- Beijing Key Laboratory of Basic Research on Traditional Chinese Medicine to Prevent and Control Major Diseases, Experimental Research Center, China Academy of Chinese Medical Sciences, Beijing, China
Introduction: Cannabidiol (CBD) has a variety of pharmacological effects including antiepileptic, antispasmodic, anxiolytic and anti-inflammatory among other pharmacological effects. However, since CBD is a terpene-phenolic compound, its clinical application is limited by its poor water solubility, low stability, and low bioavailability.
Methods: In this study, we used several strategies to address the above problems. Hydrochloric acid was used to modify zein to improve the molecular flexibility. Flexible zein nanoparticles (FZP-CBD) loaded with CBD was prepared to improve the stability and bioavailability of CBD. The parameters were evaluated in terms of morphology, particle size (PS), polydispersity index (PDI), zeta potential (ZP), entrapment efficiency (EE%), loading capacity (LC%), and storage stability. Simulated gastrointestinal fluid release experiment and bioavailability assay were applied in the evaluation.
Results: The simulated gastrointestinal fluid experiment showed that the release rates of FZP-CBD and natural zein nanoparticles (NZP-CBD) loaded with CBD were 3.57% and 89.88%, respectively, after digestion with gastric fluid for 2 h, 92.12% and 92.56%, respectively, after intestinal fluid digestion for 2 h. Compared with NZP-CBD, the Cmax of FZP-CBD at 3 different doses of CBD was increased by 1.7, 1.3 and 1.5 times respectively, and AUC0-t was increased by 1.4, 1.1 and 1.7 times respectively, bioavailability (F) was increased by 135.9%, 114.9%, 169.6% respectively.
Discussion: The experimental results showed that FZP-CBD could protect most of the CBD from being released in the stomach, and then control its release in the intestines, promote the absorption of CBD in the small intestine, and increase the bioavailability of CBD. Therefore, FZP-CBD could improve the utilization value of CBD and provide a new idea for the application of CBD in medicine and pharmacy.
1 Introduction
Cannabidiol (CBD) is one of the main extracts of Cannabis sativa L., an annual herb of the family Moraceae. Currently, more than 130 cannabinoids have been isolated from dried plants and fresh cannabis leaves. Among them, the tetrahydrocannabinol (THC) and CBD contents are the highest. While THC is hallucinogenic, CBD is a non-addictive component because it can antagonize the psychoactivity triggered by THC agonism at cannabinoid receptor I (CB1R). CBD has antiepileptic, antispasmodic, anxiolytic and anti-inflammatory effects among other pharmacological effects (1, 2). However, CBD is a terpene phenolic compound. It is highly lipophilic (logP = 6.3) with a low solubility in water of 0.02–0.06 μg•mL−1 at room temperature (3). Currently approved CBD drugs are mostly ethanol or oil-based (4), such as Epidiolex® (a pure CBD oral solution suspended in sesame seed oil and ethanol) and Sativex® (a CBD and Delta-9-tetrahydrocannabinol (THC, 1:1) oromucosal spray suspended in anhydrous ethanol and propylene glycol) (5, 6) which were approved by the United States (US) Food and Drug Administration and the European Medicine Agency, respectively. However, highly lipophilic drugs delivered orally tends to precipitate in the gastrointestinal (GI) tract and are difficult to be completely solubilized. This is the main reason for its low bioavailability (7). Some studies have shown that the oral bioavailability of CBD oil in human trials is about 6% (8–10). CBD is mainly absorbed in the upper part of the small intestine (11). CBD has poor water solubility and stability, and it may be inactivated due to degradation, especially under acid, oxygen, high temperature, light radiation and other conditions, thus significantly reducing its bioavailability and utilization value (12). To improve the bioavailability and therapeutic efficacy of CBD, our study aims to develop a safe dosage form for the delivery of CBD that not only reduces metabolic degradation but also improves its solubility.
Nanodelivery system is a technology to deliver drugs or other biomolecules to specific cells or tissues through a carrier called nanoparticles. Mucoadhesion of nanoparticles depends on the presence of mucoadhesive polymers. Once properly selected, the efficiency of adhesion and interaction with cells may improve due to their size and large specific surface area. They can also improve the poor solubility of hydrophobic drugs, low bioavailability and short half-life (13–15), thereby demonstrating significant promise in increasing the oral bioavailability of highly lipophilic compounds (12). Nanoparticles are small colloidal particles with three dimensions in nanomaterials. Protein particles are ubiquitous in the field of drug delivery. They have intrinsic bioactivity, biocompatibility, biodegradability and colloidal properties, including self-assembly behavior, structural reorganization, intermolecular and intramolecular hydrophobic and hydrogen bonding interactions (16). Nanoparticles can be absorbed in organisms by two routes. The first is to be directly taken up by small intestinal epithelial cells via inducing endocytosis (14). For example, the presence of lactoferrin receptors in intestinal epithelial cells can help lactoferrin nanoparticles containing Garcinia cambogia to cross the epithelial cells into the blood vessels (17). To achieve endocytosis, the nanoparticles must be small in size and their surface properties must be carefully designed to facilitate cell entry (18, 19). Larger particles or particles with highly dense cationic surfaces may induce the formation of pores on membranes and result in cytotoxicity (18). Another way of absorption is that the nanoparticles can be digested by digestive enzymes in the gastrointestinal tract, which frees the active substance and transports it into the blood circulation. The active substance is absorbed through two main transport mechanisms, active and passive transport in the small intestine (13).
