- 1College of Grass Science and Technology, China Agricultural University, Beijing, China
- 2School of Grassland Science, Beijing Forestry University, Beijing, China
Objectives: The objective of this study was to investigate the effect of different harvest times and processing methods on the B vitamins and α-tocopherol contents of Leymus chinensis (Trin.).
Methods: L. chinensis was harvested on 11 July (T1 group), 16 July (T2 group), 21 July (T3 group), 26 July (T4 group), and 31 July (T5 group) in 2022 and processed using natural drying and silage fermentation to evaluate fermentation quality, chemical composition, in vitro digestibility and vitamin content.
Results: The fermentation quality of L. chinensis silage prepared at all five times of harvest was better. The silage fermentation group showed a significant increase (p < 0.05) in crude protein (CP), thiamin, riboflavin, pyridoxine and α-tocopherol content, a significant decrease (p < 0.05) in water-soluble carbohydrate (WSC) content, and small differences in neutral detergent fibre (NDF), acid detergent fibre (ADF), niacin and pantothenic acid content, when compared to the natural drying group. The content of thiamine, riboflavin, niacin, pantothenic acid and pyridoxine were higher in the pre-harvest period. In silage fermentation, the loss rate of thiamin, riboflavin and pyridoxine was positively correlated with pH and WSC, and the loss rate of thiamin and riboflavin was negatively correlated with lactic acid content. The loss rate of pantothenic acid was negatively correlated with pH and WSC, and positively correlated with lactic acid and ammonia nitrogen. The rate of α-tocopherol synthesis exceeded the rate of catabolism.
Conclusion: The content of CP, thiamine, riboflavin, niacin, pantothenic acid and pyridoxine were higher during the early harvest period. Silage fermentation preserved the chemical composition and vitamin content of L. chinensis better than natural drying and had no effect on in vitro digestibility. During silage fermentation, the acidic environment promoted the preservation of thiamin, riboflavin and pyridoxine, but promoted the breakdown of pantothenic acid, α-tocopherol content increased through synthesis.
1 Introduction
L. chinensis is a perennial forage grass of the genus Lymus in the family Gramineae, L. chinensis represents over 50% of the forage production of natural grassland in the Inner Mongolian grasslands, and is a significant local forage resource. The hay of L. chinensis has excellent nutritional value, good palatability and high feeding rate, so at present, natural drying is the main way of utilising L. chinensis (1). The impact of varying harvest times on nutritional components, such as crude protein and neutral detergent fibre, in L. chinensis hay has been documented. Harvesting too early results in higher nutritional quality but lower yields, while harvesting too late increases the stem-to-leaf ratio of the forage, resulting in lower nutritional quality, higher fiber content and reduced forage intake (2). Nevertheless, the impact of varying harvest times on the vitamin content of L. chinensis hay has yet to be documented. B vitamins are a group of water-soluble vitamins that participate in the body’s metabolism as coenzymes or cofactors in ruminants. While, α-tocopherol is a general term for all tocopherols and tocotrienols with d-α-tocopherol activity, the main form of which is α-tocopherol, acting as a significant fat-soluble antioxidant in animals (3). Even ruminants are able to synthesise B vitamins through rumen microorganisms, while it is difficult to maintain the requirements of high productivity animals; they are able to synthesise α-tocopherol, neither. B vitamins and α-tocopherol still need to be supplemented through the intake of fresh or preserved forage (4). Dornbach et al. (5) concluded that supplementation of bulls with α-tocopherol increased their antioxidant capacity and reduced hair cortisol concentrations. Kanyar et al. (6) added α-tocopherol (400 mg/d) to lamb diets and found that it improved meat colour stability and lipid peroxidation in muscle tissue. Castagnino et al. (7) fed dairy cows with forages of different maturity levels and found that different types of forages at the same maturity level had different effects on the intake of B vitamins in cows. It is therefore of great importance to investigate the impact of varying harvest times on the vitamin content of L. chinensis.
Natural drying results in significant nutrient loss due to respiration during drying, if it rains during the drying process, it will exacerbate the loss of nutrients, resulting in a serious decline in feed quality. In comparison to hay, silage of L. chinensis has been demonstrated to reduce forage nutrient losses, improve digestibility, increase palatability and livestock intake, and also to improve some vitamin content (8). Harvest time is a crucial factor affecting forage quality. Czurgiel et al. (9) found that rams contained more α-tocopherol in their muscle tissue after feeding silage. Castagnino et al. (10) found that particle length of silage affected the efficiency of rumen synthesis of B vitamins in dairy cows. Van den Oever et al. (11) fed hay and silage separately to dairy cows and found that feeding silage increased B12 levels in milk relative to hay. Nevertheless, there is a paucity of research investigating the effects of B vitamins and vitamin E on L. chinensis silage harvested at different times, and the changing patterns and influencing factors in the silage process remain largely unexplored. Therefore, the aim of this study was to investigate the effects of different harvest times and processing methods on B vitamins and α-tocopherol, as well as to analyze the chemical composition and in vitro digestion.
2 Materials and methods
2.1 Test materials and experimental design
The study area was located at the Grassland Agroecosystem Experimental Station in Chenbalhu Banner, Hulunbeier City, Inner Mongolia (N 49°20′–49°26′, E 119°55′–120°09′, altitude 628–649 m), P. R. China. and Leymus chinensis (Zhongke no. 2) (12) was harvested in the meadow grassland sample plots, using a five-point sampling method in 2022 on July 11 (T1 group), July 16 (T2 group), July 21 (T3 group), July 26 (T4 group), July 31 (T5 group). The processing methods of natural drying and silage fermentation were designed. Figure 1 provides the operational steps of the test. The specific methods of operation were as follows.
Natural drying group: L. chinensis was harvested with 4 to 5 cm stubble height. Approximately 500 g of L. chinensis was taken and placed in a nylon mesh bag to dry naturally in the sun until constant weight. Then chopped into about 2 cm pieces with a forage cutter, and then sampled. Each treatment had three repetitions.
Silage fermentation group: L. chinensis was harvested with 4 to 5 cm stubble height. The L. chinensis was chopped into about 2 cm piece with forage cutters. Then approximately 150 g of chopped L. chinensis forage were filled into a polyethylene bag silo (30 × 40 cm; 0.19 mm thickness) without any additives added. The silo was sealed with a vacuum sealer (FW3150; Fresh World Electir Co., Ltd., Guangzhou, China). Each treatment had three repetitions. All silos were stored at room temperature and opened and sampled after 70 days of ensiling.
2.2 Measurement content and methods
2.2.1 Chemical analysis
Twenty-gram samples were mixed with 180 mL of distilled water and homogenized in a juicer for 2 min. The resulting mixture was filtered, and the filtrate was used to determine pH, ammonia nitrogen and organic acid content. The pH was measured using a pH meter (PHS-3C, INESA Scientific Instrument Co., Ltd., Shanghai, China). The levels of organic acids, including lactic, acetic, propionic, and butyric acids, were analysed using high-performance liquid chromatography (HPLC; column: Shodex RS Pak KC-811, Showa Denko KK, Kawasaki, Japan; detector: DAD, 210 nm, SPD-20A, Shimadzu Co., Ltd., Kyoto, Japan; eluent: 3 mmol/L HClO4, at a flow rate of 1.0 mL/min; column temperature: 50°C). The concentration of ammonia nitrogen was analysed using the phenol and sodium hypochlorite method (13). The dry matter (DM) content was measured after oven-drying at 65°C for 48 h. The oven-dried samples were milled and passed through a 0.425 mm screen to analyse conventional compositional of feeds, which included crude protein (CP), water-soluble carbohydrate (WSC), neutral detergent fiber (NDF), acid detergent fiber (ADF) and ether extract (EE). The CP content was analyzed according to the method provided by AOAC (14). The WSC content was analyzed by anthrone-sulphuric acid colourimetric method (15). The NDF and ADF contents were analyzed according to the procedures of Van Soest et al. (16).
