- 1The First School of Clinical Medicine, Lanzhou University, Lanzhou, Gansu Province, China
- 2Department of Gastroenterology, The First Hospital of Lanzhou University, Lanzhou, Gansu Province, China
- 3Gansu Province Clinical Research Center for Digestive Diseases, Lanzhou University, Lanzhou, Gansu Province, China
Background: Evidence from observational studies on the association between folate and metabolic dysfunction-associated steatotic liver disease (MASLD) is conflicting.
Aims: This study aimed to investigate the association between serum folate concentration and MASLD and further assess the causal relationship using Mendelian randomization (MR) analysis.
Methods: To investigate the causal relationship between serum folate and MASLD, we conducted a cross-sectional study that selected 1,117 participants from the 2017–2020 National Health and Nutrition Examination Survey (NHANES). The association between serum folate level and the risk of MASLD was evaluated under a multivariate logistic regression model. In addition, we conducted a two-sample MR study using genetic data from a large genome-wide association study (GWAS) to compare serum folate level (37,465 individuals) and MASLD (primary analysis: 8,434 cases/770,180 controls; Secondary analysis:1,483 cases/17,781 controls) were performed to infer causal relationships between them. Inverse variance weighted (IVW) was used as the primary method of MR Analysis.
Results: The results from the NHANES database showed that Tertile 3 group (Tertile 3: ≥ 48.6 nmol/L) had a significantly lower risk (OR = 0.58, 95% CI: 0.38–0.88, p = 0.010) of MASLD than Tertile 1 group (Tertile 1: < 22.3 nmol/L) after complete adjustments. However, in the IVW of MR analysis, there was no causal relationship between serum folate level and MASLD risk in the primary analysis (OR = 0.75, 95% CI: 0.55–1.02, p = 0.065) and secondary analysis (OR = 0.83, 95% CI: 0.39–1.74, p = 0.618).
Conclusion: In observational analyses, we observed an inverse association between higher serum folate concentrations and a reduced risk of MASLD. Our MR study generated similar results, but the association failed to reach the significance threshold of p < 0.05, suggesting that our MR study does not support a causal relationship between serum folate levels and MASLD risk. Additional research involving a larger number of cases would contribute to enhancing the confirmation of our preliminary findings.
1 Introduction
Metabolic dysfunction-associated steatotic liver disease (MASLD) is the leading cause of end-stage liver disease globally, with an estimated global prevalence of about 29.8% (1). MASLD poses significant threats to human health and imposes substantial economic burdens on society. MASLD is characterized by steatosis affecting more than 5% of liver cells and is unrelated to heavy alcohol consumption, lipid-causing drugs, or genetic disorders (2). MASLD includes various chronic liver conditions like simple hepatic steatosis, non-alcoholic hepatitis (NASH), and liver fibrosis (3, 4). Although the etiology of MASLD remains unclear, multiple factors such as oxidative stress, gut microbes, lipid metabolism, genetic susceptibility, insulin resistance, and some nutritional and lifestyle factors have been shown to work together to drive the development of MASLD (5, 6). However, there is currently no globally approved drug therapy for MASLD treatment (7). Therefore, it is particularly important to explore new intervention mechanisms, therapeutic agents, and targets for MASLD.
Folate, a water-soluble vitamin vital for one-carbon metabolism and methylation reactions, has been implicated in processes such as oxidative stress, liver fat metabolism, and chronic liver inflammation, all recognized risk factors for MASLD (8). Animal studies have demonstrated that folate supplementation reduces hepatic steatosis (9, 10). Previous epidemiological analyses have indicated an inverse relationship between higher serum total folate concentrations and the prevalence of MASLD and liver fibrosis (11). A recent study employed meta-analysis and MR analysis to illustrate an inverse association between serum folate and MASLD risk (12). However, past evidence has been inconsistent at times. A meta-analysis of eight studies published up to 2015 found no significant difference in folate concentrations between patients with and without MASLD (standardized mean difference: −0.26 nmol/L; 95% CI: −0.69 to 0.17) (13). Two recent cross-sectional studies among MASLD-related populations in the United States reported no observed association between serum or dietary folate and MASLD (14, 15). Although the main topic of their study was not serum folate, it does suggest that the association between serum folate and MASLD remains controversial. Therefore, further investigation into the association between serum folate levels and MASLD is warranted.
