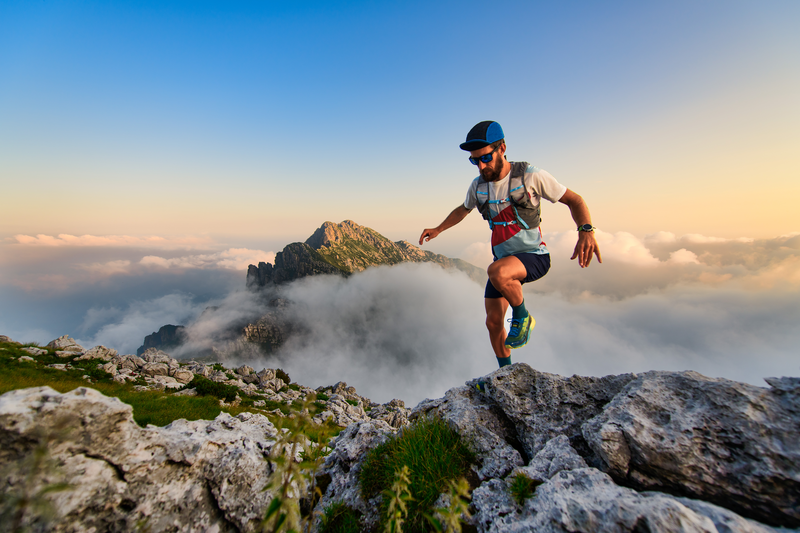
94% of researchers rate our articles as excellent or good
Learn more about the work of our research integrity team to safeguard the quality of each article we publish.
Find out more
SYSTEMATIC REVIEW article
Front. Nutr. , 01 October 2024
Sec. Nutrition and Metabolism
Volume 11 - 2024 | https://doi.org/10.3389/fnut.2024.1437681
This article is part of the Research Topic Targeting Metabolic Disorders by Trace Elements and Minerals: New Insights and Strategies View all 3 articles
Aim: This study aims to assess the dose–response impact of iron load on systemic and hepatic metabolic disorders including metabolic syndrome (MetS) and non-alcoholic fatty liver disease (NAFLD).
Methods: Serum ferritin (SF) and dietary iron intake were selected to represent the indicators of iron load in the general population. PubMed, EMBASE and Web of Science databases were searched for epidemiological studies assessing the impact of SF/dietary iron intake on MetS/NAFLD occurrence. All literature was published before September 1st, 2023 with no language restrictions.
Results: Fifteen and 11 papers were collected with a focus on connections between SF and MetS/NAFLD, respectively. Eight papers focusing on dietary iron and MetS were included in the following meta-analysis. For the impact of SF on MetS, the pooled odds ratio (OR) of MetS was 1.88 (95% CI: 1.58–2.24) for the highest versus lowest SF categories. In males, the OR was 1.15 (95% CI: 1.10–1.21) per incremental increase in SF of 50 μg/L, while for females, each 50 μg/L increase in SF was associated with a 1.50-fold higher risk of MetS (95% CI: 1.15–1.94). For connections between SF and NAFLD, we found higher SF levels were observed in NAFLD patients compared to the control group [standardized mean difference (SMD) 0.71; 95% CI: 0.27–1.15], NASH patients against control group (SMD1.05; 95% CI:0.44–1.66), NASH patients against the NAFLD group (SMD 0.6; 95% CI: 0.31–1.00), each 50 μg/L increase in SF was associated with a 1.08-fold higher risk of NAFLD (95% CI: 1.07–1.10). For the impact of dietary iron on MetS, Pooled OR of MetS was 1.34 (95% CI: 1.10–1.63) for the highest versus lowest dietary iron categories.
Conclusion: Elevated SF levels is a linear relation between the incidence of MetS/NAFLD. In addition, there is a positive association between dietary iron intake and metabolic syndrome. The association between serum ferritin and metabolic syndrome may be confounded by body mass index and C-reactive protein levels.
Metabolic syndrome (MetS) affects at least one-fourth of the global adult population (1, 2), presenting a complex array of clinical features including abdominal obesity [waist circumference (WC) >90 cm for men, WC > 80 cm for women], dyslipidemia [triglyceride (TG) >150 mg/dL], hyperglycemia [fasting blood glucose (FBG) ≥ 100 mg/dL or medication for anti-hyperglycemia], and hypertension [systolic blood pressure (SBP) ≥ 130 mmHg and/or diastolic blood pressure (DBP) ≥ 85 mmHg or the medication of anti-hypertension] (3). MetS surpasses its benign nature, significantly amplifying the risk of CVD and T2DM events by approximately two-and six-fold, respectively (4, 5).
Non-alcoholic fatty liver disease (NAFLD) stands as the most prevalent chronic liver ailment worldwide, with a prevalence of 25.24% among the general population (6). Often intertwined with insulin resistance (IR) and MetS, NAFLD commonly coexists with type 2 diabetes mellitus (T2DM), dyslipidemia, obesity, and hypertension (7).
Serum ferritin (SF), a ubiquitous intracellular protein pivotal in regulating iron homeostasis, serves as an established biomarker for assessing body iron stores (8). Nevertheless, mounting evidence suggests that elevated body iron stores may correlate with adverse health outcomes.
Iron is an essential trace element for the human body. Its sources can be divided into heme iron food sources, such as liver, animal blood, beef, lean meat, and fish, and non-heme iron food sources, such as spinach, beans, pumpkin seeds, and beetroots (9). It plays a crucial role in numerous cellular processes including signaling, respiration, DNA replication and synthesis, nucleic acid repair, and energy metabolism (10). The consumption, uptake, transfer, and storage of iron are important for maintaining iron balance in the body (11). However, excessive levels of iron can lead to inflammation and tissue damage by generating harmful hydroxyl radicals through oxidative reactions. These radicals cause oxidative damage to important cellular components like lipids, proteins, and DNA (12). Considering that oxidative stress and inflammation contribute significantly to the development of Metabolic Syndrome (MetS), there exists a close association between dietary iron levels and MetS (13).
Considering the close association between markers of iron metabolism and metabolic derangements, numerous studies have investigated the relationships between SF/dietary iron and the occurrence of MetS/NAFLD. Moreover, past studies have explored the effects of SF/dietary iron on metabolic diseases (14–19). However, inconsistent and controversial findings suggest that potential confounding factors may influence the predictive role of SF/dietary iron in monitoring MetS and NAFLD. We have experience on quantitative evaluation of risk covariates on specific disease (20–22). Therefore, we conducted a systematic review and meta-analysis to quantitatively assess the trend of MetS/NAFLD incidence associated with SF/dietary iron variations based on published literature (23–56). Subgroup analysis was employed to explore latent confounders. Therefore, we conducted a systematic review and meta-analysis to quantitatively assess the trend of MetS/NAFLD incidence associated with SF/dietary iron variations based on published literature (23–56). Subgroup analysis was employed to explore latent confounders. Comprehensive dose–response analyses are warranted to elucidate the effects of quantitative SF/dietary iron variables on the persistent risk of metabolic diseases.
We conducted a systematic evaluation and meta-analysis following the guidelines of Preferred Reporting Entries for Systematic Evaluation and Meta-Analysis (PRISMA) (refer to Supplementary material). A thorough search was performed in Medline, Embase, and Web of Science (WOS) databases from the time of establishment until February 10, 2023 (without any language limitations). We utilized various terms such as “Metabolic syndrome,” “MetS,” “Non-alcoholic fatty liver disease,” “non-alcoholic Steatohepatitis,” “NAFLD,” “NASH,” “Iron,” “Fe,” “Serum ferritin” and “SF” to retrieve relevant literature. In case any pertinent studies were missed during the initial search, we also manually retrieved additional references. The detailed database search strategy can be found in Supplementary Table S1.
