- 1College of Physical Education, LiaoNing Petrochemical University, Fushun, Liaoning, China
- 2School of Medicine, Tehran University of Medical Sciences, Tehran, Iran
Ashwagandha or Withania somnifera is an herbal plant belonging to the Solanaceae family. Because of its wide range of phytochemicals, ashwagandha root extract has been used in numerous research studies, either alone or in conjunction with other natural plants, for various biomedical applications, which include its anti-microbial, anti-inflammatory, anti-stress, anti-tumor, cardioprotective, and neuroprotective properties. Additionally, it improves endothelial function, lowers reactive oxygen species, controls apoptosis, and improves mitochondrial function. These properties make it a useful treatment for a variety of conditions, including age-related symptoms, anxiety, neurodegenerative diseases, diabetes, stress, arthritis, fatigue, and cognitive/memory impairment. Despite the numerous benefits of ashwagandha supplementation, there have been just four meta-analyses on the herb’s effectiveness in treating anxiety, neurobehavioral disorders, impotence, and infertility. Moreover, no reviews exist that examine how ashwagandha affects antioxidant response and physical sports performance. Consequently, the goal of this study was to analyze the scientific literature regarding the effects of ashwagandha consumption on antioxidant response and athletic performance.
Introduction
A resistance training program consists of exercises that force skeletal muscles to contract against external resistance. Such regimens frequently cause the body to increase muscle mass and produce greater strength (1, 2). However, studies have shown that prolonged and/or high-intensity exercise can damage muscle tissue. In addition, oxidative stress and inflammatory cytokines damage biomolecules, which may lead to additional muscle injury caused by the rise in free radical levels following muscle damage (3). Physical fitness affects the formation of reactive oxygen species (ROS) and causes changes in antioxidant enzymes, which can lead to different degrees of oxidative damage and lipid peroxidation (4, 5). Fatigue and compromised cellular and muscular function have been linked to oxidative stress (6, 7). Superoxide dismutase (SOD), glutathione peroxidase (GPx), and catalase (CAT) are antioxidant enzymes acting as the first line of defense to prevent the generation of free radicals and ROS (8).
Numerous investigations have evaluated the efficacy of dietary alterations and micronutrient supplements in mitigating the oxidative stress generated by exercise (9, 10). Certain medicinal herbs can improve exercise capacity and prevent illness because they contain antioxidants and antifatigue compounds (11). It was reported that supplementation with ashwagandha may enhance the adaptations and gains produced by exercise, making it a helpful addition to a resistance training regimen. There are some justifications for this theory. Ashwagandha has been shown in studies to enhance muscular strength and coordination as well as cardiorespiratory endurance in healthy, normal individual (12, 13). Ashwagandha is classified as a “rasayana” or Ayurvedic rejuvenation therapy which has been used for ages to promote health, increase lifespan, slow down aging, revitalize the body, and produce overall wellbeing (14, 15). Ashwagandha has been reported to possess an extensive spectrum of pharmacological activity, including analgesic, anti-inflammatory, sedative, hypotensive, anxiolytic, immunomodulatory, central nervous system, cardiac, anabolic, and antioxidant properties (14, 16–18). Moreover, it increases thyroid activity and respiratory function, and relaxes smooth muscle (19). Human studies showed that ashwagandha was well tolerated and linked to an increase in testosterone (20, 21) and a decrease in cortisol (22). Ashwagandha may lessen the rise in blood urea nitrogen, lactic acid, and corticosterone that occurs during stress and exercise (14). It may also lessen the activation of dopamine receptors in the brain during stressful conditions (16, 23).
The fact that ashwagandha has several active ingredients may explain the different mechanisms of action. These include compounds like anaferine, isopelletierine, steroidal lactones (withaferins and withanolides), and saponins (23–25).
As exercise may be thought of as a kind of acute stress, and the stress response after consumption of ashwagandha results in improvements in human physical performance, the adaptagenic properties of ashwagandha means it could act as an active ergogenic supplement. Therefore, we summarize the studies that have investigated the role of ashwagandha in exercise performance, antioxidant responses, and increased adaptation.
Ashwagandha and antioxidant responses
The antioxidant properties of ashwagandha have been documented using in vitro cell culture, in vivo animal research, and clinical trials involving healthy individual (24, 26–28). Additionally, researchers have proposed that ashwagandha could be beneficial for those with oxidative stress-related diseases (29). Ashwagandha has been shown to contain high levels of flavonoids, phenolic compounds, and antioxidant compounds (30). Therefore ashwagandha can repair oxidative damage in cells and lipid peroxidation, as well as combat the formation of reactive oxygen species (ROS) as shown in Figure 1. When ROS attack cell membranes, they produce harmful lipid peroxides and malondialdehyde (MDA) (31). Nuclear factor erythroid 2-related factor 2 (Nrf2) controls the gene expression of antioxidant enzymes involved in combating oxidative damage by activating the PI3K/PTEN/Akt pathway. The compound Withaferin A has been found to have beneficial effects on the nervous system, such as preventing cell death and promoting cell growth. This is achieved by inhibiting the protein PTEN and activating the PI3K/AKT/mTOR and PI3K/AKT/GSK3β pathways, as well as preventing the movement of vascular smooth muscle cells (Figure 2) (32, 33). Nrf2 is also strongly induced by withaferin A where it has a cytoprotective effect (34, 35). Antioxidant proteins, including heme oxygenase-1 (HO-1) and heat shock protein 70 (HSP70), as well as antioxidant enzymes like CAT and SOD, glutathione (GSH), GSH reductase, GPx, thioredoxin (Trx), and Trx reductase are all downstream products of Nrf2 activation (36). Since these enzymatic and protein antioxidants do not need to be continuously produced, they show a longer duration of action compared to vitamins and coenzymes, which are depleted during the initial redox reactions (37). In healthy people, the antioxidant function of the bioactive compounds in ashwagandha, support the herb’s usage in promoting oxidative equilibrium and possibly preventing disorders linked to oxidative stress.
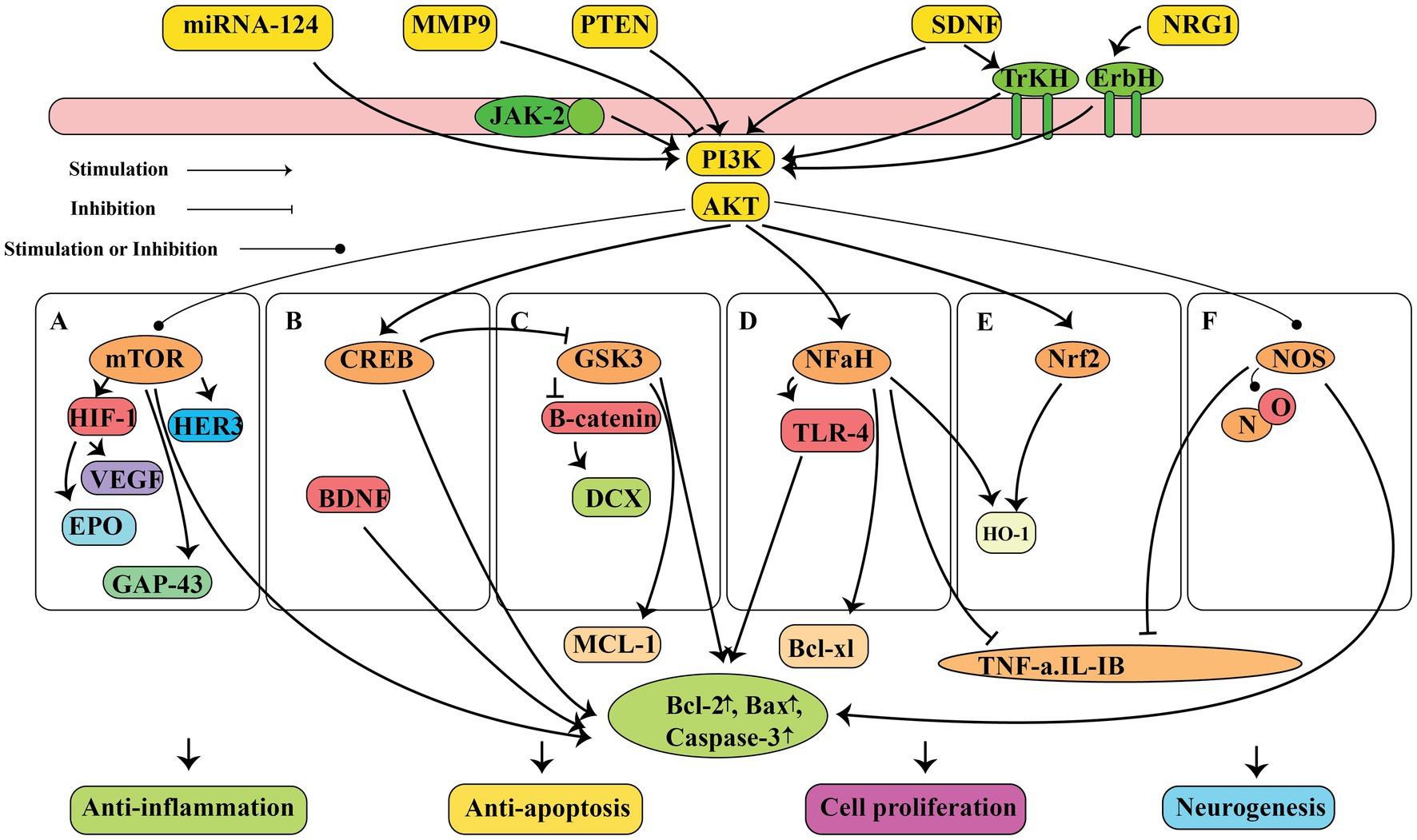
Figure 2. The neuroprotective properties of ashwagandha is attributed to the molecular and pathway mechanisms, such as suppression of inflammation, inhibition of cell death, promotion of cell growth, and stimulation of neurogenesis. These effects are mediated by the PI3K/AKT signaling pathway, which activates different pathways including mTOR, CREB, GSK3, NF-κB, Nrf2, and NOS. This figure adapted from Gu et al. (32).