2.2.2 In vitro digestibility analysis
In vitro dry matter digestibility (IVDMD), in vitro neutral detergent fiber digestibility (IVNDFD) and in vitro crude protein digestibility (IVCPD) were determined by the following method: the oven-dried (65°C for 48 h) samples were milled and passed through a 0.425 mm screen, then 0.5 g was weighed accurately and placed in a fibre bag that had been soaked in acetone and naturally dried. The bag was put into the digestion tanks of the in vitro simulation incubator (Ankom Technologies, Macedon, NY, United States), with 24 samples and one blank control in each tank. 1,330 mL of A buffer and 266 mL of B buffer (Buffer A: 10 g KH2PO4, 0.5 g MgSO4-7H2O, 0.5 g NaCl, 0.1 g CaCl2-2H2O and 0.5 g urea dissolved in one litre of water. Buffer B: 15 g Na2CO3 and 1 g Na2S-9H2O dissolved in one litre of water) was added to the tanks, followed by 400 mL of filtered rumen fluid. Carbon dioxide (CO2) was then passed into the tanks until they were filled, and the lids were closed. Subsequently, the digestion jar was placed in an in vitro simulation incubator and digested at 39°C for 48 h. The samples were removed and rinsed with distilled water and dried in an oven at 65°C for 48 h (17).
2.2.3 Vitamin analysis
The vitamin concentrations were determined by HPLC. The sample was freeze-dried using a vacuum freeze-drying machine for 2 days (FreeZone 4.5 L, LABCONCO Corp., Kansas City, MO, United States) before analysis. The freeze-dried samples were passed through a 0.425 mm screen.
Using the method of Jia et al. (18), thiamin, riboflavin, niacin, pantothenic acid, pyridoxine, and α-tocopherol were determined: thiamin concentrations were determined in duplicate based on the method of GB/T 14700-2018, riboflavin levels were analyzed in duplicate according to the method of GB/T 14701-2019, niacin levels were examined according to the method of GB/T 17813-2018, pantothenic acid levels were measured based on the method of GB/T 18397-2014, pyridoxine levels were determined based on the method of GB/T 14702-2018, the α-tocopherol levels were analyzed based on the method of GB/T 17812-2008.
2.3 Statistical analysis
The vitamin content, fermentation quality, in vitro digestibility, and chemical composition data were analyzed by analysis of variance (ANOVA), using the general linear model-univariate procedure of SPSS 19.0 software. ANOVAs were performed for harvest times and processing methods as the two main parameters and for the interaction between the two parameters. The mean values were compared using Duncan’ s multiplerange tests. Differences between means were considered significant when p < 0.05. Correlation analysis between the loss rate of vitamin B group or the growth rate of α-tocopherol in L. chinensis silage and other analyzed variables was performed using the Pearson correlation coefficient. Correlation analysis between the loss rate of vitamin B group or the growth rate of α-tocopherol in L. chinensis silage and other analyzed variables was performed using the Pearson correlation coefficient in SPSS (19).
3 Results and analysis
3.1 Chemical composition, vitamin content, and in vitro digestibility of L. chinensis before processing at different harvest times
Table 1 summarizes the chemical composition, vitamin content and in vitro digestibility of L. chinensis before processing at different harvest times. DM content was affected by harvest time (p < 0.001). The effect of harvest time on the chemical composition was significant (p < 0.05). The content of CP increased initially and then decreased, while the content of NDF and ADF decreased initially and then increased with increasing of harvest time. The T3 group had the lowest NDF, ADF, and EE content and the highest CP content. The effect of harvest time on the content of thiamine, riboflavin, niacin, pyridoxine and α-tocopherol was significant (p < 0.05) and insignificant (p > 0.05) on pantothenic acid content. The content of thiamine, riboflavin, niacin, pantothenic acid and pyridoxine increased initially and then decreased with the prolongation of the harvest time and then was maximum in the T2 group. The content of α-tocopherol increased initially and then decreased with increasing of the harvest time and then was maximum in the T4 group. There was no significant effect of harvest time on IVDMD and IVNDFD (p > 0.05), but there was a significant effect on IVCPD (p < 0.05). IVCPD increased initially and then decreased with increasing harvest time, with the highest value observed in the T2 group.
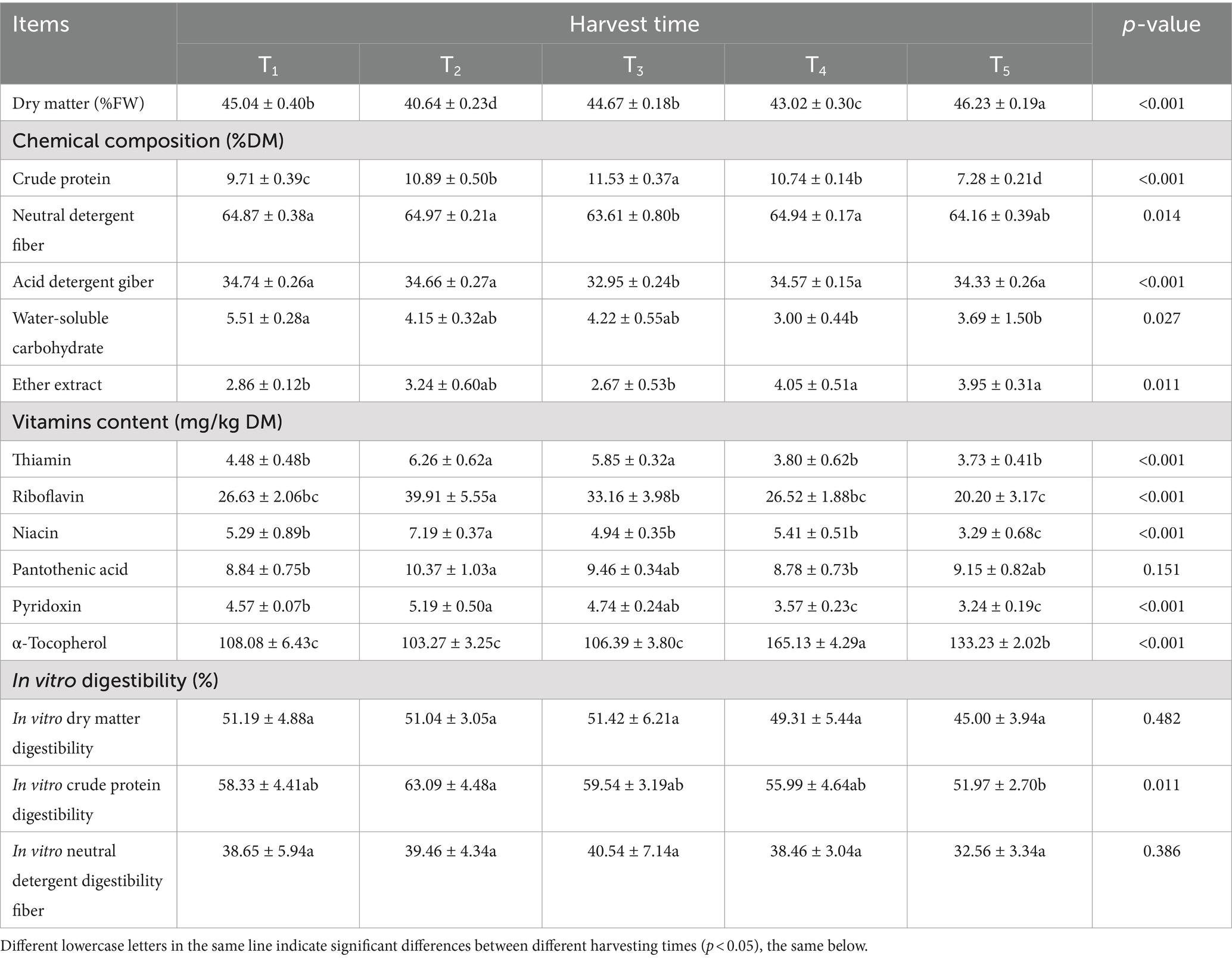
Table 1. Chemical composition, vitamin content, and in vitro digestibility of L. chinensis before processing at different harvest times.
