33 IU/mL, Q3: 23.38 ± 99.57 IU/mL, Q4: 26.11 ± 117.29 IU/mL, p < 0.001; TgAb: Q1: 4.79 ± 48.68 IU/mL, Q2: 8.39 ± 81.31 IU/mL, Q3: 12.34 ± 103.75 IU/mL, Q4: 14.24 ± 111.40 IU/mL, p = 0.009). Individuals in the higher WWI quartiles tended to be older, more likely to be female, and had lower incomes and education levels. They also showed a higher prevalence of diabetes and hypertension, elevated TSH levels, and lower HDL-C levels.
Multiple regression analysis
We utilized three models to investigate the relationship between WWI and thyroid autoimmune antibodies (Tables 2, 3). The crude model did not adjust for any covariates. Model I adjusted for gender, age, and race. Model II adjusted for all covariates. When considering WWI as a continuous variable, there was a significant positive relationship between WWI and TPOAb, which remained statistically significant across all three models. In the crude model without controlling for any covariates, the β was 6.38 (95% CI 3.76, 9.00, p < 0.0001). Similarly, the β was 3.78 (95% CI 0.64, 6.93, p = 0.0183) in model I and the β was 4.78 (95% CI 1.52, 8.05, p = 0.0041) in model II. However, we only identified a significant positive relationship between WWI and TgAb in the crude model, where the β was 4.54 (95% CI 2.03, 7.04, p = 0.0004). After dividing WWI into quartiles, we observed that this positive relationship remained significant for WWI and TPOAb across all three models. In the crude model, higher WWI groups showed an increasing trend in TPOAb levels compared to the lowest quartile (p for trend <0.0001). In the fully adjusted model, for individuals in the highest quartile of WWI, each unit increase in WWI was associated with an increase in TPOAb levels by 11.08 IU/mL compared to those in the lowest quartile (β: 11.08, 95% CI: 3.75, 18.41). Similarly, the association between WWI quartiles and TgAb was statistically significant only in the crude model (p for trend = 0.0007), where for individuals in the highest quartile of WWI, each unit increase in WWI was associated with an increase in TgAb levels by 9.45 IU/mL compared to those in the lowest quartile (β: 9.45, 95% CI: 3.53, 15.37).
Linear correlation between WWI and thyroid autoimmune antibodies
A smooth curve fitting was used to describe the linear relationship between WWI and thyroid autoantibodies (Figures 2A,B). Smooth curves were constructed based on model II. Results showed that WWI was linearly positively correlated with TPOAb levels (p = 0.0038). However, the linear relationship between WWI and TgAb (p = 0.5688) was less pronounced than its relationship with TPOAb.