Ashwagandha and brain function
Extensive research has been conducted on the diverse physiological effects of Withania somnifera (WS), with a particular focus on its potential use to treat brain disorders (14, 38). The effects of WS root and WS leaf on the nervous system have been investigated in both preclinical and clinical studies. Two recent reviews have comprehensively compiled and analyzed the findings, providing evidence for the effectiveness of WS in treating neurodegenerative diseases such as Alzheimer’s, Huntington’s, and Parkinson’s (17, 39–41). One of the primary reasons for utilizing ashwagandha products is to alleviate stress, which is a common practice in some populations. There is widespread acknowledgement that stress can lead to both physiological and anatomical alterations within the brain, and stress has been linked to the development of several neuropsychiatric conditions such as anxiety, depression, and insomnia (42). The ways in which stress plays a role in these disorders involve increased activity of the hypothalamic–pituitary–adrenal (HPA) axis and disruption of the normal function of the immune system (43, 44). Based on the strong connection between stress and neuropsychiatric disorders, the anti-stress properties of WS are believed to play a crucial role in its potential benefits for depression, anxiety, and insomnia.
The mechanism of anxiety largely involves GABAergic neurotransmission, as GABA is the primary inhibitory neurotransmitter in the central nervous system (45). The chief location where GABA agonist drugs exert their effects is at GABA type A (GABAA) receptors, which enhance GABAergic function, and these drugs are frequently prescribed for managing anxiety disorders (46). Extensive research in non-human subjects has indicated that substances present in WS actively engage and regulate GABAA receptors, potentially explaining the anxiety-reducing effect of WS. The initial evidence of the ability of WS to mimic GABA was reported by Mehta et al. in 1991. The researchers discovered that a methanolic extract of WS root increased the influx of chloride ions in spinal cord neurons of mammals without the presence of GABA. The extract also hindered the binding of GABA to its receptor similar to how GABAA receptor agonists act (47). Research studies using receptor-binding assays have confirmed that the substances found in methanolic extracts of WS root show a strong binding to GABAA receptors, while they show a noticeably weaker binding to GABAB, glutamatergic, and opioid receptors (48). The GABAA receptor-specific function of WS has been confirmed by various animal experiments. The effects of morphine and ethanol on the dopamine-producing neurons in the ventral tegmental area of the rat brain were inhibited by a methanolic extract of WS roots. This was attributed to the activation of the GABAA pathway, rather than the GABAB pathway (49). In a mouse experiment, it was observed that a small amount of an unspecified extract from WS roots helped increase the threshold for seizures caused by pentylenetetrazol (PTZ). This effect was enhanced when combined with low doses of GABA to activate GABA receptors, and diazepam, which alters the function of GABAA receptors (50). In a previous mouse experiment, Kulkarni and colleagues (1993) reported that a methanolic extract of WS (specific plant parts not mentioned) in conjunction with pentobarbital (a GABAA receptor activator) resulted in better defense against PTZ-induced damage, compared to each agent on its own (51). Moreover, when a lower dose of WS was added to GABA, it enhanced the protective properties of the extract.
In a model using rats to simulate sleep disruption, the administration of a specific GABAA activator (muscimol) increased the sedative properties of an unspecified root extract from the WS plant. Conversely, the use of a GABAA receptor blocker (picrotoxin) counteracted this effect (52). Inhibitors of GABAA receptors, specifically picrotoxin and bicuculline, completely abrogated the ability of both methanolic and aqueous extracts from WS roots to cause depolarization in gonadotropin releasing hormone neurons in mice. These inhibitors also prevented the increase of inward ion currents in substantia gelatinosa neurons in mice, as well as in GABAA channels in rat brains (53).
Because the brain is particularly vulnerable to oxidative stress, and anxiety disorders are characterized by a decrease in protective antioxidants as well as an increase in oxidative damage, treatments that protect against oxidative stress are desirable (54, 55). Multiple suggested mechanisms have been suggested to explain how oxidative stress plays a role in brain disorders. Oxidative stress can act as a trigger for these disorders and can also be a result of neuroinflammation, which has also been linked to brain-related disorders (56). Figure 3 shows how the components of ashwagandha can affect cell signaling pathways and the production of inflammatory mediators. Inflammation in peripheral tissues may directly play a role in increasing neuroinflammation and oxidative stress in the brain (56). Although there is evidence linking both oxidative stress and inflammation to brain-related disorders, it has not been conclusively proven which one causes the other or vice versa (57). Studies on animals exploring the effects of WS on anxiety have revealed a strong link between improvements in anxiety-related behavior and the amelioration of oxidative stress and inflammatory indicators.
The root extract and leaf extract of WS, although not clearly described, were able to increase the levels of catalase activity and reduced glutathione (GSH) in the brain, and also lower the levels of lipid peroxidation in a mouse model of sleep deprivation and a zebrafish model of neurotoxicity induced by benzo[a]pyrene (58, 59). Furthermore, WS demonstrated the ability to decrease nitrite levels in the mouse model and also lower protein carbonylation in the zebrafish model (58, 59). In an experiment using rats to simulate an ischemic stroke, a uniform hydroalcoholic extract derived from WS roots effectively decreased the levels of lipid peroxidation and enhanced antioxidant function in the brain (60). ASH-WEX, an aqueous leaf extract, was shown to decrease pro-inflammatory cytokines, specifically TNFα and IL-6, both in the peripheral and central nervous systems, in animal models of neuroinflammation and sleep deprivation (61). ASH-WEX produced a significant reduction in indicators of reactive gliosis, such as GFAP, as well as neuroinflammation, such as NOX2, iNOS, and COX2. Furthermore, it successfully regulated various inflammatory pathways and reduced cellular death in the brain (62). In a rat experiment examining the effects of a high fat diet on obesity, the use of WS dry leaf powder significantly lessened the expression of pro-inflammatory cytokines both in the body and brain, decreased indicators of reactive gliosis and neuroinflammation, modulated the nuclear factor NF-kappa-B (NF-κB) pathway, and lowered cell death (63).