3.2 Fermentation quality of L. chinensis silage at different harvest times
As shown in Table 2, harvest time significantly (p < 0.05) affected lactic acid, acetic acid, propionic acid, ammoniacal nitrogen content and pH for L. chinensis silage. The pH of the T1 group was significantly higher than that of the other groups (p < 0.05); the content of acetic acid in the T1 and T3 groups was significantly lower than that of the T2 and T5 groups (p < 0.05); the content of propionic acid in the T2 group was significantly higher than that of the other groups (p < 0.05); the content of butyric acid in the T1 group was significantly lower than that in the T5 group (p < 0.05), while the content of ammoniacal nitrogen in the T5 group was significantly higher than that in the other groups (p < 0.05).
3.3 Chemical composition of L. chinensis forage with different harvest times and processing methods
Table 3 show that the interaction effect of harvest time and processing method on NDF, ADF, WSC and DM content was significant (p < 0.05). Harvest time and processing method had a significant (p < 0.05) effect on all indicators.
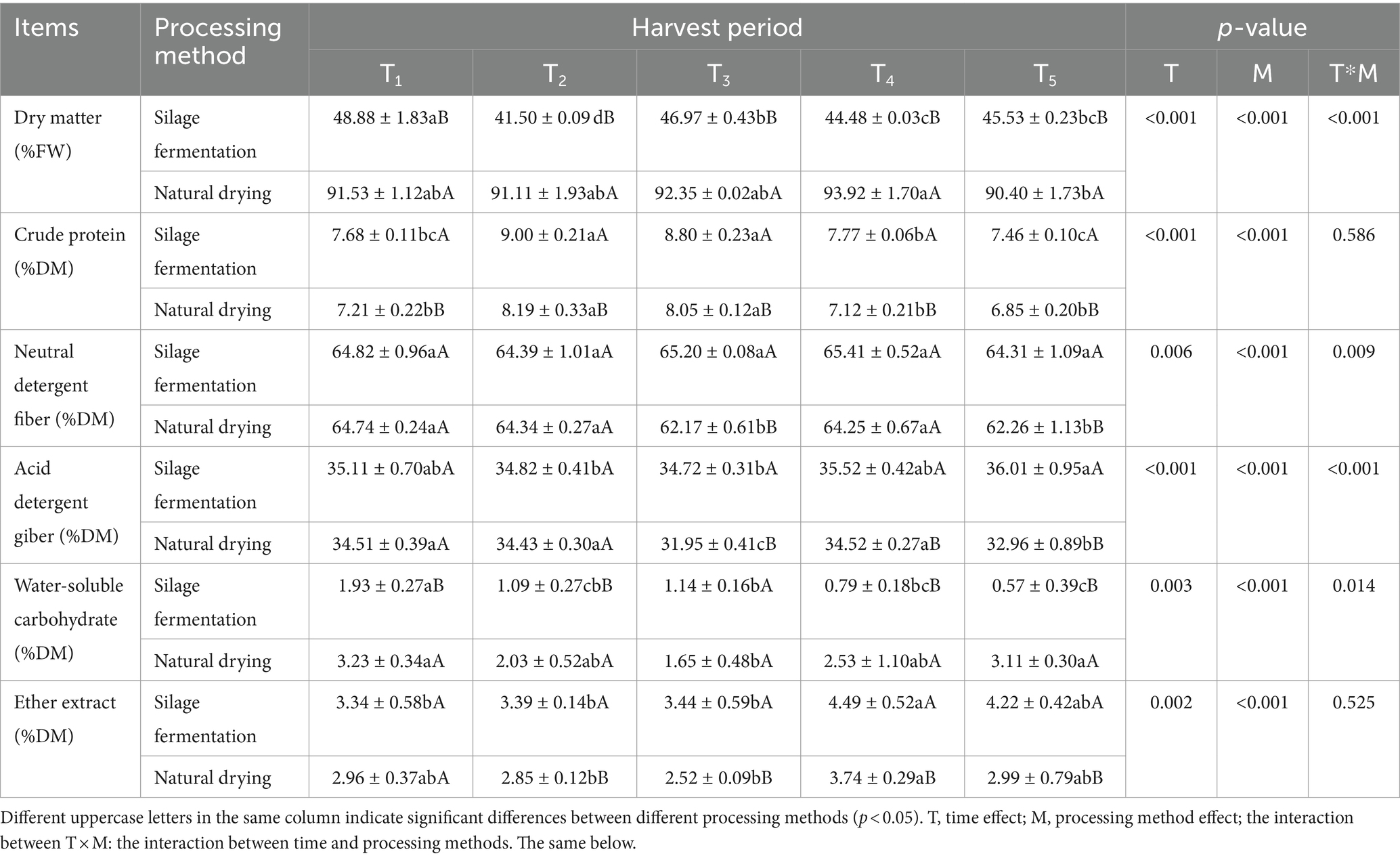
Table 3. Chemical composition of L. chinensis feed with different harvest times and processing methods.
Within the silage fermentation group, the DM content was significantly higher (p < 0.05) in group T1 and significantly lower (p < 0.05) in group T2 than in the other harvest times; the CP content was highest in the T2 group, which did not differ significantly from the T3 group (p > 0.05), but was significantly higher than that of the T1, T4, and T5 groups (p < 0.05), while the T5 group had the lowest CP content; the NDF content of the groups did not differ significantly (p > 0.05); the content of ADF in the T2 and T3 groups did not differ significantly from that in the T1 and T4 groups (p > 0.05) and was significantly lower than that in the T5 group (p < 0.05); WSC content in T1 group was significantly higher than that in the other the harvest times (p < 0.05); and the EE content in T4 group was significantly higher than that of the T1, T2 and T3 groups (p < 0.05).
Within the natural drying group, the DM content of the T4 group was significantly higher than that of the T5 group (p < 0.05); the CP content of the T2 and T3 groups was significantly higher than that of the T1, T4 and T5 groups (p < 0.05); the NDF and ADF content of the T3 and T5 groups was significantly lower than that of the T1, T2 and T4 groups (p < 0.05), while the ADF content of the T3 group was significantly lower than that of the T5 group (p < 0.05); the WSC content of the T3 group was significantly lower than that of the T1 and T5 groups (p < 0.05), while the difference with T2 and T4 groups was not significant (p > 0.05); the EE content of the T4 group was significantly higher than that of the T2 and T3 groups (p < 0.05).
The CP content of silage fermentation group was significantly higher than that of natural drying group (p < 0.05); the NDF and ADF content of silage fermentation group was significantly higher than that of natural drying group in T3 and T5 groups (p < 0.05); the WSC content of silage fermentation group was significantly lower than that of natural drying group in T1, T2, T3 and T4 groups (p < 0.05); the EE content of silage fermentation group was significantly higher than that of natural drying group in T2, T3, T4 and T5 groups (p < 0.05).
