- 1College of Food Science and Engineering, Jilin Agricultural University, Changchun, China
- 2Jilin Provincial Center for Disease Control and Prevention, Changchun, China
Aging brings about physiological dysfunction, disease, and eventual mortality. An increasing number of studies indicate that aging can easily lead to dysbiosis of the gut microbiota, which can further affect digestion, nerves, cognition, emotions, and more. Therefore, gut bacteria play an important role in regulating the physical functions of aging populations. While saponins, the primary components of American ginseng, are frequently utilized for treating common ailments in the elderly due to their potent antioxidant properties, there is a scarcity of comprehensive studies on aging organisms. This study focused on 18 month old aging mice and investigated the effects of single intervention and combined intervention of Rb1 and Re, the main components of Panax quinquefolium saponins, on the gut microbiota of aging mice. High throughput 16s RNA gene sequencing analysis was performed on the gut contents of the tested mice, and the results showed that Rb1 and Re had a significant impact on the gut microbiota. Rb1, Re, and Rb1 + Re can effectively enhance the diversity of gut microbiota, especially in the combined Rb1 + Re group, which can recover to the level of young mice. Re can promote the abundance of probiotics such as Lactobacillus, Lactobacillaceae, and Lactobacillus, and inhibit the abundance of harmful bacteria such as Enterobacteriaceae. This indicates that the intervention of Rb1, Re, and Rb1 + Re can maintain the homeostasis of gut microbiota, and the combined application of Rb1 + Re has a better effect. The relationship between aging, brain gut axis, and gut microbiota is very close. Saponins can improve the gut microbiota of aging individuals by maintaining the balance of gut microbiota and the normal function of the brain gut axis, enabling the body to achieve a gut microbiota homeostasis closer to that of young healthy mice.
1 Introduction
American ginseng (Panax quinquefolius) is a perennial herb belonging to the Ginseng genus in the Ginkgoaceae family (1). Indigenous to the deciduous woodlands of eastern North America, it has been widely cultivated worldwide (2). Extensive research has demonstrated the efficacy of Panax quinquefolius in regulating blood pressure, boosting immunity, reducing blood glucose levels, mitigating inflammation, and safeguarding the cardiovascular system, owing to its antioxidant properties (3). Saponins, the pivotal constituents of Panax quinquefolius, play a crucial role in positive immunomodulation, oxidative regulation, blood glucose control, and anti-radiation effects (4). Among the key saponin components, Rb1, Re, Rd, Rg1, and Rb3 collectively constitute over 70% of the total saponin content (5). Some of these components have been revealed to inhibit cancer cell growth, act as antioxidants, lower blood glucose levels, activate estrogen receptors, and enhance memory, among other beneficial effects (6). Furthermore, the saponin composition of Panax quinquefolius closely resembles that of ginsenosides, exhibiting similar efficacy in various aspects. As a result, Panax quinquefolius is often compared to traditional ginseng (7). Aging is an unavoidable biological phenomenon characterized by deteriorative changes in an individual’s functionality as they age. Ultimately, these changes compromise the organism’s ability to sustain normal functioning, culminating in eventual mortality (8). Numerous studies have indicated that the principal component of ginseng has the potential to extend lifespan, as demonstrated in models like Drosophila and Cryptobacterium hidradii nematodes (9). Additionally, the Panax quinquefolius extract CVT-E002 has been shown to prolong the lifespan of infants and juvenile mice with leukemia (10). Ginsenosides, non-starch polysaccharides from ginseng, and certain phenolic compounds exhibit antioxidant effects, with even ginseng leaf essential oil demonstrating free radical scavenging activity (11). The antioxidant properties of ginseng extracts have captured the interest of researchers, revealing that these extracts can shield cells from AA+ iron-induced reactive oxygen species (ROS) production and mitochondrial damage by activating AMP-activated protein kinase (AMPK). At the molecular level, ginseng is capable of initiating LKB1-dependent AMPK activation, contributing to enhanced cell survival (12).
The gut microbiota, including a large number of various microorganisms residing in the gastrointestinal tract, plays an important role as a component of the host system in maintaining the stability of the gastrointestinal environment and regulating host metabolism. Research has shown that each individual has at least 160 taxonomic species, belonging to 1,000–1,150 popular bacteria; their collective genome (“microbiota”) contains at least 100 times the number of genes in the genome (13). The composition of microbial communities develops in the first few years of life and is relatively stable in adulthood (14). The gut microbiota may be influenced by host genetics, health, diet, aging, and probiotics (15, 16). The changes in gut microbiota composition related to aging may be related to many diseases and disorders in the elderly (17). Recent studies have also shown that gut microbiota, especially certain specific strains, may enhance cell-mediated immunity in host animals, thereby altering age-related immune aging (18). As is well known, aminobutyric acid (GABA) is the most important inhibitory factor in the central nervous system of humans and other mammals, participating in neurotransmitters and metabolic processes in the brain. Research has shown that the levels of GABA in the gut are closely related to those in the central nervous system, and bifidobacteria and lactobacilli in the gut are involved in the production of GABA. Therefore, bifidobacteria and lactobacilli in the gut are closely related to some brain dysfunction, such as depression, cognitive impairment, synaptic dysfunction, etc. (19). Compared to fecal samples from young subjects, the proportion of Clostridium, Streptococcus, and Staphylococcus in fecal samples from elderly individuals has increased (20), which are commonly believed to have adverse effects on the body.
In addition, changes in the gut microbiota associated with aging can damage the host’s immune homeostasis, leading to pro-inflammatory responses, the formation of a malignant inflammatory cycle, and the possible occurrence of age-related diseases (21). Slowing gastrointestinal peristalsis or constipation in elderly people can reduce the proportion of intestinal bacteria excreted from the body, leading to the accumulation of pathogenic microorganisms in the intestine and causing intestinal bacterial overload (22). Aging can also affect the motor and perceptual functions of the gastrointestinal tract (23). The increase in age will be accompanied by changes in gastrointestinal function, such as decreased esophageal peristaltic pressure leading to swallowing difficulties and reduced colon motility leading to constipation (24), which will further increase the burden on the intestines of elderly people. The study by Kim et al. (25) suggests that age-related intestinal homeostasis disorders in model organisms can trigger congenital immune responses and chronic low-grade inflammation, leading to degenerative diseases and unhealthy aging. The study of gut microbiota and the gut brain axis, as well as their neural related functions, has received widespread attention in recent years. Therefore, the homeostasis of gut microbiota is closely related to the recovery of cognitive function in aging mice. The imbalance of gut microbiota is a key trigger for many diseases, including digestive system diseases directly associated with it, cardiovascular and neurological diseases indirectly associated with it. Therefore, having a healthy gut not only enriches the variety of gut microbiota, but also has a positive effect on the elderly population.
According to the previous research results of our team, saponins have a positive effect on aging mice and can improve the adverse effects of aging in multiple aspects such as immunity and cognition. Meanwhile, previous studies have found that dysregulation of the gut microbiota is closely related to neurological disorders such as Alzheimer’s Disease (AD), Parkinson’s Disease (PD), Huntington’s Disease (HD), and multiple sclerosis (MS) (26, 27). We do not know whether saponins have a positive effect on the gut microbiota of aging mice. Therefore, this study used 16s RNA sequencing technology to analyze the intestinal contents of mice, in order to reveal the effects of saponin monomer Rb1 and Re intervention alone and in combination on the intestinal microbiota of mice. In short understanding the changes in gut microbiota in aging mouse models is of great significance for understanding the mechanism of saponin action.
2 Materials and methods
2.1 Materials and reagents
Pharmaceutical grade saponins Rb1(CDCV-ASB-00007192-101,purity≥98%) and Re(CAS:52286–59-6,purity≥98%) were purchased from the Natural Medicine Chemistry Laboratory of Jilin University and stored at 4°C according to the drug instructions. Aging model mice (C57BL/6,18 months old) were purchased from Changchun Yishi Experimental Animal Technology Co., LTD., and raised in Jilin Provincial Health Inspection Center (approved by the Experimental Animal Management Committee of Jilin Provincial Center for Disease Control and Prevention (JCDC [2023] No. 1)). DNA extraction kit, Phusion Flash High Fidelity PCR Premix, DNA Library Construction Kit, Purchased from ThermoFisher.
2.2 Grouping and administration of experimental mice
Forty healthy male C57BL/6 mice (18 months old, weight 28-32 g) were selected. The aging mouse models were put into the mouse house for 7 days, then they were divided into 4 groups on average, with 10 mice in each group. They were divided into Aged model group, Rb1 administration group (Rb1), Re administration group (Re) and Rb1, Re administration group (Rb1 + Re). The dosage was formulated according to the optimal concentration of the team’s previous trial, that is, the dosage of Rb1 was 30 mg/kg, and the dosage of Re was 15 mg/kg. In addition, 10 6-month-old male healthy C57BL/6 mice (weight 20-25 g) were used as a control group (Col).
The mice were raised in Jilin Provincial Health Inspection Center in a constant temperature (21 ± 2°C) and 50% humidity environment, and the light was set to 12 h dark light cycle, and they were free to drink and eat. The saponin monomer drug was dissolved in distilled water according to the experimental design, and the mice were given the drug through drinking water every day. Control and aging groups drank distilled water. Before feeding, each mouse was starved for 12 h and then numbered and weighed. After 8 weeks of continuous feeding, each mouse was starved for 12 h, and then weighed. The mice were killed 20 h after the last administration and the relevant indexes were detected (28).
