- 1Graduate School, Beijing University of Chinese Medicine, Beijing, China
- 2Xiyuan Hospital, China Academy of Chinese Medical Sciences, Beijing, China
Background: Minerals play an important role in human health, but their effect on urinary function remains controversial. The aim of this study was to assess the association between dietary intake of minerals (Ca, P, Mg, Fe, Zn, Cu, Na, K, Se) and urine flow rate (UFR).
Methods: We conducted a cross-sectional study using the National Health and Nutrition Examination Survey (NHANES, 2009–2018) database. Multivariate regression and smooth curve fitting were used to investigate the association between dietary mineral intakes and UFR. Subgroup analyses and interaction tests were used to investigate whether this association was stable in the population.
Results: Our study involving 10,229 representative adult NHANES participants showed an association between Mg intake and UFR in a linear regression model for continuous variables. And in the model analysis of tertile categorical variables, we observed a positive association between six mineral intakes (Ca, Mg, Zn, Cu, Na, and K) and UFR. Smoothed curve fitting and threshold effect analysis further support the nonlinear relationship between mineral intakes and UFR. Subgroup analyses and interaction tests ensured the reliability and robustness of the findings.
Conclusion: This study examined the effects of nine dietary minerals on UFR and found that intake of Ca, Mg, Zn, Cu, Na, and K were positively correlated with UFR, suggesting that these minerals may have a positive effect on improving urinary function. In particular, Mg showed a more significant positive correlation with UFR in women, while Na showed a stronger positive correlation in diabetics. However, P, Fe and Se did not show significant correlations. In summary, although these findings provide a preliminary understanding of the relationship between dietary minerals and urinary function, further prospective studies are still necessary to validate these relationships and explore the physiologic mechanisms underlying them.
1 Introduction
Voiding dysfunction is a dysfunction of the bladder, urethra, and their associated nervous and muscular systems that results in an abnormality in the normal process of urination, and is the most common symptom seen in urologic clinics, especially in the elderly (1, 2). It is estimated that by 2025, more than 42 million people in the United States will experience urinary dysfunction (3). Difficulty in voiding significantly reduces the quality of life for patients and leads to serious economic and public health problems (4, 5). Urine flow rate (UFR) is a measurement of the amount of urine produced over a specific period of time and can provide some assessment of voiding function (6, 7). A daily urine output of less than 400 mL is referred to as oliguria and is the minimum amount of urine required to eliminate metabolic waste (8). Healthy eating patterns play an important role in the prevention and treatment of many chronic diseases (9–11), although dietary minerals do not make up a large proportion of total dietary intake, they play a very important role in health (12). Several studies have reported that inadequate mineral intake is associated with blood pressure and abnormalities in lipid metabolism and glucose metabolism (13–15). Many studies have reported dietary associations with voiding function (16–18), but only few have examined the correlation between dietary minerals and voiding function (19). Therefore, the aim of this study was to investigate the relationship between dietary intake of nine common minerals [calcium (Ca), phosphorus (P), magnesium (Mg), iron (Fe), zinc (Zn), copper (Cu), sodium (Na), potassium (K) and selenium (Se)] and UFR. We hope that this study will provide some information for risk management and preventive interventions for patients with urinary dysfunction.
2 Methods
2.1 Study design and participants
NHANES data from five cycles (2009–2010, 2011–2012, 2013–2014, 2015–2016, and 2017–2018) were used in this study. Figure 1 shows a flowchart of the participant recruitment process. There were a total of 49,693 participants over the five cycles, and we first excluded 14,274 participants with missing UFR, then 6,805 with missing dietary mineral intake, 16,728 with missing covariates data, finally 1,657 participants with failing kidneys, kidney stones, and average energy intake below 600 kcal/day or above 4,000 kcal/day were excluded. Ultimately, a total of 10,229 participants were included in this study. The NHANES study was conducted with the approval of the Ethics Review Committee of the National Center for Health Statistics, and it met the ethical standards of the 1964 Declaration of Helsinki and its subsequent amendments, with written informed consent signed by all participants.
2.2 Measurement of UFR
Participants will be asked to record the time of their last urination prior to coming to the mobile examination center (MEC), and then, record the time and volume of urine samples collected while the participant is at the MEC and calculate the UFR based on the volume of the urine samples collected as well as the duration of time that elapsed between urinations. To ensure sufficient urine volume for analysis, each participant was allowed to provide up to three urine samples. The formula for calculating UFR was: UFR = (total urine volume)/(total time duration). Detailed instructions for urine sample collection and processing can be found in the NHANES Laboratory Procedures Manual (LPM).
2.3 Intake of dietary minerals
The NHANES dietary intake data are the types and amounts of food and beverages (including all types of water) consumed by participants in the 24 h prior to the interview (midnight to midnight), and estimates of energy, nutrient, and other food components consumed from these foods and beverages. All participants underwent two 24-h dietary recall interviews, the first dietary recall interview was collected in person at the MEC, and the second interview was collected by telephone 3 to 10 days later. Dietary mineral intakes were derived by calculating the average of the two interview data.
2.4 Covariates
A number of covariates were included in this study, including age (years), gender (male/female), race (Mexican American, non-Hispanic Black, non-Hispanic White and other race), education level (lower than high school, high school or equivalent and college or above), income-to-poverty ratio (PIR), body mass index (BMI), serum creatinine (SCr), energy intake, protein intake, carbohydrate intake, total sugars intake, total fat intake, smokers (yes/no), alcohol drinkers (yes/no), hypertension (yes/no), diabetes (yes/no), history of cardiovascular diseases (CVD). BMI was calculated by dividing weight (in kilograms) by the square of height (in meters) and was divided into 4 categories: underweight (< 18.50 kg/m2), normal weight (18.5 kg/m2 ≤ BMI < 25.0 kg/m2), overweight (25 kg/m2 ≤ BMI < 30.00 kg/m2) and obese (≥ 30.0 kg/m2). History of CVD included congestive heart failure, coronary heart disease, angina pectoris, heart attack, and stroke.