The study inclusion criteria for this meta-analysis were (1) observational studies examining the association between SF/iron levels and MetS/NAFLD; (2) studies that directly provided effect sizes (corrected for age, gender, smoking, alcohol consumption, family history, hs-CRP, BMI, etc.), including risk ratios (HR), odds ratios (OR), standardized mean differences (SMD), 95% confidence intervals (CI), or had enough data to calculate these metrics; (3) studies published in English. Studies that met the following exclusion criteria were not included in the meta-analysis: (1) reviews or other types of non-original studies; (2) non-breeding trials; (3) studies that used pregnant women as the study population; (4) had other complications that could affect SF/iron detection; (5) incomplete information or inability to obtain the necessary information by contacting the corresponding authors; and (6) duplicate study populations. In the case of multiple studies on the same population, only one data set containing more individuals or more comprehensive data was included, depending on the specific analytic needs.
Two investigators independently assessed the quality of each study, and any disagreements were resolved by a third author. The included studies were cross-sectional and were evaluated using the American Association for Healthcare Research and Quality (AHRQ) scale (57). The evaluation tool includes 11 entries for the recommended criteria. A score of 1 was assigned to “yes” and 0 to “no” or “unclear.” 0 to 3 was categorized as a low-quality study, 4–7 as a moderate-quality study, and 8–11 as a high-quality study.
Two investigators (LY and YZ) independently collected data from specific eligible articles. The information recorded included study design (prospective cohort, cross-sectional, and other designs), association metrics used (ratio or risk ratio), country of origin, population, sex, mean participant age, number of participants, ferritin assay technique, ferritin levels, metabolic syndrome criteria and outcomes, histologic extent of NAFLD (if any), methods of NAFLD assessment, and additional information (categories of NAFLD, casel/control number).
Initially, we combined the results of the included studies using a random-effects model (inverse variance) of selected ratio ratios (for cross-sectional studies) and standardized mean differences (for prospective cohort studies), as well as 95% confidence intervals, to quantify the associations between SF and MetS, dietary iron and MetS, and SF and NAFLD. If significant heterogeneity existed, a random effects model was used and heterogeneity was assessed using the I2 statistic (low, high, and medium heterogeneity defined as 25, 50, and 75%, respectively) (58). First, a meta-analysis was conducted on the highest quartile/third quartile/quartile/quintile versus the lowest or reference quartile. Heterogeneity between studies was then assessed using the I2 statistic. If no statistically significant heterogeneity was found (I2 < 50% and p > 0.10), the Mantel–Haenszel fixed-effects model would be applied (59). Otherwise, the DerSimonian-Laird random effects model will be used (60). We performed subgroup analyses to explore potential sources of variation, such as geographic region (East-Asian and Non-East-Asian), diagnostic criteria for metabolic syndrome [International Diabetes Foundation (IDF), Adult Treatment Panel (ATP) III, and Others], ferritin assay (chemiluminescence, immunoradiometric, immunoturbidimetric, not reported), study design (case–control, and cross-sectional and prospective study) and so on. Sensitivity analyses were performed using a systematic exclusion of 1 study at a time to assess its effect on the overall effect size.
We used the method developed by Greenland and Longnecker (61), which extracts the following data from studies that provide information on at least 3 quantitative exposure categories: SF/dietary level, number of cases and participants, OR, and 95% CI. Based on the data obtained, the median or mean SF level was assigned to each category. Where median or mean values were not provided for each category, we used the midpoint estimate between the upper and lower bounds. If the highest category had an open range, we assumed it was the same width as the second-highest category (62). To assess potential linear or nonlinear associations between SF and MetS, Dietary iron and MetS, and SF and NAFLD, we used restricted cubic spline with four nodes located at the 5, 35, 65, and 95% fixed percentiles of the distribution. In the absence of nonlinear associations observed in the dose–response analyses, we performed a meta-analysis focusing exclusively on linear trends to examine the association between each 50 μg/L increase in SF and the risk of MetS/NAFLD across sexes. Egger’s test and Begg’s test (63) were used to assess publication bias. Stata 14.0 software was used for statistical analysis. A p < 0.05 was considered statistically significant unless explicitly stated otherwise. Our study flow diagram was shown in Supplementary Figure S1.
We conducted a comprehensive screening of 5,067 publications that may be relevant to this study. After removing 1,566 duplicates from three databases (PubMed, Embase, and WOS), we finalized 34 eligible articles. These included 15 articles on the relationship between SF-MetS, 11 articles on SF-NAFLD, and eight articles on dietary iron-MetS. Inter-rater agreement was high (Cohen’s kappa = 0.766). See Figure 1 for a flowchart of our literature selection process.
Figure 1. Flow diagram of eligible literature selection. (A) Literature on the association between SF and MetS; (B) literature on the association between SF and NAFLD; (C) literature on the association between Dietary and MetS. SF, serum ferritin; MetS, metabolic syndrome; NAFLD, non-alcoholic fatty liver disease.
All included studies were of high quality according to the AHRQ evaluation system. The AHRQ scores ranged from 7 to 11 with a mean of 8.17 points. For more details on the quality assessment, see Supplementary Table S3.
Table 1 summarizes the characteristics of the included studies. In our meta-analysis, we analyzed data from 15 cross-sectional studies (23–37) examining the relationship between SF and MetS. These studies involved a total sample size of 89,205 individuals and were conducted before 2003 in China, Korea, the United States, Croatia, Europe, and Spain, with participants aged between 18 and 80 years.
Regarding the diagnostic criteria for MetS: eight studies used the International Diabetes Federation (ATPIII) guidelines; four referenced the modified National Cholesterol Education Program Adult Treatment Panel III (NCEP ATP III) guidelines; and two referenced the International Diabetes Federation (IDF) guidelines. In these studies, different methods were used to determine SF levels: chemiluminescence in six cases; immunoradiation in five cases; immunoturbidimetry in three cases; and electrochemiluminescence immunoassay in one case. For statistical analysis models: 11 results were calculated using multifactorial logistic regression models; three using multiple linear regression models; and one group using binary logistic regression models.
A total of 11 articles (38–46, 48, 64) were included in this study, focusing on the relationship between SF and NAFLD. Among these articles, two were prospective cohort studies, six were case-sectional studies, and three were cross-sectional studies. The diagnosis of NAFLD (NASH) was confirmed using hepatic ultrasonography in two studies and liver biopsy in nine studies. Geographically, two studies were conducted in Europe, eight in Asia, and one in America. The cumulative number of participants across all studies reached a substantial count of 8,302 individuals.
Eight articles (49–56) were selected to investigate the association between dietary iron intake and MetS. The diagnostic criteria for MetS varied among the included articles; five used NCEP ATP III criteria while two employed IDF criteria and one utilized American Heart Association (AHA) criteria. Out of these eight articles, six adopted a cross-sectional design while the remaining two followed a cohort design approach. Dietary iron levels were assessed through either 24-h or 3-day recall methods across all included studies with sample sizes ranging from 284 to 5,322 individuals resulting in a combined total sample size of 22,061 participants. Age, gender, and MetS components were corrected as key covariates in all studies.
The combined OR of the 15 included papers was 1.88 (95% CI: 1.58–2.44) with low heterogeneity (p < 0.001; I2 = 68.5%; Figure 2A). The pooled OR for men was 1.96 (95% CI: 1.56, 2.47); heterogeneity: p < 0.001; I2 = 74.8%; for premenopausal women it was 1.64 (95% CI: 1.06, 2.54); heterogeneity: p = 0.104; I2 = 51.4% and for postmenopausal women it was 1.84 (95% CI: 1.42, 2.38); heterogeneity: p = 0.267; I2 = 24.1%.
Figure 2. Forest plot of association between SF and MetS in cross-sectional studies. Pooled odds ratios of MetS compared between highest and lowest SF categories. SF, serum ferritin; MetS, metabolic syndrome.
Due to the limited literature on dose–response meta-analysis, only four papers were included (24, 26, 30, 31). A linear dose–response relationship between SF and MetS across genders is shown in Figures 3A,B, with a linear dose–response relationship between the two populations (male: p < 0.05, P-nonlinearity = 0.0516, female: p < 0.05, P-nonlinearity = 0.9269).