3.4 In vitro digestibility of L. chinensis feed with different harvest times and processing methods
Table 4 shows that the interaction between harvest time and processing method did not have a significant effect (p > 0.05) on IVDMD, IVCPD, and IVNDFD. However, harvest time had a significant effect (p < 0.05) on IVNDFD, while processing method had a significant effect (p < 0.05) on IVDMD, IVCPD, and IVNDFD. In silage fermentation group, IVNDFD in T4 group was significantly higher than T1 and T5 groups (p < 0.05), and the difference of IVNDFD in T2, T3 and T4 groups was not significant (p > 0.05). In natural drying group, IVNDFD of group T3 was not significantly different from that of groups T2, T3 and T4 (p > 0.05) and was significantly higher than that of group T1 (p < 0.05). In T5 group, the silage fermentation group had significantly higher IVCPD (p < 0.05) and significantly lower IVDMD and IVNDFD (p < 0.05) compared to the natural drying group.
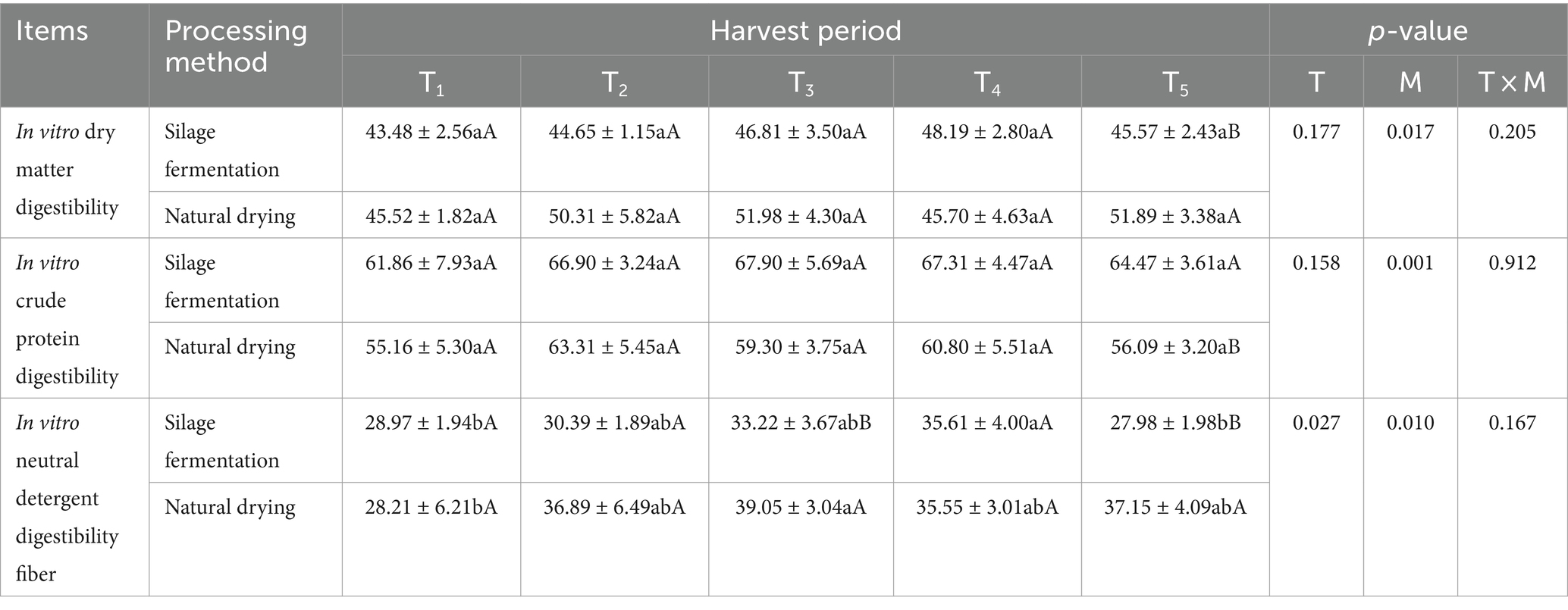
Table 4. In vitro digestibility of L. chinensis feed with different harvest times and processing methods (%).
3.5 Vitamin content in L. chinensis feed with different harvesting times and processing methods
As shown in Table 5, the interaction effect of harvesting time and processing method on thiamin, riboflavin and α-tocopherol content was significant (p < 0.05); harvest time on all vitamin contents was significant (p < 0.05); processing method did not have significant effect on niacin content (p > 0.05), and the rest of the vitamins were significantly affected (p < 0.05).
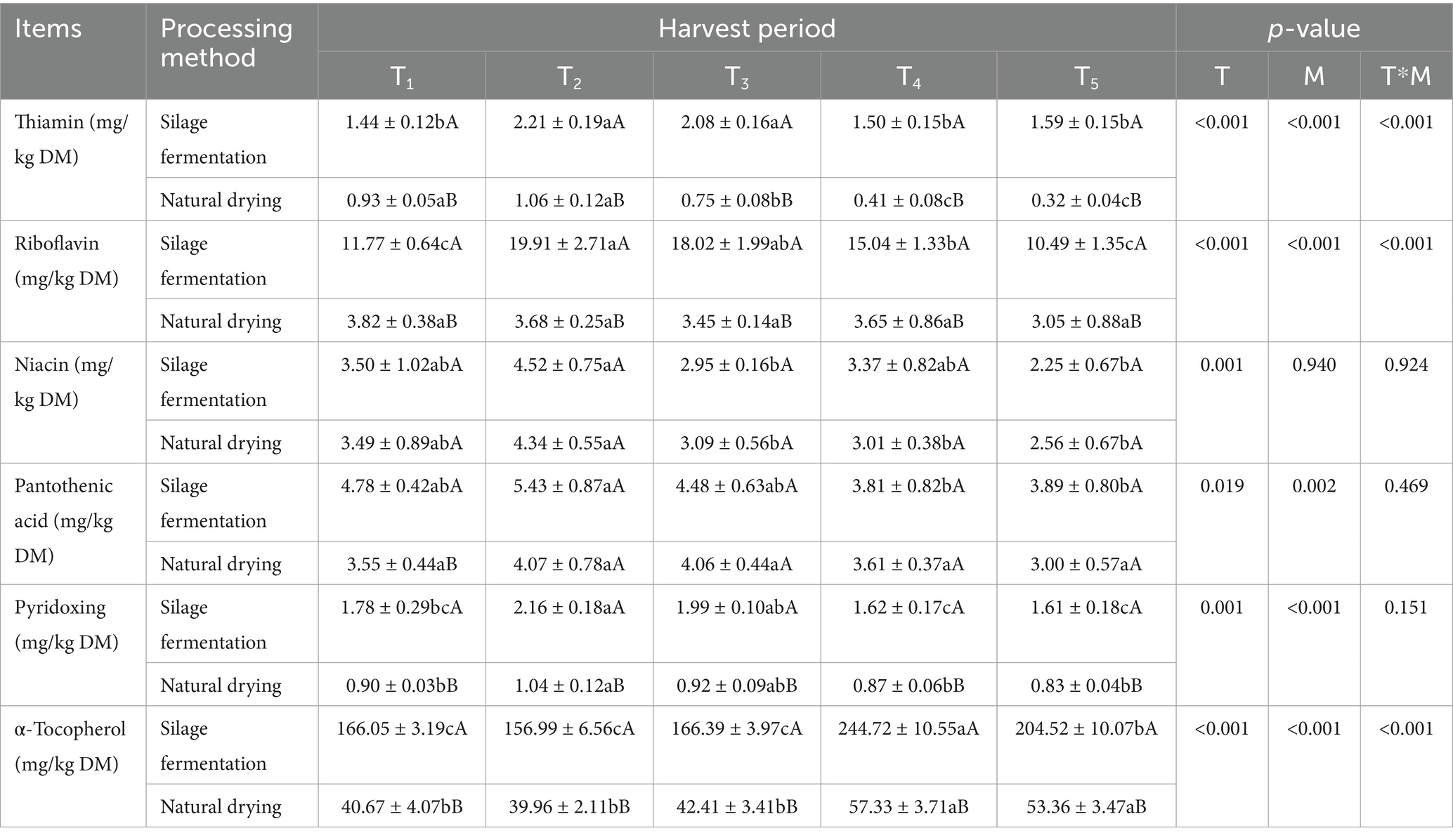
Table 5. Vitamin content in L. chinensis feed with different harvesting times and processing methods.