2.3 Laboratory mouse anatomy
The mouse model was anesthetized with 0.5% pentobarbital sodium. After anesthesia, all mice were subjected to a cesarean section experiment, and the intestinal segments from the jejunum to the ileum were cut off. Then, a scraper was used to sample the contents of the duodenum. All samples were immediately frozen in a−80°C freezer after collection. The remaining biological tissues after dissection were treated harmlessly.
2.4 16s rRNA gene sequence amplification
This study used the V3-V4 region of the 16s rRNA gene for detection. The primers used for amplification were the universal 520F universal primer (5‘- AYTGGGYDTAAAGNG-3’) and the 802R universal primer (5‘- TACNVGGTATCTAATCC-3’). Amplification was performed using Taq high fidelity enzyme. The PCR amplification system is shown in the Table 1 as follows:
PCR amplification conditions: pre denaturation at 98°C for 30 s; Then deform at 98°C for 30 s, anneal at 50°C for 30 s, and extend at 72°C for 30 s, a total of 25 cycles; Finally, extend at 72°C for 5 min. The PCR product gel was recovered and subjected to 16s rDNA V3-V4 sequencing by Tianjin Nuohe Zhiyuan Biotechnology Co., Ltd.
2.5 Sequencing data analysis
2.5.1 Data quality control
After data sequencing is completed, raw data is obtained, which includes sequences with lower sequencing quality, sequences with splices, and sequences with a higher proportion of N. Raw data must first go through data quality control to obtain high-quality data before proceeding with subsequent analysis. In this study, FastQC 0.11.9 (29) was used for data quality control under the following conditions: (1) Remove reads containing adapters; (2) Remove reads with a N content ratio greater than 10%; (3) Remove reads containing all A bases; (4) Remove low-quality reads (alkaline bases with a quality value Q ≤ 20 account for more than 50% of the entire reads). The data after quality control is clean data.
2.5.2 Sequence assembly and OTU classification
Use Qiime 2 (30) software to assemble all clean reads, divide them into an Operational Taxonomic Unit (OTU) based on 97% similarity, and then select the longest sequence of each class as the representative sequence. Then, use Qiime 2 to perform Blast alignment of all OTU sequences with Nr and Nt databases to obtain sequence annotation information for each OTU. Next, remove all sequencing samples with OTU abundance ratios below 0.001% to obtain effective OTUs. Then all obtained OTUs are sorted in descending order of abundance.
2.5.3 Alpha diversity index analysis
Alpha diversity refers to the diversity within a specific region or ecosystem, which is a comprehensive indicator reflecting richness and evenness. This study analyzed the Chao1 index, Shannon index, ACE index, and Simpson index in Alpha diversity. Chao1 is commonly used in ecology to estimate the total number of species; The ACE index can estimate the actual number of species present in a community, and the larger the ACE index, the higher the richness of the community; The Shannon index comprehensively evaluates the richness and evenness of communities. The higher the Shannon index value, the greater the diversity and complexity of information in the system (31). Comprehensively evaluate the Alpha diversity index in each group to evaluate the diversity and evenness of gut microbiota in each group.
2.5.4 Beta diversity index analysis
Beta diversity analysis is a comparison of diversity between different ecosystems, which is the rate of change in species composition along environmental gradients or between communities, used to represent the response of biological species to environmental heterogeneity. In this study, the Beta diversity analysis used the PCoA index to evaluate the similarity or dissimilarity of the study sample community composition.
2.5.5 Species composition analysis
Based on the annotation information, this study analyzed the community structure of each observed sample at different classification levels. When comparing the community structure analysis of multiple samples together, one can also observe their changes. Here, we use bar charts to visualize the species composition of different groups, and use bar charts to visualize the bacterial content of the same sample (or unified group). This study analyzes the species composition of each group from five levels: phylum, class, order, family, genus, etc.
2.5.6 Analysis of differences in species composition
In this study, species composition differences were analyzed using LEfSe (32). LDA Effect Size analysis can achieve comparison between multiple groups, and internal subgroup comparison analysis can be carried out to identify species with significant differences in abundance between groups. According to its analysis principle, it can be divided into three steps. The analysis principle is as follows: (1) Firstly, the parameter test ANOVA test is used in multiple group samples to detect species with significant differences in abundance between different groups, and the threshold is set to 0.05; (2) Then, the significantly different species obtained in the previous step were subjected to intergroup difference analysis using Wilcoxon rank sum test, with a threshold set at 0.05; (3) Finally, linear discriminant analysis was used to perform dimensionality reduction analysis on the data, and the influence of species with significant differences (i.e., LDA score) was evaluated. In this study, the LDA threshold was set to 3.5.
2.6 Joint data analysis
Usually, we conduct cluster analysis by studying the community function of gut microbiota, enrich OTUs based on the function of microbial abundance, and display them in the form of heatmaps. Heat maps are drawn using TBTools software. In addition, this study conducted redundancy analysis (RDA) based on significantly correlated microbial environmental factors, revealing the relationship between environmental variables and community species composition through dimensionality reduction. Used to visualize and quantify the association between multiple environmental variables and multivariate response variables (such as species abundance or presence/absence). Then, using JSD distance and Partition Around Medoids (PAM) clustering algorithm for intestinal type analysis, the samples are clustered based on relative species abundance. Use the Calinski Harabasz index to evaluate the results and determine the optimal number of clusters.
2.7 Data processing and analysis
SPSS 19.0 was used for statistical analysis. The two sets of data were directly compared with the practical one-way ANOVA or T-test. Three or more sets of data were compared for analysis using ANOVA. Data are expressed as mean ± standard deviation of no less than 3 biological replicates. The significance level was significant with p < 0.05.
3 Results
3.1 Data quality control results
All sequencing data were subjected to data quality control, and the results showed that the data quality in this study was good. Offline data is obtained by removing barcode and primer and concatenating them to obtain raw tags. Raw tags are further removed from chimeras and short sequences to obtain high-quality clean tags. The results show that the proportion of clean data is higher than 96.11%, and the average proportion of data is 98.16%.
The specific results are shown in the Table 2.
3.2 Analysis of dilution curve and rank abundance curve
Based on sequencing data, draw dilution curves to compare the species richness of different sequencing samples, and can also be used to demonstrate whether the sequencing data of the samples is sufficient. The dilution curve results show that the sequencing data tends to plateau after exceeding 20,000, reaching a plateau period, indicating that the sequencing data volume for each sample is sufficient (Figure 1A).
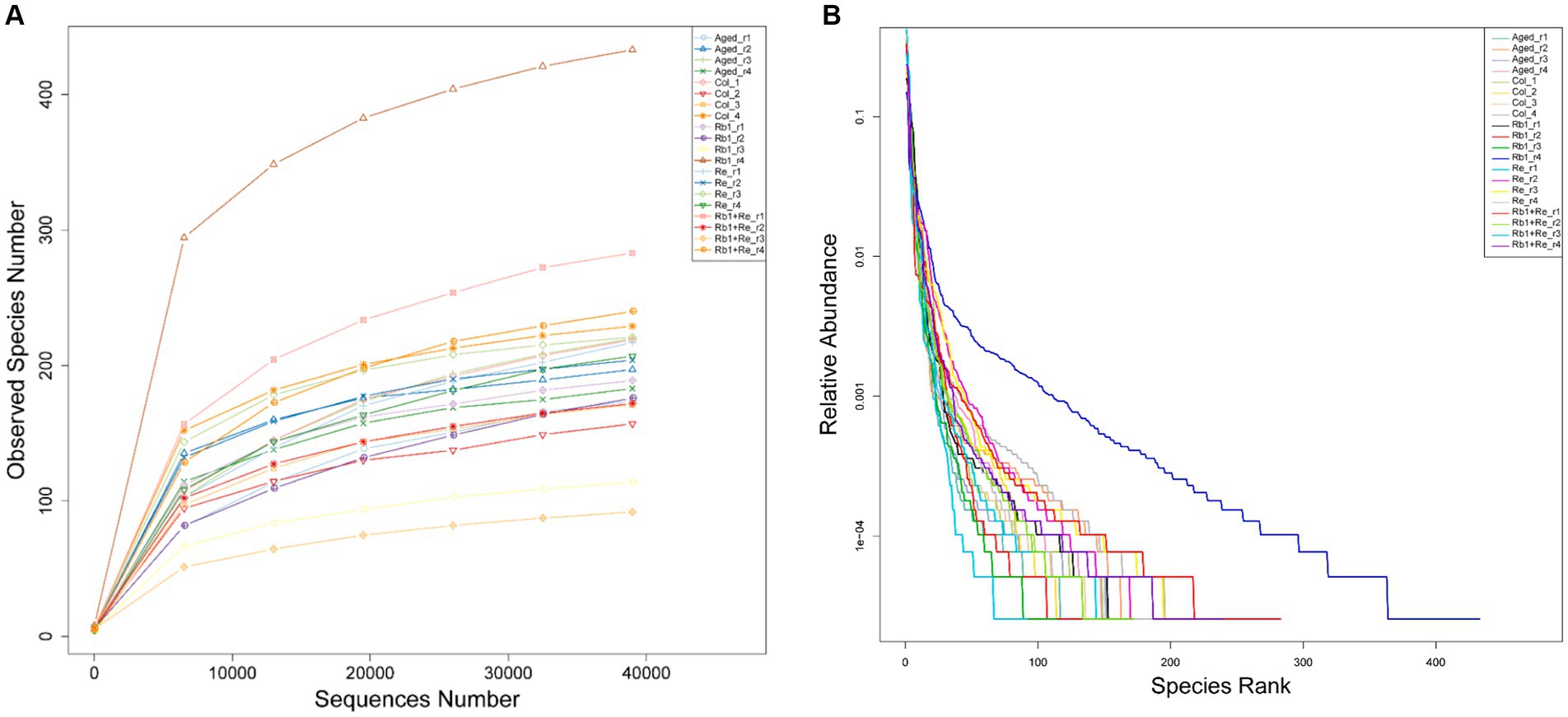
Figure 1. 16s rarefaction curves and the rank-abundance distribution. (A) Within-sample richness, estimated by OTU analysis of 16S sequences with QIIME. The rarefaction curves are computed by sampling the number of 16S sequences indicated on the x-axis and counting the number of distinct observed species, plotted on the y-axis. The shaded areas around the lines represent the 95% confidence intervals. None of the confidence intervals overlap, suggesting that each group significantly differs from the other two even when only 10,000 sequences are considered. (B) The horizontal axis in the figure is the serial number of each OTU, and the vertical axis is the relative abundance count of bacteria in this category.
