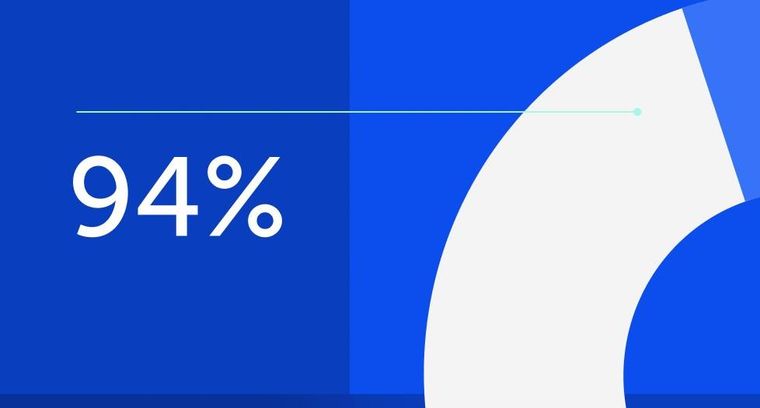
94% of researchers rate our articles as excellent or good
Learn more about the work of our research integrity team to safeguard the quality of each article we publish.
Find out more
ORIGINAL RESEARCH article
Front. Nutr., 02 October 2024
Sec. Nutritional Epidemiology
Volume 11 - 2024 | https://doi.org/10.3389/fnut.2024.1453064
Background: Sleep disorders have emerged as a major public health concern. Observational research indicates that antioxidants might mitigate the risk of sleep disturbances, yet the causal relationship remains uncertain.
Materials and methods: This study utilized data from the National Health and Nutrition Examination Survey (NHANES) spanning 2007 to 2018, focusing on adults who reported sleep disorders. The analysis included 25,178 American adults. We examined the association between the Composite Dietary Antioxidant Index (CDAI) and the prevalence of sleep disorders. Additionally, a two-sample Mendelian randomization analysis was conducted to explore the potential causal link between CDAI and the risk of sleep disorders.
Results: Analysis of data from the 2007–2018 NHANES survey revealed a significant negative association between CDAI and sleep disorders (OR = 0.854, 95% CI 0.821–0.888, P < 0.001). A multivariable logistic regression model showed that each unit increase in CDAI corresponded to a 14.6% reduction in sleep disorder risk, exhibiting a nonlinear trend where the risk decreased until reaching the inflection point of −0.134. Additionally, MR analysis demonstrated that genetically determined selenium reduces the risk of OSA (OR = 0.992, 95% CI 0.860–0.989, P = 0.023). Furthermore, vitamin E (γ-tocopherol) and vitamin C were protective against sleep-wake disorders (OR = 0.016, 95% CI 0.001–0.674, P = 0.03) and (OR = 0.049, 95% CI 0.007–0.346, P = 0.002), respectively.
Conclusion: Dietary antioxidants may help prevent sleep disorders. However, further studies are required to clarify the pathways through which antioxidants exert this protective effect.
Sleep is a ubiquitous behavior across species, with most people spending about one-third of their day sleeping. It is considered a crucial determinant of human health and performance (1, 2). Sleep disorders are among the most common clinical issues. According to a survey by the World Health Organization, approximately 27% of the world’s population experiences sleep disturbances. The China Sleep Research Society reports that over 300 million Chinese people experience sleep disorders, making China a country with a high rate of sleep problems. The prevalence of sleep disorders is continually increasing due to lifestyle changes, such as night shifts and the frequent use of electronic devices (3–5). Sleep deprivation disrupts physical, psychological, social, and emotional functions, impacting health, safety, and quality of life. Research indicates that individuals suffering from insomnia experience a notably impaired quality of life. The American Sleep Medicine Association and the Society for Sleep Research indicate that sleep disorders are closely associated with cardiovascular diseases, mental health disorders, immune system diseases, cancer, pain, mortality, and overall health (6, 7). However, sleep health remains underrecognized and is a challenging topic in public health. Even after decades of specialized research, the mechanisms regulating human sleep and its functions largely remain elusive. Studies have shown that in animal models, sleep deprivation induces cellular oxidative stress, which can be mitigated by various antioxidants (8, 9). Studies have shown that vitamin C possesses immunomodulatory and antioxidant properties (10, 11). Vitamin E, as a potent antioxidant, can prevent oxidative stress and provides neuroprotective effects for the brain (12, 13). Current research mainly investigates how specific antioxidants impact sleep disorders, but the findings are inconsistent. Notably, meta-analyses have indicated that increasing antioxidant supplements is not always beneficial and can sometimes even increase mortality rates (14).