To explore the association between serum folate concentration and the risk of MASLD, we conducted an observational study utilizing data from the National Health and Nutrition Examination Survey (NHANES) database for the United States population (16). Additionally, we employed MR analysis. This epidemiological approach leverages genetic instrumental variables (IVs) to infer causality and evaluate the causal link between circulating folate levels and the risk of MASLD. MR circumvents potential confounding effects from environmental risk factors and disease progression, as an individual’s genotype is fixed at conception and remains unaffected by environmental and lifestyle factors (17). Consequently, genetic variants are recommended as IVs to mitigate reverse causality and other confounding factors. The present study systematically examined the genetic causal association between folate and MASLD risk, employing case–control and two-sample MR analyses. The findings of this study offer novel insights into clinical prevention and treatment strategies.
2 Materials and methods
2.1 Cross-sectional study
2.1.1 Study design and participants
National Health and Nutrition Examination Survey, a continuous national survey program administered by the National Center for Health Statistics (NCHS), aims to evaluate the health and nutritional standing of adults and children in the United States. The selection of participants followed the stratified, multistage sampling design employed by NHANES. Participants in this study underwent interviews covering demographics, socioeconomic factors, dietary habits, and health status. All participants provided their written informed consent prior to participation.
We utilized samples from the 2017–2020 NHANES survey with MASLD diagnoses to investigate the association between serum folate concentration and MASLD. Among the initial cohort of 15,560 participants, the following exclusion criteria were applied: (1) age < 18 years; (2) hepatitis B antibody, hepatitis C antibody, or hepatitis C virus RNA positive; (3) excessive alcohol drinkers (male >30 g/day, female >20 g/day); (4) participants with incomplete data on fatty liver index or serum folate; and (5) missing data for other relevant covariates. Consequently, the final sample comprised 1,117 participants. Figure 1 depicts the process of participant inclusion and exclusion.
2.1.2 Measurement of serum folate
In the NHANES, serum folate concentrations were quantified using isotope dilution high-performance liquid chromatography–tandem mass spectrometry (LC–MS/MS) (18). The serum samples were initially mixed with an ammonium format buffer and an internal standard mixture. Next, automated solid-phase extraction (SPE) using a 96-well phenyl SPE plate was employed for sample extraction and purification. Within 6 min, the folate form was isolated using an isocratic mobile phase condition and analyzed using LC–MS/MS.
2.1.3 Measurement of MASLD
In this study, the diagnosis of MASLD in adults was determined by measuring the controlled attenuation parameter (CAP) using vibration-controlled transient elastography (VCTE). Hepatic steatosis was assessed based on a CAP threshold of ≥248 dB/m; values exceeding this threshold indicated the presence of hepatic steatosis (19). MASLD is defined as hepatic steatosis combined with metabolic dysfunction, without other causes of hepatic steatosis. Metabolic dysfunction is diagnosed if at least one of the following criteria is met: (1) Body Mass Index (BMI) ≥ 25 kg/m2 or waist circumference > 94 cm for men and > 80 cm for women (adjusted for race); (2) Fasting blood glucose ≥5.6 mmol/L, 2-h postprandial blood glucose ≥7.8 mmol/L, HbA1c ≥ 5.7%, or a diagnosis of type 2 diabetes or current treatment for type 2 diabetes; (3) Blood pressure ≥ 130/85 mmHg or use of antihypertensive medication; (4) Plasma triglycerides(TG) ≥ 1.7 mmol/L or treatment for elevated blood lipids; and (5) Plasma high-density lipoprotein cholesterol(HDL-C) ≤ 1.0 mmol/L for male or ≤ 1.3 mmol/L for female, or treatment for elevated blood lipids.
2.1.4 Statistical analysis
R version 4.3.2 was used for all analyses. Categorical variables were described using counts and proportions, while continuous data were summarized using means and standard deviations. A two-sided p value less than 0.05 was considered statistically significant. Logistic regression models were constructed to evaluate the relationship between serum folate levels and MASLD risk. Four logistic regression models were formulated, incorporating different combinations of covariates. The Crude model did not include any adjustments for variables. Model 1 was adjusted for age, sex, race, education level, and economic status. Model 2 was further adjusted for total cholesterol (TC), HDL-C, BMI, Hypertension, Diabetes, and cardiovascular disease (CVD). Model 3, a fully adjusted model, encompassed the additional adjustments of smoking status, physical activity status, dietary energy intake, protein intake, folate intake, and alcohol intake. This logistic regression analysis categorized serum folate into tertiles, with Tertile 1 as the reference group.
