- 1Division of Nutritional Sciences, University of Illinois, Urbana, IL, United States
- 2Department of Food Science and Human Nutrition, University of Illinois, Urbana, IL, United States
- 3IFF Health and Biosciences, Kantvik, Finland
- 4IFF R&D—Enabling Technologies, Brabrand, Denmark
- 5IFF Health and Biosciences, Saint Louis, MO, United States
- 6Department of Animal Sciences, University of Illinois, Urbana, IL, United States
Introduction: The oligosaccharide 2′-fucosyllactose (2′-FL) is a predominant component of human milk, serving as a prebiotic for gut microbiota and influencing immune development in infants. Bifidobacterium longum subspecies infantis (B. infantis) is a commensal bacterium found in breastfed infants. Both 2′-FL and a specific strain of B. infantis, Bi-26™, are commercially available. This study investigates the potential synbiotic relationship between 2′-FL and Bi-26™ on immune development.
Methods: Two-day-old piglets (n = 53) were randomized in a 2 × 2 design, receiving either a commercial milk replacer ad libitum without (CON) or with 1.0 g/L 2′-FL (FL). Piglets in each diet were further randomized to receive either glycerol stock alone or Bi-26™ (109 CFU) (BI and FLBI) orally once daily. On postnatal day (PND) 34/35, animals were euthanized, and blood was collected for serum cytokine analysis. Additionally, peripheral blood mononuclear cells (PBMCs) were isolated for ex vivo stimulation and flow cytometry analysis. Serum and ex vivo cytokines were analyzed using a multivariate model. All other outcomes were analyzed using a two-way ANOVA, considering prebiotic and probiotic fixed effects. The significance level was set at a p value <0.05, with trends reported for 0.05 < p < 0.1.
Results: Immune cell populations in PBMCs were unaffected by the experimental treatment. However, serum interleukin (IL)-1RA, IL-1β, IL-12, and IL-18 were all higher (p < 0.05) in the FL group than in the CON group. In isolated PBMCs, lipopolysaccharide (LPS) stimulation resulted in higher IL-1RA and a trend for higher IFN-γ secretion in the FL group vs. the CON group.
Conclusion: 2′-FL stimulates a balanced cytokine profile in healthy piglets without changing immune cell populations. When immune cells are stimulated ex vivo with LPS, 2′-FL primes T-cells for a proinflammatory response, which is moderated by co-administration of Bi-26™.
1 Introduction
The newborn infants’ immune system is functionally naïve, leaving them vulnerable to pathogens. Morbidity and mortality are higher in non-breastfed infants (1), which is attributed to the bioactive components in human milk that stimulate immune maturation and development of the gut microbiome (2). Among these bioactive components are the multifunctional human milk oligosaccharides (HMOs), which serve as prebiotics and inhibitors of pathogen attachment to the epithelial lining (3). Infant formulas are virtually devoid of these glycans since they are in low concentration in bovine milk, which is the starting material for most infant formulas (3).
In human milk, 2′-fucosyllactose (2′-FL) is one of the most abundant HMOs in mothers who express the 2-fucosyltransferase gene (4). A small amount of 2′-FL is absorbed into the systemic circulation in human infants (5) and rats (6). There is evidence that 2′-FL modulates cytokine production and receptor signaling, but data are not entirely consistent. Co-culturing cord blood mononuclear cells with neutral HMOs had little effect on intracellular production of IFN-γ, IL-4, and IL-13, but acidic HMOs increased the production of these cytokines (7). Preclinical and clinical studies have demonstrated that 2′-FL alone directly impacts immune development and inflammation. Infants fed formula supplemented with 0.2 or 2.0 g/L 2′-FL had serum IL-1RA, IL-1β, IL-1α, tumor necrosis factor-α, and IL-6 concentrations that were not statistically different from those of breastfed infants (8). In human infant and mouse intestinal explants, 2′-FL decreased the severity of necrotizing enterocolitis by binding to the TLR4-myeloid differentiation factor 2-[MD2] complex, which prevented lipopolysaccharide (LPS) binding (9). Furthermore, 2′-FL ameliorated inflammatory response to rotavirus (RV) infection in the small intestine of rat pups and reduced IL-β, IL-6, and IFN-γ production at the peak of infection (10). In addition, in healthy suckling rat pups, an oral gavage of 2′-FL increased plasma IgG and IgA levels as well as T-helper cell populations in the mesenteric lymph nodes (MLNs) (11). Thus, 2′-FL has shown a range of immunomodulatory characteristics that may benefit infants.
Bifidobacterium longum subspecies infantis (B. infantis) is a common resident of the gut microbiota of breastfed, but not formula-fed infants. A body of literature also supports the ability of B. infantis to influence cytokine production and health outcomes. In human intestinal NEC models, B. infantis reduced markers of inflammation, such as IL-6 and IL-8 production, and decreased the upregulation of NFκB-related genes in vitro (12). In a clinical trial evaluating the enteric immunomodulatory effects of B. infantis EVC001, IL-8, IL-22, IL-1β, and IFN-γ were lower in infants receiving probiotic supplementation than in control infants only being breastfed (13). In addition, in a placebo-controlled, double-blind, randomized study in human infants, supplementation with B. infantis increased the IL-10/IL-12 ratio toward an anti-inflammatory effect (14). In a piglet model of Staphylococcus aureus infection, post-infection, B. infantis supplementation increased serum IL-10 level concentrations and decreased memory T-cell populations (15). Thus, B. infantis holds promise as an immunomodulatory probiotic supplement for human infants.
