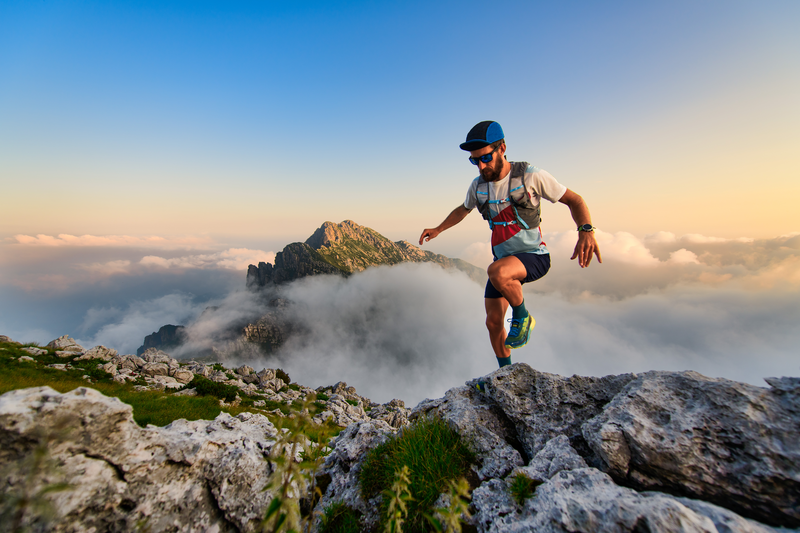
94% of researchers rate our articles as excellent or good
Learn more about the work of our research integrity team to safeguard the quality of each article we publish.
Find out more
MINI REVIEW article
Front. Nutr. , 14 June 2024
Sec. Nutrition and Metabolism
Volume 11 - 2024 | https://doi.org/10.3389/fnut.2024.1424246
This article is part of the Research Topic Unraveling the Links between Nutrients and Metabolic Dysfunction-Associated Liver Disease: Insights and Implications View all 15 articles
MAFLD has become a major global health problem and is the leading cause of liver disease worldwide. The disease progresses from a simple fatty liver to gradual fibrosis, which progresses to cirrhosis and even hepatocellular liver cancer. However, the methods currently used for diagnosis are invasive and do not facilitate clinical assessment of the condition. As a result, research on markers for the diagnosis of MAFLD is increasing. In addition, there are no clinical medications for the treatment of MAFLD, and lifestyle interventions remain effective in the prevention and treatment of MAFLD. In this review, we attempt to make a summary of the emerging diagnostic indicators and effective lifestyle interventions for MAFLD and to provide new insights into the diagnosis and treatment of MAFLD.
In 2020, the hepatology community introduced the novel term “metabolic dysfunction-associated fatty liver disease” (MAFLD) to replace “non-alcoholic fatty liver disease” (NAFLD) (1). Recently, this term was again replaced by “metabolic dysfunction-associated steatotic liver disease” (MASLD), along with a revised definition of metabolic dysfunction (2). MAFLD is a common chronic liver disease worldwide. The high prevalence of MAFLD has been noted in several reports (3–5). Overall prevalence is statistically increasing steadily and is projected to reach 55.7% by 2040 (6). MAFLD is a type of liver injury characterized by increased fatty deposits in hepatocytes with varying histological severity and the potential to progress to fibrosis, cirrhosis, and hepatocellular liver cancer. Fibrosis stage was the only independent predictor of long-term prognosis in MAFLD. Liver biopsy remains the gold standard for the diagnosis of liver fibrosis, but because it is an invasive test that is difficult to use frequently in the clinic, it has become important to find a noninvasive index for the effective assessment of MAFLD. In addition, there are no approved medications for the treatment of MAFLD, and lifestyle interventions continue to be the most recognized method of preventing and treating MAFLD.
The purpose of this review is to summarize current diagnostic indicators and effective lifestyle intervention methods for MAFLD.