Figure 3. Egger’s funnel plot analysis of publication bias (A) between SF and MetS, Egger’s test: p = 0.561. (B) Between NASH/NAFLD and control, Egger’s test: p = 0.104. (C) Between NASH VS NAFLD, Egger’s test: p = 0.417. (D) Between Dietary Iron and MetS, Egger’s test: p = 0.560. SF, serum ferritin; MetS, metabolic syndrome; NAFLD, non-alcoholic fatty liver disease; NASH, non-alcoholic steatohepatitis.
For every 50 μg/L increase in SF in men, the risk of MetS increased 1.15-fold (95% CI: 1.10–1.21). In women, a 50 μg/L increase in SF was associated with a 1.50-fold increase in the risk of MetS (95% CI: 1.15–1.94).
Because of the large variability across the study, we sought to assess possible causes of heterogeneity through subgroup analysis. Subgroup analyses were categorized according to sample size, geographic region, and ferritin measurements and corrected for BMI, CRP, HOMA-IR, ALT, and metabolic syndrome criteria.
Nevertheless, significant differences were found in subgroups of ferritin measurements corrected for CPR and BMI. The correlation between ferritin levels and metabolic syndrome was stronger in studies corrected for CRP [OR = 1.90 (95% CI: 1.41–2.56; I2 = 82.4%)] versus studies not corrected for CRP [OR = 1.63 (95% CI: 1.47–1.81; I2 = 24.8%)]. In addition, studies not corrected for BMI showed a stronger correlation between serum ferritin levels and metabolic syndrome compared with studies corrected for BMI (OR = 1.93 vs. OR = 1.60). There was no significant difference in the other subgroups, suggesting that SF can increase the risk of MetS (Table 2).
A sensitivity analysis was conducted to investigate the sources of heterogeneity. By systematically excluding one study at a time and recalculating the pooled odds ratios for the remaining studies, it was observed that removing Li′s study eliminated the heterogeneity (as depicted in Figure 4A). This observation can be attributed to the larger sample size of Li′s study compared to other studies.
Figure 4. Forest plot of the association between Dietary Iron and MetS in cross-sectional studies. Pooled odds ratios of MetS compared between highest and lowest Dietary Iron categories. MetS, metabolic syndrome.
The log odds ratio’s standard error (SE) for each study was compared to the OR on Egger’s funnel plot (Figure 5A) for visual inspection. Despite slight asymmetry observed in the funnel plot, Egger’s test did not detect any indication of publication bias (p = 0.132).
Figure 5. Sensitivity analyses of the association between SF/Dietary Iron and MetS /NAFLD. (A) Represents eliminated heterogeneity excluding a study of association between SF and MetS in cross-sectional studies. (B,C) Represent eliminated heterogeneity excluding studies of association between SF and NAFLD in cross-sectional studies. (D) Represent eliminated heterogeneity excluding studies of association between Dietary and MetS in cross-sectional studies. SF, serum ferritin; NAFLD, non-alcoholic fatty liver disease; MetS, metabolic syndrome.
SF was significantly higher in (1) NAFLD patients compared with the control group; (2) NASH patients compared with the control group and (3) NASH patients compared with NAFLD patients. In all cases of juxtaposition, the differences between studies were severe (I2 ranged from 81.5 to 96.8%; Figures 6A,B). No meaningful bias was observed in any of the comparisons (p > 0.05 for all comparisons; Figure 4).
Figure 6. Forest plot of association between SF and NAFLD in cross-sectional studies. (A) NAFLD/NASH patients against the control group; (B) NASH patients against NAFLD against SF, serum ferritin; NAFLD, non-alcoholic fatty liver disease; NASH, non-alcoholic steatohepatitis.
Combined analysis of baseline SF levels and risk of NAFLD incidence in study subjects of different genders using different models revealed a dose–response relationship between baseline SF levels and risk of NAFLD incidence. There was a non-significant non-linear relationship between SF variants and NAFLD incidence (p = 0.2011 Figure 7B). Linear trend test (p = 0.041 < 0.05).
Figure 7. Dose–response relations between SF and risk of MetS/NAFLD in prospective studies. (A) Risk between SF and MetS in men estimates from a dose–response meta-analysis. (B) Risk between SF and MetS in women estimates from the dose–response meta-analysis. (C) Risk between SF and NAFLD estimates from the dose–response meta-analysis. SF, serum ferritin; MetS, metabolic syndrome; NAFLD, non-alcoholic fatty liver disease.
Continuous SMD values for NAFLD incidence in 2 studies were either raw data or extracted by calculation (44, 65). For every 50 μg/L increase in SF, there was a 1.08-fold increase in the risk of NAFLD (95% CI: 1.07–1.10).
Subgroup analyses of the ferritin and NAFLD are shown in Table 3. Stratified analyses were classified by ethnicity, sample size, study design, mean age, NAFLD diagnosis approach/tool, and calculation method.
However, there were significant differences in the subgroups of ethnicity, sample size, NAFLD diagnosis approach/tool, calculation method, and study design on NAFLD/NASH and control groups. In the NASH and NAFLD groups, there were no statistically significant differences between subgroups for each of the potential confounders (p > 0.05 for comparisons between subgroups).
In the sensitivity analysis, the exclusion of individual studies did not significantly change the estimates, and the combined RR ranged from 0.33 to 1.29. Egger’s test was used to assess publication bias. No significant publication bias was observed (Egger’s p = 0.104, 0.417, Figures 3B,C).
The multifactorial adjusted overall RR value showed that dietary iron levels were positively associated with MetS (RR = 1.34, 95% CI: 1.10–1.63; p < 0.001) (Figure 4). There was no significant heterogeneity between studies (p = 0.065, I2 = 47.4%).
See Figure 5 for subgroup analyses of dietary iron and MetS. Stratified analyses were categorized according to ethnicity, sample size, adjusted for BMI, study design, mean age, diagnostic criteria of MetS, and exposure assessment. However, differences were statistically significant in racial subgroups corrected for BMI, MetS diagnostic criteria, and exposure assessment. These results were confirmed in the East-Asian (OR = 1.34, 95% CI: 1.21–1.62; p = 0.038), no adjusted for BMI (OR = 1.57, 95% CI: 1.23–2.00; p = 0.01), the NCEP ATPIII/IDF (OR = 1.59, 95% CI: 1.30–1.93; p = 0.002) and 24 h or 3-day recall method (OR = 1.31, 95% CI: 1.07–1.59; p = 0.009) in Table 4.
In the sensitivity analysis, individual studies were excluded that did not significantly change the estimates, and the combined OR was 1.10–1.63. Egger’s test was used to assess publication bias. No significant publication bias was observed (Egger’s p = 0.560, Figure 3D).
This meta-analysis aimed to conduct a comprehensive examination of all available data on the association of SF with MetS/NAFLD and the association of dietary iron with MetS and to integrate this information to draw conclusive conclusions about this possible association. There were three primary endpoints of this trial. First, there was a complex association between SF and MetS. We obtained some new results supporting the primary outcome after subgroup analyses, meta-regression analyses, and dose–response analyses. There was a linear dose–response relationship between SF and the prevalence of MetS over a range of values. Each 50 μg/L increase in body SF was associated with a 15% (95% CI: 1.10–1.21) increase in prevalence risk in men and a 50% (95% CI: 1.15–1.94) increase in prevalence risk in women. It was concluded that the risk of metabolic syndrome differed between genders. Second, SF levels were significantly associated with NAFLD. SF levels may increase the incidence of NAFLD. For every 50 μg/L increase in body SF, the incidence of NAFLD increased by approximately 8% (95% CI: 1.07–1.10). Third, the pooled results showed that dietary iron levels were positively associated with MetS.