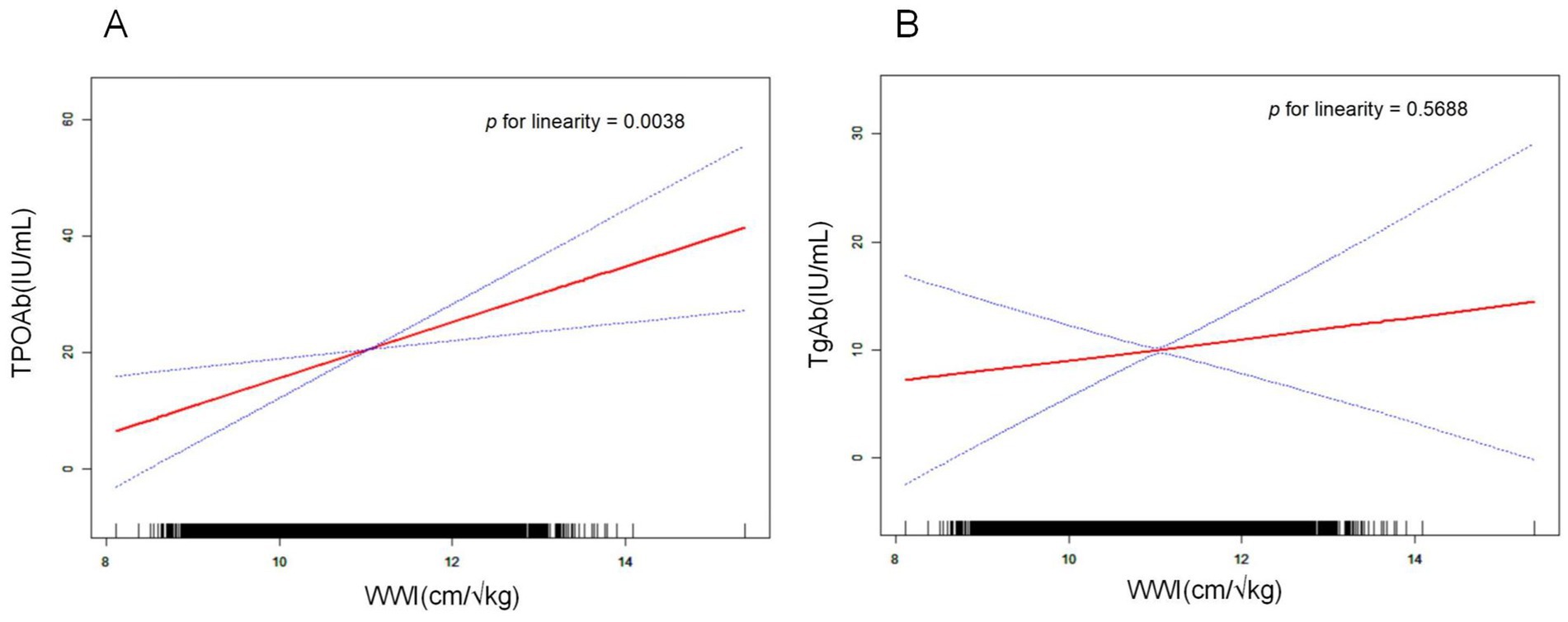
Figure 2. Relationship between WWI and thyroid autoantibodies. The natural spline curve shows a linear relationship between WWI and thyroid autoantibodies. The area between the blue dashed lines is considered to be the 95% confidential interval. Each read dot reveals the thyroid autoantibody levels corresponding to the WWI value, forming a continuous fitted curve. Age, gender, race, education level, PIR, marital status, smoking, alcohol intake, diabetes status, hypertension status, FT3, FT4, TSH, Tg, and HDL-C were adjusted. (A) Linear correlation between WWI and TPOAb (p for linearity = 0.0038). (B) Linear correlation between WWI and TgAb (p for linearity = 0.5688).
Subgroup analysis
As shown in Figures 3A,B, subgroup analysis and interaction tests were conducted to assess the consistency of the relationship between WWI and thyroid autoantibody levels across various subgroups. After adjusting for all covariates, significant differences in the association between WWI and TPOAb levels were found across different gender groups (p for interaction = 0.0079). Notably, this significant positive correlation was observed only in the female subgroup and not in the male subgroup. For female individuals, each unit increase in WWI was associated with an increase in TPOAb levels by 8.13 IU/mL (β: 8.13, 95% CI: 4.14, 12.12, p < 0.0001). Other stratified factors such as age, race, education level, smoking, alcohol intake, diabetes status, and hypertension status did not significantly influence the positive association between WWI and TPOAb levels.
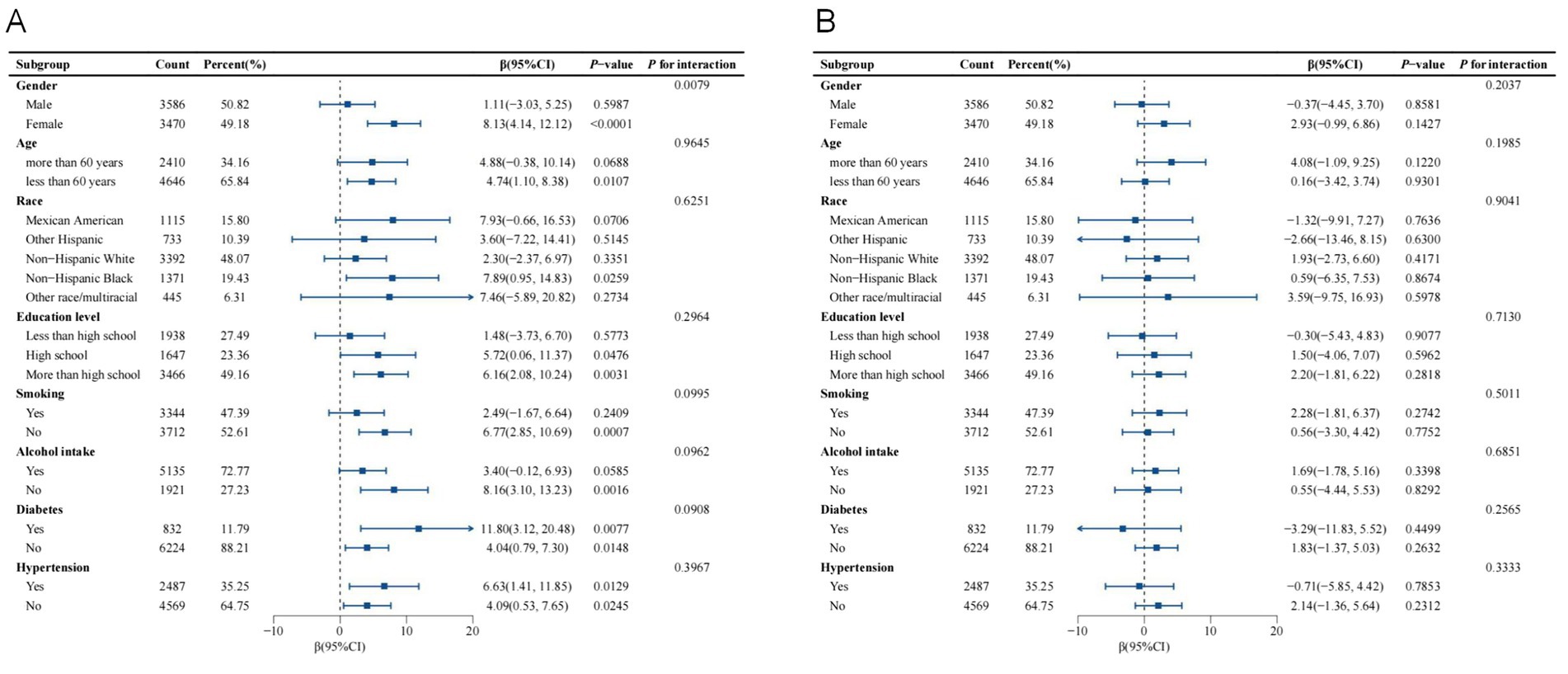
Figure 3. Subgroup analysis of the association between WWI and thyroid autoantibodies. Age, gender, race, education level, PIR, marital status, smoking, alcohol intake, diabetes status, hypertension status, FT3, FT4, TSH, Tg, and HDL-C were adjusted. (A) Subgroup analysis of the association between WWI and TPOAb. (B) Subgroup analysis of the association between WWI and TgAb.
Discussion