Magnetic Resonance Proton Density Fat Fraction (MRI-PDFF) has the ability to quantify hepatic fat over the entire dynamic range. It is a more accurate quantitative imaging biomarker that can be used to evaluate liver fat content. In a meta-analysis (n = 6 studies in 635 patients with biopsy-proven NAFLD), the summary AUROC values of MRI-PDFF for detecting steatosis S0 and S1–S3 (≥5%), S0–S1 and S2–S3 (≥33%), S0–S2 and S3 (≥66%) were 0.98, 0.91, and 0.90, respectively. Pooled sensitivity and specificity were 93 and 94%, 74 and 90%, and 74 and 87%, respectively (7). However, due to its high cost, it is not recommended for routine clinical use.
Controlled attenuation parameter (CAP) is the algorithm available on the FibroScan system (Echosens, Paris, France) for quantification of the liver fat content. This is a convenient and readily available modality that has been widely used to assess liver fat content. However, several covariates such as NAFLD, diabetes mellitus, and BMI affect CAP values. Currently, guidelines consider values above 275 dB/m to have high sensitivity and PPV (>90%) in NAFLD, although there is no uniform cut-off value for CAP (8). One study summarized that in healthy adolescent boys/girls, the upper limit of normal for CAP is 258.9/243.1 dB/m (12–13.9 years old), 251.9/266.3 dB/m (14–15.9 years old), 247.0/265.2 dB/m (16–17.9 years old), and 249.3/246.0 dB/m (18–19.9 years old) (9). Recent studies have analyzed that in children with NAFLD, using MRI-PDFF grading as a criterion for steatosis, the optimal cutoffs for CAP in the subgroups of S0 vs. S1–S3 (≥6.4%), S0–S1 vs. S2–S3 (≥17.4%), and S0–S2 vs. S3 (≥22.1%) were 265 dB/m, 299 dB/m, and 303 dB/m, respectively (10).
Recently, a study constructed a new CAP-based scoring scale, CBST.CBST = −14.27962 + 0.05431 × CAP −0.14266 × BMI + 0.01715 × AST. Confirmed that CBST scores are more accurate than CAP itself. The optimal CBST cutoff values for MRI-PDFF diagnosis of ≥20%, ≥10%, and ≥5% were −0.5345 (sensitivity = 72.1%, specificity = 70.8%, PPV = 53.7%, NPV = 84.4%), −1.7404 (sensitivity = 88.7%, specificity = 76.0%, PPV = 91.2%, NPV = 70.4%) and −1.9959 (sensitivity = 86.4%, specificity = 92.9%, PPV = 99.4%, NPV = 35.1%) (11). This would be a practical diagnostic basis if more studies were available to verify its accuracy.
The FIB-4 and APRI is a score that uses routine laboratory parameters to assess liver fibrosis, and the formula is FIB-4 score = age(year) × AST(U/L)/[PLT(109/L) × ALT1/2(U/L)] APRI = [AST(U/L)/(AST(upper limit of normalcy)(U/L)]/PLT(109/L) × 100). It has been shown that it can be used to assess the degree of hepatic fibrosis in chronic hepatitis B patients. The sensitivity and specificity of the APRI score > 0.342 to differentiate between patients with “no and mild fibrosis” and those with “severe fibrosis” were 63 and 64%. Respectively, and the sensitivity of the FIB-4 score > 0.70 to differentiate between patients with “no and mild fibrosis” and those with “severe fibrosis” was 71%, with a sensitivity of 71% (12). It has been noted that despite the high diagnostic accuracy of FIB-4 and APRI for advanced fibrosis in patients with NAFLD, their ability to diagnose hepatic fibrosis in its early stages remains controversial (13). Furthermore, it was shown that the APRI (AUROC range 0.73–0.80) and FIB-4 (AUROC range 0.66–0.82) outperformed the NAFLD fibrosis score (NFS) (AUROC range 0.63–0.75) in predicting liver fibrosis in MAFLD (14). In a meta-analysis of more than 40,000 participants, it was noted that when used to predict the severity of liver fibrosis in patients with MASLD, FIB-4 had good predictive diagnostic accuracy for any fibrosis, and FIB-4 had good diagnostic accuracy for cirrhosis, and could be used to assess the prognosis of MASLD (15).