The Composite Dietary Antioxidant Index (CDAI) is considered an extensive measure for evaluating overall dietary antioxidant capacity. Its calculation is based on six standardized vitamins and minerals, including vitamins A, C, and E, selenium, zinc, and carotenoids derived exclusively from dietary sources (15).
The objective of this research was to evaluate whether CDAI has a preventive effect on the likelihood of sleep disorders. We initially examined data from the National Health and Nutrition Examination Survey (NHANES) to investigate the potential association between CDAI and sleep disorders. Subsequently, we employed the Mendelian randomization (MR) technique, incorporating data from genome-wide association studies (GWAS) on six antioxidants and two subtypes of sleep disturbances, to further assess the causal link between antioxidants and sleep disorder risk. This approach aimed to establish the possible causal impact of antioxidants on the likelihood of developing sleep disturbances.
The NHANES survey, which included questionnaires, examination data, and laboratory test results, was conducted with the approval of the National Center for Health Statistics (NCHS) Institutional Review Board. Biennial data releases ensured up-to-date information, and all participants provided written informed consent. The data for these cross-sectional surveys were obtained from the NCHS and are accessible at https://www.cdc.gov/nchs/index.htm. Additionally, we performed a two-sample Mendelian randomization analysis to investigate the causal relationship between dietary antioxidants and different subtypes of sleep disorders.
From 2007 to 2018, the NHANES survey included 50,588 participants. We excluded participants according to specific criteria in our analysis, leading to a final sample size of 25,178 subjects (Supplementary Figure 1). The main outcome measured was the incidence of sleep disorders, defined by responses to the medical conditions questionnaire item: “Over the last two weeks, how often have you been bothered by the following problems: trouble falling asleep or staying asleep, or sleeping too much?” A response of “More than half the days” or “Nearly every day” signified a sleep disorder. On the other hand, answers of “Several days” or “Not at all” showed that no sleep disorder was present.
CDAI was used as the exposure variable, derived from the sum of standardized intakes of six dietary antioxidants (vitamins A, C, and E, selenium, zinc, and carotenoids from food sources). Dietary intake data was obtained through a detailed dietary interview, which assessed the variety and amounts of foods and beverages consumed by respondents in the 24 h before the interview. We also gathered covariates to minimize bias, including demographic factors such as age, sex, ethnicity, level of education, marital status, and household income. Additionally, we documented risk factors associated with sleep disorders, including body mass index (BMI), smoking habits, alcohol use, hypertension, and diabetes. The inclusion and exclusion criteria are detailed in Supplementary Figure 1. The criteria used to diagnose these covariates can be found in Supplementary Table 1.
Our methods and findings are reported according to the MR-STROBE checklist (Supplemental File). The research framework and the three fundamental assumptions of Mendelian randomization (MR) are illustrated in Supplementary Figure 2. We utilized data from separate, extensive genome-wide association studies (GWAS) on sleep and circulating antioxidants to evaluate the causal relationships between them. Detailed sources are available in Supplementary Table 2. We used genetic instruments for circulating antioxidants in CDAI, including vitamins A, C, and E (which contains both α-tocopherol and γ-tocopherol), as well as selenium and zinc. Additionally, we used carotene instead of carotenoids, as carotene is the predominant form present in human circulation (16). To identify genetic variants that estimate the causal effects of Vitamins A, C, and E (containing both α-tocopherol and γ-tocopherol), as well as selenium, zinc, and carotene on sleep disorders, we set a significance threshold of P < 5 × 10–6 to identify single-nucleotide polymorphisms (SNPs) strongly associated with these exposures. We also excluded SNPs with linkage disequilibrium (LD distance > 10,000 kb, r2 < 0.001). Subsequently, we used the PhenoScanner database to examine each SNP and remove those significantly associated with potential confounders and other sleep disorder-related traits. Sleep disorders as outcome variables encompass a range of conditions, including obstructive sleep apnea (OSA), and sleep-wake disorders. To better understand these conditions, we analyzed different subgroups of sleep disorders. To prevent bias, we ensured that the exposure and outcome cohorts had no overlapping samples.
In our NHANES analysis, we utilized multivariable-adjusted logistic regression to investigate the relationship between CDAI and sleep disorders. We evaluated three covariate adjustment models: Model 1 was unadjusted, Model 2 was adjusted for gender, age, and ethnicity, while Model 3 included additional variables.
Due to NHANES’s complex, multi-stage, probability sampling design, this study accounted for weights in the statistical analysis to address unequal selection probabilities. All participants completed the first day’s dietary recall, so the “minimum common denominator” rule was used to determine the “day 1 dietary sample weight.” Since the data covered 12 years across 6 cycles, the weight was calculated as 1/6 * wtdrd 1.