Recent evidence also supports a synbiotic potential for combined administration of 2′-FL and B infantis. In addition to direct effects on the immune system, 2′-FL stimulates the growth of Bifidobacterium, and B. infantis can use 2′-FL for growth and metabolite production (16, 17). Bi-26™ is a commercially available strain of B. infantis in the Danisco Global Culture Collection. Its genome reveals that Bi-26™ contains the enzymes necessary to digest 2′-FL. Utilization of 2′-FL by Bi-26™ produces a more diverse group of metabolites than those generated from lactose utilization (18). However, the potential symbiotic effects of Bi-26 and 2′-FL on immune function and development in vivo are not well studied.
Therefore, we aimed to better understand the individual and synbiotic effects of dietary 2′-FL and Bi-26™ on piglet systemic immune development. Because of their individual effects, we hypothesized that both 2′-FL and Bi-26™ would promote healthy development of systemic immunity. We further hypothesized that piglets exposed to the combination of 2′-FL and Bi-26™ would have lower levels of serum pro-inflammatory cytokines than CON or 2′-FL, but immune cells isolated from these piglets would demonstrate a more robust response to ex vivo LPS stimulation than cells from piglets fed either 2′-FL or Bi-26™ alone.
2 Materials and methods
2.1 Experimental design
The Institutional Animal Care and Use Committee at the University of Illinois approved all animal care and experimental procedures. The experimental design, dietary treatments, and probiotic administration were previously described by Daniels et al. (19). Briefly, naturally farrowed piglets (n = 63) from a commercial swine herd received colostrum from the sow for up to 2-day (PND2) after birth before being transported to a specialized neonatal pig rearing system at the Piglet Nutrition and Cognition Laboratory (PNCL) on the University of Illinois campus. Piglets were randomized into the four treatment groups in a 2 × 2 design. Two experimental milk replacer diets (CON and 2′-FL) were manufactured in powder form by TestDiet (Purina Mills, St. Louis, MO). The composition was identical to ProNurse (Land O’Lakes, North Arden Hills, MN) except that the CON diet had added lactose equal to the 2′-FL content in the test diet (1 g/L in the reconstituted formula). The 2′-FL (CARE4U®) was provided by International Flavors & Fragrances (New York, NY, United States). CARE4U® is a high purity (≥94%) infant-grade 2′-FL containing ≤300 EU endotoxins/g product. Liquid milk replacer was reconstituted daily at 20% solids and automatically delivered to piglets beginning at 1,000 h each day and ending at 0600 h the following day (i.e., ad libitum access to treatment diets over a 20-h feeding period each day). Milk disappearance was calculated per individual pig based on the initial and final reservoir weights collected before and after the daily feeding cycle. Piglets in the CON and 2′-FL diet groups were further randomized to probiotic or no probiotic, resulting in four experimental treatment groups (CON, FL, BI, and FLBI). Aliquots of the probiotic treatment (Bi-26™; Danisco Global Culture collection DGCC11473 Niebüll, Germany) at the dose of 109 CFU/day were solubilized before the start of the study in bacteria glycerol stock (12.1% glycerol) and frozen until administration. The treatment was thawed and orally dosed daily. Piglets not receiving probiotic treatment received an equal dose of glycerol stock.
2.2 Sample collection
On PND 34 or 35, piglets were sedated with an intramuscular injection of Telazol® (Tiletamine HCl and Zolazepam HCl, 3.5 mg/kg BW each, Pfizer Animal Health, Fort Dodge, IA). Blood samples were collected by intracardiac puncture into heparin-laced vials (BD Biosciences, Franklin Lakes, NJ, United States) to isolate peripheral blood mononuclear cells (PBMCs). In addition, blood samples were collected and prepared as serum and or plasma by centrifugation. Piglets were then euthanized by an intracardiac injection of sodium pentobarbital (72 mg/kg BW; Fatal Plus; Vortech Pharmaceuticals, Dearborn, MI).
2.3 Immune cell isolation
Peripheral blood mononuclear cells were isolated on PND 34 or 35 following necropsy as previously described (20). Blood was initially diluted with DPBS (Dulbecco’s PBS, no Ca++, no Mg++, Life Technologies, Thermo Fisher, Carlsbad, CA) at a 1:1 ratio, then layered onto Ficoll-Paque Plus (GE Healthcare, Piscataway, NJ), and spun at 400 × g for 40 min at 20°C. PBMCs were collected from the gradient interface and washed three times in wash buffer (Hanks Buffered Salt Solution, no Ca++, no Mg++, Life Technologies) supplemented with 2% bovine serum albumin (BSA; Sigma-Aldrich, St. Louis, MO), 0.01 M EDTA (Sigma-Aldrich), 50 μg/mL Gentamycin (Life Technologies), 1,000 U/mL penicillin (Sigma-Aldrich), and 100 μg/mL streptomycin (10 mg/mL stock, Sigma-Aldrich). The remaining red blood cells in the pellet were lysed with lysis buffer (0.15 M of NH4Cl, 10 mM KHCO3, and 0.1 mM Na2EDTA). PBMCs were suspended in RPMI-1640 supplemented with 10% fetal bovine serum (FBS), 2 mM glutamine, 50 μg/mL gentamycin, 1 mM sodium pyruvate (Life Technologies), 20 mM HEPES (Life Technologies), and 20 mM 1,000 U/mL penicillin/100 μg/mL streptomycin prior to quantification. The number of viable cells was assessed with Countess® Automated Cell Counter (Life Technologies). Cells were then used for phenotypic cell identification by flow cytometry and ex vivo stimulation as described below.