In silage fermentation group, the contents of thiamine, riboflavin, and pyridoxine initially increased and then decreased as the harvest time was prolonged, while the T2 group had significantly higher levels of these vitamins compared to the T1, T4, and T5 groups (p < 0.05), but there was no significant difference between the T2 and T3 groups (p > 0.05). The T2 group had the highest niacin content, which was significantly higher than the T3 and T5 groups (p < 0.05). The pantothenic acid content of the T2 group was significantly higher than T4 and T5 groups (p < 0.05); The α-tocopherol content initially increased and then decreased as the harvest time was prolonged, while the highest content was observed in the T4 group, which was significantly higher than the other groups (p < 0.05). Additionally, the T5 group also had significantly higher α-tocopherol content than the T1, T2, and T3 groups (p < 0.05).
In natural drying group, the T2 group had significantly higher levels of thiamine and niacin compared to the T3, T4, and T5 groups (p < 0.05), and the difference with the T1 group was not significant (p > 0.05). pyridoxine content was significantly higher in the T2 group than in the T1, T4 and T5 groups (p < 0.05); and α-tocopherol content in the T4 and T5 groups was significantly higher than in the T1, T2 and T3 groups (p < 0.05).
Thiamin, riboflavin, pyridoxine and α-tocopherol content was significantly higher (p < 0.05) in silage fermentation group than in natural drying group.
3.6 Pearson correlation analysis between the loss rate of vitamin B group or the growth rate of α-tocopherol in L. chinensis silage and other analyzed variables
As shown in Table 6. In L. chinensis silage, the rate of thiamin loss was positively correlated with pH and WSC (p < 0.05), and negatively correlated with lactic acid, propionic acid, ammoniacal nitrogen, and ADF (p < 0.05); the rate of riboflavin loss was positively correlated with pH and WSC (p < 0.05), and negatively correlated with lactic acid, EE and IVCPD (p < 0.05); the rate of pyridoxine loss was positively correlated with pH and WSC (p < 0.05), and negatively correlated with propionic acid and ammoniacal nitrogen (p < 0.05); the rate of pantothenic acid loss was positively correlated with lactic acid and ammoniacal nitrogen (p < 0.05), and negatively correlated with pH and WSC (p < 0.05); There was no correlation found between the rate of niacin loss and the other variables (p > 0.05); The growth rate of α-tocopherol was negatively correlated with IVCPD (p < 0.05).
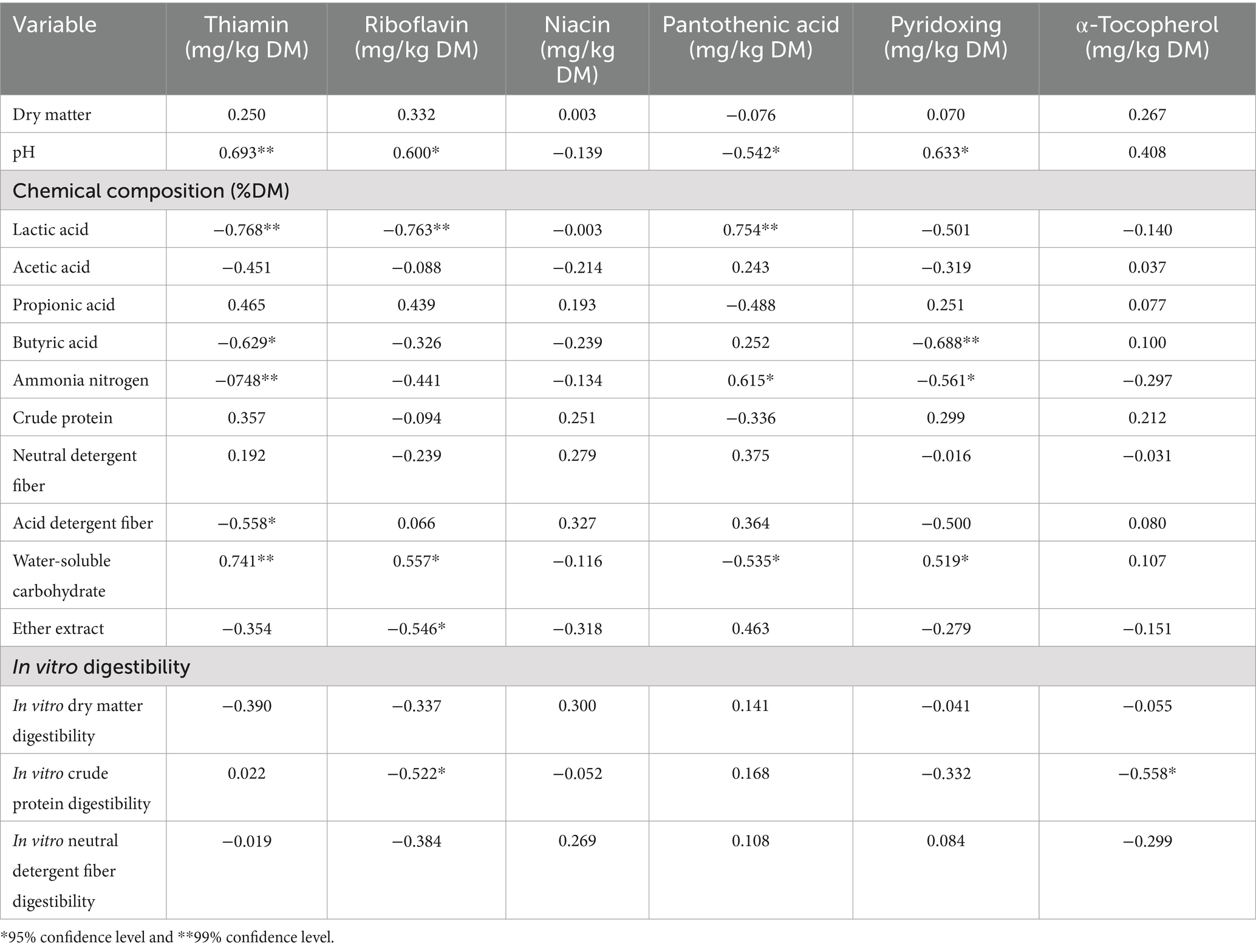
Table 6. Pearson correlation analysis between the loss rate of vitamin B group or the growth rate of α-tocopherol in L. chinensis silage and other analyzed variables.
4 Discussion
4.1 Effect of different harvest times on the fermentation quality of L. chinensis silage
It is erroneous to assume that pH is the sole criterion for evaluating the quality of fermentation. A multitude of factors, including dry matter content and buffering energy, exert a profound influence on the pH of silage (17). The pH values of L. chinensis silage at different harvest times were higher than 4.2, but the NH3-N/TN values were lower than 8%, while the fermentation quality was still good. The low moisture content of L. chinensis silage inhibits the growth of moulds and spoilage bacteria such as clostridia, which hinders the production of butyric acid and the decomposition of proteins (17). Additionally, the anaerobic state inhibits the activity of fungi. The activity of lactic acid bacteria is also weakened by low moisture content, resulting in fewer organic acids being produced and a higher pH value (20).