For the two-sample MR analysis, the Inverse-Variance Weighted (IVW) method was primarily used to evaluate the causal link between genetically predicted CDAI and sleep disorders (17). Additionally, four supplementary MR methods—MR Egger, weighted median, weighted mode, and MR-PRESSO—were employed to verify the robustness of the IVW results. Since IVW estimates may be biased due to the inclusion of pleiotropic instruments, sensitivity analyses were performed to address pleiotropy in the causal estimates (18, 19).
From the 2007–2018 NHANES database, this study enrolled 25,178 individuals. We analyzed the baseline characteristics of those with and without sleep disorders (Table 1). Among these participants, 4,063 reported having sleep disorders. Compared to healthy controls, those with sleep disorders tended to be female, have lower education levels, be White, unmarried, obese (BMI ≥ 30), have lower household income, smoke, drink and have diabetes and hypertension. Participants with sleep disorders had lower CDAI scores than the control group. Intake of vitamins A, C, E, zinc, selenium, and carotenoids was found to have a protective effect against sleep disorders.
In this study, we constructed three weighted logistic regression models to examine the relationship between CDAI and sleep disorders (Table 2). Model 1 indicated that each unit rise in CDAI corresponded to a 14.6% decrease in the risk of sleep disorders (OR = 0.854, 95% CI 0.821–0.888, P < 0.001). Model 2, adjusted for age, gender, and ethnicity, showed a 14.3% decrease in sleep disorder risk (OR = 0.857, 95% CI 0.824–0.892, P < 0.001). In Model 3, which included additional variables, each unit increase in CDAI was linked to a 10% reduction in sleep disorder risk (OR = 0.900, 95% CI 0.866–0.937, P < 0.001).
During the sensitivity analysis, CDAI was divided into four categories for trend analysis (Supplementary Table 3). Similarly, the six elements included in CDAI were also divided into four categories (Supplementary Tables 10–15). The results indicated that higher CDAI levels significantly decreased the risk of sleep disorders (P < 0.001) (Table 2). Further investigation examined the link between the six dietary components in CDAI and sleep disorders. The findings showed that Vitamins A, C, and E (containing both α-tocopherol and γ-tocopherol), as well as selenium, zinc, and carotene, had a influence on sleep disorders after controlling for confounders (Supplementary Tables 4–9). Additionally, RCS model, adjusted for other variables, revealed a nonlinear relationship between CDAI and sleep disorder risk, indicating a saturation effect (nonlinearity P < 0.001) (Figure 1). The inflection point was −0.134; beyond this, each unit increase in CDAI was linked to an 13.1% reduction in sleep disorder risk (OR = 0.869, 95% CI 0.832–0.907, P < 0.001). Beyond this point, the association reversed but was not statistically meaningful (OR = 1.008, 95% CI 0.992–1.025, P = 0.328).
Figure 1. Restricted cubic spline plot of the association between CDAI and sleep disorder. All variables are taken into account and adjusted accordingly. The shaded part represented the 95% CI. CDAI, Composite Dietary Antioxidant Index, OR, odds ratio.
To explore the potential cause-and-effect link between antioxidants and sleep disorders, we conducted an MR analysis. The IV summaries for antioxidants are provided in Supplementary Table 16. The IVW results indicated that selenium (Se) has a protective role in OSA (OR = 0.992, 95% CI 0.860–0.989, P = 0.023). Both Vitamin E (γ-tocopherol) and Vitamin C showed positive effects on sleep-wake cycles (OR = 0.016, 95% CI 0.001–0.674, P = 0.03) and (OR = 0.049, 95% CI 0.007–0.346, P = 0.002), respectively (Figures 2, 3). However, in the other four MR methods, no associations between antioxidants and the likelihood of OSA and sleep-wake cycles were observed (Supplementary Table 17). Subsequently, we conducted a sensitivity analysis of antioxidants on sleep disorders. The findings indicated potential SNP heterogeneity in the MR-Egger analysis of Vitamin E (α-tocopherol) and OSA (P = 0.029). No evidence of horizontal pleiotropy was detected (Supplementary Tables 18, 19), and the MR-PRESSO analysis revealed no outliers. Additionally, the leave-one-out analysis showed consistent SNP risk estimates when each SNP was excluded individually (Supplementary Figures 3–5), suggesting the robustness of our results.
Figure 2. Forest plot for the causal effect of diet-derived antioxidant on the risk of sleep disorder derived from inverse variance weighted (IVW). OR, odds ratio, CI, confidence interval, OSA, obstructive sleep apnea.
