- 1Graduate School, Changchun University of Traditional Chinese Medicine, Changchun, Jilin, China
- 2Geriatric Department, Suzhou Hospital of Integrated Traditional Chinese and Western Medicine, Suzhou, Jiangsu, China
- 3Respiratory Disease Department, Affiliated Hospital of Changchun University of Traditional Chinese Medicine, Changchun, Jilin, China
Objective: Dietary factors and nutritional status may be among the risk factors for Chronic Obstructive Pulmonary Disease (COPD). There exists a certain correlation between trace elements and COPD. Through Mendelian Randomization (MR) analysis, we investigated the causal relationships between trace elements, inflammatory proteins, and COPD.
Methods: We employed MR, multivariable MR (MVMR), and two-step MR (TSMR) approaches to assess the causal links between 15 trace elements and COPD, with 91 inflammatory proteins serving as mediators to further elucidate the tripartite causal relationships.
Results: Trace elements such as Folate (OR = 1.293, 95%CI 1.027–1.628; p = 0.029), Vitamin D (OR = 1.331, 95%CI 1.071–1.654; p = 0.010), Vitamin B12 (OR = 1.424, 95%CI 1.108–1.828; p = 0.006), and Iron (OR = 0.741, 95%CI 0.580–0.946; p = 0.016) demonstrated causal relationships with COPD. No causal relationship was observed in reverse MR. After adjusting for BMI, Folate (OR = 1.633, 95%CI 1.098–2.429; p = 0.015), Iron (OR = 0.507, 95%CI 0.31–0.778; p = 0.001), and Vitamin D (OR = 1.511, 95%CI 1.029–2.217; p = 0.034) were identified as independent risk factors for COPD, whereas Vitamin B12 (OR = 1.118, 95%CI 0.751–1.666; p = 0.581) was not. Mediation analysis indicated that CDCP1 (5.76%) may play a mediating role between Iron and COPD.
Conclusion: Trace elements such as Folate, Vitamin D, Vitamin B12, and Iron have causal relationships with COPD. After BMI adjustment, Folate, Vitamin D, and Iron emerge as independent risk factors. Furthermore, the inflammatory protein CDCP1 may partially mediate the causal relationship between Iron and COPD, offering a scientific basis for dietary recommendations that could benefit COPD patients. The supplementation of trace elements may be advantageous for individuals suffering from COPD.
1 Introduction
Chronic Obstructive Pulmonary Disease (COPD) is a heterogeneous ailment that is progressively becoming the third leading cause of death globally (1). It is primarily characterized by airway pathologies (bronchitis, bronchiolitis) and/or alveolar abnormalities (emphysema) leading to chronic respiratory symptoms (dyspnea, cough, expectoration) and a persistent, progressive limitation of airflow (2). Studies have revealed that nearly half of COPD patients experience weight loss (3) and diminished appetite (4), often resulting in an intake of trace elements significantly below the recommended dietary allowances (5). Observational studies have identified that malnutrition and weight loss are prevalent among COPD outpatient attendees (6), and nutritional supplementation can enhance the quality of life for these patients (7). Trace elements play a protective role in lung function, potentially decelerating the rate of pulmonary decline (8). They also influence the diffusing capacity of the lungs and the strength of the respiratory muscles (9). Deficiencies in trace elements are common in COPD and may influence the progression of the disease (10). Dietary interventions and targeted supplementation of single or multiple trace elements could be beneficial for patients with COPD (11).
Dietary factors and nutritional status may be among the risk factors for COPD. Alterations in dietary habits can modulate the impact of adverse environmental exposures on the lungs (12). For instance, excessive consumption of processed red meat has been associated with an increased risk of developing COPD (13), whereas a high dietary fiber intake is inversely related to the risk of COPD (14). Malnutrition can heighten the risk of mortality in patients with COPD (15), underscoring the pivotal role that nutrition plays in respiratory diseases (16). Relevant studies have identified that diet can influence the development of COPD through three primary mechanisms, with the most significant being the modulation of inflammation (17). Inflammatory responses are correlated with various diseases (18–20), and the intake of trace elements can alleviate the inflammatory reactions associated with COPD (21). Metal ions such as iron and copper in trace elements are crucial to the presence of pulmonary inflammation and oxidative stress in COPD (22), potentially leading to diminished activity of macrophages (23). Exposure to environments like iron factories increases the risk of COPD (24), whereas improving environmental risks can decrease it (25). Inhibiting ferroptosis may alleviate emphysema and airway inflammation (26). There is a correlation between copper and pulmonary inflammation (27). Zinc can mitigate the progression of COPD induced by harmful gasses and offers protective benefits to lung tissue (28). There is also a correlation between zinc and the pathogenesis of COPD (29). Supplementing with vitamins A and K may reduce the risk of emphysema (30), with vitamin K potentially improving the condition (31). Carotene is correlated with lung function (32) and may enhance pulmonary health (33). Vitamin D is associated with respiratory diseases (34), and vitamin E can reduce the risk of COPD (35). Thus, trace elements may be significant influencing factors for patients with COPD (36).
Although observational studies and systematic reviews have established a connection between trace elements, nutritional status (37–41), and COPD, suggesting that malnutrition and deficiencies in trace elements can adversely affect COPD patients, the precise causal relationships and underlying mechanisms remain unclear. Mendelian randomization (MR) is a potential method for causal inference, used to estimate the causal effects of exposure factors on outcomes while controlling for confounding factors and avoiding reverse causation (42). Therefore, we aim to utilize MR analysis to elucidate the causal relationships between trace elements, inflammatory factors, and COPD, thereby providing scientifically sound dietary recommendations for COPD patients.
2 Methods
2.1 Study design
This study employs MR analysis, focusing on 15 trace elements, including Copper, Calcium, Folate, Iron, Vitamin D, and Vitamin B6, as the primary exposures, with COPD as the outcome. To further explore the mechanisms underlying the causal relationship between trace elements and COPD, we consider 91 inflammatory proteins as potential mediators to determine whether these proteins play a significant mediating role in the causal pathway between trace elements and COPD. This research adheres to the guidelines of the Strengthening the Reporting of Observational Studies in Epidemiology using Mendelian Randomization (STROBE-MR) Statement (43).
Our MR analysis is structured into three distinct phases. Initially, we employ a two-sample MR approach to investigate whether a causal relationship exists between trace elements and COPD, and to ascertain the presence of any reverse causality, thereby determining the feasibility of further mediation analysis. Subsequently, after adjusting for BMI, we conduct MVMR to identify which trace elements independently contribute to risk. Lastly, we utilize TSMR to examine whether the causal effects are mediated by any of the 91 inflammatory proteins, thus performing mediation analysis and elucidating the proportion of the mediation effect (Figure 1).
2.2 Data sources
The genetic information for the 15 trace elements is sourced from the GWAS database,1 all pertaining to European populations. The data for the 91 inflammatory proteins are derived from a 2023 study involving 14,824 Europeans (44), cataloged under the identifiers GCST90274758 to GCST90274848. The COPD data is obtained from the tenth round of analysis by the FinnGen database (45),2 also concerning European populations. Additionally, the genetic information for BMI is acquired from the GWAS database and is likewise representative of European demographics (Table 1).
2.3 Instrumental variable selection
The selection of instrumental variables must satisfy several assumptions (46): the instrumental variables should be closely associated with trace elements, independent of confounding factors in the exposure-outcome relationship, and must influence COPD solely through the trace elements (47). To ensure their relevance (48), we conduct an association analysis on the 15 trace elements using a significance threshold of p < 5 × 10−6. Subsequently, we eliminate any single nucleotide polymorphisms (SNPs) exhibiting linkage disequilibrium by applying criteria of R2 < 0.001 and Kb = 10,000 (49). We then calculate the F-statistic for the selected SNPs to exclude weak instrumental variables, considering an F-value greater than 10 as indicative of the absence of weak instrumental variables (50, 51).