Detection of hepatic steatosis HS is a mandatory criterion for the diagnosis of MAFLD, and the development of a non-invasive means of rapid and standardized detection of HS has become very important. Han et al. retrospectively evaluated the performance of FLI and HSI with respect to the prediction of MAFLD diagnosed by computed tomography (CT), and FLI (AUROC, 0.793) and HSI (HSI 0.784) were feasible in the prediction of MAFLD (16). In a large cross-sectional survey in China including 135,436 patients, the FLI AUROC used to predict MAFLD for male and female patients was 0.870 and 0.923 (17). A Mexican population-based study developed a score, MAFLD-S, for the prediction of MAFLD with an AUC of 0.852, 95% CI = 0.828–0.877, an optimal cutoff of 0.548, a sensitivity of 78.8%, and a specificity of 82.8% (18). In a cross-sectional study, assessment of MAFLD-S, FLI, and HSI is a valid tool to accurately predict MAFLD in patients with inflammatory bowel disease IBD (19). Valuable tool for FLI prediction of MAFLD noted in Japanese population-based survey (20). In a cross-sectional study based on adults in Xinjiang, FLI, and HSI had good screening for MAFLD in both men and women, with FLI having the best screening ability (21). FLI and HSI have excellent discriminatory ability in predicting MAFLD in the general population, both in the general population and in individuals at metabolic risk, FLI and HIS will be effective tools for predicting and screening for MAFLD (22).
A cross-sectional study of people aged 25–75 years showed that TyG-WC, TyG-BMI were the best predictors of MAFLD, independent of age, sex, obesity or diabetes status (23). Some studies have shown that TyG-BMI has good diagnostic efficacy in identifying people at risk for MAFLD in western China. In contrast, TyG-WC had the best diagnostic performance for identifying MAFLD risk in the US population. These findings suggest the need to select the most appropriate predictive model based on regional and racial differences (24). A Korean population-based study suggests that TyG-WC, TyG-BMI are positively correlated with MAFLD risk and can be used clinically to rapidly identify patients at risk of MAFLD (25).
Chitinase-3-like protein 1 (CHI3L1, YKL-40 protein) is a glycoprotein that is abundantly expressed in liver tissues and is mainly involved in inflammation and tissue remodeling (26). It has been shown that CHI3L1 is significantly elevated in chronic liver diseases such as HBV-Related Liver Diseases, HCV-Related Liver Diseases, and hepatocellular carcinoma, and is associated with the degree of fibrosis (27–31). Studies have shown that CHI3L1 is an indicator used to assess fibrosis, and Xiaoting Huang el confirmed that serum CHI3L1 is a viable indicator for measuring liver fibrosis. The AUC for serum CHI3L1 was 0.812, p < 0.000. The Youden index was highest at a serum CHI3L1 level of 83.36 ng/mL, with a sensitivity of 88.2% and a specificity of 66.4% (32). Yanqiang Liao el demonstrated that CHI3L1 detected by chemiluminescent immunoassay (CLIA) has good diagnostic value for HBV-associated hepatocellular carcinoma (27). It has been shown that CHI3L1 is a good biomarker in MAFLD and assesses the risk of severe liver fibrosis. The AUC for CHI3L1 in the diagnosis of significant liver fibrosis was 0.716 (95% CI, 0.596, 0.836), with a corresponding optimal cutoff value of 125.315 ng/mL (33). High levels of serum CHI3L1 in patients with type 2 diabetes may indicate liver fibrosis in patients with MAFLD, CHI3L1 showed the area under the ROC curve (AUC) for detection of significant fibrosis (0.749, 95% CI, 0.668–0.829, p < 0.001), and the value of 94.89 ng/mL (sensitivity:54.4%, specificity:87.6%, p < 0.001) was the best cutoff point to predict significant liver fibrosis in T2DM-MAFLD patients (34).