2.2 Mendelian randomization study
2.2.1 Overview
Mendelian randomization analysis hinges on three core assumptions for establishing causal inference: (1) the IV must be strongly associated with the exposure; (2) the IV should be independent of potential confounding factors; and (3) IV should affect the outcome only through exposure. Figure 2 depicts an overview of the MR design implemented in this study. Notably, all studies incorporated in the GWASs received approval from the respective review boards, thus eliminating the need for additional ethical approval or participant consent.
2.2.2 Data sources
Our study utilized summary-level data derived from GWASs of European ancestry, as outlined in Table 1. Specifically, we identified single-nucleotide polymorphisms (SNPs) associated with serum folate concentrations, employing a genome-wide meta-analysis encompassing 37,645 participants of European descent. These SNPs were selected based on their significance below the genome-wide threshold (p < 5 × 10−8) and their independence from linkage disequilibrium (r2 < 0.01) (12, 20, 21). Notably, the variance in folate concentrations explained by the selected SNPs was estimated to be approximately 1.0%. Additionally, we extracted abstract-level data on MASLD from various GWAS meta-analyses, including the Electronic Medical Records and Genomics, United Kingdom Biobank, FinnGen, and Estonian Biobank studies, encompassing 8,434 MASLD cases and 770,180 controls (22). Furthermore, we included data from another GWAS comprising 4,761 MASLD cases and 373,227 controls (23).
2.2.3 MR estimates
For the primary MR analysis, IVW was employed to derive causal estimates through a meta-analysis of Wald ratio estimates for each SNP. Cochran’s Q statistic was utilized to assess the heterogeneity of SNP estimates across the MR associations. Additionally, the F-statistic was calculated, with an F value greater than 10 indicating a good strength of the genetic instrument (24). All MR analysis were conducted using the “TwosampleMR” program package, and odds ratios (ORs) with corresponding 95% confidence intervals (CIs) were computed. A two-sided p < 0.05 was considered statistically significant.
3 Results
3.1 Cross-sectional study
3.1.1 Baseline characteristics of the study population based on serum folate quartile groups
The study utilized data from the National Health and Nutrition Examination Survey collected between 2017 and 2020, including 1,117 participants. Table 2 displays the baseline characteristics of the study population stratified by serum folate quartiles. Participants in the Tertile 4 group, who had the highest folate levels, were significantly older, more likely to be non-Hispanic White, had higher household incomes, had never smoked, and exhibited higher levels of physical activity compared to those in the Tertile 1 group, who had the lowest folate levels. Additionally, those in the Tertile 4 group had higher dietary folate intake, as well as elevated levels of alanine aminotransferase (ALT), aspartate transaminase (AST), and HDL-C. They also had lower waist circumference, BMI, platelet count, Fibrosis 4 index (FIB-4), and a lower prevalence of MASLD. However, no statistically significant differences were observed among the quartiles regarding TG, TC, γ-glutamyl transpeptidase (GGT), dietary energy and protein intake, alcohol consumption, education level, sex distribution, or the prevalence of diabetes, hypertension, and CVD.
3.1.2 Associations between MASLD and serum folate (all participants)
Table 3 presents the crude and adjusted odds ratios for the association between serum folate levels and MASLD. Our results indicate a significant inverse relationship between serum folate levels and MASLD in all models. When compared to the crude model, Model 1 exhibited a modest increase in the strength of this association after adjusting for confounders such as age, sex, race, education level, and economic status (OR: 0.988, 95% CI: 0.982–0.994, p < 0.001). Model 2, which builds upon Model 1, incorporates additional adjustments for TC, HDL-C, BMI, Hypertension, Diabetes status, and CVD (OR: 0.991, 95% CI: 0.985–0.998, p = 0.011). Finally, Model 3 extends Model 2 by incorporating further adjustments for smoking status, physical activity status, TKCAL, TPROT, TFOLA, and drinking status. Notably, the association remained stable in Model 3 (OR = 0.991, 95% CI: 0.984 ~ 0.998, p = 0.017).