Supplementation with ashwagandha has been shown to lower C-reactive protein (CRP) activity (64), one of the most important indicators of inflammation. Furthermore, other studies have shown that ashwagandha exerts a number of anti-inflammatory effects on chronic inflammation-mediated diseases (65), particularly rheumatoid arthritis (66), inflammatory bowel disorder (67), and systemic lupus erythematosus (68). These studies suggest that ashwagandha may be a useful tool for reducing the cytokine storm. Withanolides, particularly Withaferin A, are responsible for the majority of ashwagandha’s anti-inflammatory effects, according to several reports (69, 70). Ashwagandha may work by interacting with components of the proinflammatory cell signaling pathway, such as NF-κB, signaling kinases, HSP90, Nrf2, and the inflammasome complex, even though the mechanisms behind the anti-inflammatory effect of withanolides are not fully understood (71). Since the NF-κB transcription factor family is implicated in a number of chronic disorders caused by inflammation, individuals with high NF-κB levels may benefit from therapeutic targeting of NF-κB. In this situation, ashwagandha has the ability to inhibit and mediate the activity of the NF-κB pathway (72). It is thought that strong protein kinase inhibitor activity is necessary for ashwagandha to function. Ashwagandha has the ability to inhibit the signaling cascades of protein kinases, which are essential in inflammatory pathways (72). Furthermore, it appears that kinase inhibition takes place when nitric oxide synthesis is inhibited, which also benefits the inflammatory process. Another possible explanation for ashwagandha’s anti-inflammatory properties is the downregulation or destabilization of HSP activity, which is implicated in regulatory kinase pathways. As previously mentioned, ashwagandha regulates Nrf2 to moderate oxidative stress (34, 73). Nrf2 activation may account for ashwagandha’s anti-inflammatory properties, as oxidative stress frequently takes place in sites of inflammation and is thought to be one cause of chronic inflammation (74). Finally, by blocking inflammasomes, cytokines, and other multiprotein pro-inflammatory complexes, ashwagandha may lessen inflammation (72, 75).
According to some reports, ashwagandha is an adaptogen that, due to its antioxidant properties, boosts immunity, helps the body respond to stress more effectively, increases resilience, and fights oxidative stress and cellular damage (76, 77). It has been demonstrated that the bioactive C28-steroidal lactones present in WS leaves possess neuroprotective, anxiolytic, antioxidant, and anti-inflammatory properties. Moreover, Withanoside IV and its metabolite sominone, found in WS roots, have been shown to promote synaptogenesis and neuronal outgrowth. Furthermore, it has been demonstrated that the herb inhibits acetylcholinesterase and protects rats from cognitive decline (78). One study evaluated the effects of ashwagandha root extract 300 mg twice a day in humans with moderate cognitive impairment (79). After 8 weeks, the treatment group outperformed the placebo group in tests measuring immediate and general memory, information-processing speed, executive function, and attention. However, the benefits on working memory and visuospatial processing were not definitive because there was little difference between the two groups’ performance on these tasks. Another study examined the effect of ashwagandha extract on cognitive impairment in individuals with bipolar disorder (80). Tests were conducted at baseline and after the intervention, with rats randomly allocated to receive 500 mg/day of ashwagandha or a placebo for 8 weeks. When compared to the placebo, subjects in the treatment group showed significantly better results on the Flanker Test (neutral mean reaction time), the Penn Emotional Acuity Test (mean social cognition response rating), and the Auditory Digit Span (mean digit span backward). These findings suggested that ashwagandha extract may safely enhance cognitive function in bipolar disorder patients, including verbal working memory, response time, and social cognition response. Another study was carried out using ashwagandha root extract on a group of horses. The animals were subjected to a variety of stressors, including loud noises, prolonged physical activity, and separation. Following a 21-day period, the treated group showed a statistically significant reduction in cortisol, glucose, adrenaline, IL-6, lipids, creatinine, aspartate aminotransferase, and alanine aminotransferase (81).
Effects of ashwagandha on sports performance
The physiological measure known as maximum oxygen consumption, or VO2 max, acts as a measure of an individual’s aerobic capability. It is a measure of cardiorespiratory fitness that governs both athletic performance and overall health condition (82, 83). With an emphasis on competitive sports, the VO2 max is one of the important variables that control success in endurance activities (84), along with running efficiency and the anaerobic threshold. It also helps to improve team sports performance by raising the intensity of work, the distance covered, and the quantity of sprints completed (85, 86). In the realm of general health, VO2 max holds particular significance beyond athletic performance. In adults and the elderly, low VO2 max values have been linked to an elevated risk of death and the loss of an independent lifestyle (87), whereas high cardiorespiratory fitness levels have been linked to a lower risk of cardiovascular disease (88, 89). Since a stronger aerobic capacity is linked to a higher quality of life, the VO2 max level is also significant in children (90).
Table 1 lists some clinical trials that have examined the effect of ashwagandha on VO2 max, sports performance and metabolic profiles. The ability of Indian cyclists to sustain cardiorespiratory endurance was assessed in a clinical trial of ashwagandha supplements. Forty top-level Indian cyclists were divided into groups for the intervention and placebo. For 8 weeks, the experimental group was given 500 mg capsules containing aqueous ashwagandha root extract twice a day, while the placebo group received starch capsules for 8 weeks. Compared to the placebo group, which did not exhibit any changes in baseline levels, the experimental group showed a substantial improvement in all parameters, including VO2 max, time to exhaustion on the treadmill, and METS (metabolic equivalents) (91). The conclusions showed that the anaerobic capacity of both males and females had significantly improved (92).
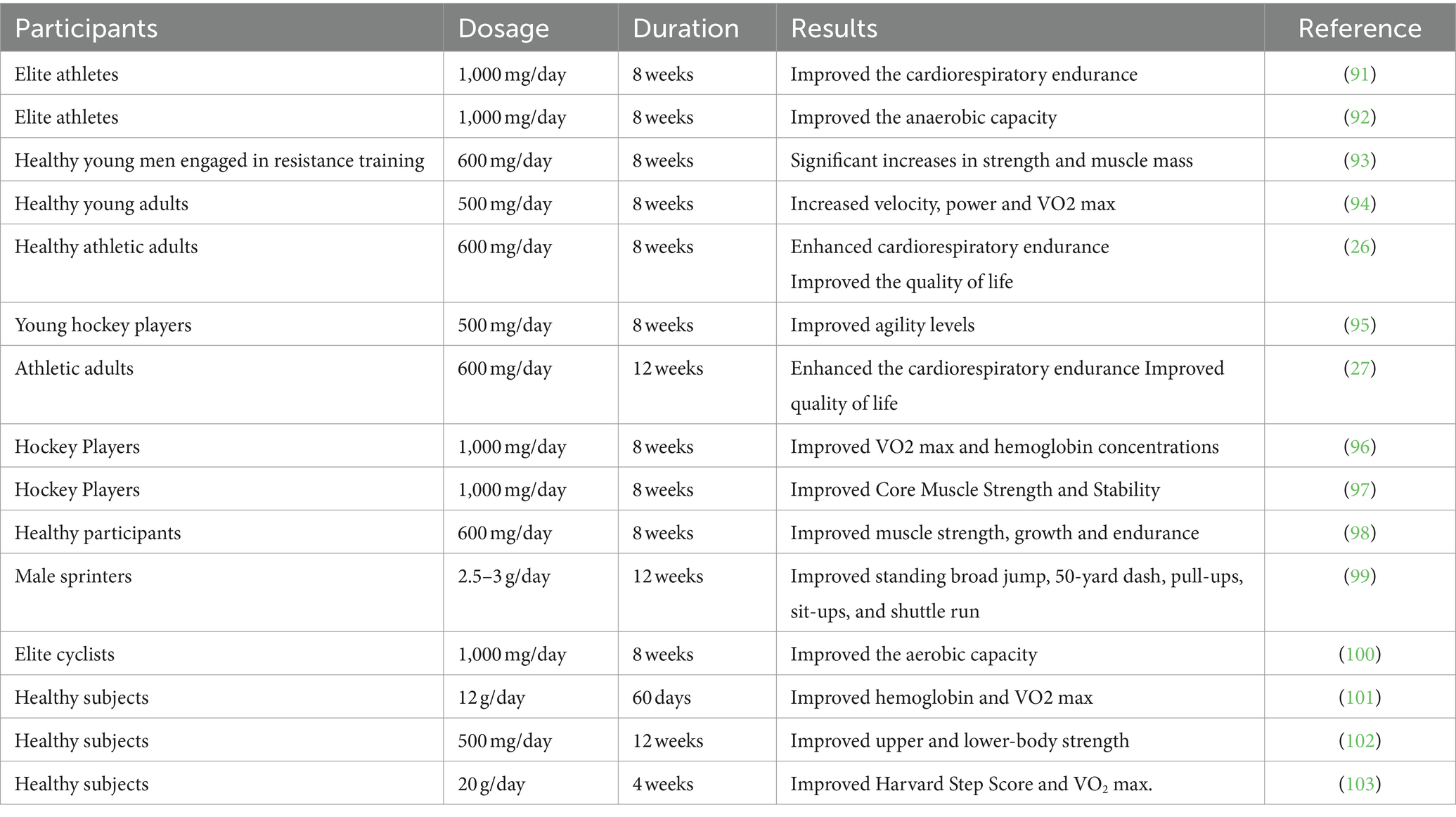
Table 1. Clinical trials examining the effects of ashwagandha on VO2 max, sports performance and metabolic profiles.