2.4 Phenotypic identification of immune cells in PBMC
Phenotypes of PBMC subpopulations were analyzed by flow cytometry (BD™ LSRII, Biosciences) in the Roy J Carver Biotechnology Center Flow Cytometry facility at the University of Illinois. A panel of fluorescein (FITC) or Phycoerythrin (PE)-labeled mAbs was following a previously established staining procedure (20, 21). Briefly, T-lymphocytes were identified by mouse anti-pig CD4 (FITC, Clone 74-12-4) and mouse anti-pig CD8 (PE, Clone 76-2-11) antibodies (Southern Biotech, Birmingham, AL). B-lymphocytes were identified by mouse anti-pig SLA class II (FITC, Clone 2E9/13) and mouse anti-pig CD21 (PE, Clone BB6-11C6.9). A total of 10 μL of each antibody was added to 1 × 106 cells from each sample. Staining procedures were carried out on ice, and the samples were protected from light when possible. In brief, all cells were initially incubated with 5 μg/mL of unlabeled mouse anti-pig CD16 (20 μL; AbD Serotec, Raleigh, NC) to prevent non-specific binding. Each well was blocked with 5% mouse serum (Southern Biotech) for 5 min each. After centrifugation, CD3 was added to the wells, incubated for 20 min (50 μL: CD3:PE-Cy5; Southern Biotech), and centrifuged again. CD4:FITC and CD8:PE were added or SLAII:FITC and CD21:PE (10 μL each) and incubated for an additional 15 min until centrifuged. Cells were washed with PBS/1% BSA/0.1% sodium azide and then fixed with 2% paraformaldehyde. Cells were assessed using an LSRII flow cytometer (BD™, Biosciences). The percentage of T-cell subpopulations and B-cell populations was determined using FCS 4 Express software (DeNovo Software; Glendale, CA). CD3+ events were considered T-cells. CD3+CD4+CD8− events were considered T-helper cells, and CD3 + CD4-CD8+ and CD3 + CD4 + CD8+ were considered cytotoxic T and memory T-cells, respectively. B-cells were identified at CD21+ and SLA class II+ dual positive cells using SLAII:FITC- and CD21PE-labeled antibodies.
2.5 Serum cytokine concentrations
The concentrations of 13 cytokines, namely, granulocyte-macrophage colony-stimulating factor (GM-CSF), interferon-gamma (IFNγ), tumor necrosis factor-alpha (TNFα), interleukin (IL)-1α, IL-1RA, IL-1β, IL-2, IL-4, IL-6, IL-8, IL-10, IL-12, and IL-18 were measured using a Millipore (Milliplex) porcine cytokine array at the University of Illinois Flow Cytometry Center. When the value for a sample fell below the limit of detection (Supplementary Table 1), values were set to 0.
2.6 Ex vivo cell stimulation and cytokine production
Isolated PBMC were cultured to assess ex vivo cytokine secretion in response to LPS and phytohemagglutinin (PHA) stimulation as previously described (20, 21) Briefly, cells (2 × 105 cells/well) were plated in 96-well plates in a final volume of 200 μL in complete RPMI and were maintained at 37°C under 5% CO2. Triplicate wells per cell type and experimental treatment were immediately stimulated with 2 μg/mL LPS and 2.5 μg/mL PHA, a lymphocyte activation and proliferation modulator. Cell culture supernatants were collected 72 h after culture initiation and frozen at −80°C until analyzed. Supernatants from the ex vivo assay were analyzed for 13 cytokines using a Millipore (Milliplex) pig cytokine array described for serum. In addition, IL-17 was analyzed using an ELISA kit (Raybiotech, Norcross, GA) according to the manufacturer’s instructions, with the exception that PBS was used as the basis for the assay buffers.
2.7 Statistical analyses
All data, except for the cytokine data, were analyzed using SAS version 9.4 (SAS Institute Inc., Cary, NC) using the PROC MIXED procedure. Immunoglobulin and immune cell data were analyzed using a two-way ANOVA. The statistical model was nested for random effects with replicate nested within sow. Both models included fixed effects of diet (prebiotic), probiotics, and the interaction between diet and probiotics with Dunnett’s post hoc test to compare the statistical differences between the control and experimental treatment groups. If samples were ± 3 standard deviations from the mean, they were excluded as outliers. Significance was defined at a p value <0.05 and trends are reported as a p value <0.10. Data are presented as means ± standard error of the mean (SEM) unless otherwise stated.
Serum and ex vivo cytokine concentrations were analyzed using a PROC LCA model using SAS version 9.4 adapted to the respective design. The design for serum samples was a basic design with a split-plot structure. Whole plots were characterized by random effects of sow ID nested within the cohort. Split plots were characterized by piglet ID with the fixed effect of diet. The diet was composed as a 2-by-2 factorial from factors Bi-26™ and 2′-FL. The response is multivariate with manifestations of each cytokine (IFN-γ, IL-1α, IL-1β, IL-1RA, IL-2, IL-4, IL-6, IL-8, IL-10, IL-12, and IL-18). The design for the ex vivo analyses was a one-split of the basic design, by a treatment (unstimulated, LPS, PHA) for PBMC. Whole plots were characterized by random effects of sow ID nested within the cohort. Split plots were characterized by piglet ID with a fixed effect of diet. The diet was composed as a 2-by-2 factorial from factors Bi-26™ and 2′-FL. Split–split plots were characterized by random residual with nested fixed effect of treatment interaction between treatment and diet.
3 Results
3.1 Serum immunoglobulin and cytokine concentrations
No differences were found in serum IgG or IgM. Serum IL-1RA, IL-1β, IL-2, and IL-18 were all significantly higher in FL but not BI alone or FLBI than in CON (Table 1). Serum IL-12 was greater in FL than CON and FLBI but not BI alone. There were trends (p < 0.1) for IL-1α, IL-4, IL-6, and IL-10 to also be affected by diet or the interaction of diet and probiotics. Serum IFN-γ and IL-8 were unaffected by experimental treatment (Table 2). Serum IL-17A was below the level of detection.
3.2 PBMC immune cell populations
No treatment effect was observed in T-cell subpopulations or B-cell populations in PBMC (Table 3).