3.1.3 Association between MASLD and serum folate levels (tertiles)
Table 4 depicts the association between varying concentrations of serum folate and the risk of developing MASLD. Logistic regression analysis revealed that individuals in the Tertile 3 group (≥48.6 nmol/L) exhibited a lower risk of MASLD compared to those in the Tertile 1 group (< 22.3 nmol/L). In the crude model, which did not account for covariates, the risk of MASLD in the Tertile 3 group was reduced by 42% (OR: 0.58, 95% CI: 0.42–0.82, p = 0.002). A similar risk reduction was observed in the Tertile 2 serum folate group. Upon adjusting for covariates, the protective effect of higher serum folate concentrations on the risk of MASLD in the Tertile 3 group remained stable across Models 1–3 (Model 1: OR: 0.50, 95% CI: 0.35–0.71, p < 0.001; Model 2: OR: 0.58, 95% CI: 0.39–0.87, p = 0.008; Model 3: OR: 0.58, 95% CI: 0.38–0.88, p = 0.010). Notably, the risk of MASLD in Model 3 was slightly reduced compared to Model 1.
3.2 Mendelian randomization study
Utilizing the SNP selection criteria, Ghodsian et al. and Fairfield et al. were left with only two SNPs each to analyze the association between circulating folate levels and MASLD. The Data sources and MR estimate describe the associated exclusion SNPs. Considering the F-statistics of the selected SNPs, which range from 39.35 to 144 (Table 5), our findings are unlikely to be influenced by weak instruments. In both the primary analysis (OR: 0.75, 95% CI: 0.55–1.02, p = 0.065) and the secondary analysis (OR: 0.83, 95% CI: 0.39–1.74, p = 0.618) using the IVW model, the association failed to reach the significance threshold of p < 0.05, indicating that the observed association is not causal (Table 6; Figure 3).

Table 5. Summary information for SNPs used as genetic instruments for Mendelian randomization analyses.
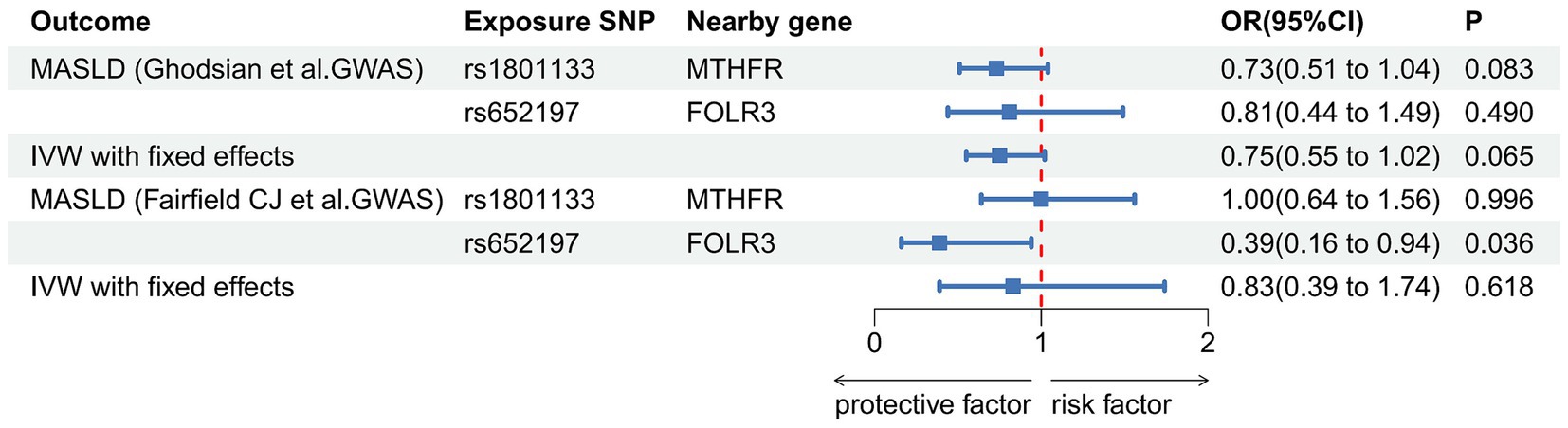
Figure 3. Association of genetically predicted concentrations of serum folate and risk of metabolic dysfunction-associated steatotic liver disease. The estimates for folate are derived from the IVW model.