Figure 3. Presents scatter plots from Mendelian randomization (MR) analyses, assessing the impact of various nutrients on sleep-related conditions: (A) effect of selenium on obstructive sleep apnea (OSA). (B) Effect of vitamin C on sleep-wake. (C) Effect of vitamin E (γ-tocopherol) on sleep-wake.
According to available literature, this investigation uniquely combines a large-scale cross-sectional and MR method to investigate the link between antioxidant intake and sleep disorder risk. The research delves into the association and potential causality between antioxidant diets and sleep disorders.
Our findings indicate that antioxidant diets may help prevent sleep disorders, providing clinical evidence for the benefits of increased antioxidant intake for sleep health. This study employed data from five NHANES cycles (2007–2018) to explore the relationship linking antioxidant diets with sleep issues. Three models were developed, and the results consistently indicated that higher antioxidant dietary intake was linked to a lower occurrence of sleep disturbances, even after adjusting for various covariates. In the RCS model, this negative association was nonlinear, with a turning point at −0.134.
In our MR analysis, we utilized extensive GWAS datasets to explore the causal links. The findings indicated a causal relationship between selenium and OSA, and between vitamin C and vitamin E with sleep awakenings. However, no significant causal links were identified between other antioxidants and these sleep-related issues. Using the IVW method as the primary analysis, the findings revealed protective effects of genetically determined selenium on OSA, vitamin C and vitamin E on sleep awakenings.
Earlier research has mainly concentrated on the link between individual antioxidants and sleep disorders, with relatively few studies assessing the impact of total dietary antioxidant capacity (DTAC) on sleep disorders. A survey conducted by Chinese researchers identified an increased occurrence of sleep disorders in children with reduced serum vitamin A levels. Similarly, a questionnaire-based study among Japanese students found a link between vitamin A consumption and sleep disturbances (20). Animal experiments by Sei et al. (21) showed that non-rapid eye movement (NREM) sleep significantly improved in vitamin A-deficient (VAD) mice after normal diet restoration, indicating that vitamin A supplementation can enhance sleep quality. Kanagasabai and Ardern (22) found that shorter or very short sleep durations significantly reduced vitamin C levels compared to 7–8 h of adequate sleep. Current studies on how vitamin E affects sleep show inconsistent results. Research by Martens et al. (23) suggested a link between poor sleep health and high serum vitamin E levels. In contrast, Alzoubi et al. (13) found that long-term vitamin E supplementation had protective effects against cognitive impairment caused by chronic sleep deprivation. They also identified vitamin E as a potential molecular target for cognitive impairment due to chronic sleep deprivation. Interestingly, the connection between zinc and sleep exhibits similar trends. A 2009 survey conducted in Jinan, China, assessed serum zinc/copper concentrations and sleep patterns among 890 residents, revealing that adults who slept 7–9 h nightly exhibited the highest serum zinc concentrations (24). Another study in China involving 1,295 children found no notable link between blood zinc levels and sleep quality (25). An Italian randomized, controlled trial discovered that combining zinc with melatonin and magnesium enhanced sleep quality in individuals with primary insomnia (26). A 2021 study revealed that ongoing sleep deprivation impairs both immediate and delayed memory, while selenium supplementation could mitigate this harm (27). Research by Beydoun et al. (28), involving 2,459 adults aged 20–51, found a high prevalence of sleep disorders linked to reduced concentrations of vitamins A, C, E, and carotenoids. This aligns with our study’s findings and further confirms the protective effects of antioxidant diets on sleep disorders.
Our study demonstrates a negative correlation between higher dietary antioxidant intake and a lower incidence of sleep disorders. This association suggests that increasing the intake of antioxidants in daily diets may help prevent or reduce the occurrence of sleep disorders. This preventive effect can be understood as dietary antioxidants alleviating physiological and psychological stress related to sleep disorders through their antioxidant properties and neuroprotective effects, thereby reducing the risk of sleep disorders.
In further analysis, we confirmed a nonlinear relationship between the Composite Dietary Antioxidant Index (CDAI) and sleep disorders. Before the inflection point, each unit increase in CDAI reduced the likelihood of sleep disorders by 11.3%. However, beyond this point, the association reversed and became statistically insignificant (p-value > 0.05).
Our research further reveals the potential biological mechanisms by which dietary antioxidants affect sleep disorders. These antioxidants not only directly influence sleep quality through their antioxidant and neuroprotective effects but also play an important role in sleep disorder risk through interactions with genetic variations. Specifically, antioxidants such as vitamins A, C, and E, as well as zinc and selenium, regulate neurotransmitter levels, brain function, and related gene expression through various physiological and biochemical pathways, thereby exerting their effects on sleep regulation. The following sections will discuss these mechanisms in detail and their roles in the prevention of sleep disorders.