Zein is an important storage protein in corn (20). It contains more than half of its amino acids in a nonpolar form, lacks charged amino acids, and is low in polar amino acids. As a result, it is not soluble in pure water and anhydrous alcoholic media. However, it can be dissolved into 50–95% aqueous ethanol solutions (21). Zein is subject to reduced solubility due to decreased ethanol concentration and increased solvent polarity, which causes a change in protein conformation in the process, resulting in aggregation of the molecules, a process known as self-assembly of zein (21). Zein can be induced to self-assemble into different nanostructures such as nanoparticles, micelles, fibers and membranes by adjusting the polarity of the medium (22). The anti-solvent method is one of the commonly used methods for the preparation of zein nanoparticles. In this method, zein undergoes self-assembly and aggregates into nanoparticles to evade highly polar environments when the polarity of the medium changes from weak to strong (23). Zein nanoparticles have been used as nanocarrier materials in the aspects of encapsulation efficiency, stability and drug release (22, 24–26). However, due to the strong hydrophobicity and rigid structure of zein, the nanoparticles formed by natural zein are poorly adapted to the environment. Therefore, they are not perfect for drug delivery. To improve the colloidal stability of zein particles or to regulate the drug release rate, many researchers have attempted to improve the EE% or control the drug release by introducing other polymers or using acid to modify zein nanoparticles (27, 28), and subsequently improving the ability of zein nanoparticles to transport small molecule drugs. For example, Dai et al. improved the entrapment efficiency (EE%) and stability of zein nanoparticles to hydrophobic drugs by adding rhamnose (29). Van Ballegooie et al. modified zein with polyethylene glycol (PEG) to improve its stability (30). Ahammed et al. used glutamine-transaminase (TG) modified gelatin-zein complex loaded with green tea polyphenols (TP) to control the release of phenolic compounds (31). In this experiment, we prepared nanoparticles to properly encapsulate and transport CBD by enhancing the flexibility of zein, facilitating its delivery of CBD to the small intestine for release and absorption. This approach can improve the utilization efficiency of CBD to a certain extent.
In this study, flexible zein was obtained by acid modification of zein and flexible zein nanoparticles (FZP-CBD) and natural zein nanoparticles (NZP-CBD) loaded with CBD were prepared. We Studied their morphology, particle size (PS), polydispersity index (PDI), Zeta potential (ZP), EE%, loading capacity (LC%), storage stability, and in vitro simulated gastrointestinal fluid release. The oral bioavailability of the two was also explored for comparison by in vivo pharmacokinetic experiments in rats. This study improves the value of CBD utilization and broadens the scope of zein application.
2 Materials and methods
2.1 Materials and instruments
Agilent 6,410 QQQ LC–MS analysis system, consisting of an Agilent 1,290 liquid chromatograph and Agilent 6,410 QQQ triple quadrupole mass spectrometry detector, Masshunter workstation was obtained from Agilent Technologies. Hettich Mikro 220R benchtop cryogenic high-speed centrifuge was obtained from Hettich. Gene G560E vortex mixer was obtained from Scientific Industries. Milli-Q Integral 3 integrated ultrapure water system was obtained from Merck KGaA. Sartorius SQP QUINTIX65-1CN one-millionth electronic balance was obtained from Sartorius. DK-98-II electrothermal thermostatic water bath was obtained from Tianjin Test Instrument Co., Ltd. PH meter was obtained from Sartorius. V-850 rotary evaporator was obtained from BUCHI. Hitachi S-4800 field emission scanning electron microscope was obtained from Hitachi. LC-250 Nanoparticle Size and Zeta Potential Analyzer was obtained from Microtrac. SW-CJ-2FD Cell Incubator was obtained from Thermo Fisher Scientific.
Zein was obtained from Yuan Ye Biotechnology Co., Ltd. Cannabidiol (CBD) was obtained from Heilongjiang Zhongsheng Bio-technology Co., Ltd. (−)-Cannabidiol-d9 (internal standard) was obtained from Shanghai Saiers Biochemical Technology Co., Ltd. Methanol for chromatography, Acetonitrile for chromatography and Formic acid for chromatography were obtained from Dikma. Anhydrous ethanol (analytically pure) and hydrochloric acid (analytically pure) were obtained from Sinopharm Chemical Reagent Co., Ltd. Sodium hydroxide (analytically pure) was obtained from Beijing Chemical Industry Co., Ltd. Potassium Dihydrogen Phosphate, pepsin and trypsin were obtained from Source Leaf Biotechnology Co., Ltd. and Ultra-pure water (self-made).
Rat adrenal pheochromocytoma cell lines (PC12 cells) were obtained from the Cell Resource Center, Shanghai Academy of Biological Sciences, Chinese Academy of Sciences.
There were 36 Sprague Dawley (SD) rats aged 7–8 weeks (half male and half female), weighing 220–300 g purchased from Beijing Huafukang Bio-technology Co., Ltd. NO. 110322230100338248. All rats were fed in SPF level animal room. All animal experiments were approved by the animal ethics committee of Experimental Research Center, China Academy of Chinese Medical Sciences (ERCCACMS21-2211-01).
2.2 Methods
2.2.1 Preparation of nanoparticles
Zein alcohol solution loaded with CBD: zein was fully dispersed in 90% ethanol to obtain 25 mg•mL−1 of zein ethanol solution. The solution was equally divided into two groups. One group was left untreated and the other was adjusted to pH 2.0 with HCl and then heated by condensation reflux in a water bath at 70°C for 10 h to obtain a flexible zein alcohol solution. NaOH solution was used to adjust the pH of the flexible zein alcohol solution to 7.0. Both groups of solutions were divided into three equal portions, then different amounts of CBD were added, respectively, to obtain zein alcohol solution containing CBD with low, medium and high concentrations (4.10 and 16 mg•mL−1).
FZP-CBD and NZP-CBD: FZP-CBD and NZP-CBD were prepared by rapid anti-solvent precipitation method, in which the zein alcohol solution loaded with CBD was rapidly poured into ultrapure water at a volume ratio of 1:4 while stirring with 40°C. The ethanol was removed by rotary evaporation, and then ultrapure water was added to replenish the volume of the ethanol that had been lost. NZP-CBD and FZP-CBD loaded with varying amounts of CBD were obtained. They were stored in colloidal solution form at 4°C in the refrigerator for future use.
2.2.2 Morphology
The microscopic morphology of NZP-CBD and FZP-CBD was observed using scanning electron microscopy (SEM) at an operating voltage of 5 kV and a magnification of 45,000×. A small amount of freshly prepared samples was applied to silicon wafers, which were naturally dried and then sprayed with gold, imaged and photographed.
2.2.3 PS, PDI, and ZP
The average PS and PDI of NZP-CBD and FZP-CBD were measured by the dynamic light scattering (DLS) method by using the Nano-ZS nanolaser, and the potential at the nanoparticle shear plane, denoted as the ZP, was measured using the above instrument.
2.2.4 EE% and LC%
High-performance liquid chromatography (HPLC) was used to determine the EE% and LC% of the nanoparticles.
2.2.4.1 Establishment of HPLC methods
The chromatographic conditions were as follows: chromatographic column Diamonsil C18 (2) (250 mm × 4.6 mm, 5 μm); mobile phase methanol–water (87:13, v/v); flow rate 1.0 mL•min−1; detection wavelength 220 nm; and column temperature 25°C.