Many scholars have endeavored to elucidate the relationship between obesity and thyroid autoantibodies levels. Meta-analyses have indicated a positive correlation between obesity and hypothyroidism, HT, and TPOAb levels, incorporating data from 22 studies that commonly used BMI as the standard measure of obesity (7). However, a study conducted in an Asian female population found no correlation between BMI and TPOAb (17), highlighting the controversy in using BMI to evaluate the relationship between obesity and thyroid autoantibodies levels. In fact, thyroid autoantibodies levels may be more closely related to metabolic disorders (18). Compared to BMI, WC, and ABSI (A Body Shape Index), WWI may serve as a superior indicator of metabolic status (14, 16, 19, 20). It has been positively associated with overall mortality, cardiovascular disease mortality, hypertension, and type 2 diabetes. Moreover, when used in conjunction with BMI, WWI can better predict the risk of developing cardiovascular metabolic diseases (11). Therefore, we conducted a cross-sectional study using the NHANES database to explore the relationship between WWI and thyroid autoantibodies levels.
The study results indicate that, after adjusting for all confounding factors, WWI was significantly positively correlated with TPOAb but not with TgAb. TPOAb is a more critical marker for HT compared to TgAb (21, 22), as 90% of patients with HT exhibit elevated serum TPOAb levels (23). Consequently, TPOAb is commonly considered the primary marker for evaluating and monitoring HT. Elevated serum TPOAb levels signify an aggressive immune attack on thyroid tissue (24). Additionally, activated lymphocytes may migrate from the thyroid to distant tissues, exacerbating the immune response and inflammation (25). In patients with HT and TPOAb levels exceeding 1,000 IU/mL, inflammation-related cytokines such as interferon-gamma and tumor necrosis factor-alpha are significantly increased, leading to systemic chronic inflammatory responses (26). This persistent immune response against thyroid tissue eventually results in irreversible hypothyroidism. Interestingly, hypothyroidism in obese patients has been shown to be a secondary response to obesity, and weight loss induced by bariatric surgery significantly reduces moderately elevated TSH levels and suppresses hypothyroidism. The degree of suppression improves over time post-surgery and correlates significantly with reductions in BMI (27). Therefore, reducing TPOAb levels by regulating WWI may effectively control thyroid inflammation, systemic inflammation, and hypothyroidism.