3.3 PBMC ex vivo cytokine secretion
To investigate the impact of in vivo exposure to 2′-FL or Bi-26™ on cytokine secretion in response to LPS or PHA stimulation, the secretion of cytokines into the media was compared between unstimulated to stimulated PBMCs (Table 4). PBMC isolated from piglets fed FLBI stimulated with LPS ex vivo secreted higher concentrations of IL-1RA than CON (p ≤ 0.05) (Table 4). There was a trend (p < 0.1) for the effect of 2′-FL on IFN-γ secretion. There was also a trend (p < 0.1) for the effect of 2′-FL on IL-6 secretion. No other cytokines were affected by experimental treatment with stimulation from LPS or PHA compared to CON.
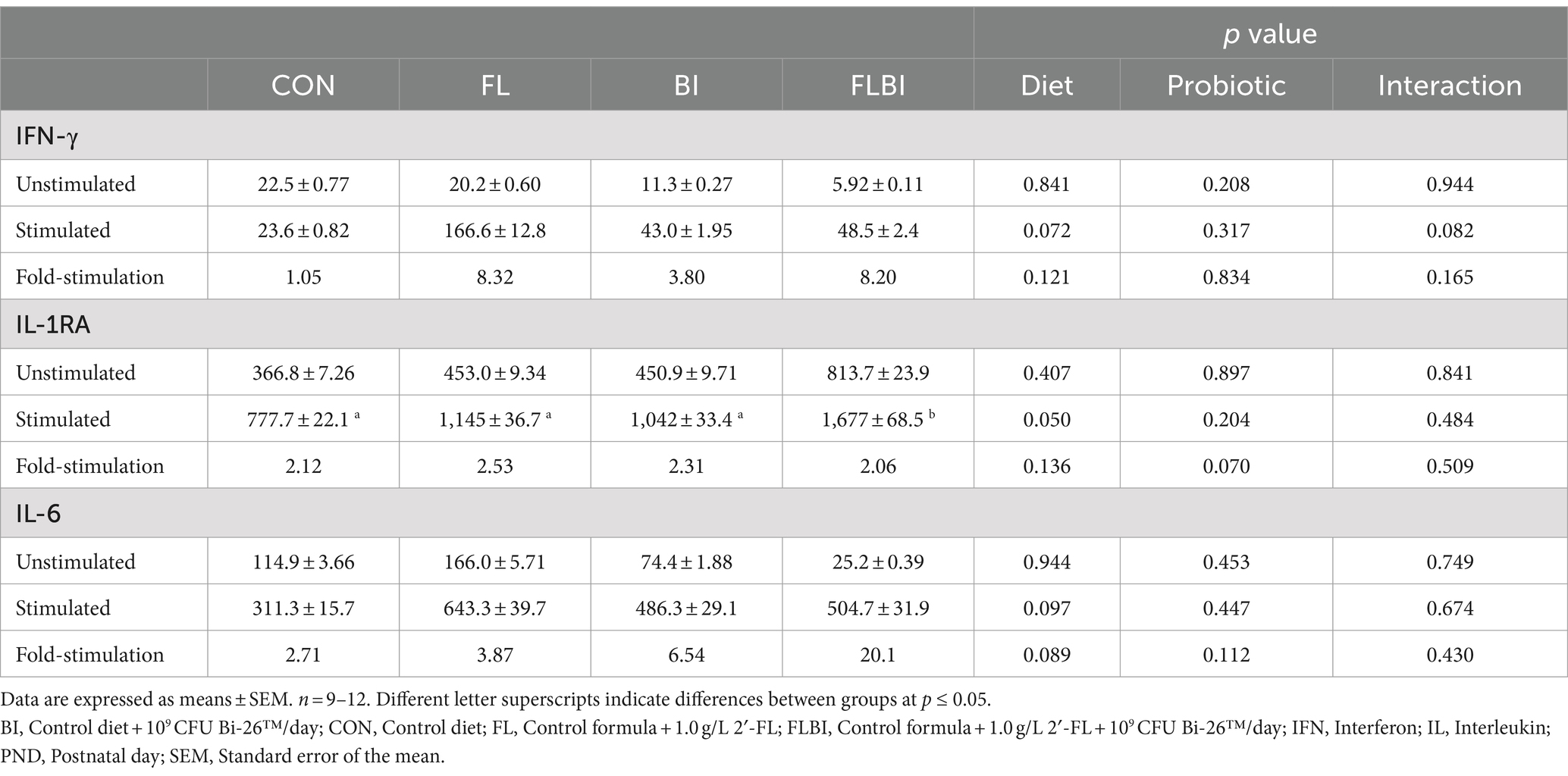
Table 4. Cytokine concentrations (pg/mL) in conditioned media from ex vivo stimulated peripheral blood mononuclear cells.
4 Discussion
Oligosaccharides in milk play an important role in modulating the development of the neonatal immune system directly and indirectly through altering the gut microbiota (22). A recent study by Rosa et al. demonstrated the direct effects of HMO in the absence of microbiota (23). Germ-free 1-day-old mice were gavaged daily with pooled HMOs (15 mg) for 7 or 14 days. Samples were collected on days 28, 35, or 50. HMO-administration induced differences in immune cell populations, antibody-secreting cells, and intestinal tissue gene expression in germ-free animals. Cytokine secretion was not measured in that study. Given that pooled HMOs were administered, it is not possible to attribute outcomes to specific individuals or categories of HMOs.
Herein, the individual and combined effects of 2′-FL and B. infantis were studied for the following reasons. Supplementing infant formula with all HMOs at the current time is not feasible. 2′-FL is a predominant HMO in the milk of secretor mothers (4), it has been supplemented to infant formulae for several years with no adverse effects (24) and has documented effects on infant immunity (8). B. infantis was studied as formula-fed infants are devoid of B. infantis, a predominant bifidobacterial strain in breastfed infants, it can use 2′-FL and has immunomodulatory effects and has documented effects on infant immunity (16, 18). Given the demonstrated benefits of supplemental 2′-FL (8, 25) and B. infantis (13, 26), documented in previous clinical trials, and their potential for symbiotic activity, we investigated the individual and combined effects of dietary 2′-FL at 1 g/L and Bi-26™ at 109 CFU on immune development and function in piglets. Our findings show the effects of 2′-FL systemic immunity and potential interactive effects when supplemented with Bi-26™.