The Rank Abundance curve can be used to explain two aspects of diversity, namely species abundance and community evenness. In the horizontal direction, species richness is reflected by the width of the curve, and the higher the species richness, the larger the range of the curve on the horizontal axis; The smoothness of the curve reflects the evenness of species in the sample, and the flatter the curve, the more uniform the distribution of species in the community (Figure 1B) (33, 34).
In summary, the dilution curve and Rank Abundance curve analysis results show that all data are sufficient, and the diversity richness and diversity of OTUs in the sample are moderate, which are consistent with the characteristics of general gut microbiota research. Therefore, this sequencing data can be well used for the analysis of gut microbial diversity.
3.3 Alpha diversity analysis
The results of Alpha diversity analysis showed that there was no significant difference in Simpson index among the groups, but Chao1 and Shannon indices showed that the Rb1, Re, and Rb1 + Re groups had higher total species numbers, and the microbial diversity of the Rb1 + Re group returned to the Col level; In addition, there was no significant difference in ACE values between the Col, Rb1, Re, and Rb1 + Re groups, but they were all significantly higher than the Aged group. In summary, the administration of Rb1 and Re alone can significantly increase the abundance of intestinal bacteria, while the administration of Rb1 and Re has a more significant effect. This indicates that saponins Rb1 and Re have a significant impact on gut microbiota, and their combined use is crucial for the restoration of gut microbiota diversity (Table 3).
3.4 Beta diversity analysis
The Beta diversity analysis results show that the samples within each group can be clustered together. Meanwhile, this study found that the samples from the Col group, Re group, and most of the Rb1 group were distributed in the second, third, and fourth quadrants. The Rb1 + Re and most of the Aged group samples are mostly distributed in the first quadrant. This indicates that the Col group, Re group, and Rb1 group may have similar diversity; the diversity of Rb1 + Re group and Aged group is relatively similar (Figure 2).
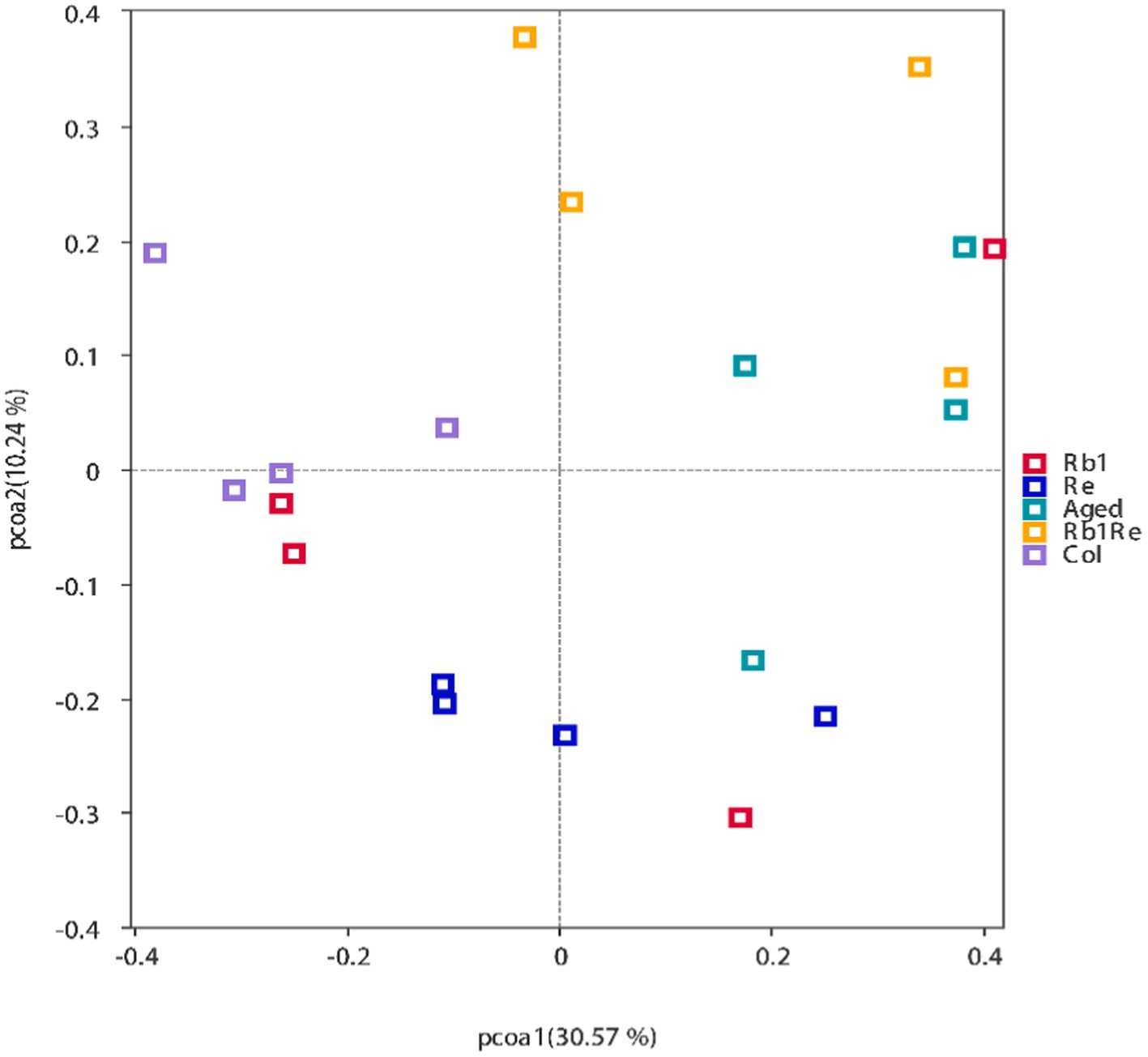
Figure 2. Principal Coordinates Analysis (PCoA) results showing the relatedness of microbial communities in the different samples. The PCoA plots were constructed with the unweighted UniFrac PCoA method.
3.5 Bar chart of relative species abundance
3.5.1 Differences in gut microbiota abundance at the phylum level
This study analyzed the classification of gut bacteria at the phylum level and the abundance of gut bacteria at the genus level, respectively. Firstly, this study analyzes gut microbiota at the phylum level. The results showed that Firmicutes, Proteobacteria, Teneriicutes, Actinobacteria, Bacteroidetes, Cyanobacteria, Fusobacteria, Acidobacteria, and Candida were among the top 10 bacteria. In the Col, Rb1, Re, and Rb1 + Re groups, Firmicutes had the highest abundance; In the aging model group, Proteobacteria had the highest abundance of bacteria. In addition, the relative abundance of Tennericutes was very low in the Aged and Rb1 + Re groups, but higher in the Col, Rb1, and Re groups.
In addition, we found a significant increase in the relative abundance of Actinobacteria in the Rb1 and Re intervention groups, but a lower abundance in the Rb1 + Re group (Figure 3A).