2.4 Statistical analysis
We employed five methods to assess causality: Inverse Variance Weighted (IVW), MR-Egger, Weighted Median, Simple Mode, and Weighted Mode, with IVW serving as the primary method (47, 52). A p-value less than 0.05 indicates a causal relationship (53), while the other four methods serve as supplementary approaches (54). To evaluate the robustness of our results, we conducted sensitivity analysis using the “leave-one-out” technique (55). Additionally, we employed Cochran’s Q test, MR-Egger intercept test, and MR-PRESSO to test for pleiotropy and heterogeneity (56, 57), with a p-value greater than 0.05 indicating the absence of both (58, 59). Using the TSMR approach, we first calculated the total effect (β0) of trace elements on COPD, the effect of trace elements on inflammatory proteins (β1), and the effect of inflammatory proteins on COPD (β2). The mediating effect was computed as β1*β2, and the direct effect as the total effect minus the mediating effect. The proportion mediated was calculated as (β1 × β2)/β0 (60). All analyses were conducted using the R language (version 4.3.3). The specific package employed was TwoSampleMR (version 0.6.0).
3 Results
3.1 Causal relationship between 15 trace elements and COPD
Through the judicious selection of instrumental variables, we conducted an associative analysis, eliminated linkage disequilibrium and weak instrumental variables, and identified 188 SNPs across 15 trace elements, with the smallest F-statistic being 20.86 and the largest 84.68. Univariate MR analysis supports a causal relationship between trace elements such as Folate, Vitamin D, Vitamin B12, and Iron, and COPD. The results of the IVW analysis indicate a positive correlation between Folate (OR = 1.293, 95% CI 1.027–1.628; p = 0.029), Vitamin D (OR = 1.331, 95% CI 1.071–1.654; p = 0.010), and Vitamin B12 (OR = 1.424, 95% CI 1.108–1.828; p = 0.006) with COPD, while Iron shows a negative correlation (OR = 0.741, 95% CI 0.580–0.946; p = 0.016). Concurrently, reverse MR analysis revealed no reverse causality between Folate, Vitamin D, Vitamin B12, and Iron with COPD (p > 0.05).
To evaluate the robustness of our analytical results, we employed Cochran’s Q test, the MR-Egger intercept test, and MR-PRESSO to examine pleiotropy and heterogeneity. No evidence of pleiotropy or heterogeneity was detected (p > 0.05). The leave-one-out analysis indicated that the exclusion of any single SNP would not significantly affect the estimation of causal relationships, suggesting that the results of the MR analysis are robust (Table 2; Figure 2).
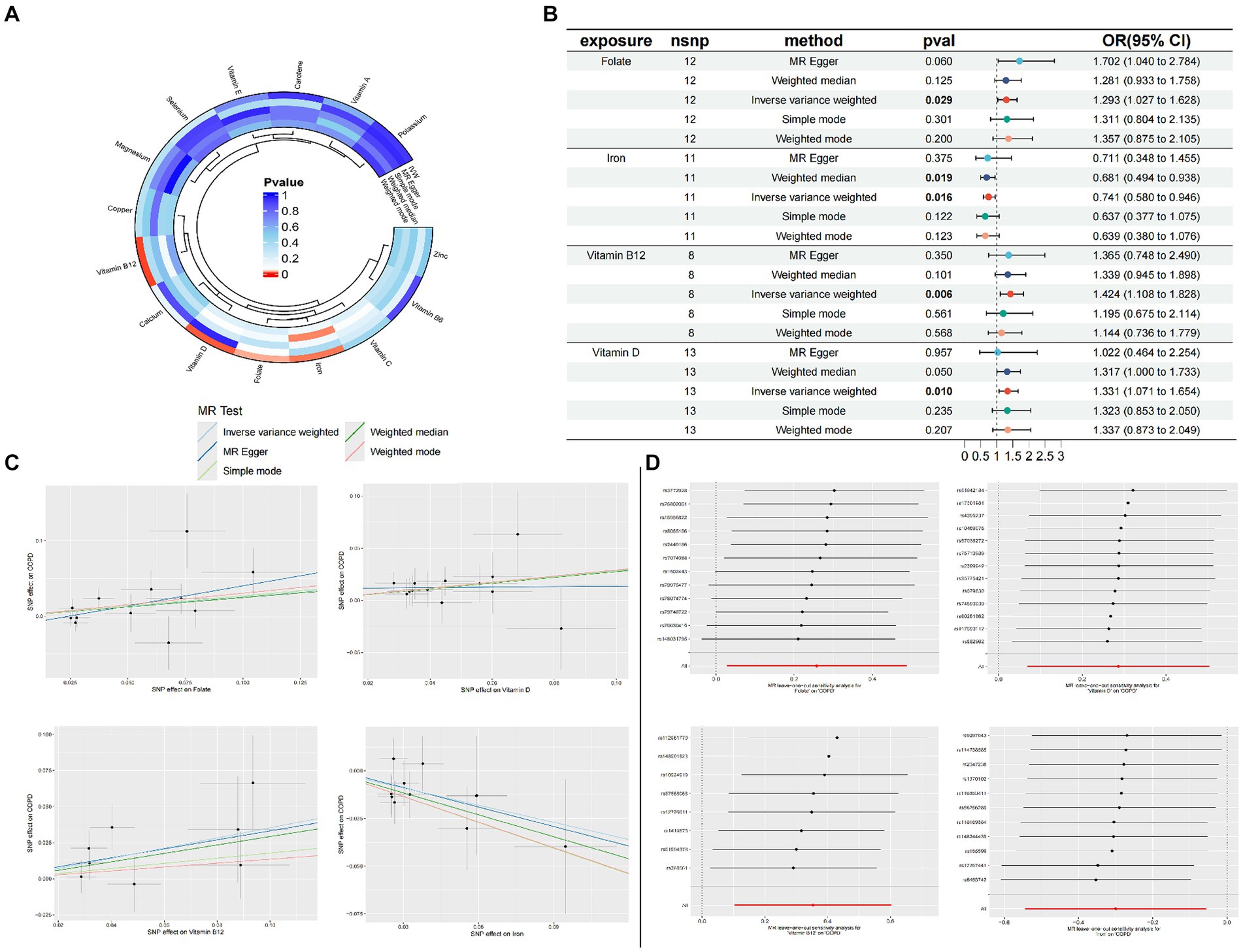
Figure 2. Circle plots of the five Mendelian randomization methods (p < 0.05) (A); Forest plot of MR Analysis of trace elements and COPD (B); MR scatter plot of trace elements and COPD (C); Result of leave-one-out sensitivity analysis of trace elements and COPD (D).
3.2 Multivariate MR analysis
According to the results of the univariate MR analysis, a causal relationship exists between Folate, Vitamin D, Vitamin B12, and Iron with COPD. By adjusting for the influence of Body Mass Index (BMI), we conducted a MVMR analysis with these four trace elements and BMI. We discovered that the causal relationships with COPD persist for Folate (OR = 1.633, 95% CI 1.098–2.429; p = 0.015), Iron (OR = 0.507, 95% CI 0.31–0.778; p = 0.001), and Vitamin D (OR = 1.511, 95% CI 1.029–2.217; p = 0.034), indicating that Folate, Vitamin D, and Iron are independent risk factors for COPD. However, Vitamin B12 (OR = 1.118, 95% CI 0.751–1.666; p = 0.581) is not an independent risk factor for COPD (Table 3).
3.3 TSMR and mediation analyses
We conducted a TSMR analysis, selecting 91 inflammatory proteins as instrumental variables. After analyzing associations, removing linkage disequilibrium, and excluding weak instrumental variables, we obtained 2,973 SNPs with the smallest F-statistic being 19.51 and the largest 1472.73. The univariate MR analysis of these 91 inflammatory proteins with COPD revealed positive causal relationships for CXCL10 (OR = 1.093, 95% CI 1.034–1.155; p = 0.001), EN-RAGE (OR = 1.117, 95% CI 1.041–1.198; p = 0.002), CD6 (OR = 1.064, 95% CI 1.022–1.107; p = 0.002), STAMPB (OR = 1.104, 95% CI 1.012–1.205; p = 0.025), and CXCL6 (OR = 1.062, 95% CI 1.015–1.112; p = 0.008). Conversely, negative causal relationships were observed for CD40 (OR = 0.948, 95% CI 0.903–0.997; p = 0.038) and CDCP1 (OR = 0.940, 95% CI 0.899–0.982; p = 0.006). Tests for pleiotropy and heterogeneity were conducted (p > 0.05), with consistent OR directions, and the leave-one-out analysis confirmed the robustness of the MR results (Table 4).