Vitamin A and its derivatives (e.g., retinoic acid, RA) regulate sleep through various mechanisms (29). RA influences EEG delta activity during NREM sleep, directly related to sleep demand, intensity, and continuity. RA plays a key role in synaptic plasticity, learning, and memory, impacting hippocampal long-term potentiation (LTP) and depression (LTD), and regulating neurotransmitters like dopamine and serotonin. Lack of sleep greatly influences RA-related gene activity, suggesting that RA signaling plays a role in sleep recovery (30–34). Vitamin C (VC) is crucial for neurotransmitter synthesis and mood regulation, functioning as an essential element for dopamine β-hydroxylase and tryptophan-5-hydroxylase, facilitating the conversion of dopamine to norepinephrine (NE) and tryptophan to 5-hydroxytryptophan (35). Chronic vitamin C deficiency lowers NE and serotonin levels, potentially leading to depression, a major psychological cause of insomnia. Additionally, vitamin C’s antioxidant properties protect the nervous system from oxidative damage. Supplementation benefits stress-related conditions like depression and anxiety, often associated with insomnia (36). As a potent antioxidant, vitamin E influences the hypothalamic orexin system through its α-tocopherol component, activating Nrf2 and triggering antioxidant response elements (ARE), enhancing cellular antioxidant capacity (37, 38). This activation increases hypothalamic orexin expression and turnover, generating orexin-specific peptides, providing neuroprotection and potentially improving sleep quality (39, 40). Zinc’s limited permeability through the blood-brain barrier enables swift entry into the central nervous system, potentially triggering sleep-promoting pathways (41). Zinc functions through the GHS-R-related gene (GPR39), a receptor abundantly found in the amygdala, hippocampus, and auditory cortex, influencing neuronal activity (42, 43). Deficiency in GPR39 is linked to depression-like symptoms and a higher risk of Alzheimer’s disease, both of which are closely connected to sleep disorders (44–47). Selenium, functioning as a cofactor for antioxidant enzymes such as GPx, mitigates memory deficits in sleep-deprived rats by stabilizing oxidative stress in the hippocampus. This is demonstrated by the normalization of antioxidant enzyme functions and oxidative stress indicators (including GSH/GSSG ratio, GPx, catalase, and SOD) following selenium administration (27, 48, 49).
Through Mendelian randomization analysis, we also identified causal relationships between specific antioxidants (such as selenium, vitamin C, and vitamin E) and sleep disorders. This suggests that certain individuals may have a genetically higher demand or sensitivity to these antioxidants. The interaction between these genetic variations and antioxidant intake may influence the risk of sleep disorders. Therefore, this is not merely a simple association between dietary antioxidant intake and sleep disorders; it also involves complex gene-environment interactions, particularly between genetic predispositions and dietary habits.
In summary, the significant negative association observed in our study may reflect both the preventive effect of dietary antioxidants on sleep disorders and the low genetic interaction between antioxidant intake and sleep disorders. This suggests that higher antioxidant intake could reduce the risk of sleep disorders through both direct physiological mechanisms and indirect genetic interactions.
These findings offer greater insight into how antioxidants impact sleep. Additional studies are required to clarify these connections and uncover the underlying biological processes.
Our study presents several strengths. Firstly, integrating cross-sectional data with Mendelian randomization (MR) analysis diminishes the impact of confounding variables and reduces the chance of reverse causation. Secondly, the extensive sample size helps to minimize sampling bias and enhances the robustness of our findings. Additionally, by dietary intervention, we established a comprehensive index that fully considers the interactions between different antioxidant nutrients. Finally, we analyzed the relationships between three subtypes of sleep disorders and antioxidants in our MR analysis. Nevertheless, our study has its limitations. Firstly, the NHANES study examined various racial groups within the US population, while the MR data is derived from a European population. Further investigation is needed to determine whether the conclusions are applicable to other racial or population groups. Consequently, future research should focus on a more homogenous population, and further research is necessary in larger and more diverse populations. Secondly, NHANES identifies sleep disorders through questionnaire surveys, which may underestimate the prevalence of the disease. Moreover, the antioxidants from dietary sources were estimated based on the 24-h recall prior to the interviews, without dynamic follow-up. Finally, our study did not perform reverse analysis to investigate whether sleep disorders could lead to reduced intake of antioxidants. Although there has been extensive discussion about the connection between antioxidant diets and sleep disturbances, our study did not offer concrete proof to confirm this hypothesis.
In this study combining NHANES cross-sectional data and MR analysis, dietary antioxidants may have a protective effect against sleep disorders, with selenium being beneficial for OSA, and vitamins C and E being advantageous for sleep awakening. Nevertheless, additional studies are essential to elucidate the mechanisms through which antioxidants prevent sleep disorders. Concurrently, we aim to develop innovative preventive measures and recommendations to reduce the impact of sleep issues on human health.