The EE% and LC% of FZP-CBD and NZP-CBD were calculated using the following Equations (1) and (2):
Csystem and Cfree are the concentrations of encapsulated CBD and free CBD in the colloidal system, respectively. Wsystem and Wtotal are the mass of the encapsulated CBD and the total mass of the nanoparticles in the colloidal system, respectively.
2.2.4.2 Free CBD extraction
We took 100 μL of different concentrations of NZP-CBD and FZP-CBD and added 300 μL of petroleum ether to extract three times. Then it was redissolved by adding 1 mL of acetonitrile after nitrogen blowing, vortexed for 30 s, and injected the sample.
2.2.4.3 Encapsulated CBD extraction
After drying under nitrogen, the nanoparticles were extracted with 900 μL acetonitrile, vortexed for 2 min at 16,000 r•min−1, 4°C, centrifuged for 10 min, 100 μL supernatant was mixed with 900 μL acetonitrile, vortexed for 30 s, and injected into the column.
2.2.5 Storage stability
The prepared NZP-CBD and FZP-CBD were stored at 4°C under light-avoidance conditions, and the degradation of CBD was measured at day 0, 3, 7, 14, and 21. The storage stability of NZP-CBD and FZP-CBD was expressed by the Leaching Rate and it was calculated using the following Equation (3).
where Wsystem and Wfree are the mass of encapsulated CBD and the mass of degraded CBD in the colloidal system, respectively.
2.2.6 Simulated gastrointestinal fluid release
Ten milliliter of prepared NZP-CBD and FZP-CBD suspensions were mixed with 10 mL of simulated gastric fluid (SGF) containing 10 mg•mL−1 pepsin at pH 2 and then incubated for 2 h (samples were collected at 0, 0.5, 1, 1.5, 2 h) in a shaker at 37°C with shaking at a speed of 100 rpm. After 2 h of digestion, the pH was adjusted to 7.5 using NaOH solution to inactivate the enzyme. Subsequently, 10 mL digestion mixture was poured into the 10 mL of simulated intestinal fluid (SIF, dissolving pancreatin (10 mg•mL−1) in phosphate buffer at pH 6.8.) and incubated for 2 h (samples were collected at 0, 0.5, 1, 1.5, 2 h) under the same conditions and then terminated by rapid cooling in ice. The CBD was determined by HPLC and its digestion was expressed by Release Rate (Eq. 4).
Where W1 is the amount of CBD released and W0 is the amount of CBD wrapped at 0 h.
2.2.7 In vitro biocompatibility
To investigate the biocompatibility of FZP-CBD, the experiments used PC12 cells as a cell model. The cytotoxicity was detected by CCK8. Logarithmic growing PC12 cells were inoculated into 96-well cell culture plates at a density of 8 × 104 cells•mL−1 per well, and cultured in a 5% CO2 cell incubator at 37°C. When the cell density reached to 60%, different concentrations of CBD solution and FZP-CBD (0, 1, 2.5, 5, 10, 15, 20, 25 μM) were added to PC12 cells after being completely diluted with DMEM medium to continue the incubation for 24 h. Then 10% CCK8 staining solution was added to each well and continued culturing for 1.5 h. Absorbance (OD value) at 490 nm wavelength was measured by BioTek Synergy H1 Hybrid multimode microplate reader. Taking the blank nanoparticles without CBD as a reference, the experiment was repeated 3 times, and cell viability was calculated using the following Equation (5).
Where A is the OD value of the cells in the sample wells, A0 is the OD value of the cells in the blank group, and A1 is the OD value of the blank wells.
2.2.8 In vivo bioavailability
36 SD rats were divided into six groups. NZP-CBD and FZP-CBD in 3 doses of 20, 50 and 100 mg·kg−1, respectively, were administrated intragastric in each group. Blood samples were collected from the oculi chorioideae vein at 0, 0.5, 1, 1.5, 2, 3, 4, 6, 8, 10, 12, 24, and 48 h after the administration. Blood samples were centrifuged at 3,000 rpm·min−1 for 10 min at 4°C. The supernatant was stored at −80°C for measurement.
Supernatant (20 μL) was added to 60 μL of methanol and centrifuged at 12,000 rpm•min−1 for 10 min at 4°C. The supernatant was extracted and analyzed by LC–MS. The pharmacokinetic parameters, including half-life (T1/2), peak time (Tmax), peak concentration (Cmax), area under the curve (AUC), and mean residence time (MRT) were statistically analyzed by Winnonlin 8.1.
Chromatographic conditions: column: Waters Atlantis T3 (100 mm × 2.1 mm, 3 μm), mobile phases: A is 0.1% formic acid-water, B is 0.1% formic acid-acetonitrile, gradient elution, 0.0–4.5 min, 85% B, 4.5–5.0 min, 85–100% B, column temperature 30°C, flow rate 0.3 mL•min−1, injection volume 1 μL.
Mass spectrometry conditions: electrospray ionization source (ESI), multiple reaction monitoring (MRM), positive ion mode detection, CBD ion pair 315.3 → 259.3, isotopic internal standard (−)-Cannabidiol-d9 ion pair 324 → 268.2, collision energy CBD is 15 V, the internal standard is 20 V, fragment 110 V for CBD and 170 V for the internal standard, atomizing gas pressure 15 psi, ion source temperature 300°C, and atomizing gas flow rate 12 L•min−1.
3 Results and discussion
3.1 Morphology
Dong (32) and Mattice and Marangoni (33) found that the percentage of the α-helical structure of zein decreased and intermolecular β-folding increased after being treated with aqueous acetic acid. This may be due to the change of α-helix to β-folding and irregularly curled structures in the protein, resulting in the protein becoming loose and disordered, which makes the protein structure flexible (34). The distribution of nanoparticles formed by natural zein is more dense and ordered, forming a reticular structure. In contrast, the nanoparticles formed by flexible zein are sparse and disordered, indicating the flexibility of this part of the protein structure (Figure 1). This facilitates the binding of zein to CBD and improves the storage stability of the drug.