In further MR analyses of four trace elements and inflammatory proteins, we found positive correlations between Iron and CDCP1 (OR = 1.321, 95% CI 1.026–1.702; p = 0.031), as well as Iron and CXCL10 (OR = 1.389, 95% CI 1.070–1.803; p = 0.013). Conversely, negative correlations were observed between Folate and EN-RAGE (OR = 0.750, 95% CI 0.583–0.964; p = 0.025), and between Vitamin D (OR = 0.724, 95% CI 0.563–0.930; p = 0.011) and EN-RAGE (Table 5).
In our final mediation analysis, we elucidated the causal effect proportions of four trace elements on COPD, mediated by seven inflammatory proteins. It was discovered that only CDCP1 mediated the impact of iron on COPD, with a mediation effect of −0.282, a direct effect of −0.017, and a mediation proportion of 5.76%. Regrettably, the other mediation effects were not established (Figure 3).
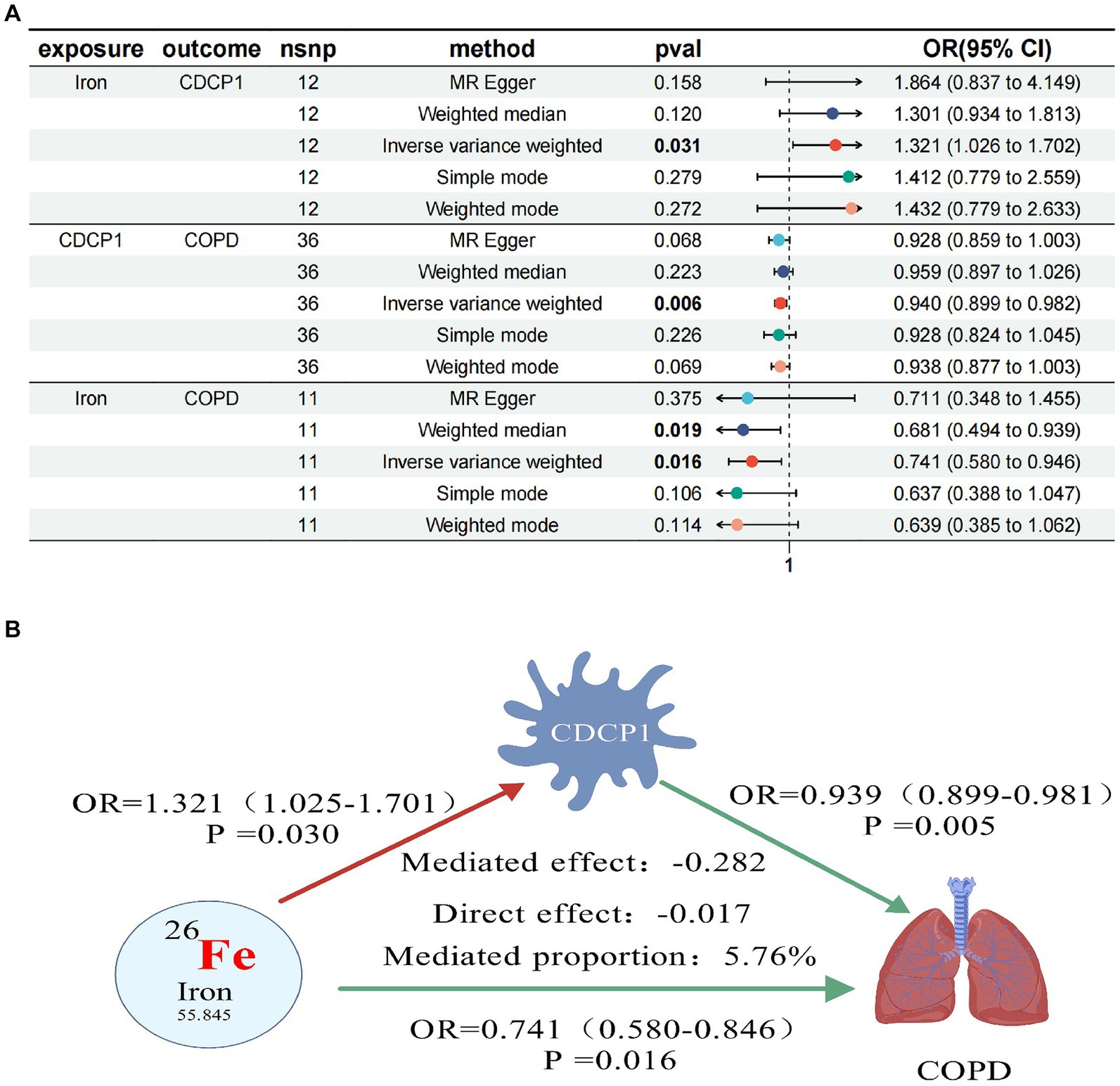
Figure 3. Forest plots of trace elements iron, CDCP1 and COPD (A); CDCP1 mediates causal relationship between trace element iron and COPD (red is a risk factor, green is a protective factor) (B).
4 Discussion
This study provides genetic evidence supporting the causal relationships between trace elements such as Folate, Vitamin D, Vitamin B12, and Iron, and COPD in univariate MR analysis. After adjusting for BMI, further MVMR analysis revealed that Folate, Vitamin D, and Iron are independent risk factors for COPD. Finally, through TSMR and mediation analysis, CDCP1 is suggested to partially mediate the causal relationship between Iron and COPD. Our findings offer insights into dietary management and trace element supplementation for patients with COPD.
Malnutrition and trace element deficiencies are integral components of the rehabilitation process for patients with COPD, exhibiting a profound connection (61). Compared to healthy controls, COPD patients exhibit significantly reduced levels of Folate, presenting a novel therapeutic target for the treatment of COPD (62). Folate possesses antioxidative properties (63) and the capability to ameliorate endoplasmic reticulum stress (64), correlating positively with pulmonary function in COPD patients (65), thereby enhancing lung function (66) and alleviating respiratory distress (67). A reduction in Folate intake may lead to restricted airflow (68), whereas increasing Folate intake could potentially benefit pulmonary function (69). Folate may confer protective effects against acute lung injury by mitigating inflammatory responses (70). Serum Folate levels are positively correlated with lung function in elderly males (71) and are also associated with pulmonary function in children with asthma (72). However, supplementation with Folate does not influence changes in FEV1 (67), nor has a significant correlation been observed between serum Folate levels and lung function in females (65). These results present contradictions, and our MR analysis serves as a complement to observational studies and systematic reviews. Vitamin D plays a crucial role in both innate and adaptive immunity (73) and acts as a significant regulator in defending against pulmonary infectionss (74). It may also contribute to reducing mortality from respiratory diseases. Additionally (75), supplementation with Vitamin D alone can enhance lung function (5). Prospective studies have identified a correlation between lower Vitamin D levels and accelerated decline in lung function (76). Systematic reviews have concluded that Vitamin D supplementation can reduce the risk of respiratory infections (34) and enhance resistance to such infections (77). In COPD patients, the response to Vitamin D supplementation is diminished compared to healthy controls (78), and supplementation does not affect the muscular response to resistance training in COPD patients treated with Vitamin D3 (79). While some studies suggest that Vitamin D supplementation does not reduce the exacerbation rate of COPD (80), it is inversely related to inflammatory signaling in COPD (81). A deficiency in Vitamin D receptors may increase pulmonary inflammation (82), and Vitamin D may inhibit COPD-related pulmonary emphysema by maintaining the homeostasis and functionality of alveolar macrophages (83). Despite some contradictions in research concerning Vitamin D and COPD (84), our analyses using MR and MVMR have established a causal relationship between Vitamin D and COPD.