4 Discussion
In this study, we integrated a cross-sectional survey utilizing data from the NHANES spanning 2017–2020 with a two-sample MR analysis of summary GWAS data to explore the relationship between serum folate levels and the risk of MASLD. In observational analyses, we observed an inverse association between higher serum folate concentrations and a reduced risk of MASLD. This finding remained robust even after adjusting for various potential confounders, such as sociodemographic characteristics, lifestyle factors, hypertension, and diabetes. In the MR analysis, two separate GWAS datasets of MASLD generated similar results, but the association failed to reach the significance threshold of p < 0.05, suggesting that the observed association is not causal. Our results indicate that elevated folate levels may be protective against MASLD, though further investigation is warranted to confirm this hypothesis.
The relationship between folate and MASLD has garnered significant attention in previous research, offering valuable insights. Notably, a randomized controlled trial investigating the effects of a green Mediterranean diet on intrahepatic fat revealed that subjects experiencing the most significant increase in serum folate exhibited greater reductions in intrahepatic fat (IHF), suggesting the efficacy of serum folate in mitigating MASLD risk (25). Additionally, the study by Mahamid et al. identified a significant correlation between lower folate levels and the severity of liver fibrosis (26). A recent meta-analysis encompassing data from 12 studies corroborated these findings and reinforced the inverse association between serum folate concentration and MASLD risk through primary and subgroup analyses (12). These concordant observations, consistent with the cross-sectional analysis presented in this study, underscore the potential role of folate in the prevention and management of MASLD.
However, some studies have reached contrasting conclusions. A meta-analysis encompassing eight studies published up to 2015 found no significant difference in folate concentrations between patients with and without MASLD (13). Li et al. (15), utilizing data from NHANES 1999–2004, suggested no direct association between serum folate and MASLD. Similarly, Liu et al. (14) examined the relationship between vitamins and MASLD but did not observe an association between dietary folate intake and MASLD. This discrepancy may stem from variations in study years, sample sizes, covariate adjustments, dietary intake measurements, and serum folate levels. Furthermore, our MR analysis revealed no significant trend in the association between genetically predicted serum folate concentration and MASLD risk. Therefore, more extensive GWAS are required to enhance our understanding of micronutrient regulation and optimize the instrumental variables in MR analysis. Additionally, clinical studies with larger sample sizes are necessary to clarify the relationship between serum folate and MASLD.
The inverse association between serum folate levels and MASLD is supported by multiple mechanisms. Firstly, folate deficiency decreases methylation capacity, which affects carnitine and phosphatidylcholine (PC) synthesis, promoting MASLD progression (27). Secondly, folic acid exhibits potent antioxidant properties, enhancing mitochondrial β-oxidation, reducing oxidative stress, and inhibiting peroxisome proliferator-activated receptor γ (PPARγ), a key regulator of lipogenesis and hepatic TG accumulation, thereby mitigating hepatic steatosis (28). Thirdly, elevated serum folate levels attenuate the expression of proinflammatory cytokines (IL-6 and TNF-α), inhibiting Kupffer cell recruitment and activation, ultimately reducing the risk of MASLD (29); Fourthly, folic acid modulates liver microRNAs, contributing to lower blood glucose and lipid concentrations, improved insulin sensitivity, and enhanced liver function (30). Fifthly, folate deficiency disrupts the fibroblast growth factor (FGF) pathway and activates adipokines and inflammatory factors, thereby fostering fatty liver disease development (31, 32). Furthermore, Kim et al. (33) demonstrated that folic acid supplementation activates the liver kinase B (LKB1)/AMP-activated protein kinase (AMPK)/acetyl coenzyme A carboxylase (ACC) pathway, increases hepatic S-adenosylmethionine, and inhibits hepatic lipogenesis, ultimately improving hepatic steatosis.