In further subgroup analysis, we observed that gender influenced the correlation between WWI and TPOAb levels. The significant positive correlation was evident only in the female group, with no such association found in males. Indeed, the relationship between obesity, thyroid function, and HT exhibits gender-specific differences (28). Studies have shown a positive correlation between obesity and hypothyroidism in Chinese women, whereas this correlation is not observed in men (17). Another study indicated a positive correlation between TSH and BMI specifically in obese women with positive thyroid autoantibodies (29). Our findings suggest that WWI may more significantly impact TPOAb levels in women compared to men. However, the specific mechanisms underlying these gender differences remain unclear. Potential mechanisms may include differences in fat distribution, the interplay between hormonal regulation and the immune system, and metabolic syndrome between genders (30). Women tend to accumulate fat in the lower abdomen and hip regions, while men predominantly accumulate fat in the abdominal area (31). Such differences in fat distribution may influence thyroid function and immune responses.
Estrogen, a key hormonal factor, appears to play a pivotal role in modulating immune system activity (32). Studies indicate that estrogen can exacerbate autoimmune responses in the thyroid among obese women by enhancing B and T cell activity, leading to excessive production of thyroid autoantibodies, including TPOAb (33). Furthermore, estrogen can directly influence thyroid function by binding to receptors in thyroid epithelial cells, thereby affecting their activity (34). It can also synergize with pro-inflammatory cytokines, increasing the risk of developing HT (35).
The relationship between gender differences and metabolic syndrome offers another potential explanation. Metabolic syndrome, which includes conditions such as insulin resistance and hyperlipidemia, is strongly associated with HT. Women with central obesity are generally more prone to metabolic syndrome, and insulin resistance may amplify thyroid tissue damage through pro-inflammatory pathways (36, 37). However, these mechanisms remain speculative and require further investigation to validate these hypotheses and deepen our understanding of the gender-specific effects of WWI on thyroid autoimmunity.
Thyroid dysfunction has long been associated with obesity, initially thought to be a consequence of thyroid hormone deficiency. However, treatment of hypothyroidism typically results in only modest weight loss (less than 10%), suggesting that severe obesity is generally not secondary to thyroid dysfunction (38). Instead, evidence suggests a bidirectional causal relationship between obesity and hypothyroidism, with HT potentially serving as a critical intermediary (37, 39–41). Central obesity, as reflected by a high WWI, may influence immune system activation and contribute to the development of HT (42). Adipose tissue in central obesity acts not only as an energy reservoir but also as a metabolically active endocrine organ, secreting various bioactive substances, including leptin, adiponectin, and pro-inflammatory cytokines (43, 44). Elevated leptin levels, in particular, play a central role in immune regulation by modulating T-cell activation and proliferation, which can amplify immune responses and potentially drive the progression of thyroid autoimmunity (45, 46).
Chronic low-grade inflammation, a hallmark of obesity, further compounds these effects (47). Pro-inflammatory cytokines such as TNF-α and IL-6 exacerbate systemic inflammation, potentially impairing thyroid epithelial cells and dysregulating immune responses (48, 49). Moreover, metabolic dysfunctions commonly associated with central obesity, including insulin resistance and oxidative stress, may create a pro-inflammatory environment that heightens the risk of immune-mediated damage to thyroid tissue (50). Together, these mechanisms underscore the intricate interplay between central obesity, chronic inflammation, and the development of HT, offering valuable insights into the pathophysiology of obesity-related thyroid autoimmunity.
However, we must acknowledge several limitations of this study. Firstly, due to its cross-sectional design, it is not possible to establish a definitive causal relationship between WWI and thyroid autoantibodies. Secondly, the study could not account for all potential covariates. Thirdly, the diagnosis of HT typically requires a combination of thyroid function tests, thyroid autoantibodies, ultrasound findings, clinical symptoms, and pathological results. Since the NHANES database lacks relevant data, our study could only examine the relationship between WWI and thyroid autoantibodies, without directly studying the association between obesity and HT. Lastly, using the NHANES dataset, which is specific to the U.S. population, may limit the generalizability of our findings globally.