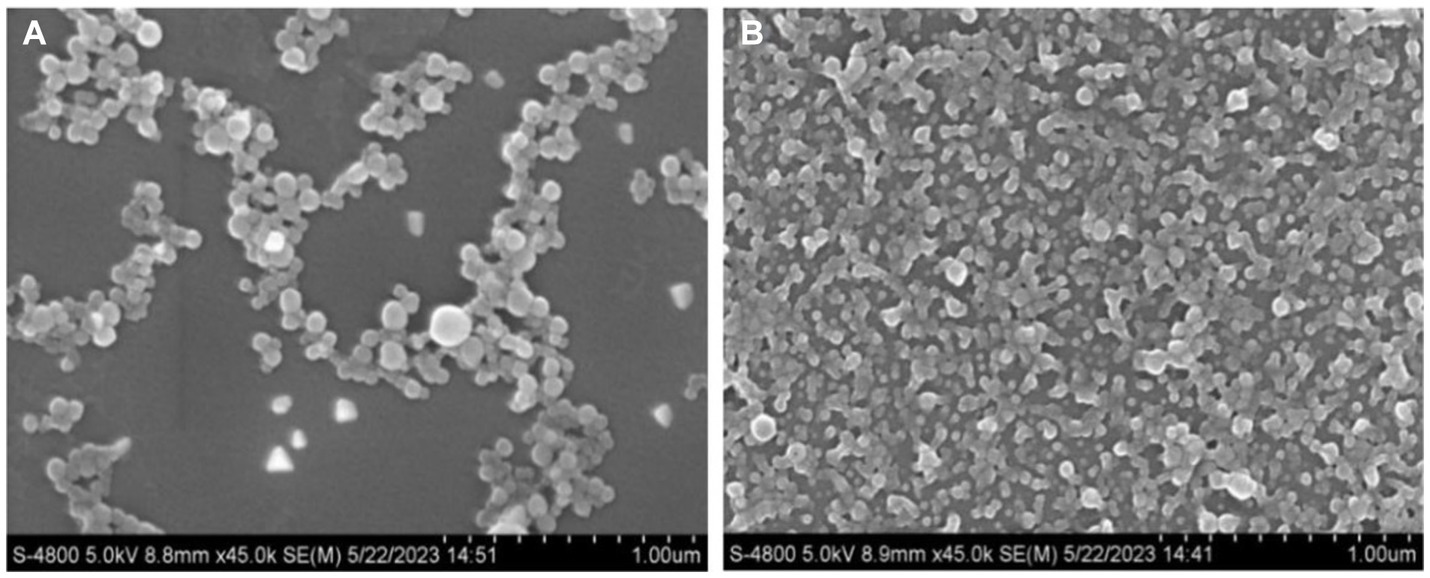
Figure 1. Micromorphology (magnification of 45,000×) of FZP-CBD (A) and NZP-CBD (B) loaded with CBD.
3.2 PS, PDI, and ZP
The PS, PDI and ZP of NZP-CBD and FZP-CBD were shown in Figure 2. The results suggested that the larger PS of FZP-CBD may be the reason for the increased LC%. The PDI of FZP-CBD and NZP-CBD at low, medium and high concentrations were 0.12, 0.15, and 0.20 and 0.17, 0.17, and 0.23, respectively. This may be because FZP-CBD could prevent protein aggregation through electrostatic interactions (35), resulting in a more uniform distribution of proteins in solution with smaller PDI.
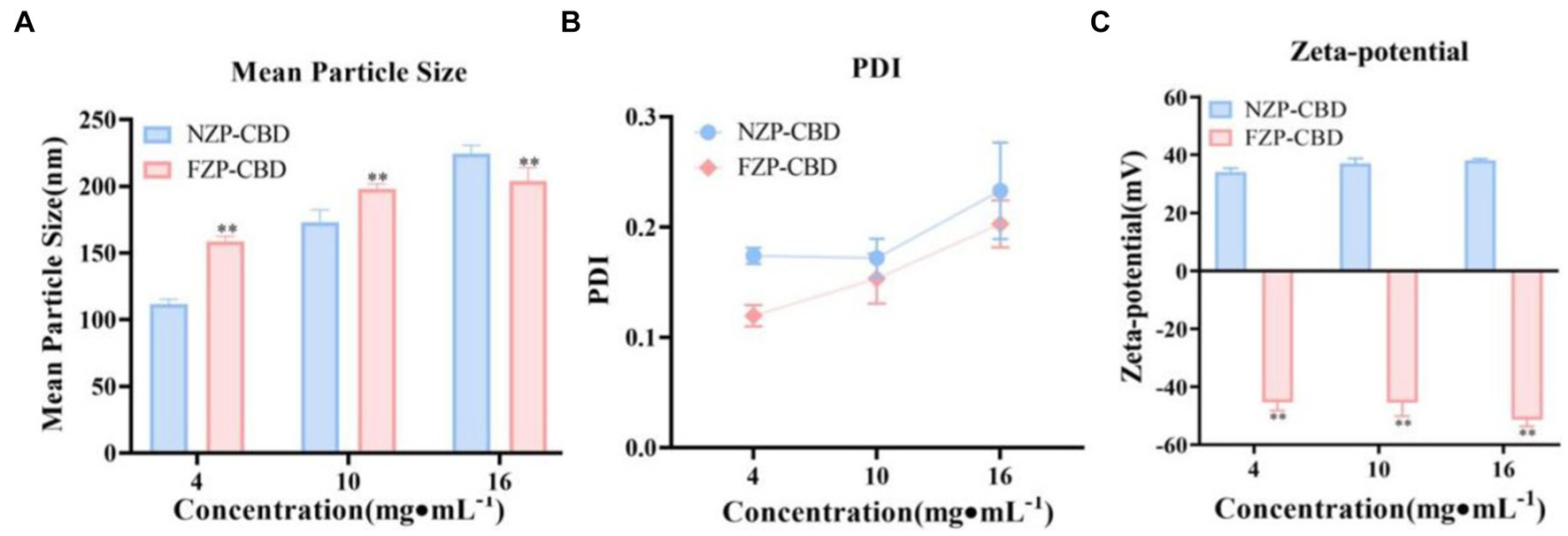
Figure 2. PS (A), PDI (B), and ZP (C) of NZP-CBD and FZP-CBD. *p < 0.05, **p < 0.01 compared to NZP-CBD (x ± s, n = 3).
As shown in Figure 2C, due to deamidation, the amide group is converted to a carboxyl group, and the FZP-CBD’s ZP became negative after ionization, which was between −52 and −45 mV. The NZP-CBD’s ZP was between 34 and 38 mV due to the ionization of amide groups. The value of ZP is related to the stability of colloidal dispersion, with the absolute value representing the degree of stability (36). The greater the absolute value, the better the stability. The absolute value of FZP-CBD was significantly larger than that of NZP-CBD (p < 0.01), indicating that FZP-CBD is employed to enhance the dispersibility of proteins by substituting the amino group in amide residues with a carboxyl group (37), thereby increasing electrostatic repulsion to prevent the aggregation of particles and thus has higher stability (32, 35).