Another study was carried out on healthy subjects undergoing resistance training to investigate the potential benefits of ashwagandha root extract intake on strength and muscle mass. Fifty-seven young male individuals with no prior resistance training experience were divided into treatment and placebo groups for the 8-week trial. The control group was given starch placebo capsules, while the treatment group received 300 mg/twice a day of ashwagandha root extract. After completing baseline assessment, both subject groups engaged in resistance training for 8 weeks, with follow-up measures conducted at the conclusion of the eighth week. The 1-RM load for the bench press and leg extension movements was used to assess muscle strength. Creatine kinase levels, a measure of muscle damage caused by exercise, was used to assess muscle healing. When compared to the group of subjects given a placebo, those treated with ashwagandha saw a significant increase in muscle strength when performing the bench-press exercise (an increase of 46.0 kg compared to 26.4 kg for the placebo group), as well as the leg-extension exercise (an increase of 14.5 kg compared to 9.8 kg for the placebo group). Additionally, the ashwagandha group also had a greater increase in muscle size at the arms (5.3 cm2 compared to 7.2 cm2 for the placebo group). These results were statistically significant, with p-values of 0.001 and 0.04 for the bench-press and leg-extension exercises, respectively. According to the study, participants who took ashwagandha had significantly improved muscle strength in their arms (8.6 cm, 95% CI, 6.9, 10.8; p = 0.01) and chest (3.3 cm, 95% CI, 2.6, 4.1; p < 0.001) compared to those who took the placebo. Additionally, those taking ashwagandha also showed decreased levels of exercise-induced muscle damage, as shown by their stabilized serum creatine kinase levels (1307.5 U/L, 95% CI, 1202.8, 1412.1) compared to the placebo group (1.4 cm, 95% CI, 0.8, 2.0). Ashwagandha supplementation resulted in a significant increase in catalase activity (1462.6 U/L, 95% CI, 1366.2, 1559.1; p = 0.03), a significantly higher rise in testosterone levels (18.0 ng/dL, 95% CI, −15.8, 51.8 vs. 96.2 ng/dL, 95% CI, 54.7, 137.5; p = 0.004), and a significant decrease in body fat percentage (1.5, 95% CI, 0.4, 2.6% vs. 3.5, 95% CI, 2.0, 4.9%; p = 0.03) compared to the placebo group (93).
Ashwagandha and arjuna (Terminalia arjuna) were shown to have different effects on the average absolute and relative power, VO2 max, maximum velocity, blood pressure, and balance in humans, both separately and in combination. Forty healthy subjects were assigned to experimental groups, 10 of whom received standardized extracts of ashwagandha root, 10 of whom received standardized extracts of Terminalia arjuna bark, 10 of whom received a combination of both herbal extracts, and the remaining 10 received flour-filled capsules as a placebo. Each medication was administered as capsules, with a daily dose of 500 mg. Ashwagandha was shown to improve power, velocity, and VO2 max, whereas arjuna was found to boost VO2 max and decrease resting systolic blood pressure. All indicators displayed improvement when both extracts were administered together, with the exception of diastolic blood pressure and balance (94).
Another study evaluated the effectiveness and safety of ashwagandha in improving cardiorespiratory endurance in fit subjects. Fifty healthy, fit individuals were randomly divided into two groups and given equal amounts of placebo and ashwagandha for 8 weeks. The ashwagandha group was given 300 mg of ashwagandha root extract capsules, twice a day. VO2 max testing and cardiorespiratory endurance was evaluated. The findings showed that the ashwagandha group’s VO2 max value was significantly higher than the placebo group. By the conclusion of the trial, the participants in the ashwagandha group showed a statistically significant rise above their baseline. Those in the ashwagandha group showed significantly higher Total Quality Recovery Scores (TQR) than those in the placebo group. When comparing the Ashwagandha group to the placebo group, the Daily Analysis of Life Demands for Athletes (DALDA) questionnaire revealed statistical significance. Better results were also obtained in the Recovery-Stress Questionnaire for Athletes (RESTQ) evaluation, particularly for lack of energy, fatigue recovery, and fitness analysis. In the ashwagandha group, there was also a notable increase in antioxidant levels (26).
An investigation into the effect of ashwagandha supplementation on hockey players’ agility level was carried out. A total of 52 male hockey players were randomly separated into two groups. Group II was a placebo control group and Group I was the experimental group receiving WS. For 8 weeks, the experimental group took ashwagandha 500 mg/twice a day, whereas the placebo group took starch capsules. The Illinois Agility Run Test Getchell Test was used to measure the level of agility in the control and experimental groups prior to and following the consumption of placebo or ashwagandha. The results showed that after 4 and 8 weeks, the agility level in the ashwagandha group had significantly improved. However, there was no discernible improvement in agility levels in the placebo group (95). Another report investigated the effects of ashwagandha supplements on hockey players’ VO2 max and hemoglobin levels (Hb) in this group of hockey players. Before and after the ashwagandha and placebo were administered, the experimental and control group VO2 max and Hb were monitored. The conclusions showed that the experimental group’s VO2 max and Hb had significantly improved (96).
After 4 and 8 weeks, respectively, the experimental group core muscle strength and stability had significantly improved. On the other hand, following 8 weeks of placebo treatment, there was no discernible increase in core muscle strength & stability in the control group (97).
In a different trial, 50 healthy athletes were assessed for their quality of life and how well their cardiorespiratory endurance was improved by ashwagandha root extract. A 20-meter shuttle run test was used to measure VO2 max in order to evaluate cardiorespiratory endurance. The findings showed that after 8 and 12 weeks, ashwagandha supplementation increased mean VO2 max from baseline more than the placebo. At 12 weeks, the quality of life scores in the ashwagandha group considerably improved in comparison to the placebo group in all subdomains (27).
An investigation was conducted on the effects of 600 mg of standardized ashwagandha on strength, muscle mass, and cardiorespiratory endurance after resistance exercise. Eighty healthy male and female volunteers who regularly exercised were divided into two groups and given either 300 mg of ashwagandha supplements twice a day for 8 weeks, or an equivalent placebo. Every participant engaged in resistance training for 8 weeks. VO2 max, muscle mass, and strength were measured at baseline and after 8 weeks. According to the findings, ashwagandha improved endurance, leg press, and bench press performance more than a placebo. Moreover, both male and female ashwagandha users showed higher gains in muscle girth for the chest, arm, and thigh (98).
Another trial examined the impact of ashwagandha consumption on specific physical fitness metrics in male sprinters. The 20 male sprinters were split evenly between experimental and control groups. For 12 weeks, ashwagandha was administered to the experimental group three times a week on alternate days taken with milk. Following a 12-week period, the 20 male sprinters undertook a physical fitness test. According to the results, ashwagandha significantly improved the standing broad jump, number of pull-ups, 50-yard dash, number of sit-ups, and shuttle run performance (99).
Another study was designed to examine the effects of ashwagandha supplements on the physical performance of top Indian cyclists based on gender differences. The study used an identical experimental design with 13 males and 19 females totaling 38 cyclists. METs and VO2 max measures of aerobic capacity were assessed directly using the Bruce technique. For 8 weeks, the experimental group received ashwagandha supplements, whereas the control group received capsules containing starch. The findings showed that whereas average power and peak power greatly improved in females, VO2 max improved significantly in males. After 8 weeks, males using ashwagandha supplements showed improvements in their aerobic and anaerobic capacity (100).
The effectiveness of ashwagandha in increasing VO2 max in healthy participants was assessed in a controlled, single-blind, randomized clinical trial. Two groups each consisted of 54 healthy volunteers, the study group started the day with 12 g of ashwagandha Choorna and 200 mL of milk on an empty stomach, whereas the control group merely had 200 mL of milk. The VO2 max of the study and control groups was assessed using the Rockport fitness walking test on days zero, 60 and 90 of follow-up. It was discovered that the study group VO2 max and Hb had significantly improved (101).