Despite the above limitations, this study holds significant clinical value. To begin with, the findings support WWI as an effective tool for assessing obesity and its associated health risks, particularly in evaluating the risk of thyroid autoimmune diseases. Moreover, controlling central obesity may help mitigate thyroid autoimmune responses, offering new clinical approaches for the prevention and management of thyroid inflammation and dysfunction. Finally, the observed gender differences in the relationship between central obesity and thyroid autoimmunity provide important insights for designing personalized clinical interventions tailored to individual risk profiles.
Conclusion
In conclusion, our study demonstrates a significant association between WWI, an indicator of central obesity, and HT, particularly in women. However, to establish causality and better understand the mechanisms involved, higher-level prospective studies are warranted. Longitudinal research would provide valuable insights into how central obesity influences HT over time, potentially guiding more effective preventive and therapeutic strategies for managing autoimmune thyroid diseases.
Data availability statement
The original contributions presented in the study are included in the article/supplementary material, further inquiries can be directed to the corresponding authors.
Ethics statement
The studies involving humans were approved by the Ethics Review Board of the National Center for Health Statistics. The studies were conducted in accordance with the local legislation and institutional requirements. The participants provided their written informed consent to participate in this study.
Author contributions
XW: Conceptualization, Data curation, Formal analysis, Visualization, Writing – original draft. YM: Data curation, Formal analysis, Investigation, Writing – original draft. ZL: Data curation, Investigation, Validation, Writing – original draft. GC: Project administration, Supervision, Writing – review & editing. SZ: Funding acquisition, Project administration, Supervision, Writing – review & editing.
Funding
The author(s) declare that financial support was received for the research, authorship, and/or publication of this article. This research was funded by Hunan Provincial Natural Science Foundation of China (Grant no. 2023JJ40419), Hunan Cancer Hospital Climb Plan (Grant no. ZX2021004), and Changsha Municipal Natural Science Foundation (Grant no. kq2208153).
Acknowledgments
The authors thank the staff and the participants of the NHANES study for their valuable contributions.
Conflict of interest
The authors declare that the research was conducted in the absence of any commercial or financial relationships that could be construed as a potential conflict of interest.
Generative AI statement
The authors declare that no Generative AI was used in the creation of this manuscript.
Publisher’s note
All claims expressed in this article are solely those of the authors and do not necessarily represent those of their affiliated organizations, or those of the publisher, the editors and the reviewers. Any product that may be evaluated in this article, or claim that may be made by its manufacturer, is not guaranteed or endorsed by the publisher.
Abbreviations
WWI, weight-adjusted waist index; NHANES, National Health and Nutrition Examination Survey; TPOAb, thyroid peroxidase antibodies; TgAb, thyroglobulin antibodies; HT, Hashimoto’s thyroiditis; BMI, Body Mass Index; WC, Waist Circumference; PIR, Poverty Income Ratio; HDL-C, High-density lipoprotein cholesterol.
Footnotes
References
1. Choi, S, Kim, MJ, Park, YJ, Kim, S, Choi, K, Cheon, GJ, et al. Thyroxine-binding globulin, peripheral deiodinase activity, and thyroid autoantibody status in association of phthalates and phenolic compounds with thyroid hormones in adult population. Environ Int. (2020) 140:105783. doi: 10.1016/j.envint.2020.105783
2. Roberts, CG, and Ladenson, PW. Hypothyroidism. Hypothyroidism Lancet. (2004) 363:793–803. doi: 10.1016/S0140-6736(04)15696-1
3. Guo, Y, Zynat, J, Xing, S, Xin, L, Li, S, Mammat, N, et al. Immunological changes of T helper cells in flow cytometer-sorted CD4(+) T cells from patients with Hashimoto's thyroiditis. Exp Ther Med. (2018) 15:3596–602. doi: 10.3892/etm.2018.5825
4. Spalding, KL, Arner, E, Westermark, PO, Bernard, S, Buchholz, BA, Bergmann, O, et al. Dynamics of fat cell turnover in humans. Nature. (2008) 453:783–7. doi: 10.1038/nature06902