3.3 EE% and LC%
The CBD entrapped inside of the nanoparticles was determined using newly prepared colloidal solutions of NZP-CBD and FZP-CBD at low, medium and high concentrations (Supplementary Table S2). There were some differences between NZP-CBD and FZP-CBD. The EE% and LC% of CBD in NZP-CBD were 72.89, 62.11, 79.27, and 29.30%, 36.86, 53.63% for low, medium and high concentrations, respectively, while that of FZP-CBD was 92.23, 86.14, 85.43 and 34.68%, 48.86, 56.94% for low, medium and high concentrations, respectively. The deamidation reaction which was performed by acid modification converts amide groups to carboxyl groups, and destroys the helical structure, thus resulting in increased β-folding and decreased.
α-helix (32). This may induce the gradual unfolding and disordering of zein’s tertiary structure and increase the protein’s interfacial adsorption capacity (38). Thereby it is conducive to the adsorption and binding of zein and CBD. Therefore, the EE% and LC% of FZP-CBD were higher than that of NZP-CBD, and the EE% and LC% of CBD could be improved by FZP-CBD.
3.4 Storage stability
The prepared NZP-CBD and FZP-CBD were stored at 4°C and protected from light. The degradation of CBD was measured on day 0, 3, 7, 14, and 21. Based on the leakage of CBD from nanoparticles in the medium after storage for 21 days, the storage stability was characterized by the leaching rate (as shown in Equation 3). The leaching rate of CBD in FZP-CBD was significantly lower than that of CBD in NZP-CBD throughout the storage period (p < 0.01) (Figure 3). It indicates that FZP-CBD improved the storage stability of CBD. This may be related to the ZP. Abdelbary et al. (39) concluded that the ZP was stable at around ±30 mV. The greater the absolute value, the better the stability. As can be seen in Figure 2C, the absolute value of FZP-CBD was significantly greater than that of NZP-CBD (p < 0.01), indicating that FZP-CBD has better storage stability.
3.5 Simulated gastrointestinal fluid release
The controlled release of CBD in the human gastrointestinal tract can play an important role in improving its bioavailability. In this study, the freshly prepared FZP-CBD and NZP-CBD were digested in SGF for 2 h and then transferred to SIF for further digestion for 2 h. The digestion was expressed in terms of the release rate. After digestion in SGF for 2 h, the release rates of FZP-CBD and NZP-CBD were 3.57 and 89.88%, respectively, which indicated that FZP-CBD had a protective effect on CBD in SGF. After digestion in SIF for 2 h, the release rates of CBD in FZP-CBD and NZP-CBD were 92.12 and 92.56%, respectively (Figure 4), indicating that both nanoparticles could fully release CBD in SIF, which may be due to the presence of pancreatin in the SIF (40). The results showed that FZP-CBD could protect most of the CBD from being released in the stomach, and then control its release in the intestines and promote the absorption of CBD in the small intestine, thus improving the bioavailability of CBD.
Pepsin causes structural changes in proteins by shearing the peptide bonds of hydrophobic amino acids. Meanwhile, deamidation decreases the hydrophobic amino acids and increases the hydrophilic amino acid content in protein molecules to protect proteins from destruction (32). Hurtado-López and Murdan (41) studied zein nanoparticles and showed that pepsin was able to digest α-zein monomers. The zein obtained via acid modification had decreased α-helix content and increased the β-fold content (33) so it was not easy to be digested by pepsin. CBD was encapsulated in the hydrophobic core of flexible zein, which was not easily destroyed by pepsin in SGF, and therefore only a small amount of CBD was released. When zein was transferred to the SIF, trypsin degraded the protein structure of flexible zein and CBD was released (22). This indicated that flexible zein could effectively avoid CBD leakage in gastric fluid. Due to the presence of pancreatin in the SIF (29, 41), FZP-CBD showed a sudden release effect during intestinal digestion. This results in the rapid decomposition of zein, which promotes the release and absorption of CBD and improves its bioavailability.
3.6 In vitro biocompatibility
Using PC12 cells as in vitro cell lines, the cytotoxicity of CBD dispersion and FZP-CBD was studied by CCK8. CBD-Dispersion and FZP-CBD increased the viability of PC12 cells within the concentration range of 1–20 μM. However, when the concentration went beyond 20 μM and reached 25 μM, the cell viability of both CBD-Dispersion and FZP-CBD decreased and resulted in cytotoxicity (Figure 5). Therefore, within the safe concentration range, FZP-CBD does not produce toxicity to cells, indicating that flexible zein has good biocompatibility.
3.7 In vivo bioavailability
The pharmacokinetic differences of NZP-CBD and FZP-CBD in the serum of rats were observed and explored after a single intragastric administration. Compared with NZP-CBD, FZP-CBD improved CBD bioavailability (Figure 6). Cmax of FZP-CBD at 3 different doses of CBD was increased by 1.7, 1.3, and 1.5 times respectively, and AUC0-t was increased by 1.4, 1.1, and 1.7 times respectively, bioavailability (F) was increased by 135.9, 114.9, 169.6%, respectively (Supplementary Table S3). Since CBD is a highly lipophilic drug, it is mainly absorbed in the small intestine. The results of the simulated gastrointestinal release experiments showed that FZP-CBD could protect most of the CBD from being released in the stomach, and then control its release in the intestine. Therefore, FZP-CBD could promote the absorption of CBD in the small intestine and improve CBD bioavailability.
4 Discussion
Currently, nano-delivery systems have been applied to CBD, including polymeric and lipid-based nanoparticles (lipid nanocapsules) (LNCs) (42), nanostructured lipid carriers (NLCs) (43, 44), nanoemulsions (NEs) (45) and self-emulsifying drug delivery systems (SEDDS) (46). These methods could improve the solubility and oral bioavailability of CBD to a certain extent, but there may be some problems in practical application. For example, lipid nanoparticulate drug delivery systems can improve drug stability. However, the high water content in the drug formulation may reduce the drug loading. In addition, the drug can easily leak due to lipid crystallization during storage (12). SEDDS is one of the most widely used encapsulation techniques of CBD with good stability and high oral bioavailability, but it contains high concentrations of surfactants which can potentially irritate the gastrointestinal tract (46).