2.5 Statistical analysis
Participant demographic data were assessed through chi-square and t-tests for gender categorization. Weighted multiple regression analyses were used to test the linear relationship between dietary mineral intakes and UFR. After transforming dietary mineral intakes from continuous variables to categorical variables (tertiles), a trend test was used to examine the trend of linear association between dietary mineral intakes and UFR. To explore the relationship between dietary mineral intakes and UFR, subgroup analyses were conducted across variables such as gender, BMI, hypertension, and diabetes to assess the consistency of these associations. Additionally, interaction tests were utilized to further examine the uniformity of these relationships across the different subgroups. Nonlinear association between dietary mineral intakes and UFR were investigated using smoothed curve fitting. All statistical analyses were conducted using R (version 4.3.0) and EmpowerStats (version 5.0), with a significance threshold set at a two-sided p-value of less than 0.05.
3 Results
3.1 Baseline characteristics
A total of 10,229 individuals with complete survey data were enrolled in this study, including 5,075 males and 5,154 females, and the mean age of the subjects was 46.80 ± 16.95 years, Table 1 shows the baseline characteristics of the subjects. The following variables showed statistically significant differences between men and women: age, race, education, BMI, SCr, energy intake, protein intake, carbohydrate intake, total sugars intake, total fat intake, smokers, alcohol drinkers, hypertension, diabetes, history of CVD, dietary mineral intakes (Ca, P, Mg, Fe, Zn, Cu, Na, K, Se), and UFR (all p < 0.05).
3.2 Association between dietary mineral intakes and UFR
Table 2 demonstrates the results of weighted generalized linear regression models based on different adjusted covariates with transformation treatment of dietary mineral intake from continuous to tertile categorical variables to assess the association between dietary mineral intake and UFR. In the linear regression models for continuous variables, both the unadjusted and partially adjusted models (Model I) showed a strong positive association between total dietary mineral intake and UFR at a significance level (all p < 0.0001). However, in the fully adjusted model (Model II), only Mg remained significant (p < 0.0001), while the p-values for the rest of the dietary minerals exceeded 0.05 and did not show statistical significance.
In the model analysis of tertile categorical variables, the unadjusted and partially adjusted models also showed significance levels of p < 0.05 for all dietary mineral intakes. In the fully adjusted model (Model II), the significance of Mg remained unchanged (p < 0.001), and the Ca, Zn, Cu, Na, K in the tertile categories showed statistical significance as well (p < 0.05). The results show that there is a significant linear relationship between the Ca, Mg, Zn, Cu, Na, K tertiles and UFR (all P for trend <0.05). In the fully adjusted model (Model II), the β-values for the highest tertile (Tertile 3) compared to the lowest tertile (Tertile 1) were determined as Ca [15.45 (9.04, 21.85)], Mg [16.97 (10.59, 23.35)], Zn [7.27 (1.24, 13.30)], Cu [18.50 (12.94, 24.07)], Na [8.35 (1.26, 15.44)], K [15.78 (9.32, 22.23)].
3.3 Smooth curve fitting
Through smoothing curve fitting, we found a nonlinear relationship between mineral intake (Ca, Mg, Zn, Cu, Na, K) and UFR (Figure 2) and further analyzed the nonlinear relationship for threshold effects (Table 3). The results of the analysis showed that when the mineral intake (Ca, Mg, Zn, Cu, Na, K) was below the inflection point, they were all significantly and positively correlated with UFR (all p < 0.05). However, when dietary Mg intake exceeded 353.5 mg/day, or dietary Zn intake exceeded 9.62 mg/day, or dietary Cu intake exceeded 1.35 mg/day, or dietary Na intake exceeded 3,005 mg/day, or dietary K intake exceeded 2979.5 mg/day, none of the effects were significant (all p > 0.05). In addition, Ca had a significant effect below the inflection point [0.01 (0.00, 0.02)] and a significant effect above the inflection point [−0.01 (−0.02, −0.00)] in opposite directions (all p < 0.05). The p-values for LRT were all less than 0.05, indicating that all models showed significant improvement over the simple linear model, supporting the nonlinear relationship between UFR and mineral intake (Ca, Mg, Zn, Cu, Na, K).