4.2 Effects of different harvest time and processing methods on the chemical composition of L. chinensis
The cell wall will be degraded into soluble monosaccharides during silage (65%–75% moisture content), and the content of NDF and ADF will be reduced to provide carbon source for lactic acid bacteria to improve silage quality (21). The NDF and ADF contents of silage fermentation group in this experiment are not different or even higher than those of natural drying group, this is because the moisture content of L. chinensis silage is low, and the osmotic pressure of plant cells reaches 55 × 105–60 × 105 Pa (22) at low moisture, in this case, the life activities of spoilage bacteria and lactic acid bacteria were close to physiological drying, which restricted their growth and reproduction. This also led to a reduction in the enzyme responsible for fiber degradation, resulting in a decrease in fiber decomposition. As a result, the content of NDF and ADF did not decrease after silage. In the silage fermentation group, the NDF and ADF content increased relatively due to the decrease in WSC content. The WSC content of the silage fermentation group was lower than that of the natural drying group. This is because lactic acid bacteria consume soluble carbohydrates during the silage process, leading to a decrease in WSC content after silage. As the plant growth time increases, the ratio of stem to leaves gradually increases, while the content of CP decreases and the content of NDF and ADF increases (23). But in this experiment, the CP content initially increased and then decreased with the prolongation of harvest time. Similarly, the NDF and ADF content fluctuated with the prolongation of harvest time. These changes may be attributed to the significant correlation between the growth state of forage grasses and environmental factors such as temperature, precipitation, humidity, and length of light exposure (24).
4.3 Effect of different harvest times and processing methods on in vitro digestibility
IVNDF in groups T3 and T5 and IVDMD in group T5 were lower in silage fermentation than in natural drying, which is consistent with the study by Riberio et al. (25). This is due to the fact that a greater loss of WSC occurs during the ensiling process, whereas a lesser loss of WSC takes place during the natural drying process. Following the loss of WSC, the NDF content expressed as a percentage of DM increased, resulting in an elevated NDF content after ensilage. WSC is readily digestible during in vitro digestion, resulting in a notable increase in IVDMD in natural drying group. Similarly, the ensiling process generates enzymes and acidic conditions that facilitate the degradation of hemicellulose, whereas the natural drying process does not degrade hemicellulose. Given that hemicellulose is an easily digestible component of the fiber composition, the readily digestible fiber components in silage decline, resulting in an increase in IVNDF in the silage fermentation group. Enzymatic in silage can alter its surface structure, increasing porosity, surface area, and accessibility between fibers and crude proteins, enhancing the contact area between rumen microbial enzymes and silage in ruminants (26). Simultaneously, the enzyme cleaves the bond connecting cellulose and hemicellulose, reducing the crystallinity of cellulose and increasing its specific surface area. This facilitates the attachment and degradation of rumen microorganisms (27). Thus, there were no significant differences in IVDMD and IVNDF between the silage fermentation group and the natural drying group during other times.
4.4 Effect of different harvest time and processing methods on vitamin content
The pre-harvest period showed higher levels of thiamine, riboflavin, niacin, pantothenic acid, and pyridoxine, which is consistent with the findings of Castagnino and Tadevosyan (7, 28). It is currently believed that B vitamins in plants are synthesized in chloroplasts, and the number of chloroplasts is directly related to the content of B vitamins. Leaves are richer in chloroplasts compared to stems. Early in the life of the plant, the stem-leaf ratio is low, with more chloroplasts per unit of mass. As the harvest time was prolonged, the stem-leaf ratio of the plant was elevated. Consequently, in the later stages of the plant, B vitamin content declined (23).
Silage fermentation effectively reduced the loss of thiamin, riboflavin, and pyridoxine during storage compared to natural drying. This is because these vitamins are highly sensitive to light and easily oxidised by oxygen, however, they are relatively stable to heat and oxygen under acidic conditions (29). Thiamine, riboflavin, and pyridoxine undergo oxidation and photodegradation during prolonged natural drying, but the acidic, anaerobic, light-avoiding environment of silage fermentation reduced the oxidation and photodegradation of these vitamins (30). However, a study by van den Oever et al. (11) found no significant difference in thiamin levels between silage and sun-drying treatments. This may be due to the instability of thiamin, which could degrade during the silage process if exposed to direct sunlight or excess oxygen in the pre-silage stage. In silage, the rates of thiamin, riboflavin and pyridoxine loss were positively correlated with pH and WSC, and thiamin and riboflavin loss rates were negatively correlated with lactic acid content. Due to the similar characteristics of thiamin, riboflavin, and pyridoxine (29), as well as the fact that the rate of loss of pyridoxine is independent of the lactate content, we cannot infer that lactate affects the rate of loss of thiamin and riboflavin, despite the negative correlation between the rate of loss of thiamin and riboflavin and the lactate content. As lactic acid production from WSC consumption by Lactobacillus in silage fermentation leaded to a decrease in pH, it was initially deduced that pH is the main factor affecting the rates of loss of thiamin, riboflavin and pyridoxine. The pH was reduced by lactic acid production by Lactobacillus to slow down the rates of loss of thiamin, riboflavin and pyridoxine in silage. The lacked of correlation between the rate of pyridoxine loss and lactic acid content may be attributed to the superior stability of pyridoxine in comparison to thiamine and riboflavin (31).
Niacin is a water-soluble vitamin that is highly stable and tolerant to oxygen, acid, heat, and light in both aqueous and solid systems (32). One study indicated that niacin-rich bakery products experienced only a slight decrease in niacin content after 8 months of storage, regardless of storage conditions (with or without light and with or without refrigeration) (33), however, niacin was still lost to varying degrees (1–12%) at different high temperatures (34). Jia et al. (18) discovered that the niacin content of L. chinensis decreased by more than 50% after silage, and that there was further loss of niacin content after the addition of lactobacilli. The results of the present experiment were consistent with those of the above experiments. There were varying degrees of loss in niacin content in L. chinensis after undergoing silage fermentation and natural drying, but there was no significant difference in the niacin content between the two processes. This could be attributed to the fact that niacin has a high tolerance to oxygen, acid, heat and light, while the effect of oxygen and light on niacin was minimal in the case of sun-drying, which led to the absence of differences.
In silage, the rate of pantothenic acid loss was negatively correlated with pH and WSC, and positively correlated with lactic acid and ammoniacal nitrogen. Consumption of WSC by lactobacilli, which produced lactic acid, affected pH and the rate of pantothenic acid loss, suggesting a pathway for pantothenic acid loss. The rate of loss of pantothenic acid decreased with an increase in pH. Pantothenic acid is more stable in weakly alkaline environments than in acidic ones. It is hydrolysed in acidic environments. The highest stability of pantothenic acid is between pH 5–7. As the pH content of T1 and T2 groups was greater than 5, the loss of pantothenic acid was lower in T1 and T2 groups than in other groups (35).