The effect of Sensoril® supplements on strength training adaptation was investigated in another trial. Men who undertook recreational activity were divided into double-blinded groups assigned to receive 500 mg/day of Sensoril® or a placebo. Measurements included 7.5 km cycling time trial, clinical blood chemistry, DEXA measurements of muscle strength, endurance, power, and body composition, at baseline and after 12-weeks of supplementation. The subjects continued to eat normally and adhered to a resistance-training regimen of progressive overload (4 days per week, divided between upper and lower body). The results showed that S500 produced much higher increases in the 1-RM squat and bench press performance. A shift in the android/gynoid ratio determined by DEXA also favored S500. Systemic hemodynamics, visual analog scales for affect and recovery, and body composition did not show any between-group differences. However, only the S500 group showed significant improvements in peak bench press power, average squat power, 7.5 km time trial performance, and perceived recovery scores. There was only a small polycythemia effect in placebo, according to the clinical chemistry measurement, and no additional statistically or clinically significant alterations were found (102).
Rasayana dravyas is a potential dietary supplement containing granules of ashwagandha, Jeeraka (Eleusine coracana), Shatavari (Asparagus racemosus), Mudga (Vigna radiata), Ragi (finfer millet), and Shunti (Zingiber officinale). Twenty-three subjects participated in an open-label, double-arm, controlled clinical trial using a modified wait-listed crossover design. For the duration of the trial, they were given 150 mL of milk and 20 g of ashwagandhadi granules in Rasayana dravyas daily for 1 month. The results revealed a statistically significant difference in the VO2 max and Harvard Step Score for the treatment group compared to the placebo group (103).
Practical applications
This review has provided useful information that will help sports performance practitioners in real-world scenarios, and to understand how supplements designed to reduce and control ROS damage work. In particular, it focuses on ashwagandha supplementation for improving athletic performance and modulating antioxidant response. In order to boost performance, sports teams may decide to adopt new dietary guidelines and working practices as a result of this evaluation.
Conclusion
Evidence suggests that ROS are key players in physiological signaling networks that control how the body reacts to exercise, and that physical activity-induced oxidative stress and inflammation are necessary for training adaption. The process of physiological adaptation requires a proper balance between antioxidants and free radicals, and it is possible that athletes who take antioxidants could counteract the advantageous effects of ROS in normal cell signaling. According to this theory, ROS produced during exercise are thought to activate PGC1-α and MAPK, two biochemical pathways essential for both muscle growth and aerobic capacity. Some studies have proposed that a brief rise in reactive oxygen species (ROS) brought on by physical activity may have advantageous consequences, including controlling the contraction of muscles, promoting muscle regeneration, and enhancing vasodilation while exercising. On the other hand, oxidative stress and elevated ROS levels can damage tissues and cells and induce inflammation. Many endurance athletes consume diets deficient in antioxidants to maintain their high demands for energy to carry out physical activity, despite their demanding training schedules. Although ashwagandha pills are frequently advised for endurance athletes, the numerous health advantages of exercise alone may make then unnecessary.
Author contributions
SG: Data curation, Investigation, Methodology, Project administration, Resources, Software, Supervision, Visualization, Writing – original draft, Writing – review & editing. MR: Conceptualization, Data curation, Investigation, Methodology, Project administration, Supervision, Visualization, Writing – original draft, Writing – review & editing.
Funding
The author(s) declare that no financial support was received for the research, authorship, and/or publication of this article.
Conflict of interest
The authors declare that the research was conducted in the absence of any commercial or financial relationships that could be construed as a potential conflict of interest.
Publisher’s note
All claims expressed in this article are solely those of the authors and do not necessarily represent those of their affiliated organizations, or those of the publisher, the editors and the reviewers. Any product that may be evaluated in this article, or claim that may be made by its manufacturer, is not guaranteed or endorsed by the publisher.
References
1. Kraemer, WJ, and Ratamess, NA. Fundamentals of resistance training: progression and exercise prescription. Med Sci Sports Exerc. (2004) 36:674–88. doi: 10.1249/01.MSS.0000121945.36635.61
2. Galvão, DA, Newton, RU, and Taaffe, DR. Anabolic responses to resistance training in older men and women: a brief review. J Aging Phys Act. (2005) 13:343–58. doi: 10.1123/japa.13.3.343
3. Nobari, H, Nejad, HA, Kargarfard, M, Mohseni, S, Suzuki, K, Carmelo Adsuar, J, et al. The effect of acute intense exercise on activity of antioxidant enzymes in smokers and non-smokers. Biomol Ther. (2021) 11:171. doi: 10.3390/biom11020171
4. Zhang, J, Yang, Y, Han, H, Zhang, L, and Wang, T. Bisdemethoxycurcumin protects small intestine from lipopolysaccharide-induced mitochondrial dysfunction via activating mitochondrial antioxidant systems and mitochondrial biogenesis in broiler chickens. Oxidative Med Cell Longev. (2021) 2021:1–18. doi: 10.1155/2021/9927864
5. Zhang, J, Han, H, Zhang, L, and Wang, T. Dietary bisdemethoxycurcumin supplementation attenuates lipopolysaccharide-induced damages on intestinal redox potential and redox status of broilers. Poult Sci. (2021) 100:101061. doi: 10.1016/j.psj.2021.101061
6. Mariacher, C, Gatterer, H, Greilberger, J, Djukic, R, Greilberger, M, Philippe, M, et al. Effects of antioxidant supplementation on exercise performance in acute normobaric hypoxia. Int J Sport Nutr Exerc Metab. (2014) 24:227–35. doi: 10.1123/ijsnem.2013-0118
7. Powers, S, Nelson, WB, and Larson-Meyer, E. Antioxidant and vitamin D supplements for athletes: sense or nonsense? J Sports Sci. (2011) 29:S47–55. doi: 10.1080/02640414.2011.602098
8. Liu, D, Liu, DC, Fan, H, and Wang, Y. Lactobacillus fermentum CQPC08 attenuates exercise-induced fatigue in mice through its antioxidant effects and effective intervention of Galactooligosaccharide. Drug Des Devel Ther. (2021) 15:5151–64. doi: 10.2147/DDDT.S317456
9. Sureda, A, Tejada, S, Bibiloni Mdel, M, Tur, JA, and Pons, A. Polyphenols: well beyond the antioxidant capacity: polyphenol supplementation and exercise-induced oxidative stress and inflammation. Curr Pharm Biotechnol. (2014) 15:373–9. doi: 10.2174/1389201015666140813123843
10. Malaguti, M, Angeloni, C, and Hrelia, S. Polyphenols in exercise performance and prevention of exercise-induced muscle damage. Oxidative Med Cell Longev. (2013) 2013:825928:1–9. doi: 10.1155/2013/825928
11. Zhang, Y, Li, A, and Yang, X. Effect of lemon seed flavonoids on the anti-fatigue and antioxidant effects of exhausted running exercise mice. J Food Biochem. (2021) 45:e13620. doi: 10.1111/jfbc.13620
12. Raut, AA, Rege, NN, Tadvi, FM, Solanki, PV, Kene, KR, Shirolkar, SG, et al. Exploratory study to evaluate tolerability, safety, and activity of Ashwagandha (Withania somnifera) in healthy volunteers. J Ayurveda Integr Med. (2012) 3:111–4. doi: 10.4103/0975-9476.100168
13. Vaidya, VG, Gothwad, A, Ganu, G, Girme, A, Modi, SJ, and Hingorani, L. Clinical safety and tolerability evaluation of Withania somnifera (L.) Dunal (Ashwagandha) root extract in healthy human volunteers. J Ayurveda Integr Med. (2024) 15:100859. doi: 10.1016/j.jaim.2023.100859
14. Mishra, LC, Singh, BB, and Dagenais, S. Scientific basis for the therapeutic use of Withania somnifera (ashwagandha): a review. Alternative Med Rev: J Clin Therapeutic. (2000) 5:334–46.
15. Winters, M. Ancient medicine, modern use: Withania somnifera and its potential role in integrative oncology. Alternative Med Rev: J Clin Therapeutic. (2006) 11:269–77.