LECT2 is a chemokine (35), it is mainly produced by the liver. It has been shown that LECT2 attenuates fatty changes and insulin resistance in the liver (36). Whereas insulin resistance is a key factor in the development of MAFLD, LECT2 may be involved in the development of MAFLD. It has been shown that LECT2 levels were 31.2 (20.9, 41.5) ng/mL in the group of people with NAFLD compared to those without NAFLD, which was significantly higher than in the group without NAFLD (37). It is proposed that LECT2 is highly expressed in both hepatic tissue and serum in biliary atresia (BA), suggesting that LECT2 can be used as a biomarker for BA (38). LECT2 levels are increased in patients with metabolic syndrome (MetS) and correlate with severity, suggesting that LECT2 can be used as a biomarker for MetS (39). Currently, there are experiments showing an increase in serum LECT2 concentration in children with NAFLD, which showed a diagnosis of NAFLD at a concentration of 3.76 ng/mL with a sensitivity of 90.5% and a specificity of 54.8%, suggesting that LECT2 can be used as a diagnostic biomarker for MAFLD in children (40), which has not been studied in adults with MAFLD.
Cathepsin D (CTSD) is a lysosomal enzyme involved in inflammatory responses and lipid metabolism, and previous data have shown that hepatic inflammation is associated with hepatic CTSD activity and expression (41, 42). In addition, plasma CTSD concentrations were associated with different stages of NAFLD, Significantly elevated in metabolic dysfunction-associated steatohepatitis (NASH) (43). A recent study analyzed RNA sequencing of MASLD and found higher expression of CTSD in severe MASLD compared to controls and mild disease, and validated the diagnostic role of serum CTSD in MASLD, showing that CTSD contributes to the accuracy of FIB-4 in diagnosing MASLD. The AUC of serum CTSD in predicting NASH fibrosis was 0.731 with a cutoff value of 10624.5 pg./mL (specificity 47.1%, sensitivity 93.3%) (44).
Plasminogen activator inhibitor-1 (PAI-1) is the most important inhibitors of the plasminogen/plasmin system (45). PAI-1 plays an important role in hepatic fibrogenesis (46). It has been shown that elevated plasma levels of PAI-1 correlate with the degree of hepatic steatosis (47). The study found that the PAI-1 gene was significantly down-regulated in NAFLD by bioinformatic analysis of the GEO database. Although bias may be induced by the small sample size, it suggests that PAI-1 has the potential to serve as a diagnostic marker for NAFLD (48). Recent studies have found that as liver pathology progresses toward nonalcoholic steatohepatitis (NASH), there is a corresponding increase in the level of mRNA expression of Serpine1, as well as the protein level of PAI-1. Use of Serum PAI-1 Levels as a noninvasive biomarker to identify NASH-associated fibrosis (49). However, no experiments have been performed to assess its diagnostic value in MAFLD.
Moderate fiber intake is associated with lower odds of MAFLD compared to low fiber intake (50). Healthy low-carbohydrate and low-fat diets are protective against MAFLD, while unhealthy low-fat diets have deleterious effects on MAFLD (51). A study based on a Korean population showed that a dairy-rich dietary pattern was associated with a lower risk of MASLD, The cumulative incidence of MASLD was also significantly lower when adhering to a dietary pattern rich in dairy products (52). βHB, one of the ketone, has been reported to have inhibitory effects on adipocyte lipolysis, liver fat accumulation and inflammatory responses, suggesting a possible protective effect against MAFLD (53). The ketogenic diet (KD) is a diet that is very low in carbohydrate intake, and KD can be beneficial in treating diseases such as MAFLD and NASH. Sugar deficiency markedly reduces the effect on insulin resistance, and despite the benefits of KD, precautions need to be taken regarding the nature of dietary fats, and saturated fatty acids can be replaced with polyunsaturated fatty acids (54). One study tried to assess the effects of 8 weeks of a very low-calorie ketogenic diet (VLCKD) on MASLD, and found that it significantly reduced liver stiffness, and substantially reduced WC, fat mass, systolic and diastolic blood pressure, and BMI, and effectively reduced fasting glucose, insulin, insulin resistance (measured by HOMAIR), triglycerides, total cholesterol, LDL cholesterol, HDL cholesterol, and GT. This suggests that VLCKD improves insulin sensitivity and results in elevated levels of vitamin D. VLCKD treatment also reduces low-grade inflammation (55) (Figure 1).