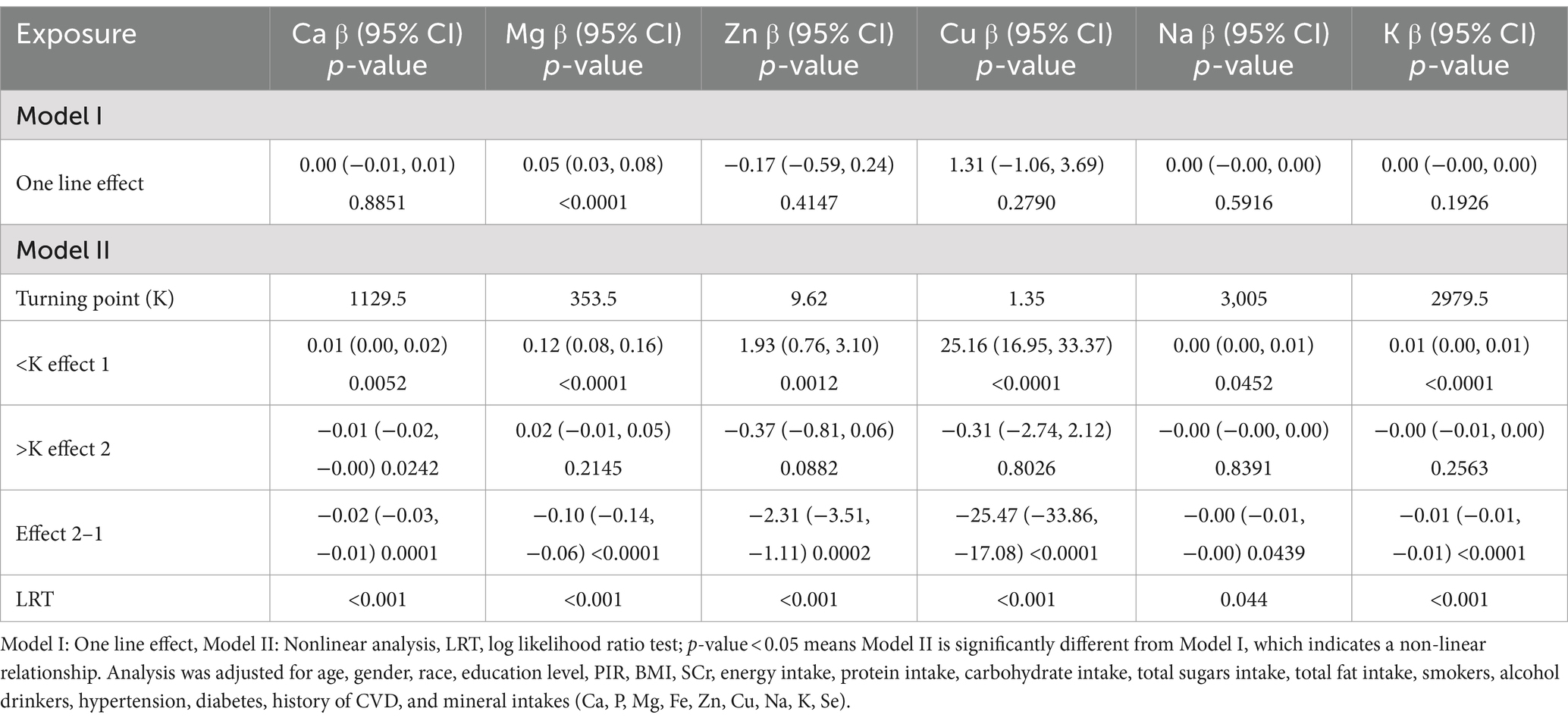
Table 3. Threshold effect analysis for the relationship between dietary mineral intakes (Ca, Mg, Zn, Cu, Na, K) and UFR using piece-wise linear regression.
3.4 Subgroup analyses
We performed subgroup analyses and interaction tests stratified by sex, BMI, hypertension, and diabetes to assess whether associations between mineral intake (Ca, Mg, Zn, Cu, Na, K) and UFR were consistent across the general population and to identify any potentially different population settings (Table 4). The results showed a significant moderating effect of gender on the effect of Mg (P for interaction <0.05), which was significantly higher for female [0.09 (0.05, 0.13)] than for male [0.03 (−0.00, 0.07)]. For diabetic patients, Na [0.01 (0.00, 0.02)] showed a significant positive effect, whereas in nondiabetic patients, the effect was not significant, indicating a significant interaction of diabetic status on the effect of Na (P for interaction <0.05). In the BMI and hypertension subgroups, the effects of the exposures differed but the interaction did not reach the level of statistical significance (all p > 0.05).
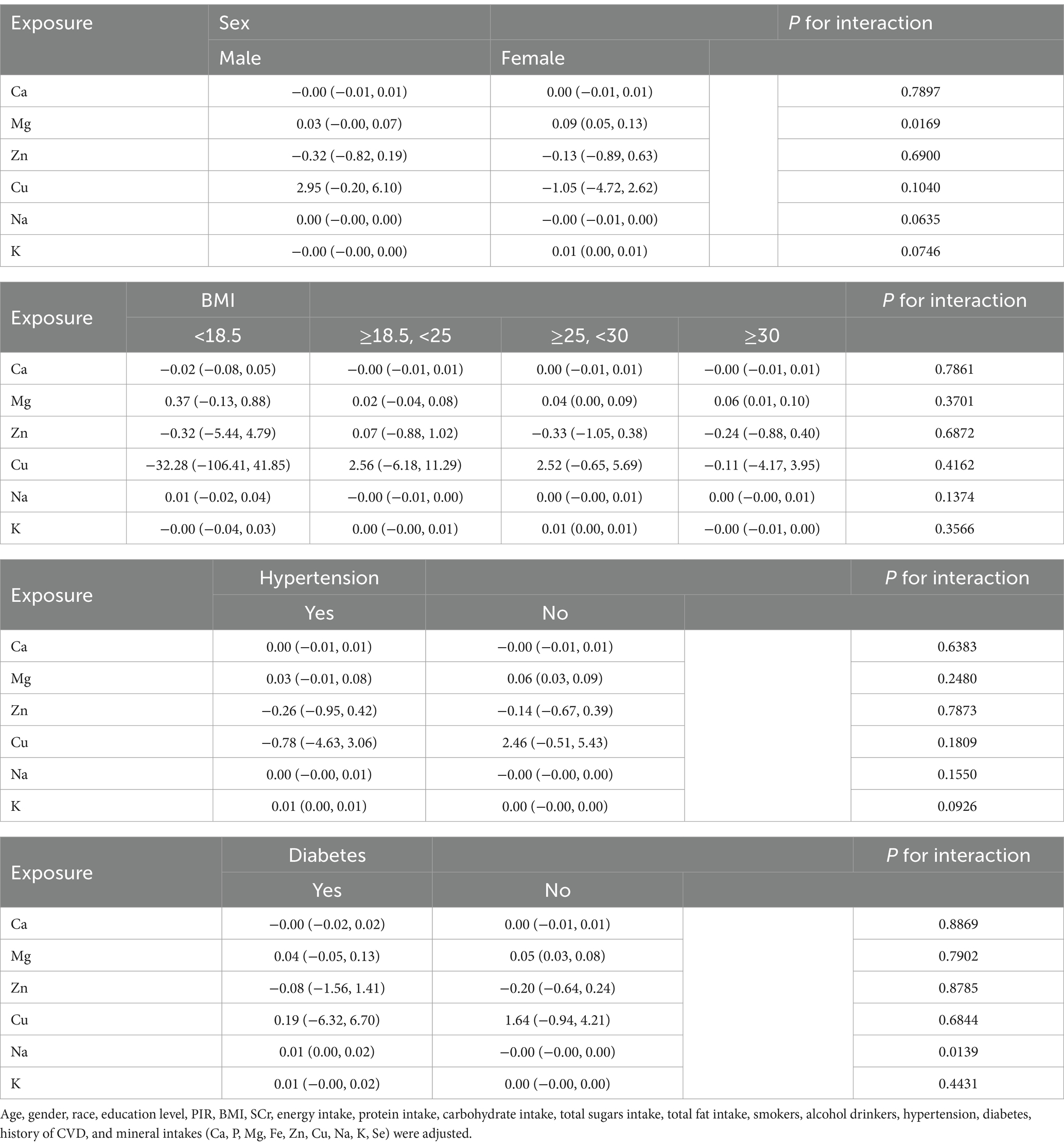
Table 4. Subgroup analysis of the association between dietary mineral intakes (Ca, Mg, Zn, Cu, Na, K) and UFR.
4 Discussion
Our study involving 10,229 representative adult NHANES participants shows an association between Mg intake and UFR in a linear regression model for continuous variables. And in the model analysis of tertile categorical variables, we observed a positive association between six mineral intakes (Ca, Mg, Zn, Cu, Na, and K) and UFR. Smoothed curve fitting and threshold effect analysis further support the nonlinear relationship between mineral intake and UFR. Subgroup analyses and interaction tests ensured the reliability and robustness of the findings.
The voiding reflex is influenced by nerve conduction, detrusor function, and bladder outlet (20). Three groups of peripheral nerves innervate the lower urinary tract: the parasympathetic, sympathetic and somatic nervous systems (21). Regarding muscle control, the muscles that primarily affect urination are the detrusor and the pelvic floor muscle groups, which are smooth and skeletal muscles, respectively (20). In terms of bladder outlet, benign prostatic hyperplasia (BPH) is the most common causative factor for bladder outlet obstruction in men (22). Causes of bladder outlet obstruction in women include pelvic organ prolapse, urethral fibrosis or stricture, and detrusor external sphincter dyssynergia (23); pathogenesis includes increased autonomic nervous system activity, detrusor sensitivity, endothelial dysfunction, chronic inflammation and oxidative damage (24).