16. Kulkarni, SK, and Dhir, A. Withania somnifera: an Indian ginseng. Prog Neuro-Psychopharmacol Biol Psychiatry. (2008) 32:1093–105. doi: 10.1016/j.pnpbp.2007.09.011
17. Dar, NJ, and Ahmad, M. Neurodegenerative diseases and Withania somnifera (L.): an update. J Ethnopharmacol. (2020) 256:112769. doi: 10.1016/j.jep.2020.112769
18. Singh, A, Naidu, PS, Gupta, S, and Kulkarni, SK. Effect of natural and synthetic antioxidants in a mouse model of chronic fatigue syndrome. J Med Food. (2002) 5:211–20. doi: 10.1089/109662002763003366
19. Ramadan, AM, Al-Ghamdi, KM, Alghamdi, AJ, Amer, M, Ibrahim, MIM, and Atef, A. Withania somnifera mitochondrial atp4 gene editing alters the ATP synthase b subunit, independent of salt stress. Saudi J Biolog Sci. (2023) 30:103817. doi: 10.1016/j.sjbs.2023.103817
20. Ambiye, VR, Langade, D, Dongre, S, Aptikar, P, Kulkarni, M, and Dongre, A. Clinical evaluation of the Spermatogenic activity of the root extract of Ashwagandha (Withania somnifera) in Oligospermic males: a pilot study. Evid Based Complement Alternat Med. (2013) 2013:571420:1–6. doi: 10.1155/2013/571420
21. Vaidya, VG, Naik, NN, Ganu, G, Parmar, V, Jagtap, S, Saste, G, et al. Clinical pharmacokinetic evaluation of Withania somnifera (L.) Dunal root extract in healthy human volunteers: a non-randomized, single dose study utilizing UHPLC-MS/MS analysis. J Ethnopharmacol. (2024) 322:117603. doi: 10.1016/j.jep.2023.117603
22. Chandrasekhar, K, Kapoor, J, and Anishetty, S. A prospective, randomized double-blind, placebo-controlled study of safety and efficacy of a high-concentration full-spectrum extract of ashwagandha root in reducing stress and anxiety in adults. Indian J Psychol Med. (2012) 34:255–62. doi: 10.4103/0253-7176.106022
23. Afewerky, HK, Ayodeji, AE, Tiamiyu, BB, Orege, JI, Okeke, ES, Oyejobi, AO, et al. Critical review of the Withania somnifera (L.) Dunal: ethnobotany, pharmacological efficacy, and commercialization significance in Africa. Bull Natl Res Cent. (2021) 45:176. doi: 10.1186/s42269-021-00635-6
24. Bhattacharya, SK, Satyan, KS, and Ghosal, S. Antioxidant activity of glycowithanolides from Withania somnifera. Indian J Exp Biol. (1997) 35:236–9.
25. Bhattacharya, SK, Bhattacharya, D, Sairam, K, and Ghosal, S. Effect of Withania somnifera glycowithanolides on a rat model of tardive dyskinesia. Phytomedicine. (2002) 9:167–70. doi: 10.1078/0944-7113-00089
26. Tiwari, S, Gupta, SK, and Pathak, AK. A double-blind, randomized, placebo-controlled trial on the effect of Ashwagandha (Withania somnifera dunal.) root extract in improving cardiorespiratory endurance and recovery in healthy athletic adults. J Ethnopharmacol. (2021) 272:113929. doi: 10.1016/j.jep.2021.113929
27. Choudhary, B, Shetty, A, and Langade, DG. Efficacy of Ashwagandha (Withania somnifera [L.] Dunal) in improving cardiorespiratory endurance in healthy athletic adults. Ayu. (2015) 36:63–8. doi: 10.4103/0974-8520.169002
28. Samadi Noshahr, Z, Shahraki, MR, Ahmadvand, H, Nourabadi, D, and Nakhaei, A. Protective effects of Withania somnifera root on inflammatory markers and insulin resistance in fructose-fed rats. Reports Biochem Molecular Biol. (2015) 3:62–7.
29. Bucci, LR. Selected herbals and human exercise performance. Am J Clin Nutr. (2000) 72:624s–36s. doi: 10.1093/ajcn/72.2.624S
30. Alam, N, Hossain, M, Khalil, MI, Moniruzzaman, M, Sulaiman, SA, and Gan, SH. High catechin concentrations detected in Withania somnifera (ashwagandha) by high performance liquid chromatography analysis. BMC Complement Altern Med. (2011) 11:65. doi: 10.1186/1472-6882-11-65
31. Vedi, M, Rasool, M, and Sabina, EP. Amelioration of bromobenzene hepatotoxicity by Withania somnifera pretreatment: role of mitochondrial oxidative stress. Toxicol Rep. (2014) 1:629–38. doi: 10.1016/j.toxrep.2014.08.009
32. Gu, C, Zhang, Q, Li, Y, Li, R, Feng, J, Chen, W, et al. The PI3K/AKT pathway-the potential key mechanisms of traditional Chinese medicine for stroke. Front Med. (2022) 9:900809. doi: 10.3389/fmed.2022.900809
33. Zhang, QZ, Guo, YD, Li, HM, Wang, RZ, Guo, SG, and Du, YF. Protection against cerebral infarction by Withaferin a involves inhibition of neuronal apoptosis, activation of PI3K/Akt signaling pathway, and reduced intimal hyperplasia via inhibition of VSMC migration and matrix metalloproteinases. Adv Med Sci. (2017) 62:186–92. doi: 10.1016/j.advms.2016.09.003
34. Yan, Z, Guo, R, Gan, L, Lau, WB, Cao, X, Zhao, J, et al. Withaferin a inhibits apoptosis via activated Akt-mediated inhibition of oxidative stress. Life Sci. (2018) 211:91–101. doi: 10.1016/j.lfs.2018.09.020
35. Guo, R, Gan, L, Lau, WB, Yan, Z, Xie, D, Gao, E, et al. Withaferin a prevents myocardial ischemia/reperfusion injury by upregulating AMP-activated protein kinase-dependent B-cell Lymphoma2 signaling. Circulation J: Official J Japanese Circulation Society. (2019) 83:1726–36. doi: 10.1253/circj.CJ-18-1391
36. Bocci, V, and Valacchi, G. Nrf2 activation as target to implement therapeutic treatments. Front Chem. (2015) 3:4. doi: 10.3389/fchem.2015.00004
37. Cai, J, Xu, L, Tang, H, Yang, Q, Yi, X, Fang, Y, et al. The role of the PTEN/PI3K/Akt pathway on prognosis in epithelial ovarian cancer: a meta-analysis. Oncologist. (2014) 19:528–35. doi: 10.1634/theoncologist.2013-0333
38. Mandlik Ingawale, DS, and Namdeo, AG. Pharmacological evaluation of Ashwagandha highlighting its healthcare claims, safety, and toxicity aspects. J Dietary Supplements. (2021) 18:183–226. doi: 10.1080/19390211.2020.1741484
39. Durg, S, Dhadde, SB, Vandal, R, Shivakumar, BS, and Charan, CS. Withania somnifera (Ashwagandha) in neurobehavioural disorders induced by brain oxidative stress in rodents: a systematic review and meta-analysis. J Pharm Pharmacol. (2015) 67:879–99. doi: 10.1111/jphp.12398
40. Yenisetti, SC, Manjunath, MJ, and Muralidhara, C. Neuropharmacological properties of Withania somnifera - Indian ginseng: an overview on experimental evidence with emphasis on clinical trials and patents. Recent Pat CNS Drug Discov. (2016) 10:204–15. doi: 10.2174/1574889810666160615014106
41. Zahiruddin, S, Basist, P, Parveen, A, Parveen, R, and Khan, W. Ashwagandha in brain disorders: a review of recent developments. J Ethnopharmacol. (2020) 257:112876. doi: 10.1016/j.jep.2020.112876
42. Yaribeygi, H, Panahi, Y, Sahraei, H, Johnston, TP, and Sahebkar, A. The impact of stress on body function: a review. EXCLI J. (2017) 16:1057–72. doi: 10.17179/excli2017-480
43. Patriquin, MA, and Mathew, SJ. The neurobiological mechanisms of generalized anxiety disorder and chronic stress. Chronic stress (Thousand Oaks, Calif). (2017) 1:247054701770399. doi: 10.1177/2470547017703993
44. Yang, L, Zhao, Y, Wang, Y, Liu, L, Zhang, X, Li, B, et al. The effects of psychological stress on depression. Curr Neuropharmacol. (2015) 13:494–504. doi: 10.2174/1570159X1304150831150507
45. Nuss, P. Anxiety disorders and GABA neurotransmission: a disturbance of modulation. Neuropsychiatr Dis Treat. (2015) 11:165–75. doi: 10.2147/NDT.S58841
46. Sonar, VP, Fois, B, Distinto, S, Maccioni, E, Meleddu, R, Cottiglia, F, et al. Ferulic acid esters and Withanolides: in search of Withania somnifera GABA(a) receptor modulators. J Nat Prod. (2019) 82:1250–7. doi: 10.1021/acs.jnatprod.8b01023
47. Mehta, AK, Binkley, P, Gandhi, SS, and Ticku, MK. Pharmacological effects of Withania somnifera root extract on GABAA receptor complex. Indian J Med Res. (1991) 94:312–5.