Figure 1. Lifestyle interventions are now a widely recognized method of prevention and treatment for MAFLD. An effective lifestyle includes a proper diet (less salt, less sugar, good quality carbohydrates, unsaturated fatty acids, vitamin D, etc.), and good habits (exercise, adequate and good quality sleep, good mood, improved gut microbiology, reduced air pollution, etc.).
The Mediterranean diet is currently the more recommended diet for the prevention of MAFLD (56). The Mediterranean diet includes plenty of vegetables, fruits, legumes, nuts and olive oil, as well as small to moderate consumption of red meat and wine, which has anti-inflammatory, antioxidant and antifibrotic components. Adherence to the Mediterranean diet, especially higher fruit intake, is associated with lower severity of MAFLD (57). Additional studies have found that the Mediterranean diet DM and the Mexican regional diet RMD are equally effective in improving MASLD symptoms, particularly steatosis, highlighting the feasibility of using regionally adapted diets to treat fatty liver. DM implementation is not feasible in all countries. Therefore, adapting the diet to the ingredients available in that country is a better option, as balanced implementation can improve the health of patients with fatty liver disease (58).
In China, three regional dietary patterns have been evaluated for their association with MAFLD: the Sichuan Basin pattern, characterized by high intakes of fish/seafood, poultry, fresh fruits and vegetables; the Yunnan-Guizhou Plateau pattern, characterized by high intakes of animal oils and salt; and the Qinghai-Tibet Plateau pattern, characterized by high intakes of coarse grains, wheat products, tubers and tea. The results showed that both the Yunnan-Guizhou Plateau dietary pattern and the Tibetan Plateau dietary pattern were positively associated with MAFLD. Studies point to an undesirable role of salt, animal oils and high carbohydrates in the progression of MAFLD, with animal oils rich in saturated fatty acids (SFA) being positively associated with MAFLD (59).
In addition, a vegetarian diet is now considered a healthy diet, and a vegetarian diet means replacing meat and fish with soy and refined carbohydrates with whole grains. Studies have shown that vegetarian diets may be associated with less pronounced liver fibrosis and that vegetarians have lower NAFLD fibrosis scores, which could mean lower cardiovascular-related mortality in the future (60). However, there has been no extensive research on its relationship with MAFLD progression, incidence or prevalence (61).
Therefore, a dietary pattern with less salt, less sugar and more vegetables and fruits has a favorable effect on the prevention of MAFLD.
In addition to daily dietary changes that are beneficial in preventing MAFLD, increasing or decreasing the intake of some dietary supplements may also be beneficial in reducing the incidence of MAFLD. Studies have shown that high dietary selenium intake increases the risk of MAFLD by modulating insulin biosynthesis and secretion dysregulation as well as stimulating glucagon secretion, insulin resistance and dyslipidemia (62). Reducing the ratio of Se and vitamin E, or not supplementing them at all, may reduce the prevalence of MAFLD (63). Patients with MAFLD consume more vitamin E in their daily diet than healthy controls, so increased vitamin E may be positively associated with MAFLD (64). Thus suggesting that to prevent MAFLD, we need to reduce se and vitamin E intake.
Patients with MAFLD consume more polyunsaturated fatty acids PUFA and iron in their daily diets than healthy controls Increased intake of these substances may be positively associated with MAFLD (64). According to an analysis of NHANES 2017–2020 data, high copper intake and moderate iron intake are associated with low odds of MAFLD (65). Therefore, we believe that there is also a relationship between copper and iron intake, and the occurrence of MAFLD. A significant association between vitamin D insufficiency and increased incidence of MAFLD suggests the potential of vitamin D as an anti-adipogenic and anti-fibrotic agent (66). A randomized controlled trial of vitamin D supplementation on serum levels of VDR, fibrotic factors and fibrotic microRNA (MiR) levels in patients with MASLD revealed for the first time significant reductions in some hepatic fibrotic factors, hepatic aminotransferases, and the corresponding changes in some fibrotic-associated MiR and some metabolic factors (67). suggests that vitamin D supplementation is beneficial in reducing the likelihood of MASLD fibrosis.
MAFLD is primarily caused by fat accumulation, and oral glutamine supplementation leads to insulin resistance in fat cells, which reduces fat (68). Glutamine was shown to contribute to attenuating the severity of hepatic lipid injury in mice exposed to high-fat diet (HFD) induced MAFLD by ameliorating changes in serum lipids, hepatic lipid metabolism, and oxidative stress (69).