We found no effect of dietary 2′-FL and/or Bi-26™ on circulating immune cell populations; all levels were within the range previously reported in our laboratory for healthy piglets (27, 28), suggesting that there was no impact on T-cell or B-cell percentages. In a clinical trial of 2′-FL supplementation, circulating CD4+ and CD8+ T-lymphocyte populations were similar between breastfed infants and infants fed formula supplemented with 2′-FL (8). Since we saw no differences in T-cell subsets or B-cells in PBMC, 2′-FL could be affecting innate immunity. Although dendritic cells make up a small percentage of circulating immune cells, they present antigens to T-cells, leading to the upregulation of cytokines to recruit immune cells to clear the pathogen (29). IL-10 and IL-6 are upregulated in dendritic cells treated with HMOs isolated from human milk (30). Increased dendritic cell activity could recruit T-helper cells, which could explain why the serum cytokines were increased in our study; however, more research is required to establish the precise mechanism at hand.
A key finding of the present study was that 2′-FL increased the concentrations of IL-1RA, IL-1β, IL-2, IL-12, and IL-18 in serum compared to control piglets. The group receiving both 2′-FL and Bi-26™ did not differ from the control group, suggesting that the co-administration of Bi-26™ may moderate the effect of 2′-FL impact on serum cytokines. Studies in human infants have shown that breastfeeding is associated with lower circulating concentrations of proinflammatory cytokines (8, 31). In a clinical trial of 2′-FL supplementation, infants fed formula alone had higher IL-1RA, IL-1β, IL-1α, tumor necrosis factor-α, and IL-6 than infants fed formula supplemented with 0.2 g/L and 2.0 g/L 2′-FL. Infants supplemented with 2′-FL had inflammatory cytokine concentrations similar to that of breastfed infants (8). Similar observations have been made in rat studies, with 2′-FL lowering IL-1β, IL-4, IL-6, IL-12, IFN-γ, and TNF-α in the small intestine (11) and serum IL-18 in male rats compared to control (32). The study of Goehring et al. (8) demonstrated similar effects of 0.2 g/L and 2.0 g/L on serum cytokines, supporting a lack of dose dependency on cytokine concentrations (8); so, it is unlikely we would have seen lower cytokine concentrations with a higher or lower dose. Intact HMOs can be absorbed into the blood, and it is likely that immune cells are directly influenced in circulation.
In addition to measuring circulating cytokines, we assessed whether in vivo exposure to 2′-FL and/or Bi-26™ would impact cytokine secretion by immune cells isolated from blood (PBMC) when exposed to LPS or PHA ex vivo. Cytokines are predominantly produced by T-helper cells and macrophages (29). Although, in the present study, T-cell and B-cell populations in PBMC were unaffected by 2′-FL and Bi-26™, these supplements impacted immune response to LPS ex vivo. We found that PBMC, isolated from piglets fed 2′-FL alone, demonstrated increased secretion of IL-1RA, after LPS stimulation. In addition, in vivo exposure to 2′-FL tended to increase IFN-γ and IL-6 secretion. IFN-γ induces a wide array of immune responses. It can promote macrophage activation, facilitate antiviral and antibacterial activities, increase antigen presentation, and influence activation of the innate immune system (33) while IL-1RA is an inhibitor of pro-inflammatory IL-1 (34). LPS is a TLR-4 agonist, which leads to the production of IL-1, so the increase in IL-1RA and IL-6 could be a response to ameliorate inflammation caused by LPS challenge since 2′-FL and B infants have been shown to have anti-inflammatory effects. These results may be due to receptors that recognize HMOs, such as galectins and TLRs. Since HMOs have been found in the blood (6, 35), binding of HMOs to galectins in the blood could result in the regulation of TLR expression. In addition, 2′-FL has been shown to bind directly to TLR-4 in silico and modulate response to LPS by reducing TLR-4 expression in mice (9). An in vitro model of necrotizing enterocolitis in immature human enterocytes, B. infantis (ATCC 15697) conditioned medium reduced expression of inflammatory markers, TLR-2 and TLR-4, as well as concentrations of IL-6 and IL-8 (36). In a piglet model of Staphylococcus aureus infection, piglets supplemented with B. infantis (ATCC 15697) at 3 × 109 CFU/day had increased serum IL-10, potentially as an attempt by the immune system to reduce the inflammatory response (15). Although no increase in IL-10 was observed following LPS stimulation, an increase in IL-1RA secretion by PBMC from piglets in the combined group was observed, suggesting that 2′-FL and Bi-26™ may still exhibit an anti-inflammatory effect. In addition, PBMC stimulated with PHA showed no effect of experimental treatment compared to the control formula. PHA is a lectin that requires monocytes, which are antigen-presenting cells, to induce T-cell proliferation (37). Our data suggests that neither 2′-FL nor Bi-26™ enhances T-cell proliferation in response to PHA compared to the control formula. Our study showed that dietary exposure to 2′-FL elicited a more robust cytokine response to the LPS challenge than from cells isolated from piglets fed the control formula. This could translate to improved immune response in infants receiving these HMOs.
Piglets were not immunologically challenged in vivo, therefore this study may not be translational to infants enduring infection. Although ex vivo stimulation shows a robust response to LPS stimulation, ex vivo conditions do not exactly mirror in vivo inflammation (38); therefore, an in vivo immune challenge could better evaluate the potential protective effects of 2′-FL and Bi-26™. A limitation of the study is that all immune analyses were from samples taken at a single time point at the end of the study, which limits our ability to evaluate the influence 2′-FL and Bi-26™ have on early immune development.