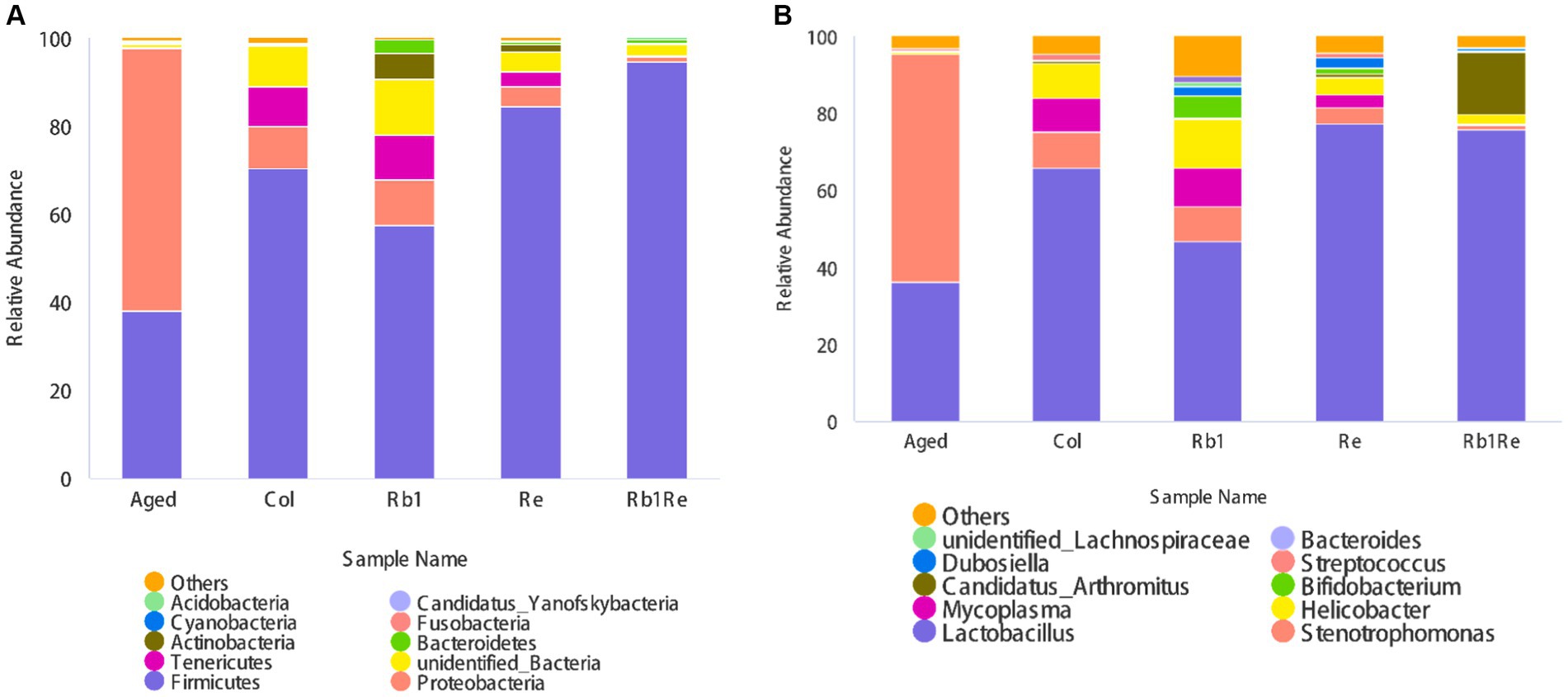
Figure 3. Relative abundance of gut microbiota in different groups. (A) Relative abundance of total intestinal microorganisms in different groups at the phylum level. (B) Relative abundance of total intestinal microorganisms in different groups at the genus level. The abscissa is grouping, and the ordinate is relative abundance (%).
3.5.2 Differences in gut microbiota abundance at the genus level
At the genus level, Lactobacillus, Stenotrophomonas, Helicobacter, Candidatus, Bifidobacterium, Dubosella, Streptococcus, unclassified Lachnospiraceae, and Bacteroides are among the top 10 bacteria in terms of relative abundance.
At the phylum level, Lactobacillus is the most abundant gut bacterium among the Col, Rb1, Re, and Rb1 + Re groups. However, in the aging model group, the abundance of Lactobacillus was lower than that of Stenotrophomonas, ranking second. The results of this study showed that Rb1 or Re intervention alone significantly reduced the abundance of Stenotrophomonas (p < 0.05). The effect of simultaneous administration is more significant.
In addition, there is a higher proportion of Helicobacter species in the Col group, which may cause gastrointestinal disorders. However, the abundance of Helicobacter in the aging model is actually lower. However, after administration of Re or Rb1 + Re, the abundance of Helicobacter species decreased compared to the Col group, but remained higher than Aged. In addition, this study also found a significant increase in the abundance of Clostridium difficile (Candidatus Arthromitus) in the Rb1 + Re group, while the abundance of Clostridium difficile (Candidatus Arthromitus) was lower in other groups. Candidatus Arthromitus is a collective term for a large group of Gram positive, anaerobic, or microaerophilic crude Bacillus bacteria, mainly found in soil, human and animal intestines, and spoilage, but most are not pathogenic. The co administration of Rb1 + Re promotes a rapid increase in the abundance of Clostridium difficile, indicating that the co intervention of Rb1 + Re may provide a more suitable living environment for Clostridium difficile. This change may be directly caused by changes in organic acids in the intestine. The co administration of Rb1 and Re, with a larger dosage and longer course of treatment, may lead to changes in organic acids in the intestine. Lactobacillus and Bifidobacterium are recognized probiotics.
The results of this study showed that after treatment with Rb1 or Re, the abundance of Lactobacillus significantly increased, reflecting the effect of saponin monomers on intestinal probiotics. In addition, the intervention of Rb1 significantly increased the abundance of Bifidobacterium, while the intervention of Re had a certain promoting effect on Bifidobacterium, but it was far less effective than that of Rb1. This suggests that Rb1 may provide a more suitable environment for gut bifidobacteria in aging mice. The simultaneous use of Rb1 and Re has limited impact on the abundance of Bifidobacterium (Figure 3B).
3.6 LEfSe analysis
This study used LEfSe analysis to screen for bacterial species with significant differences in abundance between groups. The results showed that in the Aged group, there was a significant difference in abundance among the most diverse bacterial species, while Rb1 had the least diversity among the other groups (Figures 4A,B). Meanwhile, this study found no significant differences in bacterial species between the Col group and the other groups. Lactobacillales, Lactobacillaceae, and Lactobacillus genera have a significant impact in the Re group, indicating that Re may be one of the main factors causing changes in the abundance of lactobacilli in the gut microbiota. Simultaneously using Rb1 and Re to intervene in an aging mouse model resulted in a significant increase in gut Firmicutes. In addition, the abundance of Vibrio in the Rb1 + Re group significantly increased compared to other groups. Vibrio genus contains a large number of pathogenic bacteria, such as classical and Elto biotypes of Vibrio cholerae and Vibrio parahaemolyticus. Higher levels of Vibrio may cause diarrhea. This indicates that the combined use of Rb1 and Re may have certain negative effects on the gut of aging individuals. In this study, Proteobacteria in the Aged group had the highest abundance among all groups. As is well known, Proteobacteria is the largest phylum of bacteria, including many pathogenic bacteria such as Escherichia coli, Salmonella, Vibrio cholerae, Helicobacter pylori, and other famous species. After administration of Rb1 or Re, the abundance of Proteobacteria significantly decreased, indicating that Rb1 or Re can significantly inhibit the abundance of Proteobacteria related pathogenic bacteria in the intestine. However, the simultaneous use of the two may have new issues.
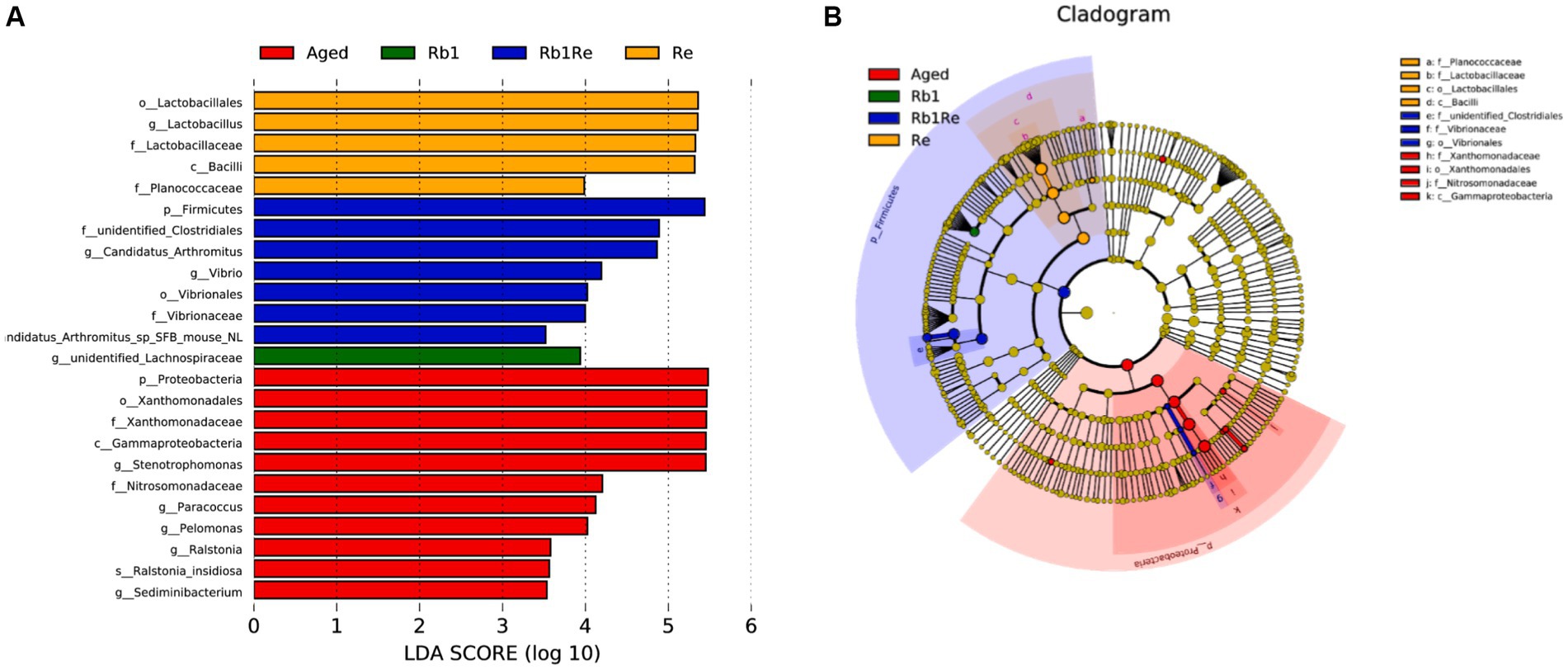
Figure 4. LEfSe analysis. (A) Bacterial biomarkers found by linear discriminant analysis effect size (LEfSe). (B) Taxonomic cladogram derived from LEfSe analysis of 16S sequences. Taxa meeting a linear discriminant analysis significant threshold >3.5 are shown. c, class level; f, family level; g, genus level; o, order level; p, phylum level; LDA, Linear discriminant analysis.