Silymarin is a flavonoid compound derived from milk thistle seed that has been used for many years, as a Chinese herbal treatment for liver diseases (70). Silymarin can not only promote the production of glutathione to enhance the ability to resist oxidative stress (71), but also can inhibit the expression of NF- κ B, reduce TNF- α, IFN- γ, IL-2, and IL-4, and reduce the inflammatory response (72). In addition, silymarin can improved glucose tolerance and insulin tolerance in NAFLD patients, and the colonizing of altered microbiota from silymarin and polyherbal extract treated mice directly ameliorated NAFLD (73).
Insulin resistance is a common pathological feature in MAFLD patients, and artichoke has been found to have positive amelioration on insulin resistance. Previous studies have shown that water extract from artichoke ameliorates high-fat diet-induced non-alcoholic fatty liver disease in rats (74). Deng’s study (75) demonstrated that artichoke water extract (AWE) reduced the expression of acid enol phosphoenolpyruvate carboxykinase (PEPCK) and glucose-6-phosphatase (G6Pase), and inhibited insulin resistance to improve glucose metabolism. Meanwhile, AWE also inhibited the endoplasmic reticulum stress to protect HepG2 cells. This is thought to be the resulting from the regulation of IRS 1/PI3K/AKT/FoxO 1 and GSK-3 β signaling.
Bergamot is a citrus fruit with extensive biological activity which has anti-proliferative, pro-apoptotic, anti-inflammatory and anti-oxidation effects to make positive influence on MAFLD (76). Bergamot extract can reduce blood glucose levels in type 2 diabetic mice, prevent hyperglycemia caused by leptin receptor disruption, and regulate glucose homeostasis by enhancing insulin sensitivity. In this study, Liu et al. (77) also observed reduced TC, TG, and LDL-C levels, which effectively controlled blood lipids and protected the liver. In NAFLD, bergamot extracts can decrease the hepatic steatosis (78).
P. tenuifolia seed oil (PWSO) therapeutic diet (17% lard and 14% PWSO diet) inactivates SREBP1 and SREBP2, which are involved in lipogenesis, to attenuate hepatic lipid accumulation and reduce inflammatory responses induced through the NF-κB signaling pathway. Studies have shown that PWSO can be a relatively effective dietary supplement to inhibit the onset and progression of MAFLD (79).
We believe that moderate dietary supplements are beneficial for the prevention of MAFLD (Table 1).
Green tea is generally recognized as a healthful drink (80). Tea consumption (≥1 cup/day) is not associated with the prevalence of newly diagnosed NAFLD among the general Chinese adult population, and further research may be needed to examine the association between higher frequency tea consumption and MASLD, says a study based on the Tianjin population (81). A 2020 study suggested that green tea reduced liver enzyme levels in participants with non-alcoholic fatty liver disease (NAFLD), but liver enzymes were significantly increased in healthy subjects (82). This suggests to us that drinking more green tea may be effective in reducing the prevalence of MAFLD.
It has also been suggested that caffeine attenuates liver fat and stiffness in patients with diabetes and NAFLD (83). Coffee is associated with NAFLD severity in type 2 diabetics (84). For overweight/obese patients with MASLD and T2D, coffee consumption may have potential benefits (85). It has also been shown that excessive soft drink consumption is still significantly associated with MASLD (86).
Therefore, appropriately increasing the consumption of green tea and coffee and decreasing the consumption of soft drinks in daily life may help prevent MAFLD.
According to data from the 2003–2006 U.S. population, longer physical activity (PA) is associated with a lower risk of cardiovascular disease-related death in patients with NAFLD (87). A study based on the 2017–2018 U.S. population shows that physical activity is strongly associated with a lower risk of obese and non-obese MAFLD (88). Active physical activity PA and adequate weekday sleep duration are both inversely associated with the risk of MASLD, and combining them can further reduce the risk of MASLD (89). Increased physical activity improves the strength of the muscles in the body. Whereas muscle strength may play a critical role in the incidence and progression of NAFLD/MAFLD, interventions to improve muscle strength in the management of NAFLD/MAFLD may be helpful (90). One study showed that adherence to an overall healthy lifestyle of non-smoking, non-alcohol use, physical activity, and healthy diet was associated with a 19% reduction in adjusted MAFLD risk (91).