Ca is the most abundant mineral in the body and needs to be obtained from food. The World Health Organisation recommends a daily Ca intake of 1,000 mg/day for young people (25). The regulation of UFR by Ca is closely linked to the extracellular calcium-sensing receptor (CaSR), which is expressed in human proximal glomerular cells, renal epithelial cells, arterial smooth muscle, and endothelial cells, and can sense and conduct Ca into intracellular signaling pathways (26). CaSR is functionally expressed on all cells of vascular smooth muscle cells and endothelial cells and can regulate changes in vascular tone and arterial blood pressure (27, 28). Activation of the endothelial CaSR allows potassium to be taken up by the myocyte Na/K-ATPase, which helps to reverse vascular myocyte depolarisation and thus vasorelaxation (29). In proximal glomerular cells, dietary Ca uptake mediated through the CaSR reduces the activity of the renin-angiotensin II-aldosterone system (30). In renal epithelial cells, signaling from the basolateral CaSR in the TAL induces natriuresis (31, 32).
Mg is an essential ion for the human body and is a cofactor for many enzymes. We need to consume Mg on a regular basis to maintain normal physiological functions and metabolism in the human body. The United States Food and Nutrition Board recommends a daily intake of 420 mg for men and 320 mg for women (33). Mg can affect urinary function by regulating muscle contraction, vasodilatory tone, muscle and nerve transmission, neuromuscular transmission, and signal transduction (34). Mg decreases small arterial tone and increases the vasodilatory effects of endogenous and exogenous vasodilators, thereby promoting urination. A deficiency in Mg enhances aldosterone synthesis mediated by angiotensin and increases the production of thromboxane and the vasoconstrictor prostaglandin, which leads to reduced urine output (34, 35). Mitochondrial dysfunction also affects bladder smooth muscle contraction, and Mg is required for mitochondria to carry out oxidative phosphorylation (36). Mg can act indirectly as part of the Mg-ATP complex or directly as an enzyme activator (37). Mg is involved in many of the enzyme systems that regulate glucose homeostasis, such as hexokinase and phosphofructokinase. Mg can also influence glucose homeostasis by affecting insulin secretion and cellular uptake of glucose, which can have an effect on UFR (38).