3.7 OTU functional clustering analysis
According to the clustering results, it was found that the Aged model group had a higher abundance of animal parasites or symbionts. Based on the results, the study identified genes involved in the parasite’s survival and adaptation to its host, as well as their potential to cause disease. In the case of symbiotes, functional enrichment analysis can reveal the unique metabolic pathways and processes of these organisms, and contribute to the symbiotic relationship with the host. In the aging model, there may be a higher risk of diseases caused by parasitic organisms, while in the Col group, this risk is significantly reduced. Furthermore, after intervention with Rb1 and Re, this risk is further reduced. Especially with Re intervention, the risk of diseases caused by parasitic organisms was significantly reduced, and there was a significant difference compared to the aging group and the control group (Figure 5). In addition, this study found that OTUs related to human gut and mammal gut have more similar abundances in the Col, Rb1, Re, and Rb1 + Re groups, forming a significant difference from the Aged group. Therefore, it can be inferred that after Re and Rb1 intervention, the gut microbiota pattern is closer to that of the Col group, greatly improving the gut microbiota status of the aging model.
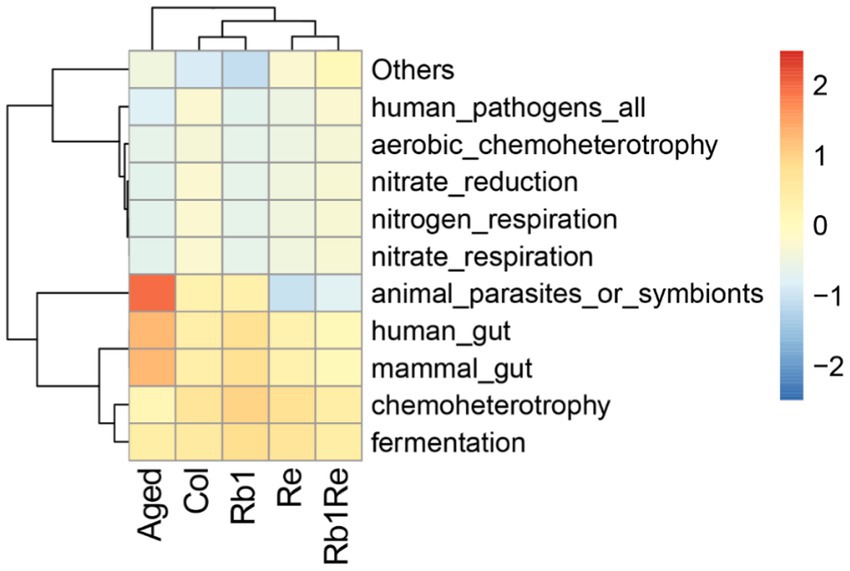
Figure 5. OTU function cluster. Predicting microbial community functions. The redder the color indicates higher abundance of the microbial community under that function, while the bluer the color indicates lower abundance of the microbial community under that function.
3.8 Microbial-environmental factor redundancy analysis
Aging negatively affects both motor and cognitive performance. In recent years, the study of gut microbiome and the gut-brain axis and its neural functions has received extensive attention, so the homeostasis of gut microbiome is closely related to the recovery of cognitive function in aging mice. Studies have found that the imbalance of intestinal microecology is associated with Alzheimer’s Disease (AD), Parkinson’s Disease (PD), Huntington’s Disease (Huntington’s disease), and Alzheimer’s disease. HD is closely related to neurological disorders such as Multiple Sclerosis (MS). The team previously assessed the mice’s ability to adapt to new environments through open field and water maze experiments. Among them, the water maze experiment studied the learning and memory ability of mice. Saponins have obvious restorative effect on the degraded motor ability and spatial cognitive ability of aging individuals. The exercise ability of aging mice decreased significantly compared with control group. The supplement of Re may be helpful to restore part of the motor ability of aging mice to a certain extent. Re or Rb1 + Re intervention can reduce the rest time of aging mice and increase the slow movement time, which reflects the effectiveness of Re. However, in the case of rapid exercise, neither Re, Rb1 nor Re + Rb1 interventions showed a significant effect. Aging mice had spatial learning and memory impairment, and the escape latency of mice after Rb1 and Re intervention was significantly reduced compared with that of the aging model group, which indicated that Rb1 or Re intervention alone could effectively improve the cognition of the aging model. In addition, simultaneous intervention with Rb1 and Re had better effects. The exploration time of the aging mice platform quadrant in Rb1 or Re treatment group was increased compared with that in aging group, but it was significantly lower than that in control group. The simultaneous intervention of Rb1 and Re did not produce a significant difference from the intervention alone, suggesting that Rb1 and Re do not have a superimposed effect on neurological recovery. Rb1 and Re intervention can effectively improve the number of synapses in the hippocampus, and Rb1 had a better effect than Re. However, simultaneous intervention with Rb1 + Re did not show a greater effect. Rb1 or Re intervention could significantly improve the morphology of microglia, which were mostly round, but the number of lysosomes did not change significantly. Therefore, Rb1 and Re intervention alone or Rb1 + Re intervention together can improve the hippocampus microglia, but cannot alleviate the influence of lysosomes, and the combined intervention of Rb1 and Re cannot form a superimposed effect.
Through redundancy analysis, the results showed that Aged group was positively correlated with inactivity duration, while Col group was negatively correlated with inactivity duration, indicating that Aged group mice had limited exercise ability and their physical function was far lower than Col group. However, the exercise ability of aging models was significantly improved after Rb1 or Re administration, which was positively correlated with the slow exercise duration in the Re and Rb1 + Re groups, indicating that the administration of Rb1 or Re could improve the exercise ability of aging mice, but could not achieve the same exercise level as Col. The platform quadrant residence time was negatively correlated with Col and each drug treatment group (Figure 6A).
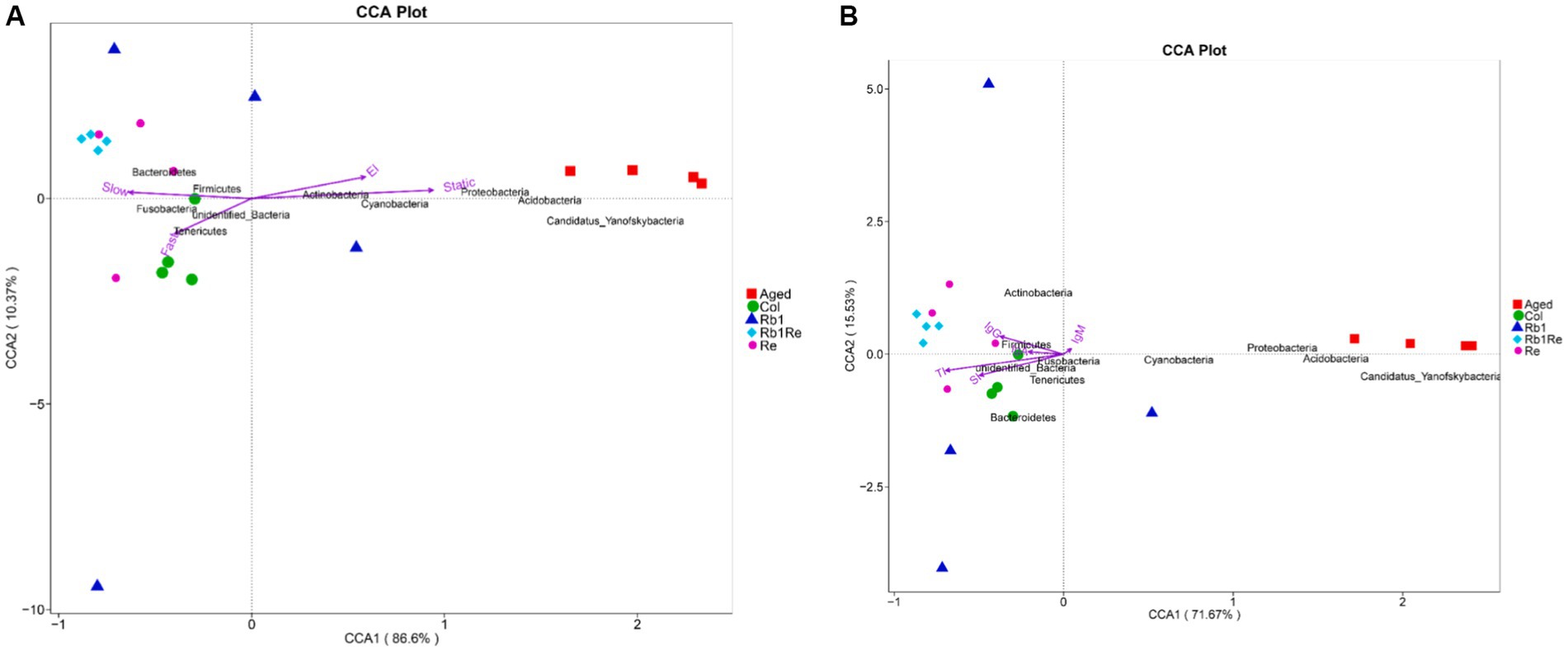
Figure 6. Multivariate redundancy analysis (RDA) of the microbiota composition. (A) Exercise and cognition. (B) Immune indexes. RDA was performed using Canoco 5.0. Taxonomic composition at the genus level was used as response data, iron level as explanatory variable, time point and experiment as supplementary variables. The variation explained by the ordination axis is significantly higher than random (permutation test).