The primary active constituent of α-tocopherol is α-tocopherol. It is present in the chloroplasts of plants and is produced in two ways within the plant body. One method involves synthesising it from scratch through chloroplasts, while the other involves phytol, a precursor of α-tocopherol synthesis, which is produced directly through the degradation of chloroplasts (36). The increase in α-tocopherol content during silage could be attributed to the degradation of chloroplasts, which produced large amounts of phytol. Phytol synthesised α-tocopherol, and the rate of synthesis was much greater than the rate of α-tocopherol degradation (37). The increase in α-tocopherol content in the T4 group compared to the previous three periods may be attributed to the large number of spikes in the L. chinensis and the α-tocopherol content in the spikes was higher than that in the stems and leaves, resulting in an overall increase in α-tocopherol content (38). The content of α-tocopherol was significantly reduced after natural drying due to its sensitivity to light and oxygen (39). Hidiroglou et al. (40) found that the α-tocopherol content of hay was 30–40% of that of silage. In this study, L. chinensis hay had a slightly higher α-tocopherol content, which may be related to the climatic conditions during sun-drying. In addition, it was found that the acidic environment is very favorable for the preservation of α-tocopherol and promotes the release of Mg+ to synthesize α-tocopherol. The lowest loss of α-tocopherol occurred at pH 4, with an increase in pH resulting in a higher loss of α-tocopherol (41). However, the growth rate of α-tocopherol in silage in this experiment was not correlated with pH and other variables. This may be because the rate of α-tocopherol synthesis in silage exceeded the rate of degradation, which is consistent with the findings of Tian et al. (42).
5 Conclusion
The silage made from L. chinensis exhibited good fermentation quality at all harvest times. Furthermore, the silage fermentation preserved the chemical component and vitamin content of the L. chinensis better than natural drying. Levels of crude protein (CP), thiamin, riboflavin, niacin, pantothenic acid and pyridoxine were higher during the early harvest period. During silage fermentation, the acidic environment promoted the preservation of thiamin, riboflavin, and pyridoxine, but it also accelerated the decomposition of pantothenic acid. In contrast, niacin was relatively stable during processing. Meanwhile α-tocopherol content was increased.
Data availability statement
The original contributions presented in the study are included in the article/supplementary material, further inquiries can be directed to the corresponding author.
Author contributions
YW: Data curation, Investigation, Methodology, Software, Writing – original draft, Writing – review & editing. XC: Investigation, Writing – review & editing. XZ: Writing – review & editing. LW: Writing – review & editing. TJ: Writing – review & editing. FJ: Writing – review & editing. HZ: Writing – review & editing. ZY: Data curation, Funding acquisition, Project administration, Writing – review & editing.
Funding
The author(s) declare that financial support was received for the research, authorship, and/or publication of this article. National Key Research and Development Programme “Intergovernmental Cooperation in Science and Technology Innovation” (Project No. 2022YFE0111000), Key Technology Integration and Demonstration for Improving the Quality and Efficiency of Characteristic Breeding Industry in Zaodo County, Yushu Prefecture, Qinghai Province (Project No. 2022YFD1602305), Key Technology Development and Demonstration of High Quality Forage Production and Grass Product Processing Key Technology R&D and Demonstration (Project No. CCPTZX2023B07), Grass-fed Animal Husbandry Increasing Quantity and Improving Quality Science and Technology Innovation Co-operation (Project No. DL2023108002L), funded by the Ministry of Finance and the Ministry of Agriculture and Rural Affairs: the National Modern Agricultural Industrial Technology System (Project No. CARS-34).
Conflict of interest
The authors declare that the research was conducted in the absence of any commercial or financial relationships that could be construed as a potential conflict of interest.
Publisher’s note
All claims expressed in this article are solely those of the authors and do not necessarily represent those of their affiliated organizations, or those of the publisher, the editors and the reviewers. Any product that may be evaluated in this article, or claim that may be made by its manufacturer, is not guaranteed or endorsed by the publisher.
References
1. Yan, R, Chen, S, Zhang, X, Han, J, Zhang, Y, and Undersander, D. Short communication: effects of replacing part of corn silage and alfalfa hay with Leymus chinensis hay on milk production and composition. J Dairy Sci. (2011) 94:3605–8. doi: 10.3168/jds.2010-3536
2. McEniry, J, King, C, and O’Kiely, P. Silage fermentation characteristics of three common grassland species in response to advancing stage of maturity and additive application. Grass Forage Sci. (2014) 69:393–404. doi: 10.1111/gfs.12038
3. Stobiecka, M, Król, J, and Brodziak, A. Antioxidant activity of milk and dairy products. Animals. (2022) 12:245. doi: 10.3390/ani12030245
4. Elgersma, A, Soegaard, K, and Jensen, SK. Vitamin contents in forage herbs. Asp Appl Biol. (2012) 115:75–80.
5. Dornbach, CW, Beenken-Bobb, AM, Shike, DW, Hansen, SL, and McCann, JC. Effects of injectable vitamin E before or after transit on receiving phase growth performance, health, and blood parameters of beef steers. J Anim Sci. (2023) 101:101. doi: 10.1093/jas/skac333
6. Kanyar, IM, and Karadas, F. Effect of melatonin and vitamin E as antioxidants on body weight, carcass traits of Awassi lambs fed a high-energy and normal diet. Iraqi J Agric Sci. (2023) 54:1339–50. doi: 10.36103/ijas.v54i5.1835
7. Castagnino, DS, Kammes, KL, Allen, MS, Gervais, R, Chouinard, PY, and Girard, CL. High-concentrate diets based on forages harvested at different maturity stages affect ruminal synthesis of B vitamins in lactating dairy cows. Animal. (2017a) 11:608–15. doi: 10.1017/S1751731116001798
8. Zhang, Q, Yu, Z, Yang, H, and Na, RS. The effects of stage of growth and additives with or without cellulase on fermentation and in vitro degradation characteristics of Leymus chinensis silage. Grass Forage Sci. (2016) 71:595–606. doi: 10.1111/gfs.12210
9. Czurgiel, S, Antoszkiewicz, Z, Mazur-Kusnirek, M, and Bogdaszewski, M. The effect of festulolium silage-based diets on the content of tocopherols, β-carotene and retinol in meat from young rams. Animals. (2023) 13:1817. doi: 10.3390/ani13111817
10. Castagnino, DS, Kammes, KL, Allen, MS, Gervais, R, Chouinard, PY, and Girard, CL. Particle length of silages affects apparent ruminal synthesis of B vitamins in lactating dairy cows. J Dairy Sci. (2016) 99:6229–36. doi: 10.3168/jds.2016-11274
11. van den Oever, SP, Haselmann, A, Schreiner, M, Fuerst-Waltl, B, Zebeli, Q, Mayer, HK, et al. Hay versus silage: does hay feeding positively affect milk composition? Int Dairy J. (2021) 118:105024. doi: 10.1016/j.idairyj.2021.105024
12. Qi, DM, Dong, XB, Liu, H, Liu, GS, and Hou, SL. Varieties and application models of Sheepgrass. GER: Springer Nature. (2019). 65–83
13. Broderick, GA, and Kang, JH. Automated simultaneous determination of ammonia and total amino-acids in ruminal fluid and in vitro media. J Dairy Sci. (1980) 63:64–75. doi: 10.3168/jds.S0022-0302(80)82888-8
14. Cunniff, PA ed. Association of Official Analytical Chemists (AOAC). Protein (crude) in animal feed and pet food 984.13 In: Official methods of analysis of official analytical chemists international. 16th ed. Arlington, VA, USA: AOAC International (1995). 1.