The current study boasts several notable strengths. Firstly, the utilization of a large cross-sectional study based on NHANES, alongside the integration of two-sample MR analysis, provides a robust framework for exploring the relationship between serum folate concentration and the risk of MASLD. The cross-sectional approach allows for an epidemiological evaluation of this relationship, while the MR studies effectively mitigate potential confounders and reverse causality. By independently examining two associations and then combining the data, we increased case numbers, thereby enhancing the power of our established associations compared to previous MR studies. However, some limitations need to be acknowledged. Firstly, despite our efforts to include a comprehensive set of covariates in the cross-sectional study to minimize bias from potential confounders, residual confounding may persist due to the possibility of unaccounted variables. Secondly, in this study, we used non-invasive VCTE to determine whether subjects had liver steatosis and liver fibrosis instead of using liver biopsy, which inevitably leads to bias in the diagnosis of MASLD. Thirdly, we could not perform a sensitivity analysis for folate due to the use of less than three SNPs in the analysis. However, it is worth noting that the association for folate was derived from an SNP located within the gene encoding methylenetetrahydrofolate reductase (MTHFR), indicating that this association is unlikely to be biased by pleiotropy from a biological perspective. Fourthly, MR relies on the assumption of a linear relationship between exposure and outcome, which may only sometimes hold in the presence of nonlinear associations. Fifthly, MR considers the lifelong impact of micronutrient status by utilizing genetic variation as an instrumental variable. Therefore, our MR results should not be generalized to extreme cases of micronutrient status. Lastly, it is essential to note that our cross-sectional and genetic data were obtained from different samples, with the MR Study focusing on individuals of European ancestry. In contrast, the cross-sectional study encompassed a multi-racial United States population. This may introduce some heterogeneity in our results, which should be considered when interpreting our findings.
5 Conclusion
In conclusion, despite cross-sectional studies indicating a significant association, our study does not endorse a causal relationship between genetically determined serum folate levels and the risk of MASLD. However, observational study results may be skewed due to uncontrolled confounding factors. Consequently, based on the current research, there is insufficient evidence to suggest that elevated serum folate levels may contribute to preventing or treating MASLD. While our findings are promising, further research with larger sample sizes is warranted to strengthen the validation of our observations and ensure broader applicability.
Data availability statement
The raw data supporting the conclusions of this article will be made available by the authors, without undue reservation.
Author contributions
YC: Writing – original draft. JG: Writing – review & editing. XW: Writing – review & editing. HL: Writing – review & editing. YZ: Writing – review & editing. QR: Supervision, Writing – review & editing.
Funding
The author(s) declare that financial support was received for the research, authorship, and/or publication of this article. This study was supported by Health Industry Research Project of Gansu Province (GSWSKY2020-07) and the Fundamental Research Funds for the Central Universities (lzujbky-2021-ct17).
Acknowledgments
We wish to express their gratitude to the authors and participants of the global working groups involved for providing the summary statistics.
Conflict of interest
The authors declare that the research was conducted in the absence of any commercial or financial relationships that could be construed as a potential conflict of interest.
Publisher’s note
All claims expressed in this article are solely those of the authors and do not necessarily represent those of their affiliated organizations, or those of the publisher, the editors and the reviewers. Any product that may be evaluated in this article, or claim that may be made by its manufacturer, is not guaranteed or endorsed by the publisher.