5. Green, WD, and Beck, MA. Obesity impairs the adaptive immune response to influenza virus. Ann Am Thorac Soc. (2017) 14:S406–9. doi: 10.1513/AnnalsATS.201706-447AW
6. Alfaradhi, MZ, Kusinski, LC, Fernandez-Twinn, DS, Pantaleão, LC, Carr, SK, Ferland-McCollough, D, et al. Maternal obesity in pregnancy developmentally programs adipose tissue inflammation in young. Lean Male Mice Offspring Endocrinol. (2016) 157:4246–56. doi: 10.1210/en.2016-1314
7. Song, RH, Wang, B, Yao, QM, Li, Q, Jia, X, and Zhang, JA. The impact of obesity on thyroid autoimmunity and dysfunction: a systematic review and Meta-analysis. Front Immunol. (2019) 10:2349. doi: 10.3389/fimmu.2019.02349
8. Kangas, S, Timonen, P, Knuuttila, M, Jula, A, Ylöstalo, P, and Syrjälä, AH. Waist circumference and waist-to-height ratio are associated with periodontal pocketing-results of the health 2000 survey. BMC Oral Health. (2017) 17:48. doi: 10.1186/s12903-017-0336-y
9. Prillaman, M. Why BMI is flawed – and how to redefine obesity. Nature. (2023) 622:232–3. doi: 10.1038/d41586-023-03143-x
10. Kim, KJ, Son, S, Kim, KJ, Kim, SG, and Kim, NH. Weight-adjusted waist as an integrated index for fat, muscle and bone health in adults. J Cachexia Sarcopenia Muscle. (2023) 14:2196–203. doi: 10.1002/jcsm.13302
11. Park, Y, Kim, NH, Kwon, TY, and Kim, SG. A novel adiposity index as an integrated predictor of cardiometabolic disease morbidity and mortality. Sci Rep. (2018) 8:16753. doi: 10.1038/s41598-018-35073-4
12. Skrivankova, VW, Richmond, RC, Woolf, BAR, Yarmolinsky, J, Davies, NM, Swanson, SA, et al. Strengthening the reporting of observational studies in epidemiology using Mendelian randomization: the STROBE-MR statement. JAMA. (2021) 326:1614–21. doi: 10.1001/jama.2021.18236
13. Zheng, J, Zhu, X, Xu, G, Wang, X, Cao, M, Zhu, S, et al. Relationship between caffeine intake and thyroid function: results from NHANES 2007-2012. Nutr J. (2023) 22:36. doi: 10.1186/s12937-023-00866-5
14. Ye, J, Hu, Y, Chen, X, Yin, Z, Yuan, X, Huang, L, et al. Association between the weight-adjusted waist index and stroke: a cross-sectional study. BMC Public Health. (2023) 23:1689. doi: 10.1186/s12889-023-16621-8
15. Guan, H, de Morais, NS, Stuart, J, Ahmadi, S, Marqusee, E, Kim, MI, et al. Discordance of serological and sonographic markers for Hashimoto's thyroiditis with gold standard histopathology. Eur J Endocrinol. (2019) 181:539–44. doi: 10.1530/EJE-19-0424
16. Wang, X, Yang, S, He, G, and Xie, L. The association between weight-adjusted-waist index and total bone mineral density in adolescents: NHANES 2011-2018. Front Endocrinol. (2023) 14:1191501. doi: 10.3389/fendo.2023.1191501
17. Wang, B, Song, R, He, W, Yao, Q, Li, Q, Jia, X, et al. Sex differences in the associations of obesity with hypothyroidism and thyroid autoimmunity among Chinese adults. Front Physiol. (2018) 9:1397. doi: 10.3389/fphys.2018.01397
18. Wu, Y, Shi, X, Tang, X, Li, Y, Tong, N, Wang, G, et al. The correlation between metabolic disorders and Tpoab/Tgab: a cross-sectional population-based study. Endocr Pract. (2020) 26:869–82. doi: 10.4158/EP-2020-0008
19. Lin, FX, and Xu, ZP. WWI: a novel lens on kidney stone risk prediction. World J Urol. (2023) 42:1. doi: 10.1007/s00345-023-04753-w
20. Qin, Z, Du, D, Li, Y, Chang, K, Yang, Q, Zhang, Z, et al. The association between weight-adjusted-waist index and abdominal aortic calcification in adults aged ≥ 40 years: results from NHANES 2013-2014. Sci Rep. (2022) 12:20354. doi: 10.1038/s41598-022-24756-8
21. Azizi, G, Keller, JM, Lewis, M, Piper, K, Puett, D, Rivenbark, KM, et al. Association of Hashimoto's thyroiditis with thyroid cancer. Endocr Relat Cancer. (2014) 21:845–52. doi: 10.1530/ERC-14-0258
22. Ni, J, Li, J, Wang, Y, Guan, L, Lin, H, Zhang, L, et al. Systemic lupus erythematosus patients with related organic damage are at high risk of hypothyroidism. Front Endocrinol. (2022) 13:920283. doi: 10.3389/fendo.2022.920283
23. Pearce, EN, Farwell, AP, and Braverman, LE. Thyroiditis. N Engl J Med. (2003) 348:2646–55. doi: 10.1056/NEJMra021194
24. Kwak, SH, Park, YJ, Go, MJ, Lee, KE, Kim, SJ, Choi, HS, et al. A genome-wide association study on thyroid function and anti-thyroid peroxidase antibodies in Koreans. Hum Mol Genet. (2014) 23:4433–42. doi: 10.1093/hmg/ddu145
25. Weetman, AP, McGregor, AM, Lazarus, JH, and Hall, R. Thyroid antibodies are produced by thyroid-derived lymphocytes. Clin Exp Immunol. (1982) 48:196–200.