To summarize, human milk is the ideal feeding mode for infants; however, the World Health Organization (WHO) reports that globally, 66% of infants are not exclusively breastfeeding by 6 months of age, in accordance with the recommendations from 2015–2020 (39). As a result, many families must rely on infant formula during this critical period of development. While 2′-FL and B. infantis are commercially available, this is the first study to our knowledge to assess their combined effect on immune development in the piglet model. We have shown that the addition of 2′-FL and/or Bi-26™ could provide formula-fed infants with certain protective benefits associated with bioactive components that are typically lacking in infant formulae. Future investigations are needed to evaluate the effects of 2′-FL and Bi-26™ in response to immune stimuli during development and to explore the underlying mechanisms of these immune responses. This study builds upon previous observations demonstrating that supplementation of formula with 2′-FL and/or Bi-26™ is well tolerated and supports normal growth of piglets (19). Additionally, supplementation with 2′-FL and/or Bi-26 has been associated with changes in exploratory behaviors in novel object recognition tests, as well as changes to brain macro/micro-structures (40). Taken together, these findings support the multifunctional activities of 2′-FL and/or Bi-26™ in neonates.
Data availability statement
The raw data supporting the conclusions of this article will be made available by the authors, without undue reservation.
Ethics statement
The animal study was approved by University of Illinois at Urbana-Champaign Institutional Animal Care and Use Committtee. The study was conducted in accordance with the local legislation and institutional requirements.
Author contributions
VD: Formal analysis, Investigation, Methodology, Writing – original draft, Writing – review & editing. MM: Formal analysis, Investigation, Writing – original draft, Writing – review & editing, Methodology, Supervision. JH: Writing – review & editing, Conceptualization, Resources. AO: Conceptualization, Writing – review & editing, Formal analysis. HJ: Conceptualization, Writing – review & editing. RM: Conceptualization, Writing – review & editing. NC: Writing – review & editing, Formal analysis. ML: Formal analysis, Writing – review & editing. RD: Writing – review & editing, Conceptualization, Funding acquisition, Project administration, Supervision, Writing – original draft. SD: Conceptualization, Funding acquisition, Project administration, Supervision, Writing – original draft, Writing – review & editing, Formal analysis, Investigation.
Funding
The author(s) declare that financial support was received for the research, authorship, and/or publication of this article. This research was funded by DuPont Nutrition Biosciences ApS (fully owned by IFF), Copenhagen, Denmark. After initial contributions to the study design, the funders had no role in the collection, analysis, or interpretation of data.
Conflict of interest
SMD and RND received grant funding from IFF, the producer of B. infantis Bi-26TM and 2´-FL. JH, HMJ, NC, MJL, and ACO are employees of IFF. RM was employed by IFF at the time the study was conducted.
The remaining authors declare that the research was conducted in the absence of any commercial or financial relationships that could be construed as a potential conflict of interest.
Publisher’s note
All claims expressed in this article are solely those of the authors and do not necessarily represent those of their affiliated organizations, or those of the publisher, the editors and the reviewers. Any product that may be evaluated in this article, or claim that may be made by its manufacturer, is not guaranteed or endorsed by the publisher.
Supplementary material
The Supplementary material for this article can be found online at: https://www.frontiersin.org/articles/10.3389/fnut.2024.1444594/full#supplementary-material
References
1. Sankar, MJ, Sinha, B, Chowdhury, R, Bhandari, N, Taneja, S, Martines, J, et al. Optimal breastfeeding practices and infant and child mortality: a systematic review and meta-analysis. Acta Paediatr. (2015) 104:3–13. doi: 10.1111/apa.13147
2. Le Doare, K, Holder, B, Bassett, A, and Pannaraj, PS. Mother’s milk: a purposeful contribution to the development of the infant microbiota and immunity. Front Immunol. (2018) 9:361. doi: 10.3389/fimmu.2018.00361
3. Martin, CR, Ling, PR, and Blackburn, GL. Review of infant feeding: key features of breast milk and infant formula. Nutrients. (2016) 8:279. doi: 10.3390/nu8050279
4. Sprenger, N, Tytgat, HLP, Binia, A, Austin, S, and Singhal, A. Biology of human milk oligosaccharides: from basic science to clinical evidence. J Hum Nutr Diet. (2022) 35:280–99. doi: 10.1111/jhn.12990
5. Dotz, V, Rudloff, S, Blank, D, Lochnit, G, Geyer, R, and Kunz, C. 13C-labeled oligosaccharides in breastfed infants' urine: individual-, structure- and time-dependent differences in the excretion. Glycobiology. (2014) 24:185–94. doi: 10.1093/glycob/cwt099