Zn is the second most prevalent trace element in the human body after iron. 85% of Zn is located in muscle and bone (39). Cu is an essential micronutrient for human health and can only be obtained from food (40). Zn is involved in immunity, energy metabolism and antioxidant processes, and is a good antioxidant with protective and therapeutic effects on the central nervous system (41), Zn enhances muscle contraction in vitro (42). A cross-sectional study shows that serum Zn is positively correlated with grip strength (43), while UFR was positively correlated with grip strength (44). Nitric oxide (NO) is thought to be an important neurotransmitter in urethral relaxation and erection and is also involved in the control of bladder afferent nerve activity (21). Zn deficiency disrupts nitric oxide production and increases oxidative stress, leading to endothelial damage (45). A deficiency in dietary Cu significantly attenuates the relaxation and dilation of vascular smooth muscle mediated by nitric oxide (46, 47). Cu deficiency also leads to impaired energy production, abnormal glucose and cholesterol metabolism, and increased oxidative damage (47).
Na is an important part of human nutrition. A study showed that plasma renin activity increased during low Na intake, while brain natriuretic peptide and creatinine clearance decreased, indicating a decrease in intravascular volume (48). Na is involved in maintaining serum electrolyte concentrations, balancing intravascular osmotic pressure, maintaining acid–base balance, and has a role in stimulating muscle contraction (49).
K is an essential nutrient for the maintenance of normal cell function in the body and plays an important role in resting membrane potential and intracellular osmotic pressure (50). K hyperpolarises endothelial cells by stimulating the sodium-potassium pump and activating plasma membrane potassium channels, thereby inducing endothelium-dependent vasodilation (51–53). K can also inhibit the sympathetic nervous system by increasing the uptake of norepinephrine into sympathetic nerve endings, thereby promoting vascular smooth muscle relaxation and increased blood flow (52). Increased K intake also significantly improves endothelial function and increases arterial compliance (54).
Our study found that the effect of dietary magnesium intake on UFR differed significantly between male and female, with female showing higher sensitivity. A clinical trial observed that estrogen levels are significantly higher in female than in male and that estrogen can affect the metabolism of a number of minerals that can enhance magnesium utilization and uptake in soft tissues (55). Differences in habits and basic conditions such as education and marital status between male and female also have an impact (56). In addition, differences in the effect of dietary Mg intake on UFR between men and women may also be related to differences in the structure of the urinary tract in men and women, for example, prostate enlargement in men can cause bladder outlet obstruction, which can affect the UFR (57, 58).
Our study has several advantages over previous studies. First, UFR was used as an outcome variable to assess the effect of dietary mineral intakes on voiding function. Second, we found positive correlations between six dietary mineral intakes (Ca, Mg, Zn, Cu, Na, K) and UFR, providing guidance for preventing and improving urinary function. Finally, this study is based on the NHANES database, which employs a rigorous random sampling process to ensure that our results are sufficiently representative of US adults. However, our study has several limitations. First, due to the cross-sectional analytic design of this study, we were unable to determine a causal relationship between dietary mineral intakes and UFR. Second, despite considering multiple covariates, we were unable to rule out the effects of all potential confounders. Finally, although self-reported dietary intake data are detailed and comprehensive, they may be subject to recall bias and estimation error.
5 Conclusion
This study investigated the association between nine dietary minerals (Ca, P, Mg, Fe, Zn, Cu, Na, K, Se) and UFR. The results showed that the intake of six of these minerals (Ca, Mg, Zn, Cu, Na, K) was positively associated with UFR, suggesting that these minerals may have a positive effect on improving urinary function. In particular, the positive correlation between Mg and UFR was more pronounced in women, while the positive correlation between Na and UFR was more prominent in diabetic patients. Although P, Fe, and Se intake did not show significant correlations in the current study, which may require more research to explore their potential association with urinary function. In summary, although these findings provide a preliminary understanding of the relationship between dietary minerals and urinary function, further prospective studies are still necessary to validate these relationships and explore the physiologic mechanisms underlying them.
Data availability statement
Publicly available datasets were analyzed in this study. This data can be found at: www.cdc.gov/nchs/nhanes/.
Ethics statement
The studies involving humans were approved by National Center for Health Statistics. The studies were conducted in accordance with the local legislation and institutional requirements. The participants provided their written informed consent to participate in this study.
Author contributions
ML: Writing – original draft, Writing – review & editing, Conceptualization, Data curation, Investigation, Software. JZ: Writing – review & editing, Conceptualization, Data curation, Investigation, Software, Writing – original draft. JD: Data curation, Software, Writing – review & editing. ZG: Supervision, Writing – review & editing, Funding acquisition.
Funding
The author(s) declare that financial support was received for the research, authorship, and/or publication of this article. This study was funded by the Project of Xiyuan Hospital, China Academy of Chinese Medical Sciences with surplus funds (Project no. 15-42).
Acknowledgments
We would like to thank all participants in this study.
Conflict of interest
The authors declare that the research was conducted in the absence of any commercial or financial relationships that could be construed as a potential conflict of interest.
Publisher’s note
All claims expressed in this article are solely those of the authors and do not necessarily represent those of their affiliated organizations, or those of the publisher, the editors and the reviewers. Any product that may be evaluated in this article, or claim that may be made by its manufacturer, is not guaranteed or endorsed by the publisher.
Abbreviations
UFR, Urine flow rate; NHANES, National Health and Nutrition Examination Survey; NCHS, National Center for Health Statistics; MEC, Mobile examination center; LPM, Laboratory Procedures Manual; PIR, Income-to-poverty ratio; BMI, Body mass index; SCr, Serum creatinine.