In addition, this study found that the abundance of Proteobacteria, Acidobacteria, Cyanobacteria and Candidatus Yanofskybacteria was positively correlated with quiescence time and platform quadrant residence time. Tenericutes were positively correlated with rapid motor ability. The abundance of Bacteroidetes and Firmicutes is related to slow motion. Although there is no direct evidence that these bacteria are involved in the regulation of motor and cognitive ability in aging models, it can at least be suggested that they are associated with exercise and cognition in aging models. These changes may be caused by Rb1 and Re by regulating the gut-brain axis.
By analyzing the relationship between intestinal microorganisms and immune indexes, we found that the Aged group was rich in Cyanobacteria, Proteobacteria, Acidobacteria and Candidatus Yanofskybacteria. Its abundance was inversely proportional to thymus index and spleen index (Figure 6B). In addition, this study found that Firmicutes were in direct proportion to IgG, and Rb1 + Re group had a higher abundance of Firmicutes. Therefore, this study suggests that Firmicutes may be related to immune indexes in aging models.
3.9 Microbial interaction network analysis
The interaction network analysis of the major intestinal microbes showed that the interactions of these microbiota were divided into four subgroups. Among them, the largest subgroup contains 18 genera, and the interaction is strong. According to the gut microbial abundance analysis, the abundance of Dubosiella increased significantly after Rb1 and Re intervention, while the abundance of unidentified_Ruminococcaceae decreased significantly after Rb1 and Re intervention. However, the abundance of these 18 genera was lower than 1%, which was a non-dominant bacterial group. The dominant bacterial group forms the second largest interaction network subgroup, in which Lactobacillus has a high abundance in Col and a low abundance in Aged, but the abundance of Lactobacillus also increases to the same level of Col after Re intervention. In addition, the interaction between Lactobacillus and Stenotrophomonas and Sphingomonas is strong, which may be that the increase of Lactobacillus competes with Stenotrophomonas and Sphingomonas. Stenotrophomonas and Sphingomonas were inhibited in abundance (Figure 7).
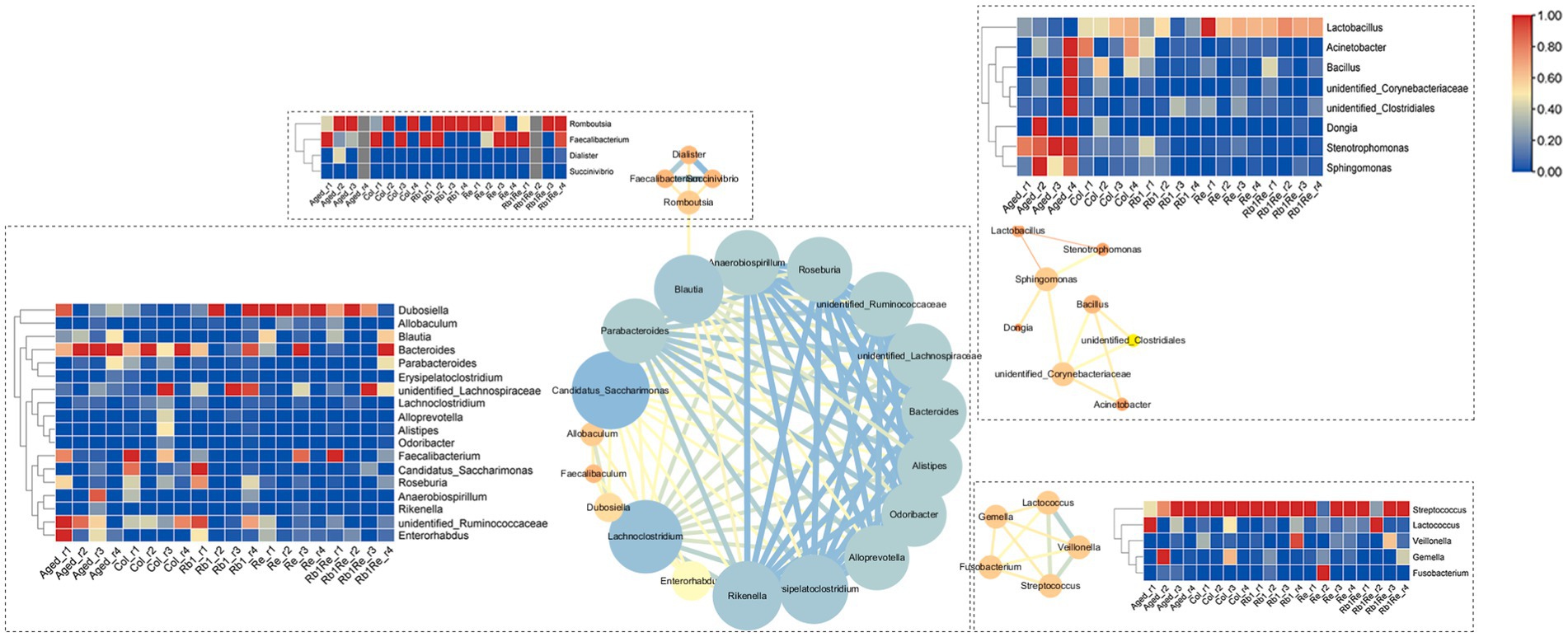
Figure 7. Analysis of microbial community interaction network. The redder the color is, the higher the abundance of the corresponding microbiota is, and the bluer the color is, the lower the abundance of the corresponding microbiota is.
4 Discussion
Rezaeiasl et al. showed that supplementation with Lactobacillus can have a positive impact on the learning ability of the rat model of Alzheimer’s disease (35). Lactobacillus also improves the gut microbiota in Drosophila melanogaster Alzheimer’s disease models (36). So the increase in the abundance of Lactobacillus from Rb1 and Re reflects its positive effect on the aging model. In addition, Rb1 and Re suppressed the number of enterobacteriaceae bacteria, particularly Proteobacteria, and increased the number of Lactobacillus and Bifidobacterium. In addition, previous studies have shown that Lactobacillus can inhibit the expression of TNF-α and IL-1β in macrophages, thereby alleviates colitis (37). Bifidobacterium longum CH57 alleviates colitis by inhibiting the NF-κB signaling pathway and TNF-α expression (38). Lactobacillus plantarum C29 improves colitis in older mice by inhibiting NF-κB signaling (39). Other studies have shown that Lactobacillus casei DN-114001 inhibits DSS induced colitis by inhibiting intestinal membrane permeability and NF-κB activation (40).Some probiotics have also been shown to restore gut microbiota composition and fecal lipopolysaccharide levels in mice with colitis. These findings suggest that increased probiotics can reduce colitis symptoms by inhibiting NF-κB activation and restoring disrupted gut microbiota composition. In addition, these probiotics significantly inhibited blood lipopolysaccharide and TNF-α levels in mice with TNBS induced colitis. Therefore, the intervention of Rb1 and Re in this study led to an increase in the number of intestinal probiotics, and the impact on the aging model may be multifaceted, requiring more experimental verification.
The aging process is accompanied by a gradual decline in body structure and function. Older adults often experience a number of health-related problems, such as insomnia, anxiety, depression, and cognitive decline. These problems may be related to changes in gut microbes and disturbances in the brain-gut axis. The brain-gut axis refers to the interaction between the digestive tract and the central nervous system. Gut microbes can influence neurotransmission and function in the brain by secreting metabolites such as short-chain fatty acids and neurotransmitters. The nervous system influences the growth and metabolism of intestinal microbes by regulating the movement and secretion of the gut. This two-way interaction is important for the health of the human body.
According to the results of this study, there may be a high risk of diseases caused by parasitic organisms in the aging model, and the risk is further reduced in the Col group and Rb1 + Re intervention group, indicating that the main components of saponins can significantly improve the intestinal microbiota status of the aging model. In addition, this study found that the abundance of Proteobacteria, Acidobacteria, Cyanobacteria and Candidatus Yanofskybacteria was positively correlated with quiescence time and platform quadrant residence time. Tenericutes were positively correlated with rapid motor ability. The abundance of Bacteroidetes and Firmicutes is related to slow motion. These changes may be achieved through the brain-gut axis, but this requires more work to prove one by one.
Gut microbiota is a complex microecosystem in the host body, and its stability is closely related to body health (41). The gut microbiome composition and the communication between the microbiome and the brain may change with age, and the microbiome composition of older people over 65 years of age is significantly different from that of a 9-year-old child (42–44). Intestinal symptoms, loss of motor ability, low immunity, and even the occurrence of neurological diseases such as Parkinson’s disease and Alzheimer’s disease have been observed in aging populations (45–47). Previous studies have confirmed that nervous system diseases are closely related to the composition of intestinal microbiota, which may be related to the fact that bacterial metabolites, such as short-chain fatty acids, can mediate the maturation of microglia (48), and may also affect the clearance of Aβ to affect the effect on neurons (49). Studies have shown that when aging rats are infected with E. coli, its Aβ deposition in intestinal and brain neurons increases, and the proliferation of glial and astrocytes is enhanced (50).
When antibiotics were used to interfere with the gut microbial diversity of Alzheimer’s mice, they showed significant reductions in Aβ deposition and glial cell proliferation (51). Therefore, there is a clear correlation between the gut microbes of mice and their cognitive ability. In this study, Rb1 and Re changed the intestinal microbiome profile of aging mouse models, and Rb1 and Re reduced Proteobacteria, indicating that the abundance of many pathogens, such as Escherichia coli, Salmonella, Vibrio cholerae and Helicobacter pylori, were inhibited by Rb1 or Re. And this has a definite positive effect on the recovery of the nervous system of the aging model. Therefore, Rb1 and Re may influence the nervous system of aging models through the brain-gut axis through the regulation of gut microbes.