The original contributions presented in this study are included in this article/Supplementary material, further inquiries can be directed to the corresponding author.
This article does not contain any studies with human participants or animals performed by any of the authors.
JJ: Writing – original draft, Writing – review & editing, Software. DL: Writing – review & editing, Formal analysis. TH: Writing – review & editing, Formal analysis. SH: Writing – review & editing, Formal analysis. HT: Writing – review & editing, Software. ZX: Writing – review & editing, Supervision.
The authors declare that no financial support was received for the research, authorship, and/or publication of this article.
The authors declare that the research was conducted in the absence of any commercial or financial relationships that could be construed as a potential conflict of interest.
All claims expressed in this article are solely those of the authors and do not necessarily represent those of their affiliated organizations, or those of the publisher, the editors and the reviewers. Any product that may be evaluated in this article, or claim that may be made by its manufacturer, is not guaranteed or endorsed by the publisher.
The Supplementary Material for this article can be found online at: https://www.frontiersin.org/articles/10.3389/fnut.2024.1453064/full#supplementary-material
NHANES, National Health and Nutrition Examination Survey; MR, Mendelian randomization; CDAI, The Composite Dietary Antioxidant Index; OR, odds ratio; CI, confidence interval; GWAS, genome-wide association studies; BMI, body mass index; NCHS, National Center for Health Statistics; CDC, Centers for Disease Control and Prevention; IRB, Institutional Review Board; SNPs, single-nucleotide polymorphisms; IVs, instrumental variables; LD, linkage disequilibrium; IVW, inverse variance weighted; DTAC, dietary antioxidant capacity; OSA, obstructive sleep apnea; Se, selenium; RCS, restricted cubic spline; NREM, non-rapid eye movement; VAD, vitamin A-deficient.
2. Perez-Pozuelo I, Zhai B, Palotti J, Mall R, Aupetit M, Garcia-Gomez JM, et al. The future of sleep health: A data-driven revolution in sleep science and medicine. NPJ Digit Med. (2020) 3:42. doi: 10.1038/s41746-020-0244-4
3. Zhou L, Kong J, Li X, Ren QJN, Reviews B. Sex differences in the effects of sleep disorders on cognitive dysfunction. Neurosci. Biobehav. Rev. (2023) 146:105067.
4. Pallesen S, Sivertsen B, Nordhus IH, Bjorvatn BJSA. 10-year trend of insomnia prevalence in the adult Norwegian population. Sleep Med. (2014) 15(2):173–9. doi: 10.1016/j.sleep.2013.10.009
5. Hauri PJ. Sleep disorders. In: SE Taylor, JE Singer, A Baum editors. In Handbook of Psychology and Health. (Volume I), Milton Park: Routledge (2021). p. 211–60.
6. Panel CC, Watson NF, Badr MS, Belenky G, Bliwise DL, Buxton OM. Recommended amount of sleep for a healthy adult: A joint consensus statement of the American academy of sleep medicine and sleep research society. Sleep (2015) 11(6):591–2.
7. Panel CC, Watson NF, Badr MS, Belenky G, Bliwise DL, Buxton OM. Joint consensus statement of the American academy of sleep medicine and sleep research society on the recommended amount of sleep for a healthy adult: Methodology and discussion. Sleep (2015) 11(8):931–52.
8. Everson CA, Laatsch CD, Hogg N. Antioxidant defense responses to sleep loss and sleep recovery. Am J Physiol Regul Integr Comp Physiol. (2005) 288(2):R374–83. doi: 10.1152/ajpregu.00565.2004
9. Villafuerte G, Miguel-Puga A, Rodríguez EM, Machado S, Manjarrez E, Arias-Carrión O. Sleep deprivation and oxidative stress in animal models: A systematic review. Oxid Med Cell Longev. (2015) 2015:234952. doi: 10.1155/2015/234952
11. Pullar JM, Carr AC, Vissers M. The roles of vitamin C in skin health. Nutrients (2017) 9(8):866.
12. Wu A, Ying Z, Gomez-Pinilla F. Vitamin E protects against oxidative damage and learning disability after mild traumatic brain injury in rats. Neurorehabil Neural Repair. (2010) 24(3):290–8. doi: 10.1177/1545968309348318
13. Alzoubi KH, Khabour OF, Rashid BA, Damaj IM, Salah H. The neuroprotective effect of vitamin E on chronic sleep deprivation-induced memory impairment: The role of oxidative stress. Behav Brain Res. (2012) 226(1):205–10.