6. Vazquez, E, Santos-Fandila, A, Buck, R, Rueda, R, and Ramirez, M. Major human milk oligosaccharides are absorbed into the systemic circulation after oral administration in rats. Br J Nutr. (2017) 117:237–47. doi: 10.1017/S0007114516004554
7. Eiwegger, T, Stahl, B, Schmitt, J, Boehm, G, Gerstmayr, M, Pichler, J, et al. Human milk-derived oligosaccharides and plant-derived oligosaccharides stimulate cytokine production of cord blood T-cells in vitro. Pediatr Res. (2004) 56:536–40. doi: 10.1203/01.PDR.0000139411.35619.B4
8. Goehring, KC, Marriage, BJ, Oliver, JS, Wilder, JA, Barrett, EG, and Buck, RH. Similar to those who are breastfed, infants fed a formula containing 2′-fucosyllactose have lower inflammatory cytokines in a randomized controlled trial. J Nutr. (2016) 146:2559–66. doi: 10.3945/jn.116.236919
9. Sodhi, CP, Wipf, P, Yamaguchi, Y, Fulton, WB, Kovler, M, Niño, DF, et al. The human milk oligosaccharides 2′-fucosyllactose and 6′-sialyllactose protect against the development of necrotizing enterocolitis by inhibiting toll-like receptor 4 signaling. Pediatr Res. (2021) 89:91–101. doi: 10.1038/s41390-020-0852-3
10. Azagra-Boronat, I, Massot-Cladera, M, Knipping, K, Van't Land, B, Stahl, B, Garssen, J, et al. Supplementation with 2'-FL and scGOS/lcFOS ameliorates rotavirus-induced diarrhea in suckling rats. Front Cell Infect Microbiol. (2018) 8:372. doi: 10.3389/fcimb.2018.00372
11. Azagra-Boronat, I, Massot-Cladera, M, Mayneris-Perxachs, J, Knipping, K, Van't, LB, Tims, S, et al. Immunomodulatory and prebiotic effects of 2′-fucosyllactose in suckling rats. Front Immunol. (2019) 31:1773. doi: 10.3389/fimmu.2019.0177
12. Guo, S, Guo, Y, Ergun, A, Lu, L, Walker, WA, and Ganguli, K. Secreted metabolites of Bifidobacterium infantis and Lactobacillus acidophilus protect immature human enterocytes from IL-1β-induced inflammation: a transcription profiling analysis. PLoS One. (2015) 10:e0124549. doi: 10.1371/journal.pone.0124549
13. Henrick, BM, Chew, S, Casaburi, G, Brown, HK, Frese, SA, Zhou, Y, et al. Colonization by B. infantis EVC001 modulates enteric inflammation in exclusively breastfed infants. Pediatr Res. (2019) 86:749–57. doi: 10.1038/s41390-019-0533-2
14. De Andrés, J, Manzano, S, García, C, Rodríguez, JM, Espinosa-Martos, I, and Jiménez, E. Modulatory effect of three probiotic strains on infants' gut microbial composition and immunological parameters on a placebo-controlled, double-blind, randomised study. Benefic Microbes. (2018) 9:573–84. doi: 10.3920/BM2017.0132
15. Reznikov, EA, Comstock, SS, Hoeflinger, JL, Wang, M, Miller, MJ, and Donovan, SM. Dietary bovine lactoferrin reduces Staphylococcus aureus in the tissues and modulates the immune response in piglets systemically infected with S. aureus. Curr Dev Nutr. (2017) 2:nzy001. doi: 10.1093/cdn/nzy001
16. Underwood, MA, German, JB, Lebrilla, CB, and Mills, DA. Bifidobacterium longum subspecies infantis: champion colonizer of the infant gut. Pediatr Res. (2015) 77:229–35. doi: 10.1038/pr.2014.156
17. Sakanaka, M, Gotoh, A, Yoshida, K, Odamaki, T, Koguchi, H, Xiao, JZ, et al. Varied pathways of infant gut-associated Bifidobacterium to assimilate human milk oligosaccharides: prevalence of the gene set and its correlation with bifidobacteria-rich microbiota formation. Nutrients. (2019) 12:71. doi: 10.3390/nu12010071
18. Zabel, B, Yde, CC, Roos, P, Marcussen, J, Jensen, HM, Salli, K, et al. Novel genes and metabolite trends in Bifidobacterium longum subsp. infantis bi-26 metabolism of human milk oligosaccharide 2′-fucosyllactose. Sci Rep. (2019) 9:7983. doi: 10.1038/s41598-019-43780-9
19. Daniels, VC, Monaco, MH, Wang, M, Hirvonen, J, Jensen, HM, Ouwehand, AC, et al. Evaluation of 2′-fucosyllactose and Bifidobacterium longum subspecies infantis on growth, organ weights, and intestinal development of piglets. Nutrients. (2021) 14:199. doi: 10.3390/nu14010199
20. Comstock, S, Wang, M, Hester, S, Li, M, and Donovan, S. Select human milk oligosaccharides directly modulate peripheral blood mononuclear cells isolated from 10-d-old pigs. Br J Nutr. (2014) 111:819–28. doi: 10.1017/S0007114513003267
21. Li, M, Monaco, MH, Wang, M, Comstock, SS, Kuhlenschmidt, TB, Fahey, GC Jr, et al. Human milk oligosaccharides shorten rotavirus-induced diarrhea and modulate piglet mucosal immunity and colonic microbiota. ISME J. (2014) 8:1609–20. doi: 10.1038/ismej.2014.10
22. Donovan, SM, and Comstock, SS. Human milk oligosaccharides influence neonatal mucosal and systemic immunity. Ann Nutr Metab. (2016) 69:42–51. doi: 10.1159/000452818
23. Rosa, F, Sharma, AK, Gurung, M, Casero, D, Matazel, K, Bode, L, et al. Human milk oligosaccharides impact cellular and inflammatory gene expression and immune response. Front Immunol. (2022) 13:907529. doi: 10.3389/fimmu.2022.907529
24. Vandenplas, Y, Berger, B, Carnielli, VP, Ksiazyk, J, Lagström, H, Sanchez Luna, M, et al. Human milk oligosaccharides: 2′-fucosyllactose (2'-FL) and lacto-N-neotetraose (LNnT) in infant formula. Nutrients. (2018) 10:1161. doi: 10.3390/nu10091161
25. Marriage, BJ, Buck, RH, Goehring, KC, Oliver, JS, and Williams, JA. Infants fed a lower calorie formula with 2'FL show growth and 2'FL uptake like breast-fed infants. J Pediatr Gastroenterol Nutr. (2015) 61:649–58. doi: 10.1097/MPG.0000000000000889