In this experiment, we prepared zein nanoparticles to properly encapsulate and transport CBD. Encapsulating insoluble CBD in nanoparticles allows CBD to be well dispersed in water, which increases its solubility in the gastrointestinal tract and improves the passive transport of CBD across the epithelial cells of the small intestinal villi (47, 48). Nanoparticles can be absorbed by epithelial cells through endocytosis, and PS is the most important physical property that determines the endocytosis pathway (49). The preparation of nanoparticles in this study has a small size, which is more likely to be endocytosed by epithelial cells (50).
Furthermore, acid-modified zein was used to undergo a deamidation reaction, which improved the molecular flexibility. FZP-CBD could effectively improve the bioavailability of CBD and it has a more homogeneous particle size dispersion and higher ZP, EE%, LC% and stability compared with NZP-CBD. CBD possesses high lipophilicity. CBD can precipitate in the gastrointestinal (GI) tract when delivered orally in solution. The main reason for its low bioavailability is that it forms a precipitate in the stomach that is difficult to dissolve completely. Promisingly, acid modification decreased α-helix content and increased β-fold content in flexible zein thereby making it difficult to be digested by pepsin (33). In flexible zein, CBD was encapsulated in the hydrophobic core. Due to the resistance of flexible zein to pepsin, CBD exposure to gastric juice can be effectively prevented. When FZP-CBD reached the intestinal digestion stage, a burst release was observed potentially due to the presence of trypsin in the SIF (29). This enabled flexible zein to be rapidly decomposed which facilitated the release and absorption of CBD. The experimental results showed that FZP-CBD could protect most of the CBD from being released in the stomach, and then control its release in the intestines and promote the absorption of CBD in the small intestine, thus improving the bioavailability of CBD.
In conclusion, FZP-CBD provides a way to increase bioavailability by increasing the solubilization of CBD in the aqueous environment of the GI tract. The improvement in CBD bioactivity is not only due to the rapid decomposition in the intestine but also due to the delayed release: a combination of protection from gastric fluid and burst release in the intestine. This allows more CBD to cross the inter-villous spaces at the intestinal brush border and move into blood circulation.
5 Conclusion
In this study, the FZP-CBD could control the release of CBD in the intestinal tract and promote its absorption in the small intestine. It could improve the bioavailability of CBD. Therefore, this study can improve the utilization value of CBD and broaden the application scope of zein. Flexible zein can be used as a potential carrier of the highly lipophilic drug CBD, which provides a new idea to achieve the controlled release of highly lipophilic drugs in the intestine and the application of CBD in pharmaceutics.
Data availability statement
The original contributions presented in the study are included in the article/Supplementary material, further inquiries can be directed to the corresponding author.
Ethics statement
The animal studies were approved by the Animal Ethics Committee of Experimental Research Center, China Academy of Chinese Medical Sciences. The studies were conducted in accordance with the local legislation and institutional requirements. Written informed consent was obtained from the owners for the participation of their animals in this study.
Author contributions
YK: Data curation, Formal analysis, Investigation, Writing – original draft. YN: Funding acquisition, Writing – review & editing. JP: Data curation, Formal analysis, Writing – review & editing. JS: Funding acquisition, Writing – review & editing. BF: Funding acquisition, Writing – review & editing.
Funding
The author(s) declare that financial support was received for the research, authorship, and/or publication of this article. The authors extended their appreciation to the Natural Science Foundation Project (JJPY2022007), the National Natural Science Foundation of China (82104391), Shandong Taishan Scholars Young Experts Project (tsqn202211360), and the Fundamental Research Funds for the Central Public Welfare Research Institutes (XTCX2021003).
Conflict of interest
The authors declare that the research was conducted in the absence of any commercial or financial relationships that could be construed as a potential conflict of interest.
Publisher’s note
All claims expressed in this article are solely those of the authors and do not necessarily represent those of their affiliated organizations, or those of the publisher, the editors and the reviewers. Any product that may be evaluated in this article, or claim that may be made by its manufacturer, is not guaranteed or endorsed by the publisher.
Supplementary material
The Supplementary material for this article can be found online at: https://www.frontiersin.org/articles/10.3389/fnut.2024.1431620/full#supplementary-material
References
1. Cassano, T, Villani, R, Pace, L, Carbone, A, Bukke, VN, Orkisz, S, et al. From Cannabis sativa to Cannabidiol: promising therapeutic candidate for the treatment of neurodegenerative diseases. Front Pharmacol. (2020) 11:124. doi: 10.3389/fphar.2020.00124
2. Lujan, MA, and Valverde, O. The pro-neurogenic effects of Cannabidiol and its potential therapeutic implications in psychiatric disorders. Front Behav Neurosci. (2020) 14:109. doi: 10.3389/fnbeh.2020.00109
3. Li, H, Chang, S, Chang, T, You, Y, Wang, X, Wang, L, et al. Inclusion complexes of cannabidiol with β-cyclodextrin and its derivative: physicochemical properties, water solubility, and antioxidant activity. J Mol Liq. (2021) 334:116070. doi: 10.1016/j.molliq.2021.116070
4. Hobbs, JM, Vazquez, AR, Remijan, ND, Trotter, RE, McMillan, TV, Freedman, KE, et al. Evaluation of pharmacokinetics and acute anti-inflammatory potential of two oral cannabidiol preparations in healthy adults. Phytother Res. (2020) 34:1696–703. doi: 10.1002/ptr.6651
5. Chen, JW, Borgelt, LM, and Blackmer, AB. Epidiolex (Cannabidiol):A new Hope for patients with Dravet or Lennox-Gastaut syndromes. Ann Pharmacother. (2019) 53:603–11. doi: 10.1177/1060028018822124
6. Vermersch, P. Sativex((R)) (tetrahydrocannabinol + cannabidiol), an endocannabinoid system modulator: basic features and main clinical data. Expert Rev Neurother. (2011) 11:15–9. doi: 10.1586/ern.11.27
7. Helen Chan, O, Schmid, HL, Kuo, B, Scott Wright, D, Howson, W, and Stewart, BH. Absorption of Cam-2445, an NK1 neurokinin receptor antagonist: in vivo, in situ, and in vitro evaluations. J Pharm Sci. (1996) 85:253–7. doi: 10.1021/js9503338
8. Bialer, M, Johannessen, SI, Koepp, MJ, Levy, RH, Perucca, E, Tomson, T, et al. Progress report on new antiepileptic drugs: a summary of the fourteenth Eilat conference on new antiepileptic drugs and devices (EILAT XIV). II. Drugs in more advanced clinical development. Epilepsia. (2018) 59:1842–66. doi: 10.1111/epi.14555
9. Louis, R. Cannabidiol (CBD) critical review report expert committee on drug dependence fortieth meeting. Geneva: (2018).