MetS were proved to be associated with variations on indicators like alanine aminotransferase, uric acid and adiponectin (20, 66, 67). With regard to iron metabolism, it has long been postulated that hyperferritinemia plays a causal role and possesses predictive value in the development of MetS due to its ability to induce inflammation and reactive oxidative stress (ROS). Our prior omics study also found the disturbed iron metabolism had interaction with impaired anti-oxidative capacity (68). Serum ferritin functions as both a signaling molecule and a direct mediator of the immune system (69). Ferritin has been demonstrated to regulate an iron-independent signaling pathway, leading to NF-κB activation and subsequent release of proinflammatory molecules. Chronic inflammation is widely recognized as one of the primary mechanisms underlying MetS (70). Cai and Liu (71) proposed that NF-κB activation serves as the core molecular basis for the pathological process of MetS. Iron, being a transition metal, catalyzes free radical formation which causes oxidative damage in cells and tissues (72). Oxidative stress disrupts the balance between oxidants and antioxidants, resulting in aberrant intracellular signal transmission and gene expression changes. This can contribute to pathological conditions such as insulin resistance (73). Therefore, it has been suggested that ferritin-mediated inflammation and oxidative stress are responsible for linking MetS with Type-2 Diabetes Mellitus (DM) and Cardiovascular Disease (CVD) (74).
To investigate the impact of gender on SF levels and the risk of developing MetS, we observed a slightly lower increased risk of MetS per 1-level increase in SF among men compared to women. Previous studies have highlighted significant differences between women and men regarding insulin action, susceptibility to insulin resistance development, and response to factors influencing insulin sensitivity (75). Females inherently exhibit higher levels of insulin resistance than males, potentially attributed to sex-specific variations in gene expression and metabolic control elements (e.g., signaling pathways, substrate shuttling elements, receptors) (75). Additionally, sex hormones along with environmental and lifestyle factors that either exacerbate or mitigate the genetic predisposition in women may also possess a genetic basis (76). Punnonen et al. (77) investigated the cardiovascular disease (CVD) risk following premenopausal hysterectomy or myomectomy and found that normal uterine function as well as blood loss and iron depletion were essential for maintaining cardiovascular protection in women. However, it is possible that the actual CVD risk in women might not be as high as anticipated. Nevertheless, subgroup analyses were conducted to demonstrate increased heterogeneity based on BMI and CRP levels. After adjusting for BMI, a well-established anthropometric predictor of cardiovascular metabolic disease (CMD) that is positively associated with iron stores, the pooled ferritin-MetS correlations in all evaluated studies were attenuated (78). Fibromodulin is a small leucine-rich proteoglycan that plays a role in the regulation of collagen fibril assembly and has been implicated in various physiological processes, including tissue repair and tumor suppression (79). Adipocytokines have been found to stimulate the synthesis and secretion of fibromodulin hormone, which inhibits intestinal iron absorption and tissue iron release, ultimately leading to iron deficiency. Similarly, obesity-related low-grade inflammation can result in elevated ferritin levels even in the presence of iron deficiency (80). The mechanisms by which insulin resistance affects iron homeostasis explain the association between obesity and excess iron accumulation (81). Future studies should consider incorporating BMI correction when assessing confounders, effect modification, and potential mechanisms. CRP serves as an acute-phase reactant and nonspecific marker of inflammation that has proven utility in predicting future development of cardiovascular disease (82). Recent research has also demonstrated elevated CRP levels in patients with MetS and its predictive value for MetS development (83).
The liver disorders, known as non-alcoholic fatty liver disease (NAFLD), encompass a spectrum ranging from mild hepatic steatosis to non-alcoholic steatohepatitis (NASH) (84). NAFLD is recognized as a metabolic disorder in liver with major impact on hepatic and biliary system (85, 86). Unlike MetS, limited research has been conducted on the longitudinal risk of ferritin about the variability of NAFLD. Our findings indicate that elevated levels of serum ferritin may be linked to the severity of NAFLD, as controls exhibited lower levels compared to patients with NASH or NAFLD, and NAFLD patients had lower levels than NASH patients. Sensitivity analyses and subgroup analyses did not alter these results significantly. Therefore, serum ferritin could potentially serve as a less invasive and more effective biomarker for predicting the progression of NAFLD. Moreover, further insights from age-, sex-, and race-specific longitudinal studies are necessary to fully elucidate the association between serum ferritin and NAFLD.
The pathophysiological mechanisms underlying elevated serum ferritin levels in patients with NAFLD have been investigated by researchers. Hyperferritinemia (HFE) in NAFLD patients is often associated with lipocalin, a marker of insulin resistance and hepatic inflammation (87). Lipocalin-2 (LCN2) is involved in the transport and storage of iron. In NAFLD, disruptions in iron metabolism can lead to iron accumulation in the liver, promoting oxidative stress and hepatocyte injury (88). Alterations in serum iron levels among adults with NAFLD are typically characterized by increased serum ferritin levels and normal transferrin saturation, which is referred to as metabolic abnormal iron overload syndrome (89). Furthermore, serum ferritin levels in NAFLD patients are closely linked to the iron-regulating hormone hepcidin and hepatic iron concentrations (90). In male obese adolescents, elevated serum ferritin levels primarily result from hepatic fat content and inflammation rather than body iron status (91). Elevated serum ferritin levels in NAFLD patients are associated with abnormal markers of iron metabolism or inflammation due partly to β-cell dysfunction and insulin resistance (92). In 2001, Manousou et al. (93) found that hyperferritinemia with normal transferrin saturation indicates glucose/lipid metabolism disorders; when combined with various metabolic abnormalities and iron overload, it identifies individuals at risk for NASH. Bugianesi et al. (94) demonstrated that elevated ferritin levels serve as an indicator of severe histological damage rather than iron overload, and the contribution of iron loading and HFE mutations to hepatic fibrosis in most patients with non-alcoholic fatty liver disease (NAFLD) is not significant. In contrast, Angulo et al. (95) found that although serum ferritin levels were associated with more severe hepatic fibrosis, the results of ferritin assays did not provide a satisfactory classification for fibrosis or improve the accuracy of noninvasive scoring when including serum ferritin. Through corrected multivariate logistic regression analysis, they concluded that serum ferritin levels alone exhibited low diagnostic accuracy for detecting the presence or severity of liver fibrosis in NAFLD patients.
In our research, we have identified a positive correlation between dietary iron consumption (both total iron and hemoglobin) and various components of MetS. Our analysis indicates that higher intakes of dietary iron, particularly from non-heme sources, are associated with a modest increase in the risk of developing MetS. This relationship remains significant after adjusting for potential confounders such as age, gender, physical activity levels, and overall dietary patterns. Considering the pivotal role of dietary iron intake in determining body iron stores, it is plausible to propose that higher levels of iron intake may be associated with an increased risk of developing MetS. Humans obtain iron from their diet in two forms: non-heme and heme iron (96). It is widely recognized that the absorption of heme iron remains relatively unaffected by other dietary factors compared to non-heme iron absorption. Several dietary elements, including heme iron, supplemental iron, Vitamin C from food sources, animal protein, copper, and alcohol exhibited significant positive associations with diabetes. Conversely, coffee consumption along with phytate, oxalate, zinc, and calcium intake demonstrated significant negative associations with the occurrence of diabetes (97, 98). Our findings have practical implications for individuals managing MetS daily. For instance, there should be an emphasis on providing comprehensive dietary education specifically tailored for individuals with metabolic syndrome which includes guidance on avoiding excessive intake of dietary sources rich in iron.
The mention of the robustness and applicability of our findings is essential. All literature included in this study met the predefined inclusion criteria. The selected studies collectively demonstrated a high level of quality, establishing a strong basis for evaluating their relevance. SF displayed an approximately linear dose–response correlation, thereby serving as a valuable biomarker for cost-effective prediction and screening of metabolic disorders. Considering the potential causal relationship between hyperferritinemia and metabolic abnormalities, reducing ferritin levels may emerge as a promising therapeutic target in preventing conditions such as metabolic syndrome or NAFLD, which are prevalent risk factors for serious diseases like CVD.
The findings of the meta-analysis were robust; however, certain limitations should be considered in this study. The evaluation primarily relied on cross-sectional studies due to the absence of randomized controlled trials and longitudinal studies. Future research should aim to validate the association between SF-Mets in other ethnicities, as most prospective studies focused on East Asian populations. Combining the results from different studies posed a challenge due to inconsistencies in risk indicators and statistical methods used. To enhance comparability, HR values were converted into OR values before data integration. Additionally, variations in diagnostic criteria for MetS among the included studies may introduce bias when distinguishing patients from non-patients (Supplementary Table S4).