48. Orrù, A, Marchese, G, Casu, G, Casu, MA, Kasture, S, Cottiglia, F, et al. Withania somnifera root extract prolongs analgesia and suppresses hyperalgesia in mice treated with morphine. Phytomed: Int J Phytotherapy Phytopharmacol. (2014) 21:745–52. doi: 10.1016/j.phymed.2013.10.021
49. Bassareo, V, Talani, G, Frau, R, Porru, S, Rosas, M, Kasture, SB, et al. Inhibition of morphine- and ethanol-mediated stimulation of mesolimbic dopamine neurons by Withania somnifera. Front Neurosci. (2019) 13:545. doi: 10.3389/fnins.2019.00545
50. Kulkarni, SK, Akula, KK, and Dhir, A. Effect of Withania somnifera Dunal root extract against pentylenetetrazol seizure threshold in mice: possible involvement of GABAergic system. Indian J Exp Biol. (2008) 46:465–9.
51. Kulkarni, S, Sharma, AC, Verma, A, and Ticku, MJID. GABA receptor mediated anticonvulsant action of Withania somnifera root extract. COM Scholarly Papers. (1993) 30:305.
52. Kumar, A, and Kalonia, H. Effect of Withania somnifera on sleep-wake cycle in sleep-disturbed rats: possible GABAergic mechanism. Indian J Pharm Sci. (2008) 70:806–10. doi: 10.4103/0250-474X.49130
53. Candelario, M, Cuellar, E, Reyes-Ruiz, JM, Darabedian, N, Feimeng, Z, Miledi, R, et al. Direct evidence for GABAergic activity of Withania somnifera on mammalian ionotropic GABAA and GABAρ receptors. J Ethnopharmacol. (2015) 171:264–72. doi: 10.1016/j.jep.2015.05.058
54. Cobley, JN, Fiorello, ML, and Bailey, DM. 13 reasons why the brain is susceptible to oxidative stress. Redox Biol. (2018) 15:490–503. doi: 10.1016/j.redox.2018.01.008
55. Fedoce, ADG, Ferreira, F, Bota, RG, Bonet-Costa, V, Sun, PY, and Davies, KJA. The role of oxidative stress in anxiety disorder: cause or consequence? Free Radic Res. (2018) 52:737–50. doi: 10.1080/10715762.2018.1475733
56. Salim, S, Chugh, G, and Asghar, M. Inflammation in anxiety. Adv Protein Chem Struct Biol. (2012) 88:1–25. doi: 10.1016/B978-0-12-398314-5.00001-5
57. Bouayed, J, Rammal, H, and Soulimani, R. Oxidative stress and anxiety: relationship and cellular pathways. Oxidative Med Cell Longev. (2009) 2:63–7. doi: 10.4161/oxim.2.2.7944
58. Mohanty, R, Das, SK, Singh, NR, and Patri, M. Withania somnifera leaf extract ameliorates benzo[a]pyrene-induced behavioral and Neuromorphological alterations by improving brain antioxidant status in zebrafish (Danio rerio). Zebrafish. (2016) 13:188–96. doi: 10.1089/zeb.2015.1215
59. Kumar, A, and Kalonia, H. Protective effect of Withania somnifera Dunal on the behavioral and biochemical alterations in sleep-disturbed mice (grid over water suspended method). Indian J Exp Biol. (2007) 45:524–8.
60. Sood, A, Kumar, A, Dhawan, DK, and Sandhir, R. Propensity of Withania somnifera to attenuate Behavioural, biochemical, and histological alterations in experimental model of stroke. Cell Mol Neurobiol. (2016) 36:1123–38. doi: 10.1007/s10571-015-0305-4
61. Kaur, T, Singh, H, Mishra, R, Manchanda, S, Gupta, M, Saini, V, et al. Withania somnifera as a potential anxiolytic and immunomodulatory agent in acute sleep deprived female Wistar rats. Mol Cell Biochem. (2017) 427:91–101. doi: 10.1007/s11010-016-2900-1
62. Gupta, M, and Kaur, G. Withania somnifera as a potential anxiolytic and anti-inflammatory candidate against systemic lipopolysaccharide-induced Neuroinflammation. NeuroMolecular Med. (2018) 20:343–62. doi: 10.1007/s12017-018-8497-7
63. Kaur, T, and Kaur, G. Withania somnifera as a potential candidate to ameliorate high fat diet-induced anxiety and neuroinflammation. J Neuroinflammation. (2017) 14:201. doi: 10.1186/s12974-017-0975-6
64. Panda, V, Deshmukh, A, Hare, A, Singh, S, Hingorani, L, and Sudhamani, S. Effect of Withania somnifera hydroalcoholic extract and other dietary interventions in improving muscle strength in aging rats. J Ayurveda Integr Med. (2021) 12:623–32. doi: 10.1016/j.jaim.2021.06.001
65. White, PT, Subramanian, C, Motiwala, HF, and Cohen, MS. Natural Withanolides in the treatment of chronic diseases. Adv Exp Med Biol. (2016) 928:329–73. doi: 10.1007/978-3-319-41334-1_14
66. Kumar, G, Srivastava, A, Sharma, SK, Rao, TD, and Gupta, YK. Efficacy & safety evaluation of Ayurvedic treatment (Ashwagandha powder & Sidh Makardhwaj) in rheumatoid arthritis patients: a pilot prospective study. Indian J Med Res. (2015) 141:100–6. doi: 10.4103/0971-5916.154510
67. Pawar, P, Gilda, S, Sharma, S, Jagtap, S, Paradkar, A, Mahadik, K, et al. Rectal gel application of Withania somnifera root extract expounds anti-inflammatory and muco-restorative activity in TNBS-induced inflammatory bowel disease. BMC Complement Altern Med. (2011) 11:34. doi: 10.1186/1472-6882-11-34
68. Minhas, U, Minz, R, and Bhatnagar, A. Prophylactic effect of Withania somnifera on inflammation in a non-autoimmune prone murine model of lupus. Drug Discoveries Therapeutics. (2011) 5:195–201. doi: 10.5582/ddt.2011.v5.4.195
69. Sun, GY, Li, R, Cui, J, Hannink, M, Gu, Z, Fritsche, KL, et al. Withania somnifera and its Withanolides attenuate oxidative and inflammatory responses and up-regulate antioxidant responses in BV-2 microglial cells. NeuroMolecular Med. (2016) 18:241–52. doi: 10.1007/s12017-016-8411-0
70. Ajit, D, Simonyi, A, Li, R, Chen, Z, Hannink, M, Fritsche, KL, et al. Phytochemicals and botanical extracts regulate NF-κB and Nrf2/ARE reporter activities in DI TNC1 astrocytes. Neurochem Int. (2016) 97:49–56. doi: 10.1016/j.neuint.2016.05.004
71. Bhat, JA, Akther, T, Najar, RA, Rasool, F, and Hamid, A. Withania somnifera (L.) Dunal (Ashwagandha); current understanding and future prospect as a potential drug candidate. Front Pharmacol. (2022) 13:1029123. doi: 10.3389/fphar.2022.1029123
72. Logie, E, and Vanden, BW. Tackling chronic inflammation with Withanolide phytochemicals-a Withaferin a perspective. Antioxidants (Basel, Switzerland). (2020) 9:1107. doi: 10.3390/antiox9111107
73. Wang, Z, Su, G, Zhang, Z, Dong, H, Wang, Y, Zhao, H, et al. 25-hydroxyl-protopanaxatriol protects against H(2)O(2)-induced H9c2 cardiomyocytes injury via PI3K/Akt pathway and apoptotic protein down-regulation. Biomed Pharmacother. (2018) 99:33–42. doi: 10.1016/j.biopha.2018.01.039
74. Biswas, SK. Does the interdependence between oxidative stress and inflammation explain the antioxidant paradox? Oxidative Med Cell Longev. (2016) 2016:1–9. doi: 10.1155/2016/5698931
75. Kumar, P, Sharma, R, and Garg, N. Withania somnifera - a magic plant targeting multiple pathways in cancer related inflammation. Phytomedicine. (2022) 101:154137. doi: 10.1016/j.phymed.2022.154137
76. Priyanka, G, Anil Kumar, B, Lakshman, M, Manvitha, V, and BJFivs, KK. Adaptogenic and immunomodulatory activity of Ashwagandha root extract: an experimental study in an equine model. Front Vet Sci. (2020) 7:541112. doi: 10.3389/fvets.2020.541112
77. Upendra, R, and Ahmed, MRJIJPR. Healthcare prominence and immune boosting activity of ashwagandha against various clinical conditions and covid 19 disease outbreak. Int J Pharm Res. (2021) 13:489–97. doi: 10.31838/ijpr/2021.13.03.051
78. Ng, QX, Loke, W, Foo, NX, Tan, WJ, Chan, HW, Lim, DY, et al. A systematic review of the clinical use of Withania somnifera (Ashwagandha) to ameliorate cognitive dysfunction. Phytother Res. (2020) 34:583–90. doi: 10.1002/ptr.6552
79. Choudhary, D, Bhattacharyya, S, and Bose, S. Efficacy and safety of Ashwagandha (Withania somnifera (L.) Dunal) root extract in improving memory and cognitive functions. J Diet Suppl. (2017) 14:599–612. doi: 10.1080/19390211.2017.1284970
80. Chengappa, KN, Bowie, CR, Schlicht, PJ, Fleet, D, Brar, JS, and Jindal, R. Randomized placebo-controlled adjunctive study of an extract of withania somnifera for cognitive dysfunction in bipolar disorder. J Clin Psychiatry. (2013) 74:1076–83. doi: 10.4088/JCP.13m08413
81. Bhattacharya, SK, and Muruganandam, AV. Adaptogenic activity of Withania somnifera: an experimental study using a rat model of chronic stress. Pharmacol Biochem Behav. (2003) 75:547–55. doi: 10.1016/S0091-3057(03)00110-2
82. Dela, F, Finkenzeller, T, Ingersen, A, Pötzelsberger, B, and Müller, E. Trajectories of cardio-metabolic health in successful aging. Scand J Med Sci Sports. (2019) 29:44–51. doi: 10.1111/sms.13370
83. Masley, SC, Weaver, W, Peri, G, and Phillips, SE. Efficacy of lifestyle changes in modifying practical markers of wellness and aging. Altern Ther Health Med. (2008) 14:24–9.