10. Perucca, E, and Bialer, M. Critical aspects affecting cannabidiol oral bioavailability and metabolic elimination, and related clinical implications. CNS Drugs. (2020) 34:795–800. doi: 10.1007/s40263-020-00741-5
11. Izgelov, D, Freidman, M, and Hoffman, A. Investigation of cannabidiol gastro retentive tablets based on regional absorption of cannabinoids in rats. Eur J Pharm Biopharm. (2020) 152:229–35. doi: 10.1016/j.ejpb.2020.05.010
12. Millar, SA, Maguire, RF, Yates, AS, and O’Sullivan, SE. Towards better delivery of cannabidiol (CBD). Pharmaceuticals. (2020) 13:219. doi: 10.3390/ph13090219
13. Acosta, E. Bioavailability of nanoparticles in nutrient and nutraceutical delivery. Curr Opin Colloid Interface Sci. (2009) 14:3–15. doi: 10.1016/j.cocis.2008.01.002
14. Ha, H, Kim, JW, Lee, M, Jun, W, and Lee, W. Cellular uptake and cytotoxicity of β-Lactoglobulin nanoparticles: the effects of particle size and surface charge. Asian Australas J Anim Sci. (2015) 28:420–7. doi: 10.5713/ajas.14.0761
15. Zakaria, MY, Abd El-Halim, SM, Beshay, BY, Zaki, I, and Abourehab, MAS. ‘Poly phenolic phytoceutical loaded nano-bilosomes for enhanced caco-2 cell permeability and SARS-CoV 2 antiviral activity’: in-vitro and in silico studies. Drug Deliv. (2023) 30:2162157. doi: 10.1080/10717544.2022.2162157
16. Zhang, C, Liu, Z, Wang, F, Zhang, B, Zhang, X, Guo, P, et al. Nanomicelles for GLUT1-targeting hepatocellular carcinoma therapy based on NADPH depletion. Drug Deliv. (2023) 30:2162160. doi: 10.1080/10717544.2022.2162160
17. Zhang, ZH, Wang, XP, Ayman, WY, Munyendo, WL, Lv, HX, and Zhou, JP. Studies on lactoferrin nanoparticles of gambogic acid for oral delivery. Drug Deliv. (2013) 20:86–93. doi: 10.3109/10717544.2013.766781
18. Verma, A, Uzun, O, Hu, Y, Hu, Y, Han, HS, Watson, N, et al. Surface-structure-regulated cell-membrane penetration by monolayer-protected nanoparticles. Nat Mater. (2008) 7:588–95. doi: 10.1038/nmat2202
19. Won, Y, Lim, KS, and Kim, Y. Intracellular organelle-targeted non-viral gene delivery systems. J Control Release. (2011) 152:99–109. doi: 10.1016/j.jconrel.2011.01.013
20. Shukla, R, and Cheryan, M. Zein: the industrial protein from corn. Ind Crop Prod. (2001) 13:171–92. doi: 10.1016/S0926-6690(00)00064-9
21. Wang, Y, and Padua, GW. Nanoscale characterization of zein self-assembly. Langmuir. (2012) 28:2429–35. doi: 10.1021/la204204j
22. Berardi, A, Bisharat, L, AlKhatib, HS, and Cespi, M. Zein as a pharmaceutical excipient in oral solid dosage forms: state of the art and future perspectives. AAPS PharmSciTech. (2018) 19:2009–22. doi: 10.1208/s12249-018-1035-y
23. Sousa, FFO, Luzardo-Álvarez, A, Blanco-Méndez, J, Otero-Espinar, FJ, Martín-Pastor, M, and Sández Macho, I. Use of 1H NMR STD, WaterLOGSY, and Langmuir monolayer techniques for characterization of drug–zein protein complexes. Eur J Pharm Biopharm. (2013) 85:790–8. doi: 10.1016/j.ejpb.2013.07.008
24. André de Almeida Campos, L, Francisco Silva Neto, A, Cecília Souza Noronha, M, Ferreira de Lima, M, Macário Ferro Cavalcanti, I, and Stela Santos-Magalhães, N. Zein nanoparticles for drug delivery: preparation methods and biological applications. Int J Pharm. (2023) 635:122754. doi: 10.1016/j.ijpharm.2023.122754
25. De Marco, I. Zein microparticles and nanoparticles as drug delivery systems. Polymers. (2022) 14:2172. doi: 10.3390/polym14112172
26. Paliwal, R, and Palakurthi, S. Zein in controlled drug delivery and tissue engineering. J Control Release. (2014) 189:108–22. doi: 10.1016/j.jconrel.2014.06.036
27. Liao, L, Zhao, M, Ren, J, Zhao, H, Cui, C, and Hu, X. Effect of acetic acid deamidation-induced modification on functional and nutritional properties and conformation of wheat gluten: acetic acid deamidation of wheat gluten. J Sci Food Agric. (2010) 90:409–17. doi: 10.1002/jsfa.3830
28. Zhou, L, Xu, G, Zhang, Z, Li, H, and Yao, P. Surface activity and safety of deamidated zein peptides. Colloids Surf A Physicochem Eng Asp. (2018) 540:150–7. doi: 10.1016/j.colsurfa.2017.12.070
29. Dai, L, Li, R, Wei, Y, Sun, C, Mao, L, and Gao, Y. Fabrication of zein and rhamnolipid complex nanoparticles to enhance the stability and in vitro release of curcumin. Food Hydrocoll. (2018) 77:617–28. doi: 10.1016/j.foodhyd.2017.11.003
30. van Ballegooie, C, Wretham, N, Ren, T, Popescu, IM, Yapp, DT, and Bally, MB. PEG conjugated Zein nanoparticles for in vivo use. Pharmaceutics. (2022) 14:1831. doi: 10.3390/pharmaceutics14091831
31. Ahammed, S, Easdani, M, Liu, F, and Zhong, F. Encapsulation of tea polyphenol in zein through complex coacervation technique to control the release of the phenolic compound from gelatin–zein composite film. Polymers. (2023) 15:2882. doi: 10.3390/polym15132882
32. Dong, S. Study on the loading capacity of flexible corn alcohol soluble protein nanoparticles with lutein. China Flavorings. (2022) 47:190–3. doi: 10.3969/j.issn.1000-9973.2022.09.038
33. Mattice, KD, and Marangoni, AG. Functionalizing zein through antisolvent precipitation from ethanol or aetic acid. Food Chem. (2020) 313:126127. doi: 10.1016/j.foodchem.2019.126127