15. Murphy, RP . A method for the extraction of plant samples and the determination of total soluble carbohydrates. J Sci Food Agric. (1958) 9:714–7. doi: 10.1002/jsfa.2740091104
16. Van Soest, PJ, Robertson, JB, and Lewis, BA. Methods for dietary fiber, neutral detergent fiber, and nonstarch polysaccharides in relation to animal nutrition. J Dairy Sci. (1991) 74:3583–97. doi: 10.3168/jds.S0022-0302(91)78551-2
17. Bao, JZ, Wang, L, and Yu, Z. Effects of different moisture levels and additives on the ensiling characteristics and in vitro digestibility of stylosanthes silage. Animals. (2022) 12:1555. doi: 10.3390/ani12121555
18. Jia, TT, Sun, ZQ, Gao, R, and Yu, Z. Lactic acid bacterial inoculant effects on the vitamin content of alfalfa and Chinese leymus silage. Asian-Australasian J Anim Sci. (2019) 32:1873–81. doi: 10.5713/ajas.19.0135
19. Milton, JS . Statistical methods in the biological and health sciences 2nd ed. New York: Mcgraw-Hill Inc. (1992): 346–390
21. Zhao, MM, and Yu, Z. Effects of lactic acid bacteria and cellulase on napier grass silages. Acta Agrestia Sin. (2015) 23:205–10.
22. Zhuang, YF, Anzhai, YF, and Zhang, WC. Effects of low moisture ensiling on cell wall composition and in vitro dry matter digestibility of triticale. J Gansu Agric Univ. (2006) 41:94–7.
23. Tian, QZ, Wang, G, Ma, XX, Shen, QW, Ding, ML, Yang, XY, et al. Riboflavin integrates cellular energetics and cell cycle to regulate maize seed development. Plant Biotechnol J. (2022) 20:1487–501. doi: 10.1111/pbi.13826
24. Xie, Y, Shi, D, Li, PH, and Zhang, YJ. Effects of meteorological and soil factors on the quality of Leymus chinensis. Acta Agrestia Sin. (2012) 20:631–6.
25. Ribeiro, GO, Teixeira, AM, Velasco, FO, Faria, WG, Pereiea, LGC, Chaves, AV, et al. Production, nutritional quality and in vitro methane production from Andropogon gayanus grass harvested at different maturities and preserved as hay or silage. Asian Austra J Anim. (2014) 27:330–41. doi: 10.5713/ajas.2013.13161
26. Li, JF, Yuan, XJ, Dong, ZH, Mugabe, W, and Shao, T. The effects of fibrolytic enzymes, celulolytic fungi and bacteria on the fermentation characterstics, structural carbohydrates degradation, and enzymatic conversion yields of silage. Bioresour Technol. (2018) 264:123–30. doi: 10.1016/j.biortech.2018.05.059
27. Desta, ST, Yuan, XL, Li, JF, and Shao, T. Ensiling characteristics, structural and nonstructurat carbohydrate composition and enzymatic dioestibity of napier orass ensiled with additives. Bioresour Technol. (2016) 221:447–54. doi: 10.1016/j.biortech.2016.09.068
28. Tadevosyan, L, Martirosyan, G, Tsereteli, I, Vardanyan, I, Zadayan, M, and Avagyan, A. Dynamics of bioactive substances accumulation during cauliflower maturation as a way to ensure functional crop properties. Funct Foods in Health Dis. (2023) 13:584–94. doi: 10.31989/ffhd.v13i11.1197
29. Hunt, CH, and Bethke, RM. The riboflavin content of certain hays and grasses. J Nutr. (1940) 20:175–80. doi: 10.1093/jn/20.2.175
30. Gunaydin, S, and Alibas, I. The influence of short, medium, and long duration common dehydration methods on total protein, nutrients, vitamins, and carotenoids of rosehip fruit (Rosa canina L.). J Food Compos Anal. (2023) 124:105631. doi: 10.1016/j.jfca.2023.105631
31. Albarrán, G, Mendoza, E, and Beltrán, JM. Influence of concentration on the radiolytic decomposition of thiamine, riboflavin, and pyridoxine in aqueous solution. Rev Colomb Quím. (2014) 43:35–40. doi: 10.15446/rev.colomb.quim.v43n3.53614
32. Kumar, S, and Aalbersberg, B. Nutrient retention in foods after earth-oven cooking compared to other forms of domestic cooking-2.Vitamins. J Food Compos Anal. (2006) 19:311–20. doi: 10.1016/j.jfca.2005.06.007
33. Ayhan, DK, and Koksel, H. Investigation of the effect of different storage conditions on vitamin content of enriched pasta product. Qual Assur Saf Crops. (2019) 11:701–12. doi: 10.3920/QAS2019.1575
34. Aktas-Akyildiz, E, and Koksel, H. Minimisation of vitamin losses in fortified cookies by response surface methodology and validationof the determination methods. Eur Food Res Technol. (2021) 247:1345–54. doi: 10.1007/s00217-021-03712-2
35. Schnellbaecher, A, Binder, D, Bellmaine, S, and Zimmer, A. Vitamins in cell culture media: stability and stabilization strategies. Biotechnol Bioeng. (2019) 116:1537–55. doi: 10.1002/bit.26942
36. Ischebeck, T, Zbierzak, AM, Kanwischer, M, and Dörmann, P. A salvage pathway for phytol metabolism in Arabidopsis. J Biol Chem. (2006) 281:2470–7. doi: 10.1074/jbc.M509222200
37. Sato, S, Uegaki, R, Kawamura, J, and Kimura, T. Enhancement of-tocopherol by ensiling in barley Hordeum vulgare and wheat Triticum aestivum. Grassl Sci. (2018) 64:245–51. doi: 10.1111/grs.12204
38. Antoszkiewicz, Z, Fijalkowska, M, Mazur-Kusnirek, M, Przemieniecki, S, and Purwin, C. Effect of a harvest date and cutting height on the concentrations of carotenoids and tocopherols in virginia fanpetals (sida hermaphr odita) herbage and silage. J Elem. (2019) 24:1195–202. doi: 10.5601/jelem.2019.24.2.1857
39. Vidalvalverde, C, Ruiz, R, and Medrano, A. Effects of frozen and other storage-conditions on alpha-tocopherol content of cow milk. J Dairy Sci. (1993) 76:1520–5. doi: 10.3168/jds.S0022-0302(93)77484-6
40. Hidiroglou, M, Batra, TR, and Roy, GL. Changes in plasma alpha-tocopherol and selenium of gestating cows fed hay or silage. J Dairy Sci. (1994) 77:190–5. doi: 10.3168/jds.S0022-0302(94)76941-1
41. Liu, QH, Shao, T, and Bai, YF. The effect of fibrolytic enzyme, Lactobacillus plantarum and two food antioxidants on the fermentation quality, alpha-tocopherol and beta-carotene of high moisture napier grass silage ensiled at different temperatures. Anim Feed Sci Technol. (2016) 221:1–11. doi: 10.1016/j.anifeedsci.2016.08.020
Keywords: B vitamins, α-tocopherol, silage fermentation, natural drying, Leymus chinensis
Citation: Wang Y, Chen X, Zhuo X, Wang L, Jia T, Ji F, Zhang H and Yu Z (2024) Effect of different harvest times and processing methods on the vitamin content of Leymus. Front. Nutr. 11:1424334. doi: 10.3389/fnut.2024.1424334
Edited by:
Nassim Naderi, Independent Researcher, St Hyacinthe, CanadaReviewed by:
Shuai Du, Inner Mongolia Agricultural University, ChinaRadjassegarin Arumugam, A. V. C. College, India
Mohamed El-Mogy, King Faisal University, Saudi Arabia
Copyright © 2024 Wang, Chen, Zhuo, Wang, Jia, Ji, Zhang and Yu. This is an open-access article distributed under the terms of the Creative Commons Attribution License (CC BY). The use, distribution or reproduction in other forums is permitted, provided the original author(s) and the copyright owner(s) are credited and that the original publication in this journal is cited, in accordance with accepted academic practice. No use, distribution or reproduction is permitted which does not comply with these terms.
*Correspondence: Zhu Yu, MDIwNTlAY2F1LmVkdS5jbg==