26. Smilowitz, JT, Moya, J, Breck, MA, Cook, C, Fineberg, A, Angkustsiri, K, et al. Safety and tolerability of Bifidobacterium longum subspecies infantis EVC001 supplementation in healthy term breastfed infants: a phase I clinical trial. BMC Pediatr. (2017) 17:133. doi: 10.1186/s12887-017-0886-9
27. Comstock, SS, Li, M, Wang, M, Monaco, MH, Kuhlenschmidt, TB, Kuhlenschmidt, MS, et al. Dietary human milk oligosaccharides but not prebiotic oligosaccharides increase circulating natural killer cell and mesenteric lymph node memory T cell populations in noninfected and rotavirus-infected neonatal piglets. J Nutr. (2017) 147:1041–7. doi: 10.3945/jn.116.243774
28. Radlowski, EC, Wang, M, Monaco, MH, Comstock, SS, and Donovan, SM. Combination-feeding causes differences in aspects of systemic and mucosal immune cell phenotypes and functions compared to exclusive sow-rearing or formula-feeding in piglets. Nutrients. (2021) 13:1097. doi: 10.3390/nu13041097
29. Zhang, JM, and An, J. Cytokines, inflammation, and pain. Int Anesthesiol Clin. (2007) 45:27–37. doi: 10.1097/AIA.0b013e318034194e
30. Xiao, L, van De Worp, WR, Stassen, R, van Maastrigt, C, Kettelarij, N, Stahl, B, et al. Human milk oligosaccharides promote immune tolerance via direct interactions with human dendritic cells. Eur J Immunol. (2019) 49:1001–14. doi: 10.1002/eji.201847971
31. Kainonen, E, Rautava, S, and Isolauri, E. Immunological programming by breast milk creates an anti-inflammatory cytokine milieu in breast-fed infants compared to formula-fed infants. Br J Nutr. (2013) 109:1962–70. doi: 10.1017/S0007114512004229
32. Chleilat, F, Klancic, T, Ma, K, Schick, A, Nettleton, JE, and Reimer, RA. Human milk oligosaccharide supplementation affects intestinal barrier function and microbial composition in the gastrointestinal tract of young Sprague Dawley rats. Nutrients. (2020) 12:1532. doi: 10.3390/nu12051532
33. Tau, G, and Rothman, P. Biologic functions of the IFN-gamma receptors. Allergy. (1999) 54:1233–51. doi: 10.1034/j.1398-9995.1999.00099.x
34. Kaur, S, Bansal, Y, Kumar, R, and Bansal, G. A panoramic review of IL-6: structure, pathophysiological roles and inhibitors. Bioorg Med Chem. (2020) 28:115327. doi: 10.1016/j.bmc.2020.115327
35. Goehring, KC, Kennedy, AD, and Prieto, PA. Buck RH direct evidence for the presence of human milk oligosaccharides in the circulation of breastfed infants. PLoS One. (2014) 9:e101692. doi: 10.1371/journal.pone.0101692
36. Ganguli, K, Meng, D, Rautava, S, Lu, L, Walker, WA, and Nanthakumar, N. Probiotics prevent necrotizing enterocolitis by modulating enterocyte genes that regulate innate immune-mediated inflammation. Am J Physiol Gastrointest Liver Physiol. (2013) 304:G132–41. doi: 10.1152/ajpgi.00142.2012
37. Ceuppens, JL, Baroja, ML, Lorre, K, Van Damme, J, and Billiau, A. Human T cell activation with phytohemagglutinin. The function of IL-6 as an accessory signal. J Immunol. (1988) 141:3868–74. doi: 10.4049/jimmunol.141.11.3868
38. Tawfik, DM, Lankelma, JM, Vachot, L, Cerrato, E, Pachot, A, Wiersinga, WJ, et al. Comparison of host immune responses to LPS in human using an immune profiling panel, in vivo endotoxemia versus ex vivo stimulation. Sci Rep. (2020) 10:9918. doi: 10.1038/s41598-020-66695-2
39. WHO (2020). Infant and young child feeding. World Health Organization. Available online at: www.who.int/news-room/fact-sheets/detail/infant-and-young-child-feeding (Accessed February 26, 2024).
40. Sutkus, LT, Joung, S, Hirvonen, J, Jensen, HM, Ouwehand, AC, Mukherjea, R, et al. Influence of 2′-fucosyllactose and Bifidobacterium longum subspecies infantis supplementation on cognitive and structural brain development in young pigs. Front Neurosci. (2022) 16:860368. doi: 10.3389/fnins.2022.860368
Keywords: B. infantis Bi-26, immune, cytokine, human milk oligosaccharides, 2-fucosyl-lactose
Citation: Daniels VC, Monaco MH, Hirvonen J, Ouwehand AC, Jensen HM, Mukerjea R, Christensen N, Lehtinen MJ, Dilger RN and Donovan SM (2024) Interactions between the human milk oligosaccharide 2′-fucosyllactose and Bifidobacterium longum subspecies infantis in influencing systemic immune development and function in piglets. Front. Nutr. 11:1444594. doi: 10.3389/fnut.2024.1444594
Edited by:
Veronique Demers-Mathieu, Janssen Pharmaceutical Companies of Johnson & Johnson, United StatesReviewed by:
Qun Zhou, Sanofi Genzyme, United StatesDouglas G. Burrin, United States Department of Agriculture (USDA), United States
Copyright © 2024 Daniels, Monaco, Hirvonen, Ouwehand, Jensen, Mukerjea, Christensen, Lehtinen, Dilger and Donovan. This is an open-access article distributed under the terms of the Creative Commons Attribution License (CC BY). The use, distribution or reproduction in other forums is permitted, provided the original author(s) and the copyright owner(s) are credited and that the original publication in this journal is cited, in accordance with accepted academic practice. No use, distribution or reproduction is permitted which does not comply with these terms.
*Correspondence: Sharon M. Donovan, c2Rvbm92YW5AaWxsaW5vaXMuZWR1
†Present address: Ratna Mukerjea, Benson Hill, Saint Louis, MO, United States