34. Arzeni, C, Martínez, K, Zema, P, Arias, A, Pérez, OE, and Pilosof, AMR. Comparative study of high intensity ultrasound effects on food proteins functionality. J Food Eng. (2012) 108:463–72. doi: 10.1016/j.jfoodeng.2011.08.018
35. Wang, C, Wang, J, Sun, Y, Freeman, K, Mchenry, MA, Wang, C, et al. Enhanced stability and Oral bioavailability of Cannabidiol in Zein and whey protein composite nanoparticles by a modified anti-solvent approach. Food Secur. (2022) 11:376. doi: 10.3390/foods11030376
36. Midekessa, G, Godakumara, K, Ord, J, Viil, J, Lättekivi, F, Dissanayake, K, et al. Zeta potential of extracellular vesicles: toward understanding the attributes that determine colloidal stability. ACS Omega. (2020) 5:16701–10. doi: 10.1021/acsomega.0c01582
37. Kunarayakul, S, Thaiphanit, S, Anprung, P, and Suppavorasatit, I. Optimization of coconut protein deamidation using protein-glutaminase and its effect on solubility, emulsification, and foaming properties of the proteins. Food Hydrocoll. (2018) 79:197–207. doi: 10.1016/j.foodhyd.2017.12.031
38. Qu, Z, Chen, G, Yang, T, Li, S, and Chen, Y. Mechanisms underlying the formation of non-thermal deamidated zein via synergistic treatment of cold plasma with acid: improved foam performance. Food Hydrocoll. (2024) 146:109195. doi: 10.1016/j.foodhyd.2023.109195
39. Abdelbary, GA, Amin, MM, Zakaria, MY, and El Awdan, SA. Adefovir dipivoxil loaded proliposomal powders with improved hepatoprotective activity: formulation, optimization, pharmacokinetic, and biodistribution studies. J Liposome Res. (2018) 28:259–74. doi: 10.1080/08982104.2017.1363228
40. Jiang, F, Yang, L, Wang, S, Ying, X, Ling, J, and Ouyang, XK. Fabrication and characterization of zein-alginate oligosaccharide complex nanoparticles as delivery vehicles of curcumin. J Mol Liq. (2021) 342:116937. doi: 10.1016/j.molliq.2021.116937
41. Hurtado-López, P, and Murdan, S. Zein microspheres as drug/antigen carriers: a study of their degradation and erosion, in the presence and absence of enzymes. J Microencapsul. (2006) 23:303–14. doi: 10.1080/02652040500444149
42. Begines, B, Ortiz, T, Pérez-Aranda, M, Martínez, G, Merinero, M, Argüelles-Arias, F, et al. Polymeric nanoparticles for drug delivery: recent developments and future prospects. Nanomaterials. (2020) 10:1403. doi: 10.3390/nano10071403
43. Shirodkar, RK, Kumar, L, Mutalik, S, and Lewis, S. Solid lipid nanoparticles and nanostructured lipid carriers: emerging lipid based drug delivery systems. Pharm Chem J. (2019) 53:440–53. doi: 10.1007/s11094-019-02017-9
44. Stella, B, Baratta, F, Della Pepa, C, Arpicco, S, Gastaldi, D, and Dosio, F. Cannabinoid formulations and delivery systems: current and future options to treat pain. Drugs. (2021) 81:1513–57. doi: 10.1007/s40265-021-01579-x
45. Nakano, Y, Tajima, M, Sugiyama, E, Sato, VH, and Sato, H. Development of a novel nanoemulsion formulation to improve intestinal absorption of cannabidiol. Med Cann. (2019) 2:35–42. doi: 10.1159/000497361
46. Knaub, K, Sartorius, T, Dharsono, T, Wacker, R, Wilhelm, M, and Schön, C. A novel self-emulsifying drug delivery system (SEDDS) based on VESIsorb® formulation technology improving the oral bioavailability of cannabidiol in healthy subjects. Molecules. (2019) 24:2967. doi: 10.3390/molecules24162967
47. Li, Z, Jiang, H, Xu, C, and Gu, L. A review: using nanoparticles to enhance absorption and bioavailability of phenolic phytochemicals. Food Hydrocoll. (2015) 43:153–64. doi: 10.1016/j.foodhyd.2014.05.010
48. Zhou, J, Zheng, G, Wang, W, Yin, Z, Chen, J, Li, J, et al. Physicochemical properties and bioavailability comparison of two quercetin loading zein nanoparticles with outer shell of caseinate and chitosan. Food Hydrocoll. (2021) 120:106959. doi: 10.1016/j.foodhyd.2021.106959
49. Islam, MS, Reineke, J, Kaushik, R, Woyengo, T, Baride, A, Alqahtani, MS, et al. Bioadhesive food protein nanoparticles as pediatric oral drug delivery system. ACS Appl Mater Interfaces. (2019) 11:18062–73. doi: 10.1021/acsami.9b00152
Keywords: flexible zein, cannabidiol, nanoparticle, bioavailability, cannabidiol release
Citation: Nie Y, Kong Y, Peng J, Sun J and Fan B (2024) Enhanced oral bioavailability of cannabidiol by flexible zein nanoparticles: in vitro and pharmacokinetic studies. Front. Nutr. 11:1431620. doi: 10.3389/fnut.2024.1431620
Edited by:
Haohao Wu, Ocean University of China, ChinaReviewed by:
Sutapa Biswas Majee, NSHM Knowledge Campus, IndiaZhengze Li, North Dakota State University, United States
Copyright © 2024 Nie, Kong, Peng, Sun and Fan. This is an open-access article distributed under the terms of the Creative Commons Attribution License (CC BY). The use, distribution or reproduction in other forums is permitted, provided the original author(s) and the copyright owner(s) are credited and that the original publication in this journal is cited, in accordance with accepted academic practice. No use, distribution or reproduction is permitted which does not comply with these terms.
*Correspondence: Bin Fan, binf@263.net
†These authors have contributed equally to this work and share first authorship