References
1. Le, MH, Yeo, YH, Li, X, Li, J, Zou, B, Wu, Y, et al. 2019 global NAFLD prevalence: a systematic review and Meta-analysis. Clin Gastroenterol Hepatol. (2022) 20:2809–2817.e28. doi: 10.1016/j.cgh.2021.12.002
2. European Association for the Study of the Liver (EASL) . European Association for the Study of diabetes (EASD), European Association for the Study of obesity (EASO). EASL-EASD-EASO clinical practice guidelines for the management of non-alcoholic fatty liver disease. Diabetologia. (2016) 59:1121–40. doi: 10.1007/s00125-016-3902-y
3. Younossi, Z, Anstee, QM, Marietti, M, Hardy, T, Henry, L, Eslam, M, et al. Global burden of NAFLD and NASH: trends, predictions, risk factors and prevention. Nat Rev Gastroenterol Hepatol. (2018) 15:11–20. doi: 10.1038/nrgastro.2017.109
4. Masarone, M, Federico, A, Abenavoli, L, Loguercio, C, and Persico, M. Non alcoholic fatty liver: epidemiology and natural history. Rev Recent Clin Trials. (2014) 9:126–33. doi: 10.2174/1574887109666141216111143
5. Byrne, CD, and Targher, G. NAFLD: a multisystem disease. J Hepatol. (2015) 62:S47–64. doi: 10.1016/j.jhep.2014.12.012
6. Rives, C, Fougerat, A, Ellero-Simatos, S, Loiseau, N, Guillou, H, Gamet-Payrastre, L, et al. Oxidative stress in NAFLD: role of nutrients and food contaminants. Biomol Ther. (2020) 10:1702. doi: 10.3390/biom10121702
7. Berná, G, and Romero-Gomez, M. The role of nutrition in non-alcoholic fatty liver disease: pathophysiology and management. Liver Int. (2020) 40:102–8. doi: 10.1111/liv.14360
8. Sid, V, Siow, YL, and Karmin, O. Role of folate in nonalcoholic fatty liver disease. Can J Physiol Pharmacol. (2017) 95:1141–8. doi: 10.1139/cjpp-2016-0681
9. Xin, F-Z, Zhao, Z-H, Zhang, R-N, Pan, Q, Gong, Z-Z, Sun, C, et al. Folic acid attenuates high-fat diet-induced steatohepatitis via deacetylase SIRT1-dependent restoration of PPARα. World J Gastroenterol. (2020) 26:2203–20. doi: 10.3748/wjg.v26.i18.2203
10. Liu, Y, Shen, J, Yang, X, Sun, Q, and Yang, X. Folic acid reduced triglycerides deposition in primary chicken hepatocytes. J Agric Food Chem. (2018) 66:13162–72. doi: 10.1021/acs.jafc.8b05193
11. Yang, S, Ye, Z, Liu, M, Zhang, Y, Wu, Q, Zhou, C, et al. Associations of different serum folate forms with indices of nonalcoholic fatty liver disease and advanced fibrosis. Obes Res Clin Pract. (2023) 17:58–65. doi: 10.1016/j.orcp.2023.01.004
12. Yuan, S, Chen, J, Dan, L, Xie, Y, Sun, Y, Li, X, et al. Homocysteine, folate, and nonalcoholic fatty liver disease: a systematic review with meta-analysis and Mendelian randomization investigation. Am J Clin Nutr. (2022) 116:1595–609. doi: 10.1093/ajcn/nqac285
13. Dai, Y, Zhu, J, Meng, D, Yu, C, and Li, Y. Association of homocysteine level with biopsy-proven non-alcoholic fatty liver disease: a meta-analysis. J Clin Biochem Nutr. (2016) 58:76–83. doi: 10.3164/jcbn.15-54
14. Liu, X, Shen, H, Chen, M, and Shao, J. Clinical relevance of vitamins and carotenoids with liver steatosis and fibrosis detected by transient Elastography in adults. Front Nutr. (2021) 8:760985. doi: 10.3389/fnut.2021.760985
15. Li, L, Huang, Q, Yang, L, Zhang, R, Gao, L, Han, X, et al. The association between non-alcoholic fatty liver disease (NAFLD) and advanced fibrosis with serological vitamin B12 markers: results from the NHANES 1999-2004. Nutrients. (2022) 14:1224. doi: 10.3390/nu14061224
16. Ahluwalia, N, Dwyer, J, Terry, A, Moshfegh, A, and Johnson, C. Update on NHANES dietary data: focus on collection, release, analytical considerations, and uses to inform public policy. Adv Nutr. (2016) 7:121–34. doi: 10.3945/an.115.009258
17. Sekula, P, Del Greco, MF, Pattaro, C, and Köttgen, A. Mendelian randomization as an approach to assess causality using observational data. J Am Soc Nephrol. (2016) 27:3253–65. doi: 10.1681/ASN.2016010098
18. Zhang, L, Sun, J, Li, Z, and Zhang, D. The relationship between serum folate and grip strength in American adults. Arch Osteoporos. (2021) 16:97. doi: 10.1007/s11657-021-00937-2