In summary, a meta-analysis of prospective studies has consistently demonstrated a direct relationship between elevated ferritin levels and the incidence of MetS/NAFLD. Moreover, there is a positive correlation between dietary iron intake and MetS. The association between ferritin and MetS may be confounded by BMI and CRP levels. Incorporating ferritin alongside other metabolic and biochemical parameters could improve the accuracy of non-invasive scoring systems for NAFLD. Additionally, targeting iron depletion remains an attractive therapeutic strategy to prevent liver damage progression.
The original contributions presented in the study are included in the article/Supplementary material, further inquiries can be directed to the corresponding author.
LY: Writing – original draft, Writing – review & editing. TQ: Writing – review & editing. YZ: Data curation, Investigation, Writing – review & editing. ZL: Conceptualization, Funding acquisition, Supervision, Writing – review & editing.
The author(s) declare that financial support was received for the research, authorship, and/or publication of this article. This study was supported by the Innovative Research Groups of National Natural Science Foundation of China (81721091), Major program of National Natural Science Foundation of China (91542205), National S&T Major Project (2017ZX10203205), Zhejiang International Science and Technology Cooperation Project (2016C04003), Zhejiang Provincial Natural Science Foundation of China (LY22H030008), Zhejiang medical S&T program (2021KY145 and 2022KY752), International Youth Exchange Program by China Association for Science and Technology (2020), Tianqing Liver Diseases Research Fund (TQGB20200114), Organ Transplantation Overseas Training for Youth Talents from Shulan Excellent Talent Project, CSCO (Chinese Society Of Clinical Oncology)-Bayer Tumor Research Funding (Y-bayer202001/zb-0003), Research fund from Chen Xiao-ping Foundation for Development of Science and Technology of Hubei Province (CXPJJH122002-078), Beijing iGandan Foundation (1082022-RGG022), Zhejiang Shuren University Basic Scientific Research Special Funds (2023XZ010), and Startup fund for Advanced Talents from Zhejiang Shuren University.
The authors declare that the research was conducted in the absence of any commercial or financial relationships that could be construed as a potential conflict of interest.
All claims expressed in this article are solely those of the authors and do not necessarily represent those of their affiliated organizations, or those of the publisher, the editors and the reviewers. Any product that may be evaluated in this article, or claim that may be made by its manufacturer, is not guaranteed or endorsed by the publisher.
The Supplementary material for this article can be found online at: https://www.frontiersin.org/articles/10.3389/fnut.2024.1437681/full#supplementary-material
1. Gade, W, Schmit, J, Collins, M, and Gade, J. Beyond obesity: the diagnosis and pathophysiology of metabolic syndrome. Clin Lab Sci. (2010) 23:51–61. doi: 10.29074/ascls.23.1.51
2. Samson, SL, and Garber, AJ. Metabolic syndrome. Endocrinol Metab Clin N Am. (2014) 43:1–23. doi: 10.1016/j.ecl.2013.09.009
3. Eckel, RH, Grundy, SM, and Zimmet, PZ. The metabolic syndrome. Lancet. (2005) 365:1415–28. doi: 10.1016/S0140-6736(05)66378-7
4. Gami, AS, Witt, BJ, Howard, DE, Erwin, PJ, Gami, LA, Somers, VK, et al. Metabolic syndrome and risk of incident cardiovascular events and death: a systematic review and meta-analysis of longitudinal studies. J Am Coll Cardiol. (2007) 49:403–14. doi: 10.1016/j.jacc.2006.09.032
5. Ford, ES. Risks for all-cause mortality, cardiovascular disease, and diabetes associated with the metabolic syndrome: a summary of the evidence. Diabetes Care. (2005) 28:1769–78. doi: 10.2337/diacare.28.7.1769
6. Chalasani, N, Younossi, Z, Lavine, JE, Diehl, AM, Brunt, EM, Cusi, K, et al. The diagnosis and management of non-alcoholic fatty liver disease: practice guideline by the American Association for the Study of Liver Diseases, American College of Gastroenterology, and the American Gastroenterological Association. Am J Gastroenterol. (2012) 107:811–26. doi: 10.1038/ajg.2012.128
7. Méndez-Sánchez, N, Arrese, M, Zamora-Valdés, D, and Uribe, M. Current concepts in the pathogenesis of nonalcoholic fatty liver disease. Liver Int Off J Assoc Study Liver. (2007) 27:423–33. doi: 10.1111/j.1478-3231.2007.01483.x
8. Cook, JD, Lipschitz, DA, Miles, LE, and Finch, CA. Serum ferritin as a measure of iron stores in normal subjects. Am J Clin Nutr. (1974) 27:681–7. doi: 10.1093/ajcn/27.7.681
9. Szudzik, M, Lipiński, P, Jończy, A, Mazgaj, R, Pieszka, M, Kamyczek, M, et al. Long-term effect of Split Iron dextran/hemoglobin supplementation on erythrocyte and Iron status, growth performance, carcass parameters, and meat quality of polish large white and 990 line pigs. Biol Trace Elem Res. (2020) 196:472–80. doi: 10.1007/s12011-019-01950-w
10. Torti, SV, and Torti, FM. Iron and cancer: more ore to be mined. Nat Rev Cancer. (2013) 13:342–55. doi: 10.1038/nrc3495
11. Abbaspour, N, Hurrell, R, and Kelishadi, R. Review on iron and its importance for human health. J Res Med Sci. (2014) 19:164–74.
12. Galaris, D, Barbouti, A, and Pantopoulos, K. Iron homeostasis and oxidative stress: an intimate relationship. Biochim Biophys Acta, Mol Cell Res. (2019) 1866:118535. doi: 10.1016/j.bbamcr.2019.118535
13. Birben, E, Sahiner, UM, Sackesen, C, Erzurum, S, and Kalayci, O. Oxidative stress and antioxidant defense. World Allergy Organ J. (2012) 5:9–19. doi: 10.1097/WOX.0b013e3182439613
14. Abril-Ulloa, V, Flores-Mateo, G, Solà-Alberich, R, Manuel-y-Keenoy, B, and Arija, V. Ferritin levels and risk of metabolic syndrome: meta-analysis of observational studies. BMC Public Health. (2014) 14:483. doi: 10.1186/1471-2458-14-483
15. Jin, Y, He, L, Chen, Y, Fang, Y, and Yao, Y. Association between serum ferritin levels and metabolic syndrome: an updated meta-analysis. Int J Clin Exp Med. (2015) 8:13317–22.