Vitamin B12, as a supplement in the rehabilitation of COPD patients, can regulate the secretion of NT-proBNP (85), exerting a positive effect on patients with advanced COPD (86). However, the intake of Vitamin B12 is not associated with the risk of frailty in COPD. After adjusting for BMI, our multivariate MR analysis indicates that Vitamin B12 is not an independent risk factor for COPD (87). Iron regulation is significantly associated with respiratory diseases (88). Dysregulation of iron homeostasis is a critical mechanism in lung injury (89). Iron-induced cell death can lead to airway remodeling and emphysema (90), exacerbating inflammation and oxidative stress (91). Targeting iron-induced cell death may ameliorate respiratory diseases (92) and alleviate the progression of COPD (93). Iron is related to the genetic susceptibility of COPD (94, 95), and COPD patients may experience non-anemic iron deficiency (96), which is associated with inflammatory responses (97), skeletal muscle disorders (98), hypoxemia, and reduced exercise tolerance (99). Clinical studies have shown that iron supplementation can improve the exercise endurance and quality of life of COPD patients (100, 101). Non-anemic iron deficiency can impair the response of COPD patients to pulmonary rehabilitation, resulting in lower aerobic capacity (102). Iron deficiency is linked to more severe pulmonary vascular diseases (103). Dysregulation of iron homeostasis in the lungs and cellular iron accumulation are factors in the development of COPD (104). Ferroptosis, an iron-dependent form of cell death, plays a role in the pathogenesis of COPD (105) and can ameliorate cigarette smoke-induced inflammation and emphysema (106). CXCL10 is a potential biomarker for impaired lung development (107), capable of modulating pulmonary inflammation (108) and the lung microenvironment (109). There is a correlation between EN-RAGE and COPD (110). CD6 serves as a therapeutic target in cancer immunotherapy (111), while CD40 is associated with the severity of COPD and the degree of pulmonary function alteration (112). Additionally, a correlation exists between CXCL6 and mortality in IPF (113). CDCP1, which may be involved in cell adhesion and matrix binding, could serve as a biomarker for lung cancer detection (114) and is somewhat associated with COVID-19 (115). Our research suggests that iron may mediate the effects on COPD through its influence on the inflammatory protein CDCP1, necessitating further exploration of the relationship between inflammatory responses, trace elements, and COPD.
This study, through MR analysis, investigates the causal relationships between trace elements, inflammatory proteins, and COPD, aiming to provide scientifically sound dietary recommendations for COPD patients and further suggest that supplementation with trace elements may be beneficial for COPD. This research has certain limitations; primarily, the study population is confined to Europeans, which may restrict the generalizability of the findings. Secondly, there is a need for a deeper exploration of the mechanisms linking trace elements, inflammatory proteins, and COPD, as the mediating effects observed were not significant, necessitating further.
5 Conclusion
In conclusion, our research demonstrates a causal relationship between genetically predicted trace elements such as Folate, Vitamin D, Vitamin B12, and Iron, and COPD. After adjusting for BMI, Folate, Vitamin D, and Iron emerge as independent risk factors for COPD. Furthermore, the inflammatory protein CDCP1 may play a partial mediating role in the causal relationship between Iron and COPD. Our findings can better inform scientifically sound dietary recommendations for patients, suggesting that supplementation with trace elements may be beneficial for those suffering from COPD.
Data availability statement
The original contributions presented in the study are included in the article/Supplementary material, further inquiries can be directed to the corresponding authors.
Ethics statement
Ethical approval was not required for the study involving humans in accordance with the local legislation and institutional requirements. Written informed consent to participate in this study was not required from the participants or the participants’ legal guardians/next of kin in accordance with the national legislation and the institutional requirements.
Author contributions
ZC: Conceptualization, Visualization, Writing – original draft, Writing – review & editing. SZ: Data curation, Methodology, Writing – original draft, Writing – review & editing. TW: Data curation, Investigation, Writing – original draft, Writing – review & editing. FS: Data curation, Writing – original draft, Writing – review & editing. HD: Data curation, Writing – original draft, Writing – review & editing. SH: Conceptualization, Funding acquisition, Supervision, Writing – original draft, Writing – review & editing. LS: Conceptualization, Funding acquisition, Supervision, Writing – original draft, Writing – review & editing.
Funding
The author(s) declare that no financial support was received for the research, authorship, and/or publication of this article.
Acknowledgments
We want to acknowledge the participants and investigators of the FinnGen study and GWAS, as well as all the authors for their contributions to this article.
Conflict of interest
The authors declare that the research was conducted in the absence of any commercial or financial relationships that could be construed as a potential conflict of interest.
Publisher’s note
All claims expressed in this article are solely those of the authors and do not necessarily represent those of their affiliated organizations, or those of the publisher, the editors and the reviewers. Any product that may be evaluated in this article, or claim that may be made by its manufacturer, is not guaranteed or endorsed by the publisher.
Supplementary material
The Supplementary material for this article can be found online at: https://www.frontiersin.org/articles/10.3389/fnut.2024.1430606/full#supplementary-material
Footnotes
References
1. WHO. Global health estimates: leading causes of death. Cause-specific mortality 2000–2019. Available at: https://www.who.int/data/gho/data/themes/mortality-and-global-health-estimates/ghe-leading-causes-of-death.
2. Global Initiative for Chronic Obstructive Lung Disease (Gold). Global strategy for the diagnosis, management and prevention of chronic obstructive lung disease (2024 Report). Available at: https://goldcopd.org/.
3. Keogh, E, and Mark, WE. Managing malnutrition in COPD: a review. Respir Med. (2021) 176:106248. doi: 10.1016/j.rmed.2020.106248
4. Fekete, M, Csipo, T, Fazekas-Pongor, V, Feher, A, Szarvas, Z, Kaposvari, C, et al. The effectiveness of supplementation with key vitamins, minerals, antioxidants and specific nutritional supplements in COPD-a review. Nutrients. (2023) 15:2741. doi: 10.3390/nu15122741
5. Li, M, Zhao, L, Hu, C, Li, Y, Yang, Y, Zhang, X, et al. Improvement of lung function by micronutrient supplementation in patients with COPD: a systematic review and meta-analysis. Nutrients. (2024) 16:1028. doi: 10.3390/nu16071028
6. Laudisio, A, Costanzo, L, Di Gioia, C, Delussu, AS, Traballesi, M, Gemma, A, et al. Dietary intake of elderly outpatients with chronic obstructive pulmonary disease. Arch Gerontol Geriatr. (2016) 64:75–81. doi: 10.1016/j.archger.2016.01.006