References
1. Ginsberg, DA, Boone, TB, Cameron, AP, Gousse, A, Kaufman, MR, Keays, E, et al. The AUA/SUFU guideline on adult neurogenic lower urinary tract dysfunction: diagnosis and evaluation. J Urol. (2021) 206:1097–105. doi: 10.1097/JU.0000000000002235
2. Gratzke, C, Bachmann, A, Descazeaud, A, Drake, MJ, Madersbacher, S, Mamoulakis, C, et al. EAU guidelines on the assessment of non-neurogenic male lower urinary tract symptoms including benign prostatic obstruction. Eur Urol. (2015) 67:1099–109. doi: 10.1016/j.eururo.2014.12.038
3. Litman, HJ, and McKinlay, JB. The future magnitude of urological symptoms in the USA: projections using the Boston area community health survey [published correction appears in BJU Int. 2007 Oct; 100(4): 971]. BJU Int (2007); 100: 820–825. doi: 10.1111/j.1464-410X.2007.07018.x
4. Kupelian, V, Wei, JT, O'Leary, MP, Kusek, JW, Litman, HJ, Link, CL, et al. Prevalence of lower urinary tract symptoms and effect on quality of life in a racially and ethnically diverse random sample: the Boston area community health (BACH) survey. Arch Intern Med. (2006) 166:2381–7. doi: 10.1001/archinte.166.21.2381
5. Bavendam, TG, Norton, JM, Kirkali, Z, Mullins, C, Kusek, JW, Star, RA, et al. Advancing a comprehensive approach to the study of lower urinary tract symptoms. J Urol. (2016) 196:1342–9. doi: 10.1016/j.juro.2016.05.117
6. Gravas, S, Gacci, M, Gratzke, C, Herrmann, TRW, Karavitakis, M, Kyriazis, I, et al. Summary paper on the 2023 European Association of Urology guidelines on the Management of non-neurogenic Male Lower Urinary Tract Symptoms. Eur Urol. (2023) 84:207–22. doi: 10.1016/j.eururo.2023.04.008
7. Yap, TL, Cromwell, DA, Brown, C, Emberton, M, and van der Meulen, J. The reliability of the frequency-volume chart in assessing lower urinary tract symptoms. BJU Int. (2007) 100:111–5. doi: 10.1111/j.1464-410X.2007.06864.x
8. Bianchi, NA, Altarelli, M, Monard, C, Kelevina, T, Chaouch, A, and Schneider, AG. Identification of an optimal threshold to define oliguria in critically ill patients: an observational study. Crit Care. (2023) 27:207. doi: 10.1186/s13054-023-04505-7
9. Nagle, CM, Ibiebele, T, Shivappa, N, Hébert, JR, DeFazio, A, Webb, PM, et al. The association between the inflammatory potential of diet and risk of developing, and survival following, a diagnosis of ovarian cancer. Eur J Nutr. (2019) 58:1747–56. doi: 10.1007/s00394-018-1779-x
10. Ghanavati, M, Rahmani, J, Clark, CCT, Hosseinabadi, SM, and Rahimlou, M. Pistachios and cardiometabolic risk factors: a systematic review and meta-analysis of randomized controlled clinical trials. Complement Ther Med. (2020) 52:102513. doi: 10.1016/j.ctim.2020.102513
11. Hashemi, R, Mehdizadeh Khalifani, A, Rahimlou, M, and Manafi, M. Comparison of the effect of dietary approaches to stop hypertension diet and American Diabetes Association nutrition guidelines on lipid profiles in patients with type 2 diabetes: a comparative clinical trial. Nutr Diet. (2020) 77:204–11. doi: 10.1111/1747-0080.12543
12. Mohammadifard, N, Gotay, C, Humphries, KH, Ignaszewski, A, Esmaillzadeh, A, and Sarrafzadegan, N. Electrolyte minerals intake and cardiovascular health. Crit Rev Food Sci Nutr. (2019) 59:2375–85. doi: 10.1080/10408398.2018.1453474
13. Mohammadifard, N, Humphries, KH, Gotay, C, Mena-Sánchez, G, Salas-Salvadó, J, Esmaillzadeh, A, et al. Trace minerals intake: risks and benefits for cardiovascular health. Crit Rev Food Sci Nutr. (2019) 59:1334–46. doi: 10.1080/10408398.2017.1406332
14. Kim, MH, and Choi, MK. Seven dietary minerals (ca, P, mg, Fe, Zn, cu, and Mn) and their relationship with blood pressure and blood lipids in healthy adults with self-selected diet. Biol Trace Elem Res. (2013) 153:69–75. doi: 10.1007/s12011-013-9656-1
15. Kheriji, N, Boukhalfa, W, Mahjoub, F, Hechmi, M, Dakhlaoui, T, Mrad, M, et al. The role of dietary intake in type 2 diabetes mellitus: importance of macro and micronutrients in glucose homeostasis. Nutrients. (2022) 14:2132. doi: 10.3390/nu14102132
16. Maserejian, NN, McVary, KT, Giovannucci, EL, and McKinlay, JB. Dietary macronutrient intake and lower urinary tract symptoms in women. Ann Epidemiol. (2011) 21:421–9. doi: 10.1016/j.annepidem.2010.11.014
17. Erickson, BA, Vaughan-Sarrazin, M, Liu, X, Breyer, BN, Kreder, KJ, and Cram, P. Lower urinary tract symptoms and diet quality: findings from the 2000-2001 National Health and nutrition examination survey. Urology. (2012) 79:1262–7. doi: 10.1016/j.urology.2012.03.004
18. Dallosso, HM, CW, MG, Matthews, RJ, and Donaldson, MMLeicestershire MRC Incontinence Study Group. The association of diet and other lifestyle factors with overactive bladder and stress incontinence: a longitudinal study in women. BJU Int. (2003) 92:69–77. doi: 10.1046/j.1464-410x.2003.04271.x
19. Yoshida, M, Fukuwatari, T, Sakai, J, Tsuji, T, and Shibata, K. Correlation between mineral intake and urinary excretion in free-living Japanese Young women. Food Nutr Sci. (2010) 1:38–44. doi: 10.4236/fns.2010.12007
20. Cheng, YW, Hung, CC, Kao, TW, and Chen, WL. Beneficial relevance of vitamin D concentration and urine flow rate. Clin Nutr. (2021) 40:2121–7. doi: 10.1016/j.clnu.2020.09.036
21. Yoshimura, N, and Chancellor, MB. Neurophysiology of lower urinary tract function and dysfunction. Rev Urol. (2003) 5:S3–S10.