16. Zhang, WCB, Xing, Y, and Shao, B. Serum ferritin and the risk of metabolic syndrome: a systematic review and dose-response meta-analysis of cross-sectional studies. Biomed Environ Sci. (2021) 34:623–31. doi: 10.3967/bes2021.086
17. Suárez-Ortegón, MF, Ensaldo-Carrasco, E, Shi, T, McLachlan, S, Fernández-Real, JM, and Wild, SH. Ferritin, metabolic syndrome and its components: a systematic review and meta-analysis. Atherosclerosis. (2018) 275:97–106. doi: 10.1016/j.atherosclerosis.2018.05.043
18. Du, SX, Lu, LL, Geng, N, Victor, DW, Chen, LZ, Wang, C, et al. Association of serum ferritin with non-alcoholic fatty liver disease: a meta-analysis. Lipids Health Dis. (2017) 16:228. doi: 10.1186/s12944-017-0613-4
19. Ding, J, Liu, Q, Liu, Z, Guo, H, Liang, J, and Zhang, Y. Associations of the dietary Iron, copper, and selenium level with metabolic syndrome: a meta-analysis of observational studies. Front Nutr. (2021) 8:810494. doi: 10.3389/fnut.2021.810494
20. Liu, Z, Que, S, Zhou, L, and Zheng, S. Dose-response relationship of serum uric acid with metabolic syndrome and non-alcoholic fatty liver disease incidence: a meta-analysis of prospective studies. Sci Rep. (2015) 5:14325. doi: 10.1038/srep14325
21. Liu, Z, Jia, J, Ning, H, Que, S, Zhou, L, and Zheng, S. Systematic evaluation of the safety threshold for allograft macrovesicular steatosis in cadaveric liver transplantation. Front Physiol. (2019) 10:429. doi: 10.3389/fphys.2019.00429
22. Liu, Z, Wang, W, Zhuang, L, Liu, J, Que, S, Zhu, D, et al. Clear mortality gap caused by graft macrosteatosis in Chinese patients after cadaveric liver transplantation. Hepatobiliary Surg Nutr. (2020) 9:739–58. doi: 10.21037/hbsn.2019.12.02
23. Chang, JS, Lin, SM, Huang, TC, Chao, JC, Chen, YC, Pan, WH, et al. Serum ferritin and risk of the metabolic syndrome: a population-based study. Asia Pac J Clin Nutr. (2013) 22:400–7. doi: 10.6133/apjcn.2013.22.3.07
24. Chen, L, Li, Y, Zhang, F, Zhang, S, Zhou, X, and Ji, L. Association of serum ferritin levels with metabolic syndrome and insulin resistance in a Chinese population. J Diabetes Complicat. (2017) 31:364–8. doi: 10.1016/j.jdiacomp.2016.06.018
25. Cho, GJ, Shin, JH, Yi, KW, Park, HT, Kim, T, Hur, JY, et al. Serum ferritin levels are associated with metabolic syndrome in postmenopausal women but not in premenopausal women. Menopause. (2011) 18:1120–4. doi: 10.1097/gme.0b013e318217e172
26. Jehn, M, Clark, JM, and Guallar, E. Serum ferritin and risk of the metabolic syndrome in U.S. adults. Diabetes Care. (2004) 27:2422–8. doi: 10.2337/diacare.27.10.2422
27. Kang, HT, Linton, JA, and Shim, JY. Serum ferritin level is associated with the prevalence of metabolic syndrome in Korean adults: the 2007-2008 Korean National Health and nutrition examination survey. Clin Chim Acta. (2012) 413:636–41. doi: 10.1016/j.cca.2011.12.011
28. Kim, CH, Kim, HK, Bae, SJ, Park, JY, and Lee, KU. Association of elevated serum ferritin concentration with insulin resistance and impaired glucose metabolism in Korean men and women. Metab Clin Exp. (2011) 60:414–20. doi: 10.1016/j.metabol.2010.03.007
29. Ledesma, M, Hurtado-Roca, Y, Leon, M, Giraldo, P, Pocovi, M, Civeira, F, et al. Association of ferritin elevation and metabolic syndrome in males. Results from the Aragon Workers' health study (AWHS). J Clin Endocrinol Metab. (2015) 100:2081–9. doi: 10.1210/jc.2014-4409
30. Lee, BK, Kim, Y, and Kim, YI. Association of serum ferritin with metabolic syndrome and diabetes mellitus in the South Korean general population according to the Korean National Health and nutrition examination survey 2008. Metab Clin Exp. (2011) 60:1416–24. doi: 10.1016/j.metabol.2011.02.008
31. Li, J, Wang, R, Luo, D, Li, S, and Xiao, C. Association between serum ferritin levels and risk of the metabolic syndrome in Chinese adults: a population study. PLoS One. (2013) 8:e74168. doi: 10.1371/journal.pone.0074168
32. Ryoo, JH, Kim, MG, Lee, DW, and Shin, JY. The relationship between serum ferritin and metabolic syndrome in healthy Korean men. Diabetes Metab Res Rev. (2011) 27:597–603. doi: 10.1002/dmrr.1211
33. Shim, YS, Kang, MJ, Oh, YJ, Baek, JW, Yang, S, and Hwang, IT. Association of serum ferritin with insulin resistance, abdominal obesity, and metabolic syndrome in Korean adolescent and adults: the Korean National Health and nutrition examination survey, 2008 to 2011. Medicine. (2017) 96:e6179. doi: 10.1097/MD.0000000000006179
34. Suárez-Ortegón, MF, McLachlan, S, Wild, SH, Fernández-Real, JM, Hayward, C, and Polašek, O. Soluble transferrin receptor levels are positively associated with insulin resistance but not with the metabolic syndrome or its individual components. Br J Nutr. (2016) 116:1165–74. doi: 10.1017/S0007114516002968
35. Sun, L, Franco, OH, Hu, FB, Cai, L, Yu, Z, Li, H, et al. Ferritin concentrations, metabolic syndrome, and type 2 diabetes in middle-aged and elderly Chinese. J Clin Endocrinol Metab. (2008) 93:4690–6. doi: 10.1210/jc.2008-1159
36. Tang, Q, Liu, Z, Tang, Y, Tan, A, Gao, Y, Lu, Z, et al. High serum ferritin level is an independent risk factor for metabolic syndrome in a Chinese male cohort population. Diabetol Metab Syndr. (2015) 7:11. doi: 10.1186/s13098-015-0004-9
37. Wang, M, Zhao, A, Szeto, IM, Wu, W, Ren, Z, Li, T, et al. Association of serum ferritin with metabolic syndrome in eight cities in China. Food Sci Nutr. (2020) 8:1406–14. doi: 10.1002/fsn3.1408
38. Chandok, N, Minuk, G, Wengiel, M, and Uhanova, J. Serum ferritin levels do not predict the stage of underlying non-alcoholic fatty liver disease. J Gastrointestin Liver Dis. (2012) 21:53–8.
39. Di Rosa, M, Mangano, K, De Gregorio, C, Nicoletti, F, and Malaguarnera, L. Association of chitotriosidase genotype with the development of non-alcoholic fatty liver disease. Hepatol Res. (2013) 43:267–75. doi: 10.1111/j.1872-034X.2012.01063.x
40. Goh, GB, Issa, D, Lopez, R, Dasarathy, S, Dasarathy, J, Sargent, R, et al. The development of a non-invasive model to predict the presence of non-alcoholic steatohepatitis in patients with non-alcoholic fatty liver disease. J Gastroenterol Hepatol. (2016) 31:995–1000. doi: 10.1111/jgh.13235
41. Hotta, K, Yoneda, M, Hyogo, H, Ochi, H, Mizusawa, S, Ueno, T, et al. Association of the rs738409 polymorphism in PNPLA3 with liver damage and the development of nonalcoholic fatty liver disease. BMC Med Genet. (2010) 11:172. doi: 10.1186/1471-2350-11-172
42. Hsiao, TJ, Chen, JC, and Wang, JD. Insulin resistance and ferritin as major determinants of nonalcoholic fatty liver disease in apparently healthy obese patients. Int J Obes Relat Metab Disord. (2004) 28:167–72. doi: 10.1038/sj.ijo.0802519
43. Jiang, Y, Zeng, J, and Chen, B. Hemoglobin combined with triglyceride and ferritin in predicting non-alcoholic fatty liver. J Gastroenterol Hepatol. (2014) 29:1508–14. doi: 10.1111/jgh.12580
44. Kim, HB, Lee, HS, and Lee, YJ. Association of serum ferritin levels with non-alcoholic fatty liver disease in postmenopausal women. Climacteric. (2018) 21:509–14. doi: 10.1080/13697137.2018.1493451
45. Sazci, A, Akpinar, G, Aygun, C, Ergul, E, Senturk, O, and Hulagu, S. Association of apolipoprotein E polymorphisms in patients with non-alcoholic steatohepatitis. Dig Dis Sci. (2008) 53:3218–24. doi: 10.1007/s10620-008-0271-5
46. Tsuchiya, H, Ashla, AA, Hoshikawa, Y, Matsumi, Y, Kanki, K, Enjoji, M, et al. Iron state in association with retinoid metabolism in non-alcoholic fatty liver disease. Hepatol Res. (2010) 40:1227–38. doi: 10.1111/j.1872-034X.2010.00719.x
47. Yang, HH, Chen, GC, Li, DM, Lan, L, Chen, LH, Xu, JY, et al. Serum iron and risk of nonalcoholic fatty liver disease and advanced hepatic fibrosis in US adults. Sci Rep. (2021) 11:10387. doi: 10.1038/s41598-021-89991-x
48. Yoneda, M, Nozaki, Y, Endo, H, Mawatari, H, Iida, H, Fujita, K, et al. Serum ferritin is a clinical biomarker in Japanese patients with nonalcoholic steatohepatitis (NASH) independent of HFE gene mutation. Dig Dis Sci. (2010) 55:808–14. doi: 10.1007/s10620-009-0771-y
49. Azadbakht, L, and Esmaillzadeh, A. Red meat intake is associated with metabolic syndrome and the plasma C-reactive protein concentration in women. J Nutr. (2009) 139:335–9. doi: 10.3945/jn.108.096297
50. Bruscato, NM, Vieira, JL, Do Nascimento, NM, Canto, ME, Stobbe, JC, Gottlieb, MG, et al. Dietary intake is not associated to the metabolic syndrome in elderly women. N. Am J Med Sci. (2010) 2:182–8.