7. Nguyen, HT, Collins, PF, Pavey, TG, Nguyen, NV, Pham, TD, and Gallegos, DL. Nutritional status, dietary intake, and health-related quality of life in outpatients with COPD. Int J Chron Obstruct Pulmon Dis. (2019) 14:215–26. doi: 10.2147/COPD.S181322
8. Zhai, T, Li, S, Hu, W, Li, D, and Leng, S. Potential micronutrients and phytochemicals against the pathogenesis of chronic obstructive pulmonary disease and lung cancer. Nutrients. (2018) 10:813. doi: 10.3390/nu10070813
9. Collins, PF, Yang, IA, Chang, YC, and Vaughan, A. nutritional support in chronic obstructive pulmonary disease (COPD): an evidence update. J Thorac Dis. (2019) 11:S2230–7. doi: 10.21037/jtd.2019.10.41
10. Huang, WJ, Fan, XX, Yang, YH, Zeng, YM, and Ko, CY. A review on the role of oral nutritional supplements in chronic obstructive pulmonary disease. J Nutr Health Aging. (2022) 26:723–31. doi: 10.1007/s12603-022-1822-8
11. Beijers, R, Steiner, MC, and Schols, A. The role of diet and nutrition in the management of COPD. Eur Respir Rev. (2023) 32:230003. doi: 10.1183/16000617.0003-2023
12. Whyand, T, Hurst, JR, Beckles, M, and Caplin, ME. Pollution and respiratory disease: can diet or supplements help? A review. Respir Res. (2018) 19:79. doi: 10.1186/s12931-018-0785-0
13. Salari-Moghaddam, A, Milajerdi, A, Larijani, B, and Esmaillzadeh, A. Processed red meat intake and risk of COPD: a systematic review and dose-response meta-analysis of prospective cohort studies. Clin Nutr. (2019) 38:1109–16. doi: 10.1016/j.clnu.2018.05.020
14. Szmidt, MK, Kaluza, J, Harris, HR, Linden, A, and Wolk, A. Long-term dietary fiber intake and risk of chronic obstructive pulmonary disease: a prospective cohort study of women. Eur J Nutr. (2020) 59:1869–79. doi: 10.1007/s00394-019-02038-w
15. Marco, E, Sanchez-Rodriguez, D, Davalos-Yerovi, VN, Duran, X, Pascual, EM, Muniesa, JM, et al. Malnutrition according to ESPEN consensus predicts hospitalizations and long-term mortality in rehabilitation patients with stable chronic obstructive pulmonary disease. Clin Nutr. (2019) 38:2180–6. doi: 10.1016/j.clnu.2018.09.014
16. Karim, T, Muhit, M, and Khandaker, G. Interventions to prevent respiratory diseases-nutrition and the developing world. Paediatr Respir Rev. (2017) 22:31–7. doi: 10.1016/j.prrv.2016.09.003
17. Marin-Hinojosa, C, Eraso, CC, Sanchez-Lopez, V, Hernandez, LC, Otero-Candelera, R, and Lopez-Campos, JL. Nutriepigenomics and chronic obstructive pulmonary disease: potential role of dietary and epigenetics factors in disease development and management. Am J Clin Nutr. (2021) 114:1894–906. doi: 10.1093/ajcn/nqab267
18. Cai, X, Song, S, Hu, J, Wang, L, Shen, D, Zhu, Q, et al. Systemic inflammation response index as a predictor of stroke risk in elderly patients with hypertension: a cohort study. J Inflamm Res. (2023) 16:4821–32. doi: 10.2147/JIR.S433190
19. Ma, H, Cai, X, Hu, J, Song, S, Zhu, Q, Zhang, Y, et al. Association of systemic inflammatory response index with bone mineral density, osteoporosis, and future fracture risk in elderly hypertensive patients. Postgrad Med. (2024) 136:406–16. doi: 10.1080/00325481.2024.2354158
20. Guo, Y, Liu, Q, Zheng, Z, Qing, M, Yao, T, Wang, B, et al. Genetic association of inflammatory marker glyca with lung function and respiratory diseases. Nat Commun. (2024) 15:3751. doi: 10.1038/s41467-024-47845-w
21. Scoditti, E, Massaro, M, Garbarino, S, and Toraldo, DM. Role of diet in chronic obstructive pulmonary disease prevention and treatment. Nutrients. (2019) 11:1357. doi: 10.3390/nu11061357
22. Cui, Y, Du, X, Li, Y, Wang, D, Lv, Z, Yuan, H, et al. Imbalanced and unchecked: the role of metal dyshomeostasis in driving COPD progression. COPD. (2024) 21:2322605. doi: 10.1080/15412555.2024.2322605
23. Han, L, Zhu, W, Qi, H, He, L, Wang, Q, Shen, J, et al. The cuproptosis-related gene glutaminase promotes alveolar macrophage copper ion accumulation in chronic obstructive pulmonary disease. Int Immunopharmacol. (2024) 129:111585. doi: 10.1016/j.intimp.2024.111585
24. Lenander-Ramirez, A, Bryngelsson, IL, Vihlborg, P, Westberg, H, and Andersson, L. Respirable dust and silica: respiratory diseases among Swedish iron foundry workers. J Occup Environ Med. (2022) 64:593–8. doi: 10.1097/JOM.0000000000002533
25. Hua, C, Ma, W, Zheng, F, Zhang, Y, Xie, J, Ma, L, et al. Health risks and sources of trace elements and black carbon in Pm(2.5) from 2019 to 2021 in Beijing. J Environ Sci (China). (2024) 142:69–82. doi: 10.1016/j.jes.2023.05.023
26. Wang, Y, Liao, S, Pan, Z, Jiang, S, Fan, J, Yu, S, et al. Hydrogen sulfide alleviates particulate matter-induced emphysema and airway inflammation by suppressing ferroptosis. Free Radic Biol Med. (2022) 186:1–16. doi: 10.1016/j.freeradbiomed.2022.04.014
27. Jiang, C, Wu, B, Xue, M, Lin, J, Hu, Z, Nie, X, et al. Inflammation accelerates copper-mediated cytotoxicity through induction of six-transmembrane epithelial antigens of prostate 4 expression. Immunol Cell Biol. (2021) 99:392–402. doi: 10.1111/imcb.12427
28. Luan, R, Ding, D, Xue, Q, Li, H, Wang, Y, and Yang, J. Protective role of zinc in the pathogenesis of respiratory diseases. Eur J Clin Nutr. (2023) 77:427–35. doi: 10.1038/s41430-022-01191-6
29. Liu, X, Ali, MK, Dua, K, and Xu, R. The role of zinc in the pathogenesis of lung disease. Nutrients. (2022) 14:2115. doi: 10.3390/nu14102115
30. Shen, T, Bimali, M, Faramawi, M, and Orloff, MS. Consumption of vitamin K and vitamin a are associated with reduced risk of developing emphysema: Nhanes 2007-2016. Front Nutr. (2020) 7:47. doi: 10.3389/fnut.2020.00047
31. Paulus, MC, Drent, M, Kouw, IWK, Balvers, MGJ, Bast, A, and van Zanten, ARH. Vitamin K: a potential missing link in critical illness-a scoping review. Crit Care. (2024) 28:212. doi: 10.1186/s13054-024-05001-2
32. Thyagarajan, B, Meyer, KA, Smith, LJ, Beckett, WS, Williams, OD, Gross, MD, et al. Serum carotenoid concentrations predict lung function evolution in young adults: the coronary artery risk development in young adults (CARDIA) study. Am J Clin Nutr. (2011) 94:1211–8. doi: 10.3945/ajcn.111.019067
33. Jun, L, and Root, M. Association of carotenoid intake with pulmonary function. J Am Coll Nutr. (2021) 40:708–12. doi: 10.1080/07315724.2020.1815608
34. Jolliffe, DA, Camargo, CA Jr, Sluyter, JD, Aglipay, M, Aloia, JF, Ganmaa, D, et al. Vitamin D supplementation to prevent acute respiratory infections: a systematic review and meta-analysis of aggregate data from randomised controlled trials. Lancet Diabetes Endocrinol. (2021) 9:276–92. doi: 10.1016/S2213-8587(21)00051-6
35. Liu, Z, Su, Y, Chen, Q, Xiao, L, Zhao, X, Wang, F, et al. Association of dietary intake of vitamin E with chronic obstructive pulmonary disease events in US adults: a cross-sectional study of Nhanes 2013-2018. Front Nutr. (2023) 10:1124648. doi: 10.3389/fnut.2023.1124648
36. Lepp, HL, Amrein, K, Dizdar, OS, Casaer, MP, Gundogan, K, de Man, AME, et al. Lll 44- module 3: micronutrients in chronic disease. Clin Nutr ESPEN. (2024) 62:285–95. doi: 10.1016/j.clnesp.2024.05.009
37. Deng, M, Lu, Y, Zhang, Q, Bian, Y, Zhou, X, and Hou, G. Global prevalence of malnutrition in patients with chronic obstructive pulmonary disease: systemic review and meta-analysis. Clin Nutr. (2023) 42:848–58. doi: 10.1016/j.clnu.2023.04.005
38. Collins, PF, Stratton, RJ, and Elia, M. Nutritional support in chronic obstructive pulmonary disease: a systematic review and meta-analysis. Am J Clin Nutr. (2012) 95:1385–95. doi: 10.3945/ajcn.111.023499