26. Karanikas, G, Schuetz, M, Wahl, K, Paul, M, Kontur, S, Pietschmann, P, et al. Relation of anti-TPO autoantibody titre and T-lymphocyte cytokine production patterns in Hashimoto's thyroiditis. Clin Endocrinol. (2005) 63:191–6. doi: 10.1111/j.1365-2265.2005.02324.x
27. Juiz-Valiña, P, Outeiriño-Blanco, E, Pértega, S, Varela-Rodriguez, BM, García-Brao, MJ, Mena, E, et al. Effect of weight loss after bariatric surgery on thyroid-stimulating hormone levels in euthyroid patients with morbid obesity. Nutrients. (2019) 11:1121. doi: 10.3390/nu11051121
28. Nie, X, Ma, X, Xu, Y, Shen, Y, Wang, Y, and Bao, Y. Characteristics of serum thyroid hormones in different metabolic phenotypes of obesity. Front Endocrinol. (2020) 11:68. doi: 10.3389/fendo.2020.00068
29. Velluzzi, F, Pisanu, S, Galletta, M, Fosci, M, Secci, G, Deledda, A, et al. Association between high Normal TSH levels and obesity in women with anti-thyroid autoantibodies (ATAs). J Clin Med. (2022) 11:5125. doi: 10.3390/jcm11175125
30. Ge, X, Zhu, T, Zhang, X, Liu, Y, Wang, Y, and Zhang, W. Gender differences in pulmonary arterial hypertension patients with BMPR2 mutation: a meta-analysis. Respir Res. (2020) 21:44. doi: 10.1186/s12931-020-1309-2
31. Hodson, L, Banerjee, R, Rial, B, Arlt, W, Adiels, M, Boren, J, et al. Menopausal status and abdominal obesity are significant determinants of hepatic lipid metabolism in women. J Am Heart Assoc. (2015) 4:e002258. doi: 10.1161/JAHA.115.002258
32. Ribeiro-Dasilva, MC, Fillingim, RB, and Wallet, SM. Estrogen-induced monocytic response correlates with TMD pain: a case control study. J Dent Res. (2017) 96:285–91. doi: 10.1177/0022034516678599
33. Zandman-Goddard, G, Peeva, E, and Shoenfeld, Y. Gender and autoimmunity. Autoimmun Rev. (2007) 6:366–72. doi: 10.1016/j.autrev.2006.10.001
34. Chakraborty, B, Byemerwa, J, Krebs, T, Lim, F, Chang, CY, and McDonnell, DP. Estrogen receptor signaling in the immune system. Endocr Rev. (2023) 44:117–41. doi: 10.1210/endrev/bnac017
35. Moulton, VR. Sex hormones in acquired immunity and autoimmune disease. Front Immunol. (2018) 9:2279. doi: 10.3389/fimmu.2018.02279
36. Biondi, B, Kahaly, GJ, and Robertson, RP. Thyroid dysfunction and diabetes mellitus: two closely associated disorders. Endocr Rev. (2019) 40:789–824. doi: 10.1210/er.2018-00163
37. Kim, HJ, Park, SJ, Park, HK, Byun, DW, Suh, K, and Yoo, MH. Thyroid autoimmunity and metabolic syndrome: a nationwide population-based study. Eur J Endocrinol. (2021) 185:707–15. doi: 10.1530/EJE-21-0634
38. Santini, F, Marzullo, P, Rotondi, M, Ceccarini, G, Pagano, L, Ippolito, S, et al. Mechanisms in endocrinology: the crosstalk between thyroid gland and adipose tissue: signal integration in health and disease. Eur J Endocrinol. (2014) 171:R137–52. doi: 10.1530/EJE-14-0067
39. García-Solís, P, García, OP, Hernández-Puga, G, Sánchez-Tusie, AA, Sáenz-Luna, CE, Hernández-Montiel, HL, et al. Thyroid hormones and obesity: a known but poorly understood relationship. Endokrynol Pol. (2018) 69:292–303. doi: 10.5603/EP.2018.0032
40. Yan, Y, Xu, M, Wu, M, Wang, X, Li, F, Zhang, J, et al. Obesity is associated with subclinical hypothyroidism in the presence of thyroid autoantibodies: a cross-sectional study. BMC Endocr Disord. (2022) 22:94. doi: 10.1186/s12902-022-00981-0