In addition, changes in the gut microbiota are not dominated by one or a few dominant microbiota. The interactions of individual microbiota are equally important in the gut microbiome. As we age, the number and diversity of gut microbes may change, causing the balance and interaction of microbes to break down. This imbalance can lead to intestinal inflammation and decreased immune function. At the same time, the increased permeability of the intestinal wall in the elderly may cause bacteria and toxins to enter the blood circulation, triggering an inflammatory response. In this study, a complex interaction regulatory network was formed among all kinds of bacteria. Other studies have shown that diet and probiotic intake can improve the balance of intestinal microbes and help prevent diseases such as cognitive decline and depression in the elderly (52). Combined with the results of this study, the supply of Rb1 and Re may have a positive effect on the abundance of probiotics.
In summary, the relationship between aging, the brain-gut axis, and gut microbes is very strong. By maintaining the balance of intestinal microbes and the normal function of brain-gut axis, saponins can improve the intestinal microbiota of aging individuals, and make the body obtain intestinal microbial homeostasis closer to that of young healthy mice.
5 Conclusion
Rb1, Re, and Rb1 + Re all significantly increased intestinal microbiota diversity, and Rb1 + Re showed a better effect than Rb1 or Re alone. The simultaneous intervention of Rb1 and Re can restore the intestinal microbiota diversity to the level of young mice. The increase in the abundance of probiotics, Lactobacillus and Bifidobacterium after Rb1 and Re intervention reflects its positive effect on aging mice, and its inhibitory effect on the number of enterobacteriaceae, such as Proteobacteria bacteria. Through the prediction of OTU function, the results showed that the risk of parasitic diseases in aging mice was further reduced when Rb1 and Re were simultaneously interfered, and there was a significant difference between the aging group and the control group. The abundance of Proteobacteria, Acidobacteria, Cyanobacteri and Candidatus Yanofskybacteria was positively correlated with stationary time and platform quadrant residence time. The abundance of Tenericutes was positively correlated with the ability to move rapidly. The abundance of Bacteroidetes and Firmicutes is related to slow motion. In addition, the abundance of Cyanobacteria, Proteobacteria, Acidobacteria and Candidatus Yanofskybacteria was negatively correlated with thymus index and spleen index. The abundance of Firmicutes was positively correlated with IgG. Although there is no direct evidence to prove that these microflora are directly involved in the regulation of motor ability, cognitive ability and immunity in aging mice, it can at least show that there is a correlation between gut microflora and movement, cognition and immunity in aging models.
Data availability statement
The raw data supporting the conclusions of this article will be made available by the authors, without undue reservation.
Ethics statement
The animal study was approved by Experimental Animal Management Committee of Jilin Provincial Center for Disease Control and Prevention (JCDC [2023] No. 1). The study was conducted in accordance with the local legislation and institutional requirements.
Author contributions
MS: Data curation, Funding acquisition, Writing – original draft. HF: Methodology, Software, Supervision, Writing – original draft. HL: Formal analysis, Methodology, Resources, Writing – original draft. YZ: Conceptualization, Funding acquisition, Writing – review & editing.
Funding
The author(s) declare that financial support was received for the research, authorship, and/or publication of this article. This study was supported by Jilin Provincial Health Department health committee project (No.2021JC088).
Conflict of interest
The authors declare that the research was conducted in the absence of any commercial or financial relationships that could be construed as a potential conflict of interest.
Publisher’s note
All claims expressed in this article are solely those of the authors and do not necessarily represent those of their affiliated organizations, or those of the publisher, the editors and the reviewers. Any product that may be evaluated in this article, or claim that may be made by its manufacturer, is not guaranteed or endorsed by the publisher.
References
1. Liu, H, Burkhart, EP, Chen, VYJ, and Wei, X. Promotion of in situ Forest farmed American ginseng (Panax quinquefolius L.) as a sustainable use strategy: opportunities and challenges. Front Ecol Evol. (2021) 9:652103. doi: 10.3389/fevo.2021.652103
2. Qiang, B, Miao, J, Phillips, N, Wei, K, and Gao, Y. Recent advances in the tissue culture of American ginseng (Panax quinquefolius). Chem Biodivers. (2020) 17:e2000366. doi: 10.1002/cbdv.202000366
3. Tang, MM, Zhao, ST, Li, RQ, and Hou, W. Therapeutic mechanisms of ginseng in coronary heart disease. Front Pharmacol. (2023) 14:1271029. doi: 10.3389/fphar.2023.1271029
4. Yang, L, Zhang, J, Zheng, K, Shen, H, and Chen, X. Long-term Ginsenoside Rg1 supplementation improves age-related cognitive decline by promoting synaptic plasticity associated protein expression in C57BL/6J mice. J Gerontol A Biol Sci Med Sci. (2014) 69A:282–94. doi: 10.1093/gerona/glt091
5. Mancuso, C, and Santangelo, R. Panax ginseng and Panax quinquefolius: from pharmacology to toxicology. Food Chem Toxicol. (2017) 107:362–72. doi: 10.1016/j.fct.2017.07.019
6. Xu, C, Wang, W, Wang, B, Zhang, T, Cui, X, Pu, Y, et al. Analytical methods and biological activities of Panax notoginseng saponins: recent trends. J Ethnopharmacol. (2019) 236:443–65. doi: 10.1016/j.jep.2019.02.035
7. Lee, JW, Ji, SH, Choi, BR, Choi, DJ, Lee, YG, Kim, HG, et al. UPLC-QTOF/MS-based metabolomics applied for the quality evaluation of four processed Panax ginseng products. Molecules. (2018) 23:2062. doi: 10.3390/molecules23082062
8. Guo, J, Huang, X, Dou, L, Yan, M, Shen, T, Tang, W, et al. Aging and aging-related diseases: from molecular mechanisms to interventions and treatments. Signal Transduct Target Ther. (2022) 7:391. doi: 10.1038/s41392-022-01251-0
9. Hui Wang, SZ, Zhai, L, Sun, L, Zhao, D, Wang, Z, and Li, X. Ginsenoside extract from ginseng extends lifespan and health span in Caenorhabditis elegans. Food Funct. (2021) 12:6793–808. doi: 10.1039/d1fo00576f
10. Miller, SC, Delorme, D, and Shan, JJ. Extract of north American ginseng (Panax quinquefolius), administered to leukemic, juvenile mice extends their life span. J Complement Integr Med. (2011) 8:1–10. doi: 10.2202/1553-3840.1315
11. De Giani, A, Oldani, M, Forcella, M, Lasagni, M, Fusi, P, and Di Gennaro, P. Synergistic antioxidant effect of prebiotic ginseng berries extract and probiotic strains on healthy and Tumoral colorectal cell lines. Int J Mol Sci. (2022) 24:373. doi: 10.3390/ijms24010373
12. Hua, K-F, Chao, AC, Lin, T-Y, Chen, WT, Lee, YC, Hsu, WH, et al. Ginsenoside compound K reduces the progression of Huntington's disease via the inhibition of oxidative stress and overactivation of the ATM/AMPK pathway. J Ginseng Res. (2022) 46:572–84. doi: 10.1016/j.jgr.2021.11.003
13. Qin, J, Li, R, Raes, J, Arumugam, M, Burgdorf, KS, Manichanh, C, et al. A human gut microbial gene catalogue established by metagenomic sequencing. Nature. (2010) 464:59–65. doi: 10.1038/nature08821
14. Mäkivuokko, H, Tiihonen, K, Tynkkynen, S, Paulin, L, and Rautonen, N. The effect of age and non-steroidal anti-inflammatory drugs on human intestinal microbiota composition. Br J Nutr. (2010) 103:227–34. doi: 10.1017/S0007114509991553
15. Sommer, F, and Bäckhed, F. The gut microbiota--masters of host development and physiology. Nat Rev Microbiol. (2013) 11:227–38. doi: 10.1038/nrmicro2974
16. Turnbaugh, PJ, Hamady, M, Yatsunenko, T, Cantarel, BL, Duncan, A, Ley, RE, et al. A core gut microbiome in obese and lean twins. Nature. (2009) 457:480–4. doi: 10.1038/nature07540
17. Guigoz, Y, Doré, J, and Schiffrin, EJ. The inflammatory status of old age can be nurtured from the intestinal environment. Curr Opin Clin Nutr Metab Care. (2008) 11:13–20. doi: 10.1097/MCO.0b013e3282f2bfdf
18. Kawase, M, He, F, Miyazawa, K, Kubota, A, Yoda, K, and Hiramatsu, M. Orally administered heat-killed Lactobacillus gasseri TMC0356 can upregulate cell-mediated immunity in senescence-accelerated mice. FEMS Microbiol Lett. (2012) 326:125–30. doi: 10.1111/j.1574-6968.2011.02440.x