39. Varraso, R, and Camargo, CA Jr. More evidence for the importance of nutritional factors in chronic obstructive pulmonary disease. Am J Clin Nutr. (2012) 95:1301–2. doi: 10.3945/ajcn.112.039834
40. Deutz, NE, Ziegler, TR, Matheson, EM, Matarese, LE, Tappenden, KA, Baggs, GE, et al. Reduced mortality risk in malnourished hospitalized older adult patients with COPD treated with a specialized oral nutritional supplement: sub-group analysis of the nourish study. Clin Nutr. (2021) 40:1388–95. doi: 10.1016/j.clnu.2020.08.031
41. Ter Beek, L, van der Vaart, H, Wempe, JB, Krijnen, WP, Roodenburg, JLN, van der Schans, CP, et al. Coexistence of malnutrition, frailty, physical frailty and disability in patients with COPD starting a pulmonary rehabilitation program. Clin Nutr. (2020) 39:2557–63. doi: 10.1016/j.clnu.2019.11.016
42. Emdin, CA, Khera, AV, and Kathiresan, S. Mendelian randomization. JAMA. (2017) 318:1925–6. doi: 10.1001/jama.2017.17219
43. Skrivankova, VW, Richmond, RC, Woolf, BAR, Yarmolinsky, J, Davies, NM, Swanson, SA, et al. Strengthening the reporting of observational studies in epidemiology using Mendelian randomization: the strobe-MR Statement. JAMA. (2021) 326:1614–21. doi: 10.1001/jama.2021.18236
44. Zhao, JH, Stacey, D, Eriksson, N, Macdonald-Dunlop, E, Hedman, AK, Kalnapenkis, A, et al. Genetics of circulating inflammatory proteins identifies drivers of immune-mediated disease risk and therapeutic targets. Nat Immunol. (2023) 24:1540–51. doi: 10.1038/s41590-023-01588-w
45. Kurki, MI, Karjalainen, J, Palta, P, Sipila, TP, Kristiansson, K, Donner, KM, et al. Finngen provides genetic insights from a well-phenotyped isolated population. Nature. (2023) 613:508–18. doi: 10.1038/s41586-022-05473-8
46. Burgess, S, Scott, RA, Timpson, NJ, Davey Smith, G, Thompson, SG, and Consortium, E-I. Using published data in Mendelian randomization: a blueprint for efficient identification of causal risk factors. Eur J Epidemiol. (2015) 30:543–52. doi: 10.1007/s10654-015-0011-z
47. Davies, NM, Holmes, MV, and Davey, SG. Reading Mendelian randomisation studies: a guide, glossary, and checklist for clinicians. BMJ. (2018) 362:k601. doi: 10.1136/bmj.k601
48. He, M, Xu, C, Yang, R, Liu, L, Zhou, D, and Yan, S. Causal relationship between human blood metabolites and risk of ischemic stroke: a Mendelian randomization study. Front Genet. (2024) 15:1333454. doi: 10.3389/fgene.2024.1333454
49. Yuan, J, Xiong, X, Zhang, B, Feng, Q, Zhang, J, Wang, W, et al. Genetically predicted C-reactive protein mediates the association between rheumatoid arthritis and atlantoaxial subluxation. Front Endocrinol (Lausanne). (2022) 13:1054206. doi: 10.3389/fendo.2022.1054206
50. Choi, KW, Chen, CY, Stein, MB, Klimentidis, YC, Wang, MJ, Koenen, KC, et al. Assessment of bidirectional relationships between physical activity and depression among adults: a 2-sample mendelian randomization study. JAMA Psychiatry. (2019) 76:399–408. doi: 10.1001/jamapsychiatry.2018.4175
51. Cheng, ZX, Hua, JL, Jie, ZJ, Li, XJ, and Zhang, J. Genetic insights into the gut-lung axis: mendelian randomization analysis on gut microbiota, lung function, and COPD. Int J Chron Obstruct Pulmon Dis. (2024) 19:643–53. doi: 10.2147/COPD.S441242
52. Pierce, BL, and Burgess, S. Efficient design for Mendelian randomization studies: subsample and 2-sample instrumental variable estimators. Am J Epidemiol. (2013) 178:1177–84. doi: 10.1093/aje/kwt084
53. Sanderson, E. Multivariable Mendelian randomization and mediation. Cold Spring Harb Perspect Med. (2021) 11:a038984. doi: 10.1101/cshperspect.a038984
54. Burgess, S, and Thompson, SG. Erratum to: interpreting findings from MENDELIAN randomization using the MR-Egger method. Eur J Epidemiol. (2017) 32:391–2. doi: 10.1007/s10654-017-0276-5
55. Burgess, S, Bowden, J, Fall, T, Ingelsson, E, and Thompson, SG. Sensitivity analyses for robust causal inference from Mendelian randomization analyses with multiple genetic variants. Epidemiology. (2017) 28:30–42. doi: 10.1097/EDE.0000000000000559
56. Bowden, J, Davey Smith, G, and Burgess, S. Mendelian randomization with invalid instruments: effect estimation and bias detection through Egger regression. Int J Epidemiol. (2015) 44:512–25. doi: 10.1093/ije/dyv080
57. Verbanck, M, Chen, CY, Neale, B, and Do, R. Detection of widespread horizontal pleiotropy in causal relationships inferred from Mendelian randomization between complex traits and diseases. Nat Genet. (2018) 50:693–8. doi: 10.1038/s41588-018-0099-7
58. Cai, J, Li, X, Wu, S, Tian, Y, Zhang, Y, Wei, Z, et al. Assessing the causal association between human blood metabolites and the risk of epilepsy. J Transl Med. (2022) 20:437. doi: 10.1186/s12967-022-03648-5
59. Shi, Y, Feng, S, Yan, M, Wei, S, Yang, K, and Feng, Y. inflammatory bowel disease and celiac disease: a bidirectional Mendelian randomization study. Front Genet. (2022) 13:928944. doi: 10.3389/fgene.2022.928944
60. Carter, AR, Sanderson, E, Hammerton, G, Richmond, RC, Davey Smith, G, Heron, J, et al. Mendelian randomisation for mediation analysis: current methods and challenges for implementation. Eur J Epidemiol. (2021) 36:465–78. doi: 10.1007/s10654-021-00757-1
61. Rondanelli, M, Faliva, MA, Peroni, G, Infantino, V, Gasparri, C, Iannello, G, et al. Food pyramid for subjects with chronic obstructive pulmonary diseases. Int J Chron Obstruct Pulmon Dis. (2020) 15:1435–48. doi: 10.2147/COPD.S240561
62. Zinellu, A, and Mangoni, AA. Arginine, transsulfuration, and folic acid pathway metabolomics in chronic obstructive pulmonary disease: a systematic review and meta-analysis. Cells. (2023) 12:2180. doi: 10.3390/cells12172180
63. Cui, S, Lv, X, Li, W, Li, Z, Liu, H, Gao, Y, et al. Folic acid modulates VPO 1 DNA methylation levels and alleviates oxidative stress-induced apoptosis in vivo and in vitro. Redox Biol. (2018) 19:81–91. doi: 10.1016/j.redox.2018.08.005
64. Nakano, H, Inoue, S, Minegishi, Y, Igarashi, A, Tokairin, Y, Yamauchi, K, et al. Effect of hyperhomocysteinemia on a murine model of smoke-induced pulmonary emphysema. Sci Rep. (2022) 12:12968. doi: 10.1038/s41598-022-16767-2
65. Kim, T, Oak, CH, Jung, MH, Jang, TW, and Kim, J. High serum folate concentration is associated with better lung function in male chronic obstructive pulmonary disease patients who are current smokers: analysis of nationwide population-based survey. Nutrients. (2020) 12:2219. doi: 10.3390/nu12082219
66. Leng, S, Picchi, MA, Tesfaigzi, Y, Wu, G, Gauderman, WJ, Xu, F, et al. Dietary nutrients associated with preservation of lung function in Hispanic and non-Hispanic white smokers from New Mexico. Int J Chron Obstruct Pulmon Dis. (2017) 12:3171–81. doi: 10.2147/COPD.S142237
67. Khan, NA, Saini, H, Mawari, G, Kumar, S, Hira, HS, and Daga, MK. The effect of folic acid supplementation on hyperhomocysteinemia and pulmonary function parameters in chronic obstructive pulmonary disease: a pilot study. J Clin Diagn Res. (2016) 10:OC17-OC21. doi: 10.7860/JCDR/2016/21322.8927
68. Jung, YJ, Lee, SH, Chang, JH, Lee, HS, Kang, EH, and Lee, SW. The impact of changes in the intake of fiber and antioxidants on the development of chronic obstructive pulmonary disease. Nutrients. (2021) 13:580. doi: 10.3390/nu13020580
69. Hirayama, F, Lee, AH, Terasawa, K, and Kagawa, Y. Folate intake associated with lung function, breathlessness and the prevalence of chronic obstructive pulmonary disease. Asia Pac J Clin Nutr. (2010) 19:103–9.