14. Bjelakovic G, Nikolova D, Gluud LL, Simonetti RG, Gluud C. Mortality in randomized trials of antioxidant supplements for primary and secondary prevention: Systematic review and meta-analysis. JAMA. (2007) 297(8):842–57.
15. Abshirini M, Siassi F, Koohdani F, Qorbani M, Khosravi S, Hedayati M, et al. Dietary total antioxidant capacity is inversely related to menopausal symptoms: A cross-sectional study among Iranian postmenopausal women. Nutrition. (2018) 55:161–7. doi: 10.1016/j.nut.2018.04.014
16. Gellenbeck KJ. Carotenoids: More than just beta-carotene. Asia Pac J Clin Nutr. (1998) 7:277–81.
17. Burgess S, Butterworth A, Thompson S. Mendelian randomization analysis with multiple genetic variants using summarized data. Genet Epidemiol. (2013) 37(7):658–65.
18. Bowden J, Davey Smith G, Burgess S. Mendelian randomization with invalid instruments: Effect estimation and bias detection through Egger regression. Int J Epidemiol. (2015) 44(2):512–25.
19. Hartwig FP, Davey Smith G, Bowden J. Robust inference in summary data Mendelian randomization via the zero modal pleiotropy assumption. Int J Epidemiol. (2017) 46(6):1985–98. doi: 10.1093/ije/dyx102
20. Sato-Mito N, Sasaki S, Murakami K, Okubo H, Takahashi Y, Shibata S. The midpoint of sleep is associated with dietary intake and dietary behavior among young Japanese women. Sleep Med. (2011) 12(3):289–94.
22. Kanagasabai T, Ardern CIJS. Contribution of inflammation, oxidative stress, and antioxidants to the relationship between sleep duration and cardiometabolic health. Sleep (2015) 38(12):1905–12. doi: 10.5665/sleep.5238
23. Martens LG, Luo J, Meulmeester FL, Ashrafi N, van Eekelen EW, de Mutsert R, et al. Associations between lifestyle factors and vitamin E metabolites in the general population. Antioxidants (2020) 9(12):1280. doi: 10.3390/antiox9121280
24. Zhang H-Q, Li N, Zhang Z, Gao S, Yin H-Y, Guo D-M, et al. Serum zinc, copper, and zinc/copper in healthy residents of Jinan. Biol Trace Elem Res. (2009) 131:25–32. doi: 10.1007/s12011-009-8350-9
25. Ji X, Liu J. Associations between blood zinc concentrations and sleep quality in childhood: A cohort study. Nutrients. (2015) 7(7):5684–96. doi: 10.3390/nu7075247
26. Rondanelli M, Opizzi A, Monteferrario F, Antoniello N, Manni R, Klersy CJ. The effect of melatonin, magnesium, and zinc on primary insomnia in long-term care facility residents in Italy: A double-blind, placebo-controlled clinical trial. J Am Geriatr Soc. (2011) 59(1):82–90. doi: 10.1111/j.1532-5415.2010.03232.x
27. Massadeh AM, Alzoubi KH, Milhem AM, Rababa’h AM, Khabour OF. Evaluating the effect of selenium on spatial memory impairment induced by sleep deprivation. Physiol Behav. (2022) 244:113669. doi: 10.1016/j.physbeh.2021.113669
28. Beydoun MA, Gamaldo AA, Canas JA, Beydoun HA, Shah MT, McNeely JM, et al. Serum nutritional biomarkers and their associations with sleep among US adults in recent national surveys. PLoS One. (2014) 9(8):e103490. doi: 10.1371/journal.pone.0103490
30. Steriade M. Slow-wave sleep: Serotonin, neuronal plasticity, and seizures. Arch Ital Biol. (2004) 142(4):359–67.
31. Maret S, Franken P, Dauvilliers Y, Ghyselinck NB, Chambon P, Tafti MJ. Retinoic acid signaling affects cortical synchrony during sleep. Science. (2005) 310(5745):111–3.
32. Samad TA, Krezel W, Chambon P, Borrelli E. Regulation of dopaminergic pathways by retinoids: Activation of the D2 receptor promoter by members of the retinoic acid receptor–retinoid X receptor family. Proc Natl Acad Sci USA. (1997) 94(26):14349–54. doi: 10.1073/pnas.94.26.14349
33. Krezel W, Ghyselinck N, Samad TA, Dupé VR, Kastner P, Borrelli E, et al. Impaired locomotion and dopamine signaling in retinoid receptor mutant mice. Science. (1998) 279(5352):863–7. doi: 10.1126/science.279.5352.863
34. Tafti M, Ghyselinck N. Functional implication of the vitamin A signaling pathway in the brain. Arch Neurol. (2007) 64(12):1706–11.