51. de Oliveira Otto, MC, Alonso, A, Lee, DH, Delclos, GL, Bertoni, AG, Jiang, R, et al. Dietary intakes of zinc and heme iron from red meat, but not from other sources, are associated with greater risk of metabolic syndrome and cardiovascular disease. J Nutr. (2012) 142:526–33. doi: 10.3945/jn.111.149781
52. Dos Santos Vieira, DA, Hermes Sales, C, Galvão Cesar, CL, Marchioni, DM, and Fisberg, RM. Influence of Haem, non-Haem, and Total Iron intake on metabolic syndrome and its components: a population-based study. Nutrients. (2018) 10:314. doi: 10.3390/nu10030314
53. Esfandiar, Z, Hosseini-Esfahani, F, Mirmiran, P, Habibi-Moeini, AS, and Azizi, F. Red meat and dietary iron intakes are associated with some components of metabolic syndrome: Tehran lipid and glucose study. J Transl Med. (2019) 17:313. doi: 10.1186/s12967-019-2059-0
54. Motamed, S, Ebrahimi, M, Safarian, M, Ghayour-Mobarhan, M, Mouhebati, M, Azarpazhouh, M, et al. Micronutrient intake and the presence of the metabolic syndrome. N Am J Med Sci. (2013) 5:377–85. doi: 10.4103/1947-2714.114171
55. Zhu, Z, He, Y, Wu, F, Zhao, L, Wu, C, Lu, Y, et al. The associations of dietary Iron, zinc and magnesium with metabolic syndrome in China's mega cities. Nutrients. (2020) 12:659. doi: 10.3390/nu12030659
56. Zhu, Z, Wu, F, Lu, Y, Wu, C, Wang, Z, Zang, J, et al. Total and nonheme dietary Iron intake is associated with metabolic syndrome and its components in Chinese men and women. Nutrients. (2018) 10:1663. doi: 10.3390/nu10111663
57. Smetana, GW, Umscheid, CA, Chang, S, and Matchar, DB. Methods guide for authors of systematic reviews of medical tests: a collaboration between the Agency for Healthcare Research and Quality (AHRQ) and the journal of general internal medicine. J Gen Intern Med. (2012) 27:1–3. doi: 10.1007/s11606-012-2053-1
58. Higgins, JP, Thompson, SG, Deeks, JJ, and Altman, DG. Measuring inconsistency in meta-analyses. BMJ. (2003) 327:557–60. doi: 10.1136/bmj.327.7414.557
59. Mantel, N, and Haenszel, W. Statistical aspects of the analysis of data from retrospective studies of disease. J Natl Cancer Inst. (1959) 22:719–48.
60. DerSimonian, R, and Laird, N. Meta-analysis in clinical trials. Control Clin Trials. (1986) 7:177–88. doi: 10.1016/0197-2456(86)90046-2
61. Greenland, S, and Longnecker, MP. Methods for trend estimation from summarized dose-response data, with applications to meta-analysis. Am J Epidemiol. (1992) 135:1301–9. doi: 10.1093/oxfordjournals.aje.a116237
62. Chen, GC, Tong, X, Xu, JY, Han, SF, Wan, ZX, Qin, JB, et al. Whole-grain intake and total, cardiovascular, and cancer mortality: a systematic review and meta-analysis of prospective studies. Am J Clin Nutr. (2016) 104:164–72. doi: 10.3945/ajcn.115.122432
63. Egger, M, Davey Smith, G, Schneider, M, and Minder, C. Bias in meta-analysis detected by a simple, graphical test. BMJ. (1997) 315:629–34. doi: 10.1136/bmj.315.7109.629
64. Yang, N, Lu, Y, Cao, L, and Lu, M. The association between non-alcoholic fatty liver disease and serum ferritin levels in American adults. J Clin Lab Anal. (2022) 36:e24225. doi: 10.1002/jcla.24225
65. Jung, JY, Shim, JJ, Park, SK, Ryoo, JH, Choi, JM, Oh, IH, et al. Serum ferritin level is associated with liver steatosis and fibrosis in Korean general population. Hepatol Int. (2019) 13:222–33. doi: 10.1007/s12072-018-9892-8
66. Liu, Z, Hu, Y, Yang, X, Tan, A, Gao, Y, Qin, X, et al. Combinative analysis of factors influence serum alanine aminotransferase activity in adult male population from southern China. Clin Biochem. (2012) 45:1683–8. doi: 10.1016/j.clinbiochem.2012.08.022
67. Liu, Z, Liang, S, Que, S, Zhou, L, Zheng, S, and Mardinoglu, A. Meta-analysis of adiponectin as a biomarker for the detection of metabolic syndrome. Front Physiol. (2018) 9:1238. doi: 10.3389/fphys.2018.01238
68. Liu, Z, Zhu, H, Zhao, J, Yu, L, Que, S, Xu, J, et al. Multi-omics analysis reveals a crosstalk between ferroptosis and peroxisomes on steatotic graft failure after liver transplantation. MedComm. (2020) 5:e588. doi: 10.1002/mco2.588
69. Sharif, K, Vieira Borba, V, Zandman-Goddard, G, and Shoenfeld, Y. Eppur Si Muove: ferritin is essential in modulating inflammation. Clin Exp Immunol. (2018) 191:149–50. doi: 10.1111/cei.13069
70. Welty, FK, Alfaddagh, A, and Elajami, TK. Targeting inflammation in metabolic syndrome. Transl Res. (2016) 167:257–80. doi: 10.1016/j.trsl.2015.06.017
71. Cai, D, and Liu, T. Inflammatory cause of metabolic syndrome via brain stress and NF-κB. Aging. (2012) 4:98–115. doi: 10.18632/aging.100431
72. Arosio, P, and Levi, S. Ferritin, iron homeostasis, and oxidative damage. Free Radic Biol Med. (2002) 33:457–63. doi: 10.1016/S0891-5849(02)00842-0
73. Guo, H, and Ling, W. The update of anthocyanins on obesity and type 2 diabetes: experimental evidence and clinical perspectives. Rev Endocr Metab Disord. (2015) 16:1–13. doi: 10.1007/s11154-014-9302-z
74. Fernández-Real, JM, López-Bermejo, A, and Ricart, W. Cross-talk between iron metabolism and diabetes. Diabetes. (2002) 51:2348–54. doi: 10.2337/diabetes.51.8.2348
75. Han, LL, Wang, YX, Li, J, Zhang, XL, Bian, C, Wang, H, et al. Gender differences in associations of serum ferritin and diabetes, metabolic syndrome, and obesity in the China health and nutrition survey. Mol Nutr Food Res. (2014) 58:2189–95. doi: 10.1002/mnfr.201400088
76. Yoo, KD, Ko, SH, Park, JE, Ahn, YB, Yim, HW, Lee, WC, et al. High serum ferritin levels are associated with metabolic risk factors in non-obese Korean young adults: Korean National Health and nutrition examination survey (KNHANES) IV. Clin Endocrinol. (2012) 77:233–40. doi: 10.1111/j.1365-2265.2011.04248.x
77. Punnonen, R, Ikäläinen, M, and Seppälä, E. Premenopausal hysterectomy and risk of cardiovascular disease. Lancet. (1987) 1:1139. doi: 10.1016/S0140-6736(87)91689-8
78. Reis, JP, Allen, N, Gunderson, EP, Lee, JM, Lewis, CE, Loria, CM, et al. Excess body mass index-and waist circumference-years and incident cardiovascular disease: the CARDIA study. Obesity. (2015) 23:879–85. doi: 10.1002/oby.21023
79. Milman, N, and Kirchhoff, M. Relationship between serum ferritin and risk factors for ischaemic heart disease in 2235 Danes aged 30-60 years. J Intern Med. (1999) 245:423–33. doi: 10.1046/j.1365-2796.1999.00465.x