70. Bai, J, Tang, L, Luo, Y, Han, Z, Li, C, Sun, Y, et al. Vitamin B complex blocks the dust fall Pm(2) (.5) -induced acute lung injury through DNA methylation in rats. Environ Toxicol. (2023) 38:403–14. doi: 10.1002/tox.23689
71. Chang, SW, Kim, MB, and Kang, JW. High serum folate level is positively associated with pulmonary function in elderly Korean men, but not in women. Sci Rep. (2022) 12:4523. doi: 10.1038/s41598-022-08234-9
72. Papamichael, MM, Katsardis, C, Tsoukalas, D, Lambert, K, Erbas, B, and Itsiopoulos, C. Potential role of folate status on pulmonary function in pediatric asthma. Nutrition. (2021) 90:111267. doi: 10.1016/j.nut.2021.111267
73. Janssens, W, Decramer, M, Mathieu, C, and Korf, H. Vitamin D and chronic obstructive pulmonary disease: hype or reality? Lancet Respir Med. (2013) 1:804–12. doi: 10.1016/S2213-2600(13)70102-4
74. Hiemstra, PS, and de Jongh, RT. Vitamin D deficiency in asthma and chronic obstructive pulmonary disease. a chicken-or-egg story. Am J Respir Crit Care Med. (2020) 202:312–3. doi: 10.1164/rccm.202004-1012ED
75. Brenner, H, Holleczek, B, and Schottker, B. Vitamin D insufficiency and deficiency and mortality from respiratory diseases in a cohort of older adults: potential for limiting the death toll during and beyond the COVID-19 pandemic? Nutrients. (2020) 12:2488. doi: 10.3390/nu12082488
76. Afzal, S, Lange, P, Bojesen, SE, Freiberg, JJ, and Nordestgaard, BG. Plasma 25-hydroxyvitamin D, lung function and risk of chronic obstructive pulmonary disease. Thorax. (2014) 69:24–31. doi: 10.1136/thoraxjnl-2013-203682
77. Anitua, E, Tierno, R, and Alkhraisat, MH. Current opinion on the role of vitamin d supplementation in respiratory infections and asthma/COPD exacerbations: a need to establish publication guidelines for overcoming the unpublished data. Clin Nutr. (2022) 41:755–77. doi: 10.1016/j.clnu.2022.01.029
78. Jolliffe, DA, Stefanidis, C, Wang, Z, Kermani, NZ, Dimitrov, V, White, JH, et al. Vitamin D metabolism is dysregulated in asthma and chronic obstructive pulmonary disease. Am J Respir Crit Care Med. (2020) 202:371–82. doi: 10.1164/rccm.201909-1867OC
79. Molmen, KS, Hammarstrom, D, Pedersen, K, Lian Lie, AC, Steile, RB, Nygaard, H, et al. Vitamin D (3) supplementation does not enhance the effects of resistance training in older adults. J Cachexia Sarcopenia Muscle. (2021) 12:599–628. doi: 10.1002/jcsm.12688
80. Rafiq, R, Aleva, FE, Schrumpf, JA, Daniels, JM, Bet, PM, Boersma, WG, et al. Vitamin D supplementation in chronic obstructive pulmonary disease patients with low serum vitamin D: a randomized controlled trial. Am J Clin Nutr. (2022) 116:491–9. doi: 10.1093/ajcn/nqac083
81. Fu, L, Fei, J, Tan, ZX, Chen, YH, Hu, B, Xiang, HX, et al. Low vitamin D status is associated with inflammation in patients with chronic obstructive pulmonary disease. J Immunol. (2021) 206:515–23. doi: 10.4049/jimmunol.2000964
82. Sundar, IK, Hwang, JW, Wu, S, Sun, J, and Rahman, I. Deletion of vitamin D receptor leads to premature emphysema/COPD by increased matrix metalloproteinases and lymphoid aggregates formation. Biochem Biophys Res Commun. (2011) 406:127–33. doi: 10.1016/j.bbrc.2011.02.011
83. Hu, G, Dong, T, Wang, S, Jing, H, and Chen, J. Vitamin D (3)-vitamin D receptor axis suppresses pulmonary emphysema by maintaining alveolar macrophage homeostasis and function. EBioMedicine. (2019) 45:563–77. doi: 10.1016/j.ebiom.2019.06.039
84. Jolliffe, DA, Greenberg, L, Hooper, RL, Mathyssen, C, Rafiq, R, de Jongh, RT, et al. Vitamin D to prevent exacerbations of COPD: systematic review and meta-analysis of individual participant data from randomised controlled trials. Thorax. (2019) 74:337–45. doi: 10.1136/thoraxjnl-2018-212092
85. Paulin, FV, Goelzer, LS, and Muller, PT. Vitamin B(12) supplementation and Nt-Probnp levels in COPD patients: a secondary analysis of a randomized and controlled study in rehabilitation. Front Neurosci. (2020) 14:740. doi: 10.3389/fnins.2020.00740
86. Paulin, FV, Zagatto, AM, Chiappa, GR, and Muller, PT. Addition of vitamin B12 to exercise training improves cycle ergometer endurance in advanced COPD patients: a randomized and controlled study. Respir Med. (2017) 122:23–9. doi: 10.1016/j.rmed.2016.11.015
87. Cheng, X, Hu, Y, Ruan, Z, Zang, G, Chen, X, and Qiu, Z. Association between B-vitamins intake and frailty among patients with chronic obstructive pulmonary disease. Aging Clin Exp Res. (2023) 35:793–801. doi: 10.1007/s40520-023-02353-7
88. Ali, MK, Kim, RY, Brown, AC, Mayall, JR, Karim, R, Pinkerton, JW, et al. Crucial role for lung iron level and regulation in the pathogenesis and severity of asthma. Eur Respir J. (2020) 55:1901340. doi: 10.1183/13993003.01340-2019
89. Kang, H, Huang, D, Zhang, W, Wang, J, Liu, Z, Wang, Z, et al. Inhaled polystyrene microplastics impaired lung function through pulmonary flora/Tlr 4-mediated iron homeostasis imbalance. Sci Total Environ. (2024) 946:174300. doi: 10.1016/j.scitotenv.2024.174300
90. Xia, H, Wu, Y, Zhao, J, Cheng, C, Lin, J, Yang, Y, et al. N6-Methyladenosine-Modified Circsav 1 triggers ferroptosis in Copd through recruiting Ythdf1 to facilitate the translation of Ireb2. Cell Death Differ. (2023) 30:1293–304. doi: 10.1038/s41418-023-01138-9
91. Galli, TT, de Campos, EC, do Nascimento Camargo, L, Fukuzaki, S, Dos Santos, TM, SSS, H, et al. Effects of environmental exposure to iron powder on healthy and elastase-exposed mice. Sci Rep. (2024) 14:9134. doi: 10.1038/s41598-024-59573-8
92. Xu, M, Zhang, D, and Yan, J. Targeting ferroptosis using Chinese herbal compounds to treat respiratory diseases. Phytomedicine. (2024) 130:155738. doi: 10.1016/j.phymed.2024.155738
93. Liu, L, Zhang, Y, Wang, L, Liu, Y, Chen, H, Hu, Q, et al. Scutellarein alleviates chronic obstructive pulmonary disease through inhibition of ferroptosis by chelating iron and interacting with arachidonate 15-lipoxygenase. Phytother Res. (2023) 37:4587–606. doi: 10.1002/ptr.7928
94. Cloonan, SM, Mumby, S, Adcock, IM, Choi, AMK, Chung, KF, and Quinlan, GJ. The "Iron"-Y of iron overload and iron deficiency in chronic obstructive pulmonary disease. Am J Respir Crit Care Med. (2017) 196:1103–12. doi: 10.1164/rccm.201702-0311PP