36. Otocka-Kmiecik A, Król A. The role of vitamin C in two distinct physiological states: Physical activity and sleep. Nutrients. (2020) 12(12):3908. doi: 10.3390/nu12123908
37. Matsuki K, Grumet FC, Lin X, Gelb M, Guilleminault C, Dement W, et al. (rather than DR) gene marks susceptibility to narcolepsy. Lancet. (1992) 339(8800):1052. doi: 10.1016/0140-6736(92)90571-j
38. Nishino S, Mignot E. Pharmacological aspects of human and canine narcolepsy. Prog Neurobiol. (1997) 52(1):27–78.
39. Mahlios J, De la Herrán-Arita AK, Mignot E. The autoimmune basis of narcolepsy. Curr Opin Neurobiol. (2013) 23(5):767–73.
40. La Torre ME, Villano I, Monda M, Messina A, Cibelli G, Valenzano A. Role of vitamin E and the orexin system in neuroprotection. Brain Sci. (2021) 11(8):1098.
41. Blair-West J, Denton D, Gibson A, McKinley MJ. Opening the blood-brain barrier to zinc. Toxicol Appl Pharmacol. (1990) 507(1):6–10.
42. McKee KK, Tan CP, Palyha OC, Liu J, Feighner SD, Hreniuk DL. Cloning and characterization of two human G protein-coupled receptor genes (GPR38 and GPR39) related to the growth hormone secretagogue and neurotensin receptors. Genomics. (1997) 46(3):426–34. doi: 10.1006/geno.1997.5069
43. Jackson VR, Nothacker H-P, Civelli OJN. GPR39 receptor expression in the mouse brain. Neuroreport. (2006) 17(8):813–6.
44. Młyniec K, Budziszewska B, Holst B, Ostachowicz B, Nowak G. GPR39 (zinc receptor) knockout mice exhibit depression-like behavior and CREB/BDNF down-regulation in the hippocampus. Int J Neuropsychopharmacol. (2015) 18(3):yu002. doi: 10.1093/ijnp/pyu002
45. Młyniec K, Doboszewska U, Szewczyk B, Sowa-Kućma M, Misztak P, Piekoszewski W. The involvement of the GPR39-Zn (2+)-sensing receptor in the pathophysiology of depression. Studies in rodent models and suicide victims. Neuropharmacology. (2014) 79:290–7. doi: 10.1016/j.neuropharm.2013.12.001
46. Khan MZA. possible significant role of zinc and GPR39 zinc sensing receptor in Alzheimer disease and epilepsy. Biomed Pharmacother. (2018) 79:263–72. doi: 10.1016/j.biopha.2016.02.026
47. Burke SL, Maramaldi P, Cadet T, Kukull W. Associations between depression, sleep disturbance, and apolipoprotein E in the development of Alzheimer’s disease: Dementia. Int Psychogeritar. (2018) 28(9):1409–24.
48. Tinggi U. Selenium: its role as antioxidant in human health. Environ Health Prev Med. (2008) 13:102–8.
Keywords: National Health and Nutrition Examination Survey MR, Mendelian randomization genome-wide association studies BMI, body mass index NCHS, National Center for Health Statistics CDC, Centers for Disease Control and Prevention IRB, Institutional Review Board SNPs, single-nucleotide polymorphisms IVs, instrumental variables LD
Citation: Jiang J, Li D, Huang T, Huang S, Tan H and Xia Z (2024) Antioxidants and the risk of sleep disorders: results from NHANES and two-sample Mendelian randomization study. Front. Nutr. 11:1453064. doi: 10.3389/fnut.2024.1453064
Received: 22 June 2024; Accepted: 16 September 2024;
Published: 02 October 2024.
Edited by:
Daniele Corbo, University of Brescia, ItalyReviewed by:
Shinsuke Hidese, Teikyo University, JapanCopyright © 2024 Jiang, Li, Huang, Huang, Tan and Xia. This is an open-access article distributed under the terms of the Creative Commons Attribution License (CC BY). The use, distribution or reproduction in other forums is permitted, provided the original author(s) and the copyright owner(s) are credited and that the original publication in this journal is cited, in accordance with accepted academic practice. No use, distribution or reproduction is permitted which does not comply with these terms.
*Correspondence: Zhongfang Xia, emhmeGlhMjAwNUAxNjMuY29t
Disclaimer: All claims expressed in this article are solely those of the authors and do not necessarily represent those of their affiliated organizations, or those of the publisher, the editors and the reviewers. Any product that may be evaluated in this article or claim that may be made by its manufacturer is not guaranteed or endorsed by the publisher.
Research integrity at Frontiers
Learn more about the work of our research integrity team to safeguard the quality of each article we publish.