19. Rinella, ME, Lazarus, JV, Ratziu, V, Francque, SM, Sanyal, AJ, Kanwal, F, et al. A multisociety Delphi consensus statement on new fatty liver disease nomenclature. Ann Hepatol. (2024) 29:101133. doi: 10.1016/j.aohep.2023.101133
20. Björnsson, E, Thorleifsson, G, Helgadóttir, A, Guðnason, T, Guðbjartsson, T, Andersen, K, et al. Association of genetically predicted lipid levels with the extent of coronary atherosclerosis in Icelandic adults. JAMA Cardiol. (2020) 5:13–20. doi: 10.1001/jamacardio.2019.2946
21. Papadimitriou, N, Dimou, N, Gill, D, Tzoulaki, I, Murphy, N, Riboli, E, et al. Genetically predicted circulating concentrations of micronutrients and risk of breast cancer: a Mendelian randomization study. Int J Cancer. (2021) 148:646–53. doi: 10.1002/ijc.33246
22. Ghodsian, N, Abner, E, Emdin, CA, Gobeil, É, Taba, N, Haas, ME, et al. Electronic health record-based genome-wide meta-analysis provides insights on the genetic architecture of non-alcoholic fatty liver disease. Cell Rep Med. (2021) 2:100437. doi: 10.1016/j.xcrm.2021.100437
23. Fairfield, CJ, Drake, TM, Pius, R, Bretherick, AD, Campbell, A, Clark, DW, et al. Genome-wide association study of NAFLD using electronic health records. Hepatol Commun. (2022) 6:297–308. doi: 10.1002/hep4.1805
24. Burgess, S, Davies, NM, and Thompson, SG. Bias due to participant overlap in two-sample Mendelian randomization. Genet Epidemiol. (2016) 40:597–608. doi: 10.1002/gepi.21998
25. Yaskolka Meir, A, Rinott, E, Tsaban, G, Zelicha, H, Kaplan, A, Rosen, P, et al. Effect of green-Mediterranean diet on intrahepatic fat: the DIRECT PLUS randomised controlled trial. Gut. (2021) 70:2085–95. doi: 10.1136/gutjnl-2020-323106
26. Mahamid, M, Mahroum, N, Bragazzi, NL, Shalaata, K, Yavne, Y, Adawi, M, et al. Folate and B12 levels correlate with histological severity in NASH patients. Nutrients. (2018) 10:440. doi: 10.3390/nu10040440
27. da Silva, RP, Kelly, KB, Al Rajabi, A, and Jacobs, RL. Novel insights on interactions between folate and lipid metabolism. Biofactors. (2014) 40:277–83. doi: 10.1002/biof.1154
28. Li, L, Fu, J, Liu, D, Sun, J, Hou, Y, Chen, C, et al. Hepatocyte-specific Nrf2 deficiency mitigates high-fat diet-induced hepatic steatosis: involvement of reduced PPARγ expression. Redox Biol. (2020) 30:101412. doi: 10.1016/j.redox.2019.101412
29. Kolb, AF, and Petrie, L. Folate deficiency enhances the inflammatory response of macrophages. Mol Immunol. (2013) 54:164–72. doi: 10.1016/j.molimm.2012.11.012
30. Salman, M, Kamel, MA, El-Nabi, SEH, Ismail, AHA, Ullah, S, Al-Ghamdi, A, et al. The regulation of HBP1, SIRT1, and SREBP-1c genes and the related microRNAs in non-alcoholic fatty liver rats: the association with the folic acid anti-steatosis. PLoS One. (2022) 17:e0265455. doi: 10.1371/journal.pone.0265455
31. Chang, S, Lu, X, Wang, S, Wang, Z, Huo, J, Huang, J, et al. The effect of folic acid deficiency on FGF pathway via Brachyury regulation in neural tube defects. FASEB J. (2019) 33:4688–702. doi: 10.1096/fj.201801536R
32. Mendez-Sanchez, N, Cruz-Ramon, VC, Ramirez-Perez, OL, Hwang, JP, Barranco-Fragoso, B, and Cordova-Gallardo, J. New aspects of lipotoxicity in nonalcoholic steatohepatitis. Int J Mol Sci. (2018) 19:2034. doi: 10.3390/ijms19072034
Keywords: folate, Mendelian randomization, NHANES, MASLD, cross-sectional study
Citation: Chen Y, Gao J, Wang X, Lu H, Zheng Y and Ren Q (2024) Serum folate levels and risk of metabolic dysfunction-associated steatotic liver disease: results from a cross-sectional study and Mendelian randomization analysis. Front. Nutr. 11:1437183. doi: 10.3389/fnut.2024.1437183
Edited by:
Marija Takic, University of Belgrade, SerbiaReviewed by:
Ricardo Adrian Nugraha, Airlangga University, IndonesiaSaid Taharboucht, Saad Dahlab University, Algeria
Copyright © 2024 Chen, Gao, Wang, Lu, Zheng and Ren. This is an open-access article distributed under the terms of the Creative Commons Attribution License (CC BY). The use, distribution or reproduction in other forums is permitted, provided the original author(s) and the copyright owner(s) are credited and that the original publication in this journal is cited, in accordance with accepted academic practice. No use, distribution or reproduction is permitted which does not comply with these terms.
*Correspondence: Qian Ren, bGR5eV9yZW5xaWFuQGx6dS5lZHUuY24=