95. Mariani, TJ. Respiratory disorders: ironing out smoking-related airway disease. Nature. (2016) 531:586–7. doi: 10.1038/nature17309
96. Perez-Peiro, M, Martin-Ontiyuelo, C, Rodo-Pi, A, Piccari, L, Admetllo, M, Duran, X, et al. Iron replacement and redox balance in non-anemic and mildly anemic iron deficiency COPD patients: insights from a clinical trial. Biomedicines. (2021) 9:1191. doi: 10.3390/biomedicines9091191
97. Verhamme, FM, De Smet, EG, Van Hooste, W, Delanghe, J, Verleden, SE, Joos, GF, et al. Bone morphogenetic protein 6 (BMP-6) modulates lung function, pulmonary iron levels and cigarette smoke-induced inflammation. Mucosal Immunol. (2019) 12:340–51. doi: 10.1038/s41385-018-0116-2
98. Dziegala, M, Josiak, K, Kasztura, M, Kobak, K, von Haehling, S, Banasiak, W, et al. Iron deficiency as energetic insult to skeletal muscle in chronic diseases. J Cachexia Sarcopenia Muscle. (2018) 9:802–15. doi: 10.1002/jcsm.12314
99. Nickol, AH, Frise, MC, Cheng, HY, McGahey, A, McFadyen, BM, Harris-Wright, T, et al. A cross-sectional study of the prevalence and associations of iron deficiency in a cohort of patients with chronic obstructive pulmonary disease. BMJ Open. (2015) 5:e007911. doi: 10.1136/bmjopen-2015-007911
100. Martin-Ontiyuelo, C, Rodo-Pin, A, Echeverria-Esnal, D, Admetllo, M, Duran-Jorda, X, Alvarado, M, et al. Intravenous iron replacement improves exercise tolerance in COPD: a single-blind randomized trial. Arch Bronconeumol. (2022) 58:689–98. doi: 10.1016/j.arbres.2021.08.011
101. Grasmuk-Siegl, E, Urban, MH, Scherrer, S, and Funk, GC. Effect of intravenous ferric carboxymaltose on exercise capacity and quality of life in patients with COPD: a pilot study. Wien Klin Wochenschr. (2023) 135:35–44. doi: 10.1007/s00508-022-02073-4
102. Barberan-Garcia, A, Rodriguez, DA, Blanco, I, Gea, J, Torralba, Y, Arbillaga-Etxarri, A, et al. Non-anaemic iron deficiency impairs response to pulmonary rehabilitation in COPD. Respirology. (2015) 20:1089–95. doi: 10.1111/resp.12591
103. Tatah, J, Keen, JL, Prisco, SZ, Pritzker, M, Thenappan, T, and Prins, KW. Iron deficiency is associated with more severe pulmonary vascular disease in pulmonary hypertension caused by chronic lung disease. Chest. (2022) 161:232–6. doi: 10.1016/j.chest.2021.07.2159
104. Faherty, L, Kenny, S, and Cloonan, SM. Iron and mitochondria in the susceptibility, pathogenesis and progression of COPD. Clin Sci (Lond). (2023) 137:219–37. doi: 10.1042/CS20210504
105. Yoshida, M, Minagawa, S, Araya, J, Sakamoto, T, Hara, H, Tsubouchi, K, et al. Involvement of cigarette smoke-induced epithelial cell ferroptosis in COPD pathogenesis. Nat Commun. (2019) 10:3145. doi: 10.1038/s41467-019-10991-7
106. Cloonan, SM, Glass, K, Laucho-Contreras, ME, Bhashyam, AR, Cervo, M, Pabon, MA, et al. Mitochondrial iron chelation ameliorates cigarette smoke-induced bronchitis and emphysema in mice. Nat Med. (2016) 22:163–74. doi: 10.1038/nm.4021
107. Wang, G, Hallberg, J, Faner, R, Koefoed, HJ, Kebede Merid, S, Klevebro, S, et al. Plasticity of individual lung function states from childhood to adulthood. Am J Respir Crit Care Med. (2023) 207:406–15. doi: 10.1164/rccm.202203-0444OC
108. Hu, L, Yamamoto, M, Chen, J, Duan, H, Du, J, He, L, et al. Integrating network pharmacology and experimental verification to decipher the immunomodulatory effect of Bu-Zhong-Yi-Qi-Tang against Poly (I: C)-induced pulmonary inflammation. Front Pharmacol. (2022) 13:1015486. doi: 10.3389/fphar.2022.1015486
109. Li, Q, Sun, J, Cao, Y, Liu, B, Zhao, Z, Hu, L, et al. Icaritin inhibited cigarette smoke extract-induced Cd8(+) T cell chemotaxis enhancement by targeting the Cxcl 10/Cxcr3 axis and Tgf-Beta/Smad 2 signaling. Phytomedicine. (2022) 96:153907. doi: 10.1016/j.phymed.2021.153907
110. Lu, T, Lahousse, L, Wijnant, S, Chen, J, Brusselle, GG, van Hoek, M, et al. The age-rage axis associates with chronic pulmonary diseases and smoking in the rotterdam study. Respir Res. (2024) 25:85. doi: 10.1186/s12931-024-02698-1
111. Ruth, JH, Gurrea-Rubio, M, Athukorala, KS, Rasmussen, SM, Weber, DP, Randon, PM, et al. Cd6 is a target for cancer immunotherapy. JCI Insight. (2021) 6:e145662. doi: 10.1172/jci.insight.145662
112. Sun, D, Lin, R, and Ouyang, Y. The role of Cd40, Cd86, and glutathione S-transferase omega 1 in the pathogenesis of chronic obstructive pulmonary disease. Can Respir J. (2022) 2022:6810745. doi: 10.1155/2022/6810745
113. Bahudhanapati, H, Tan, J, Apel, RM, Seeliger, B, Schupp, J, Li, X, et al. Increased Expression of Cxcl 6 in secretory cells drives fibroblast collagen synthesis and is associated with increased mortality in idiopathic pulmonary fibrosis. Eur Respir J. (2024) 63:2300088. doi: 10.1183/13993003.00088-2023
114. von Itzstein, MS, Gerber, DE, and Minna, JD. Contemporary lung cancer screening and the promise of blood-based biomarkers. Cancer Res. (2021) 81:3441–3. doi: 10.1158/0008-5472.CAN-21-0706
Keywords: trace elements, inflammatory proteins, COPD, Mendelian randomization, mediation analysis
Citation: Cao Z, Zhao S, Wu T, Sun F, Ding H, Hu S and Shi L (2024) Genetic information supports a causal relationship between trace elements, inflammatory proteins, and COPD: evidence from a Mendelian randomization analysis. Front. Nutr. 11:1430606. doi: 10.3389/fnut.2024.1430606
Edited by:
Sladjana Sobajic, University of Belgrade, SerbiaReviewed by:
Wencai Liu, Shanghai Jiao Tong University, ChinaShuaiwei Song, People’s Hospital of Xinjiang Uygur Autonomous Region, China
Copyright © 2024 Cao, Zhao, Wu, Sun, Ding, Hu and Shi. This is an open-access article distributed under the terms of the Creative Commons Attribution License (CC BY). The use, distribution or reproduction in other forums is permitted, provided the original author(s) and the copyright owner(s) are credited and that the original publication in this journal is cited, in accordance with accepted academic practice. No use, distribution or reproduction is permitted which does not comply with these terms.
*Correspondence: Shaodan Hu, NzM0MTY4MzAwQHFxLmNvbQ==; Li Shi, c2hpbGkwNjQ4QDE2My5jb20=