80. Zhao, L, Zhang, X, Shen, Y, Fang, X, Wang, Y, and Wang, F. Obesity and iron deficiency: a quantitative meta-analysis. Obes Rev. (2015) 16:1081–93. doi: 10.1111/obr.12323
81. Zafon, C, Lecube, A, and Simó, R. Iron in obesity. An ancient micronutrient for a modern disease. Obes Rev. (2010) 11:322–8. doi: 10.1111/j.1467-789X.2009.00638.x
82. Cushman, M, Arnold, AM, Psaty, BM, Manolio, TA, Kuller, LH, Burke, GL, et al. C-reactive protein and the 10-year incidence of coronary heart disease in older men and women: the cardiovascular health study. Circulation. (2005) 112:25–31. doi: 10.1161/CIRCULATIONAHA.104.504159
83. Laaksonen, DE, Niskanen, L, Nyyssönen, K, Punnonen, K, Tuomainen, TP, Valkonen, VP, et al. C-reactive protein and the development of the metabolic syndrome and diabetes in middle-aged men. Diabetologia. (2004) 47:1403–10. doi: 10.1007/s00125-004-1472-x
84. Liew, PL, Wang, W, Lee, YC, Huang, MT, Lin, YC, and Lee, WJ. Gallbladder disease among obese patients in Taiwan. Obes Surg. (2007) 17:383–90. doi: 10.1007/s11695-007-9068-4
85. Kim, CH, and Younossi, ZM. Nonalcoholic fatty liver disease: a manifestation of the metabolic syndrome. Cleve Clin J Med. (2008) 75:721–8. doi: 10.3949/ccjm.75.10.721
86. Lyu, J, Lin, Q, Fang, Z, Xu, Z, and Liu, Z. Complex impacts of gallstone disease on metabolic syndrome and nonalcoholic fatty liver disease. Front Endocrinol. (2022) 13:1032557. doi: 10.3389/fendo.2022.1032557
87. Wieser, V, Tymoszuk, P, Adolph, TE, Grander, C, Grabherr, F, Enrich, B, et al. Lipocalin 2 drives neutrophilic inflammation in alcoholic liver disease. J Hepatol. (2016) 64:872–80. doi: 10.1016/j.jhep.2015.11.037
88. Xiao, X, Yeoh, BS, and Vijay-Kumar, M. Lipocalin 2: an emerging player in Iron homeostasis and inflammation. Annu Rev Nutr. (2017) 37:103–30. doi: 10.1146/annurev-nutr-071816-064559
89. Sachinidis, A, Doumas, M, Imprialos, K, Stavropoulos, K, Katsimardou, A, and Athyros, VG. Dysmetabolic iron overload in metabolic syndrome. Curr Pharm Des. (2020) 26:1019–24. doi: 10.2174/1381612826666200130090703
90. Ryan, JD, Armitage, AE, Cobbold, JF, Banerjee, R, Borsani, O, Dongiovanni, P, et al. Hepatic iron is the major determinant of serum ferritin in NAFLD patients. Liver Int Off J Assoc Study Liver. (2018) 38:164–73. doi: 10.1111/liv.13513
91. Mörwald, K, Aigner, E, Bergsten, P, Brunner, SM, Forslund, A, Kullberg, J, et al. Serum ferritin correlates with liver fat in male adolescents with obesity. Front Endocrinol. (2020) 11:340. doi: 10.3389/fendo.2020.00340
92. Utzschneider, KM, Largajolli, A, Bertoldo, A, Marcovina, S, Nelson, JE, Yeh, MM, et al. Serum ferritin is associated with non-alcoholic fatty liver disease and decreased Β-cell function in non-diabetic men and women. J Diabetes Complicat. (2014) 28:177–84. doi: 10.1016/j.jdiacomp.2013.11.007
93. Manousou, P, Kalambokis, G, Grillo, F, Watkins, J, Xirouchakis, E, Pleguezuelo, M, et al. Serum ferritin is a discriminant marker for both fibrosis and inflammation in histologically proven non-alcoholic fatty liver disease patients. Liver Int Off J Assoc Study Liver. (2011) 31:730–9. doi: 10.1111/j.1478-3231.2011.02488.x
94. Bugianesi, E, Manzini, P, D'Antico, S, Vanni, E, Longo, F, Leone, N, et al. Relative contribution of iron burden, HFE mutations, and insulin resistance to fibrosis in nonalcoholic fatty liver. Hepatology. (2004) 39:179–87. doi: 10.1002/hep.20023
95. Angulo, P, George, J, Day, CP, Vanni, E, Russell, L, De la Cruz, AC, et al. Serum ferritin levels lack diagnostic accuracy for liver fibrosis in patients with nonalcoholic fatty liver disease. Clin Gastroenterol Hepatol Off Prac J Am Gastroenterol Assoc. (2014) 12:1163–9. doi: 10.1016/j.cgh.2013.11.035
96. Burke, W, Imperatore, G, and Reyes, M. Iron deficiency and iron overload: effects of diet and genes. Proc Nutr Soc. (2001) 60:73–80. doi: 10.1079/PNS200069
97. Fleming, DJ, Jacques, PF, Dallal, GE, Tucker, KL, Wilson, PW, and Wood, RJ. Dietary determinants of iron stores in a free-living elderly population: the Framingham heart study. Am J Clin Nutr. (1998) 67:722–33. doi: 10.1093/ajcn/67.4.722
98. Menzie, CM, Yanoff, LB, Denkinger, BI, McHugh, T, Sebring, NG, Calis, KA, et al. Obesity-related hypoferremia is not explained by differences in reported intake of heme and nonheme iron or intake of dietary factors that can affect iron absorption. J Am Diet Assoc. (2008) 108:145–8. doi: 10.1016/j.jada.2007.10.034
Keywords: serum ferritin, dietary iron, metabolic syndrome, non-alcoholic fatty liver disease, meta-analysis, dose–response relationship
Citation: Yu L, Que T, Zhou Y and Liu Z (2024) Dose–response relationship of serum ferritin and dietary iron intake with metabolic syndrome and non-alcoholic fatty liver disease incidence: a systematic review and meta-analysis. Front. Nutr. 11:1437681. doi: 10.3389/fnut.2024.1437681
Received: 24 May 2024; Accepted: 09 September 2024;
Published: 01 October 2024.
Edited by:
Di Wu, University of Oxford, United KingdomReviewed by:
Matteo Della Porta, University of Milan, ItalyCopyright © 2024 Yu, Que, Zhou and Liu. This is an open-access article distributed under the terms of the Creative Commons Attribution License (CC BY). The use, distribution or reproduction in other forums is permitted, provided the original author(s) and the copyright owner(s) are credited and that the original publication in this journal is cited, in accordance with accepted academic practice. No use, distribution or reproduction is permitted which does not comply with these terms.
*Correspondence: Zhengtao Liu, bGl1emhlbmd0YW9AempzcnUuZWR1LmNu
†These authors have contributed equally to this work
Disclaimer: All claims expressed in this article are solely those of the authors and do not necessarily represent those of their affiliated organizations, or those of the publisher, the editors and the reviewers. Any product that may be evaluated in this article or claim that may be made by its manufacturer is not guaranteed or endorsed by the publisher.
Research integrity at Frontiers
Learn more about the work of our research integrity team to safeguard the quality of each article we publish.