19. Huang, F, and Wu, X. Brain neurotransmitter modulation by gut microbiota in anxiety and depression. Front Cell Dev Biol. (2021) 9:649103. doi: 10.3389/fcell.2021.649103
20. Clements, SJ, and Carding, R. Diet, the intestinal microbiota, and immune health in aging. Crit Rev Food Sci Nutr. (2018) 58:651–61. doi: 10.1080/10408398.2016.1211086
21. Buford, TW . (dis)trust your gut: the gut microbiome in age-related inflammation, health, and disease. Microbiome. (2017) 5:80. doi: 10.1186/s40168-017-0296-0
22. Maloy, KJ . The Interleukin-23/Interleukin-17 axis in intestinal inflammation. J Intern Med. (2008) 263:584–90. doi: 10.1111/j.1365-2796.2008.01950.x
23. Rayner, CK, and Horowitz, M. Physiology of the ageing gut. Curr Opin Clin Nutr Metab Care. (2013) 16:33–8. doi: 10.1097/MCO.0b013e32835acaf4
24. Grassi, M, Petraccia, L, Mennuni, G, Fontana, M, Scarno, A, Sabetta, S, et al. Changes, functional disorders, and diseases in the gastrointestinal tract of elderly. Nutr Hosp. (2011) 26:659–68. doi: 10.1590/S0212-16112011000400001
25. Kim, S, and Jazwinski, SM. The gut microbiota and healthy aging: a Mini-review. Gerontology. (2018) 64:513–20. doi: 10.1159/000490615
26. Han, Y, Wang, T, Li, C, Wang, Z, Zhao, Y, He, J, et al. Ginsenoside Rg3 exerts a neuroprotective effect in rotenone-induced Parkinson's disease mice via its anti-oxidative properties. Eur J Pharmacol. (2021) 909:174413. doi: 10.1016/j.ejphar.2021.174413
27. Qian, YH, Han, H, Hu, XD, and Shi, LL. Protective effect of ginsenoside Rb1 on beta-amyloid protein(1-42)-induced neurotoxicity in cortical neurons. Neurol Res. (2009) 31:663–7. doi: 10.1179/174313209X385572
28. Shi, M, Ma, J, Jin, S, Wang, T, Sui, Y, and Chen, L. Effects of saponins Rb1 and re in American ginseng combined intervention on immune system of aging model. Front Mol Biosci. (2024) 11:1392868. doi: 10.3389/fmolb.2024.1392868
29. Wingett, SW, and Andrews, S. Fast Q screen: a tool for multi-genome mapping and quality control. F1000Res. (2018) 7:1338. doi: 10.12688/f1000research.15931.1
30. Bolyen, E, Rideout, JR, Dillon, MR, Bokulich, NA, Abnet, CC, al-Ghalith, GA, et al. Author correction: reproducible, interactive, scalable and extensible microbiome data science using QIIME 2. Nat Biotechnol. (2019) 37:1091. doi: 10.1038/s41587-019-0252-6
31. Konopiński, MK . Shannon diversity index: a call to replace the original Shannon's formula with unbiased estimator in the population genetics studies. Peer J. (2020) 8:e9391. doi: 10.7717/peerj.9391
32. Segata, N, Izard, J, Waldron, L, Gevers, D, Miropolsky, L, Garrett, WS, et al. Metagenomic biomarker discovery and explanation. Genome Biol. (2011) 12:R60. doi: 10.1186/gb-2011-12-6-r60
33. Lundberg, DS, Yourstone, S, Mieczkowski, P, Jones, CD, and Dangl, JL. Practical innovations for high-throughput amplicon sequencing. Nat Methods. (2013) 10:999–1002. doi: 10.1038/nmeth.2634
34. Bai, X . Study on the mechanism of pretreated oat bran improving intestinal flora and glucolipid metabolism inner. Mongolia agricultural university [Doctoral thesis]. Available at: https://chn.oversea.cnki.net/KCMS/detail/detail.aspx?dbcode=CDFD&dbname=CDFDLAST2024&filename=1023797495.nh&uniplatform=OVERSEA&v=5u7kUgjVEQ69AsvMcDTM2veqTdFt6Dq2n25ke9hfFrWh_4RAHfYwt9GFCaAF9DnJ
35. Rezaeiasl, Z, Salami, M, and Sepehri, G. The effects of probiotic Lactobacillus and Bifidobacterium strains on memory and learning behavior, long-term potentiation (LTP), and some biochemical parameters in β-amyloid-induced Rat's model of Alzheimer's disease. Prev Nutr Food Sci. (2019) 24:265–73. doi: 10.3746/pnf.2019.24.3.265
36. Tan, FHP, Liu, G, Lau, SA, Jaafar, MH, Park, YH, Azzam, G, et al. Lactobacillus probiotics improved the gut microbiota profile of a Drosophila melanogaster Alzheimer's disease model and alleviated neurodegeneration in the eye. Benef Microbes. (2020) 11:79–89. doi: 10.3920/BM2019.0086
37. Jang, SE, Hyam, SR, Han, MJ, Kim, SY, Lee, BG, and Kim, DH. Lactobacillus brevis G-101 ameliorates colitis in mice by inhibiting NF-κB, MAPK and AKT pathways and by polarizing M1 macrophages to M2-like macrophages. J Appl Microbiol. (2013) 115:888–96. doi: 10.1111/jam.12273
38. Jang, SE, Jeong, JJ, Kim, JK, Han, MJ, and Kim, DH. Simultaneous Amelioratation of colitis and liver injury in mice by Bifidobacterium longum LC67 and Lactobacillus plantarum LC27. Sci Rep. (2018) 8:7500. doi: 10.1038/s41598-018-25775-0
39. Jeong, JJ, Kim, KA, Jang, SE, Woo, JY, Han, MJ, and Kim, DH. Orally administrated Lactobacillus pentosus var. plantarum C29 ameliorates age-dependent colitis by inhibiting the nuclear factor-kappa B signaling pathway via the regulation of lipopolysaccharide production by gut microbiota. PLoS One. (2015) 10:e0116533. doi: 10.1371/journal.pone.0116533
40. Zakostelska, Z, Kverka, M, Klimesova, K, Rossmann, P, Mrazek, J, Kopecny, J, et al. Lysate of probiotic Lactobacillus casei DN-114 001 ameliorates colitis by strengthening the gut barrier function and changing the gut microenvironment. PLoS One. (2011) 6:e27961. doi: 10.1371/journal.pone.0027961
41. Zhang, L, Wang, Y, Xiayu, X, Shi, C, Chen, W, Song, N, et al. Altered gut microbiota in a mouse model of Alzheimer's disease. J Alzheimers Dis. (2017) 60:1241–57. doi: 10.3233/JAD-170020
42. Claesson, MJ, Cusack, S, O'Sullivan, O, Greene-Diniz, R, de Weerd, H, Flannery, E, et al. Composition, variability, and temporal stability of the intestinal microbiota of the elderly. Proc Natl Acad Sci USA. (2011) 108:4586–91. doi: 10.1073/pnas.1000097107
43. Claesson, MJ, Jeffery, IB, Conde, S, Power, SE, O’Connor, EM, Cusack, S, et al. Gut microbiota composition correlates with diet and health in the elderly. Nature. (2012) 488:178–84. doi: 10.1038/nature11319
44. Odamaki, T, Kato, K, Sugahara, H, Hashikura, N, Takahashi, S, Xiao, JZ, et al. Age-related changes in gut microbiota composition from newborn to centenarian: a cross-sectional study. BMC Microbiol. (2016) 16:90. doi: 10.1186/s12866-016-0708-5
45. McElhanon, BO, McCracken, C, Karpen, S, and Sharp, WG. Gastrointestinal symptoms in autism spectrum disorder: a meta-analysis. Pediatrics. (2014) 133:872–83. doi: 10.1542/peds.2013-3995
46. Lasheras, I, Real-López, M, and Santabárbara, J. Prevalence of gastrointestinal symptoms in autism spectrum disorder: a meta-analysis. An Pediatr. (2023) 99:102–10. doi: 10.1016/j.anpede.2023.07.003
47. Doraiswamy, PM, Leon, J, Cummings, JL, Marin, D, and Neumann, PJ. Prevalence and impact of medical comorbidity in Alzheimer's disease. J Gerontol A Biol Sci Med Sci. (2002) 57:M173–7. doi: 10.1093/gerona/57.3.m173
48. Mulak, A . Bile acids as key modulators of the brain-gut-microbiota Axis in Alzheimer's disease. J Alzheimers Dis. (2021) 84:461–77. doi: 10.3233/JAD-210608
49. Mosher, KI, and Wyss-Coray, T. Microglial dysfunction in brain aging and Alzheimer's disease. Biochem Pharmacol. (2014) 88:594–604. doi: 10.1016/j.bcp.2014.01.008
50. Chen, SG, Stribinskis, V, Rane, MJ, Demuth, DR, Gozal, E, Roberts, AM, et al. Exposure to the functional bacterial amyloid protein Curli enhances alpha-Synuclein aggregation in aged Fischer 344 rats and Caenorhabditis elegans. Sci Rep. (2016) 6:34477. doi: 10.1038/srep34477
51. Minter, MR, Zhang, C, Leone, V, Ringus, DL, Zhang, X, Oyler-Castrillo, P, et al. Antibiotic-induced perturbations in gut microbial diversity influences neuro-inflammation and amyloidosis in a murine model of Alzheimer's disease. Sci Rep. (2016) 6:30028. doi: 10.1038/srep30028
Keywords: aging, American ginseng , Rb1, Re, intestinal microbiota
Citation: Shi M, Fan H, Liu H and Zhang Y (2024) Effects of saponins Rb1 and Re in American ginseng intervention on intestinal microbiota of aging model. Front. Nutr. 11:1435778. doi: 10.3389/fnut.2024.1435778
Edited by:
Gonzalo Jorquera, University of Chile, ChileReviewed by:
Jonathan Martinez-Pinto, Universidad de Valparaiso, ChileSamanta Thomas-Valdés, Universidad de Valparaiso, Chile
Copyright © 2024 Shi, Fan, Liu and Zhang. This is an open-access article distributed under the terms of the Creative Commons Attribution License (CC BY). The use, distribution or reproduction in other forums is permitted, provided the original author(s) and the copyright owner(s) are credited and that the original publication in this journal is cited, in accordance with accepted academic practice. No use, distribution or reproduction is permitted which does not comply with these terms.
*Correspondence: YanRong Zhang, WmhhbmdZUkAxNjMuY29t