22. Fusco, F, Creta, M, De Nunzio, C, Iacovelli, V, Mangiapia, F, Li Marzi, V, et al. Progressive bladder remodeling due to bladder outlet obstruction: a systematic review of morphological and molecular evidences in humans. BMC Urol. (2018) 18:15. doi: 10.1186/s12894-018-0329-4
23. Lin, CD, Kuo, HC, and Yang, SS. Diagnosis and Management of Bladder Outlet Obstruction in women. Low Urin Tract Symptoms. (2016) 8:30–7. doi: 10.1111/luts.12094
24. Maserejian, NN, Giovannucci, EL, McVary, KT, and McKinlay, JB. Intakes of vitamins and minerals in relation to urinary incontinence, voiding, and storage symptoms in women: a cross-sectional analysis from the Boston area community health survey. Eur Urol. (2011) 59:1039–47. doi: 10.1016/j.eururo.2011.03.008
25. Shkembi, B, and Huppertz, T. Calcium absorption from food products: food matrix effects. Nutrients. (2021) 14:180. doi: 10.3390/nu14010180
26. Magno, AL, Ward, BK, and Ratajczak, T. The calcium-sensing receptor: a molecular perspective. Endocr Rev. (2011) 32:3–30. doi: 10.1210/er.2009-0043
27. Molostvov, G, Bland, R, and Zehnder, D. Expression and role of the calcium-sensing receptor in the blood vessel wall. Curr Pharm Biotechnol. (2009) 10:282–8. doi: 10.2174/138920109787847466
28. Molostvov, G, James, S, Fletcher, S, Bennett, J, Lehnert, H, Bland, R, et al. Extracellular calcium-sensing receptor is functionally expressed in human artery. Am J Physiol Renal Physiol. (2007) 293:F946–55. doi: 10.1152/ajprenal.00474.2006
29. Peterlik, M, Kállay, E, and Cross, HS. Calcium nutrition and extracellular calcium sensing: relevance for the pathogenesis of osteoporosis, cancer and cardiovascular diseases. Nutrients. (2013) 5:302–27. doi: 10.3390/nu5010302
30. Atchison, DK, and Beierwaltes, WH. The influence of extracellular and intracellular calcium on the secretion of renin. Pflugers Arch. (2013) 465:59–69. doi: 10.1007/s00424-012-1107-x
31. Wang, D, An, SJ, Wang, WH, McGiff, JC, and Ferreri, NR. CaR-mediated COX-2 expression in primary cultured mTAL cells. Am J Physiol Renal Physiol. (2001) 281:F658–64. doi: 10.1152/ajprenal.2001.281.4.F658
32. Abdullah, HI, Pedraza, PL, McGiff, JC, and Ferreri, NR. Calcium-sensing receptor signaling pathways in medullary thick ascending limb cells mediate COX-2-derived PGE2 production: functional significance. Am J Physiol Renal Physiol. (2008) 295:F1082–9. doi: 10.1152/ajprenal.90316.2008
33. de Baaij, JH, Hoenderop, JG, and Bindels, RJ. Magnesium in man: implications for health and disease. Physiol Rev. (2015) 95:1–46. doi: 10.1152/physrev.00012.2014
34. Gröber, U, Schmidt, J, and Kisters, K. Magnesium in prevention and therapy. Nutrients. (2015) 7, 7:8199–226. doi: 10.3390/nu7095388
35. Bo, S, and Pisu, E. Role of dietary magnesium in cardiovascular disease prevention, insulin sensitivity and diabetes. Curr Opin Lipidol. (2008) 19:50–6. doi: 10.1097/MOL.0b013e3282f33ccc
36. Institute of Medicine (US) standing committee on the scientific evaluation of dietary reference intakes. Dietary reference intakes for calcium, phosphorus, magnesium, vitamin D, and fluoride. Washington (DC): National Academies Press (US) (1997).
37. Garfinkel, L, and Garfinkel, D. Magnesium regulation of the glycolytic pathway and the enzymes involved. Magnesium. (1985) 4:60–72.