41. Amouzegar, A, Kazemian, E, Abdi, H, Gharibzadeh, S, Tohidi, M, and Azizi, F. Abdominal obesity phenotypes and incidence of thyroid autoimmunity: a 9-year follow-up. Endocr Res. (2020) 45:202–9. doi: 10.1080/07435800.2020.1749847
42. Yang, H, Xia, Q, Shen, Y, Chen, TL, Wang, J, and Lu, YY. Gender-specific impact of metabolic obesity phenotypes on the risk of Hashimoto's thyroiditis: a retrospective data analysis using a health check-up database. J Inflamm Res. (2022) 15:827–37. doi: 10.2147/JIR.S353384
43. Kershaw, EE, and Flier, JS. Adipose tissue as an endocrine organ. J Clin Endocrinol Metab. (2004) 89:2548–56. doi: 10.1210/jc.2004-0395
44. Lago, F, Dieguez, C, Gómez-Reino, J, and Gualillo, O. Adipokines as emerging mediators of immune response and inflammation. Nat Clin Pract Rheumatol. (2007) 3:716–24. doi: 10.1038/ncprheum0674
45. Marzullo, P, Minocci, A, Tagliaferri, MA, Guzzaloni, G, Di Blasio, A, De Medici, C, et al. Investigations of thyroid hormones and antibodies in obesity: leptin levels are associated with thyroid autoimmunity independent of bio-anthropometric, hormonal, and weight-related determinants. J Clin Endocrinol Metab. (2010) 95:3965–72. doi: 10.1210/jc.2009-2798
46. Duntas, LH, and Biondi, B. The interconnections between obesity, thyroid function, and autoimmunity: the multifold role of leptin. Thyroid. (2013) 23:646–53. doi: 10.1089/thy.2011.0499
47. Ouchi, N, Parker, JL, Lugus, JJ, and Walsh, K. Adipokines in inflammation and metabolic disease. Nat Rev Immunol. (2011) 11:85–97. doi: 10.1038/nri2921
48. Lin, L, Lee, JH, Buras, ED, Yu, K, Wang, R, Smith, CW, et al. Ghrelin receptor regulates adipose tissue inflammation in aging. Aging. (2016) 8:178–91. doi: 10.18632/aging.100888
49. Simons, PJ, van den Pangaart, PS, van Roomen, CP, Aerts, JM, and Boon, L. Cytokine-mediated modulation of leptin and adiponectin secretion during in vitro adipogenesis: evidence that tumor necrosis factor-alpha- and interleukin-1beta-treated human preadipocytes are potent leptin producers. Cytokine. (2005) 32:94–103. doi: 10.1016/j.cyto.2005.08.003
Keywords: weight-adjusted waist index, central obesity, Hashimoto’s thyroiditis, thyroid peroxidase antibodies, NHANES
Citation: Wen X, Mao Y, Li Z, Chen G and Zhou S (2025) Association between weight-adjusted waist index and Hashimoto’s thyroiditis: insights from NHANES 2007–2012. Front. Nutr. 11:1520440. doi: 10.3389/fnut.2024.1520440
Edited by:
Genco Görgü, Ministry of Health, TürkiyeReviewed by:
Sedat Arslan, Bandirma Onyedi Eylül University, TürkiyeXiabin Lan, Zhejiang Cancer Hospital, China
Copyright © 2025 Wen, Mao, Li, Chen and Zhou. This is an open-access article distributed under the terms of the Creative Commons Attribution License (CC BY). The use, distribution or reproduction in other forums is permitted, provided the original author(s) and the copyright owner(s) are credited and that the original publication in this journal is cited, in accordance with accepted academic practice. No use, distribution or reproduction is permitted which does not comply with these terms.
*Correspondence: Guangji Chen, Y2hlbmd1YW5namlAY3N1LmVkdS5jbg==; Shiwei Zhou, emhvdXNoaXdlaUBobmNhLm9yZy5jbg==