84. Brandon, LJ. Physiological factors associated with middle distance running performance. Sports Med. (1995) 19:268–77. doi: 10.2165/00007256-199519040-00004
85. Helgerud, J, Engen, LC, Wisloff, U, and Hoff, J. Aerobic endurance training improves soccer performance. Med Sci Sports Exerc. (2001) 33:1925–31. doi: 10.1097/00005768-200111000-00019
86. McMillan, K, Helgerud, J, Macdonald, R, and Hoff, J. Physiological adaptations to soccer specific endurance training in professional youth soccer players. Br J Sports Med. (2005) 39:273–7. doi: 10.1136/bjsm.2004.012526
87. Myers, J, Prakash, M, Froelicher, V, Do, D, Partington, S, and Atwood, JE. Exercise capacity and mortality among men referred for exercise testing. N Engl J Med. (2002) 346:793–801. doi: 10.1056/NEJMoa011858
88. Rebollo-Ramos, M, Velázquez-Díaz, D, Corral-Pérez, J, Barany-Ruiz, A, Pérez-Bey, A, Fernández-Ponce, C, et al. Aerobic fitness, Mediterranean diet and cardiometabolic risk factors in adults. Endocrinologia, Diabetes Nutricion. (2020) 67:113–21. doi: 10.1016/j.endinu.2019.04.004
89. Flor-Alemany, M, Acosta, P, Marín-Jiménez, N, Baena-García, L, Aranda, P, and Aparicio, VA. Influence of the degree of adherence to the mediterranean diet and its components on cardiometabolic risk during pregnancy. The GESTAFIT project. Nutr Metab Cardiovasc Dis. (2021) 31:2311–8. doi: 10.1016/j.numecd.2021.04.019
90. Gálvez Casas, A, Rodríguez García, PL, García-Cantó, E, Rosa Guillamón, A, Pérez-Soto, JJ, Tarraga Marcos, L, et al. Aerobic capacity and quality of life in school children from 8 to 12. Clin Investig Arterioscler. (2015) 27:239–45. doi: 10.1016/j.arteri.2015.01.001
91. Shenoy, S, Chaskar, U, Sandhu, JS, and Paadhi, MM. Effects of eight-week supplementation of Ashwagandha on cardiorespiratory endurance in elite Indian cyclists. J Ayurveda Integrative Med. (2012) 3:209–14. doi: 10.4103/0975-9476.104444
92. Shenoy, S, Bhaskaran, UC, Sandhu, JS, and Paadhi, M. The effect of Ashwagandha (Withania Somnifera) on anaerobic performance on elite Indian cyclist. Medicina Sportiva: J Romanian Sports Med Society. (2012) 8:1909.
93. Wankhede, S, Langade, D, Joshi, K, Sinha, SR, and Bhattacharyya, S. Examining the effect of Withania somnifera supplementation on muscle strength and recovery: a randomized controlled trial. J Int Soc Sports Nutr. (2015) 12:1–11. doi: 10.1186/s12970-015-0104-9
94. Sandhu, JS, Shah, B, Shenoy, S, Chauhan, S, Lavekar, G, and Padhi, M. Effects of Withania somnifera (Ashwagandha) and Terminalia arjuna (Arjuna) on physical performance and cardiorespiratory endurance in healthy young adults. Int J Ayurveda Res. (2010) 1:144–9. doi: 10.4103/0974-7788.72485
95. Mehta, V. Effect of ASHWAGANDHA (WITHANIA SOMNIFERA) root powder supplementation on agility level of players: a CASE study of hockey players. EPRA Int J Multidis Res (IJMR). (2022) 8:292–5. doi: 10.36713/epra11282
96. Malik, A, Mehta, V, and Dahiya, V. Effect of Ashwagandha (Withania somnifera) root powder supplementation on the VO2 max. And hemoglobin in hockey players. Int J Behav Soc Movement Sci. (2013) 2:91–9.
97. Malik, A, Mehta, V, Malik, S, and Sharma, P. Effect of Ashwagandha (Withania somnifera) root powder supplementation on the core muscle strength and stability in hockey players. Int J Behav Soc Movement Sci. (2014) 3:83–91.
98. Verma, N, Gupta, SK, Patil, S, Tiwari, S, and Mishra, AK. Effects of Ashwagandha (Withania somnifera) standardized root extract on physical endurance and VO 2max in healthy adults performing resistance training: an eight-week, prospective, randomized, double-blind, placebo-controlled study. F1000Research. (2024) 12:335. doi: 10.12688/f1000research.130932.2
99. Yadav, S. Effect of ashwagandha (Withania Somnifera) consumption on the selected physical fitness variables of male sprinters. Turkish J Sport Exerc. (2014) 16:45–7. doi: 10.15314/TJSE.201439613
100. Chaskar, U, Shenoy, S, Sandhu, JS, and Paadhi, MM. Gender differences in responsiveness to Ashwagandha supplementation on physical performance of elite Indian cyclists. Contemporary trends and research in sports, exercise and physical education. Proceedings of the 9th International Sports Science Conference Kota Bharu, Kelantan, Malaysia. 25-28th June 2012. (2013).
101. Sukumar, BS, Tripathy, T, Shashirekha, H, and Shetty, K. Efficacy of Ashwagandha (withania somnifera (L.) Dunal) in improving cardiorespiratory endurance (VO2 max test) in healthy subjects. Int J Res Pharmaceutical Sci. (2021) 12:911–8.
102. Ziegenfuss, TN, Kedia, AW, Sandrock, JE, Raub, BJ, Kerksick, CM, and Lopez, HL. Effects of an aqueous extract of Withania somnifera on strength training adaptations and recovery: the STAR trial. Nutrients. (2018) 10:1807. doi: 10.3390/nu10111807
Keywords: ashwagandha, sports performance, brain function, antioxidant response, exercise, inflammation
Citation: Guo S and Rezaei MJ (2024) The benefits of ashwagandha (Withania somnifera) supplements on brain function and sports performance. Front. Nutr. 11:1439294. doi: 10.3389/fnut.2024.1439294
Edited by:
Baoming Tian, Zhejiang University of Technology, ChinaReviewed by:
Shawn M. Talbott, Amare Global, United StatesRauf Ahmad Najar, University of Rochester, United States
Copyright © 2024 Guo and Rezaei. This is an open-access article distributed under the terms of the Creative Commons Attribution License (CC BY). The use, distribution or reproduction in other forums is permitted, provided the original author(s) and the copyright owner(s) are credited and that the original publication in this journal is cited, in accordance with accepted academic practice. No use, distribution or reproduction is permitted which does not comply with these terms.
*Correspondence: Shiyi Guo, MonkeyGuooo@163.com; Mohammad J. Rezaei, rezaei.mh11@gmail.com