39. Jarosz, M, Olbert, M, Wyszogrodzka, G, Młyniec, K, and Librowski, T. Antioxidant and anti-inflammatory effects of zinc. Zinc-dependent NF-κB signaling. Inflammopharmacology. (2017) 25:11–24. doi: 10.1007/s10787-017-0309-4
40. El Sabry, MI, Stino, FKR, and El-Ghany, WAA. Copper: benefits and risks for poultry, livestock, and fish production. Trop Anim Health Prod. (2021) 53:487. doi: 10.1007/s11250-021-02915-9
41. Wen, S, Li, Y, Shen, X, Wang, Z, Zhang, K, Zhang, J, et al. Protective effects of zinc on spinal cord injury. J Mol Neurosci. (2021) 71:2433–40. doi: 10.1007/s12031-021-01859-x
42. Lukaski, HC. Vitamin and mineral status: effects on physical performance. Nutrition. (2004) 20:632–44. doi: 10.1016/j.nut.2004.04.001
43. Chen, K, Zhou, J, Liu, N, and Meng, X. Association of Serum Concentrations of copper, selenium, and zinc with grip strength based on NHANES 2013-2014. Biol Trace Elem Res. (2024) 202:824–34. doi: 10.1007/s12011-023-03718-9
44. Chiu, HT, Kao, TW, Peng, TC, Chen, YY, and Chen, WL. Average urinary flow rate and its association with handgrip strength. Aging Male. (2020) 23:1220–6. doi: 10.1080/13685538.2020.1740201
45. Choi, S, Liu, X, and Pan, Z. Zinc deficiency and cellular oxidative stress: prognostic implications in cardiovascular diseases. Acta Pharmacol Sin. (2018) 39:1120–32. doi: 10.1038/aps.2018.25
46. Schuschke, DA, Falcone, JC, Saari, JT, Fleming, JT, Percival, SS, Young, SA, et al. Endothelial cell calcium mobilization to acetylcholine is attenuated in copper-deficient rats. Endothelium. (2000) 7:83–92. doi: 10.3109/10623320009072203
47. Uriu-Adams, JY, and Keen, CL. Copper, oxidative stress, and human health. Mol Asp Med. (2005) 26:268–98. doi: 10.1016/j.mam.2005.07.015
48. Pimenta, E, Gaddam, KK, Oparil, S, Aban, I, Husain, S, Dell'Italia, LJ, et al. Effects of dietary sodium reduction on blood pressure in subjects with resistant hypertension: results from a randomized trial. Hypertension. (2009) 54:475–81. doi: 10.1161/HYPERTENSIONAHA.109.131235
49. Veniamakis, E, Kaplanis, G, Voulgaris, P, and Nikolaidis, PT. Effects of sodium intake on health and performance in endurance and ultra-endurance sports. Int J Environ Res Public Health. (2022) 19:3651. doi: 10.3390/ijerph19063651
50. Ekmekcioglu, C, Elmadfa, I, Meyer, AL, and Moeslinger, T. The role of dietary potassium in hypertension and diabetes. J Physiol Biochem. (2016) 72:93–106. doi: 10.1007/s13105-015-0449-1
51. Büssemaker, E, Hillebrand, U, Hausberg, M, Pavenstädt, H, and Oberleithner, H. Pathogenesis of hypertension: interactions among sodium, potassium, and aldosterone. Am J Kidney Dis. (2010) 55:1111–20. doi: 10.1053/j.ajkd.2009.12.022
52. Haddy, FJ, Vanhoutte, PM, and Feletou, M. Role of potassium in regulating blood flow and blood pressure. Am J Physiol Regul Integr Comp Physiol. (2006) 290:R546–52. doi: 10.1152/ajpregu.00491.2005
53. Houston, MC. The importance of potassium in managing hypertension. Curr Hypertens Rep. (2011) 13:309–17. doi: 10.1007/s11906-011-0197-8
54. He, FJ, Marciniak, M, Carney, C, Markandu, ND, Anand, V, Fraser, WD, et al. Effects of potassium chloride and potassium bicarbonate on endothelial function, cardiovascular risk factors, and bone turnover in mild hypertensives. Hypertension. (2010) 55:681–8. doi: 10.1161/HYPERTENSIONAHA.109.147488
55. Seelig, MS. Interrelationship of magnesium and estrogen in cardiovascular and bone disorders, eclampsia, migraine and premenstrual syndrome. J Am Coll Nutr. (1993) 12:442–58. doi: 10.1080/07315724.1993.10718335
56. Yavuz, M, and Etiler, N. Addressing urinary incontinence by gender: a nationwide population-based study in Turkiye. BMC Urol. (2023) 23:205. doi: 10.1186/s12894-023-01388-2
57. Haylen, BT, Ashby, D, Sutherst, JR, Frazer, MI, and West, CR. Maximum and average urine flow rates in normal male and female populations--the Liverpool nomograms. Br J Urol. (1989) 64:30–8. doi: 10.1111/j.1464-410x.1989.tb05518.x
Keywords: urine flow rate, intakes of dietary minerals, dietary mineral intakes, cross-section study, NHANES
Citation: Li M, Zhang J, Ding J and Gao Z (2024) Association between dietary mineral intakes and urine flow rate: data from the 2009–2018 National Health and Nutrition Examination Survey. Front. Nutr. 11:1424651. doi: 10.3389/fnut.2024.1424651
Edited by:
Mohd Kamil Hussain, Govt. Raza Post Graduate College, Rampur, IndiaReviewed by:
Mehran Rahimlou, Zanjan University of Medical Sciences, IranDorota Formanowicz, Poznan University of Medical Sciences, Poland
Copyright © 2024 Li, Zhang, Ding and Gao. This is an open-access article distributed under the terms of the Creative Commons Attribution License (CC BY). The use, distribution or reproduction in other forums is permitted, provided the original author(s) and the copyright owner(s) are credited and that the original publication in this journal is cited, in accordance with accepted academic practice. No use, distribution or reproduction is permitted which does not comply with these terms.
*Correspondence: Zhan Gao, gaozhanmd@vip.163.com
†These authors have contributed equally to this work and share first authorship