It has been suggested that concentrating on physical activity 1 or 2 days per week (WW model) versus an average weekly schedule of physical activity (RA model) are both associated with lower DXA measures of fat mass (both in the abdominal region and whole body), BMI, and waist circumference. The WW model may also be applicable to the prevention of NAFLD (92).
Short sleep duration and poor sleep quality are significantly associated with increased risk of NAFLD, according to a Korean population-based study (93). A study of 2,172 people in Japan found that the prevalence of NAFLD declined progressively with increasing sleep duration, with the lowest prevalence in the subgroup with 6 to ≤7 h of sleep and the highest in the groups with ≤6 and >8 h of sleep (94). A study of 708 non-diabetic adolescents found that sleep deprivation was associated with the presence of NAFLD in the younger population (95). Patients with sleep-disordered SD have higher risk of NAFLD in a Taiwanese population-based study (96). In addition, poor sleep patterns were associated with a high risk of MAFLD and severe fibrosis, and sleep difficulties, snoring, excessive daytime sleepiness, and sleep apnea symptoms were positively associated with the odds of MAFLD when specific factors of sleep patterns were examined in isolation (97). Good sleep habits, therefore, help reduce the prevalence of MAFLD.
The stress-eating relationship is mediated by the release of cortisol from the hypothalamic pituitary adrenal (HPA) axis. In a survey studying the relationship between occupational stress and NAFLD among Chinese police officers, it was found that the higher the stress, the higher the risk of developing NAFLD (98). Chronic stress linked to obesity, stress management important in treating NAFLD (99). Studies based on the Korean population have shown direct and indirect associations between psychological factors and NAFLD, depending on individual susceptibility (100). And in another study, it was also demonstrated that higher perceived stress was associated with increased prevalence of NAFLD (101). All of these studies have shown that the greater the psychological stress the greater the risk of developing NAFLD. Another recent Mendelian randomized analysis of studies points out that depression increases the prevalence of NAFLD (102). Suggesting the impact of the psychological illness on MAFLD, attention needs to be paid to managing stress and proper relaxation and stress relief in life.
Study proves gut microbes linked to NAFLD development (103). Study finds that gut microbial diversity declines in NAFLD, and modulating gut microbiota health may help overcome NAFLD (104). In addition, probiotic yogurt significantly improves metabolic disorders through modulating intestinal microflora and lipid metabolism and effectively regulating the occurrence and development of MAFLD (105). By improving the flora of the gut microbiota, it helps reduce the risk of developing MAFLD.
It has been suggested that long-term exposure to PM2.5 significantly increases the risk of metabolic disorders, which can increase lipid accumulation and loss of liver function (106). Ambient PM2.5 stems from a complex interaction of multiple emissions and chemical reactions; it is a mixture of various chemical components such as elemental carbon, organic carbon, sulfate (SO42−), nitrate (NO3−), and ammonium (NH4+), and chronic exposure to PM2.5 and its five major chemical components may increase MAFLD risk. Of these, nitrate may have the greatest impact on MAFLD (107). In addition, in a study analyzing the correlation between cirrhosis and air pollution in patients with NAFLD, based on the UK population, it was noted that long-term exposure to air pollution was associated with the risk of NAFLD and cirrhosis in the UK population (108). There was a dose-dependent relationship between different fibrosis stages and PM2.5 levels (PM2.5 levels in patients with fibrosis stages 0, 1–2, and 3–4:27.9, 28.4, and 29.3 μg/m3, respectively; trend p < 0.001). Exposure to PM2.5 is associated with advanced liver fibrosis in patients with MAFLD (109). Recently, a cross-sectional study based on Taiwanese and Hong Kong populations analyzed airborne concentrations of nitrogen dioxide (NO2) and ozone (O3) and fine particulate matter (PM2.5) in relation to advanced fibrosis in NAFLD. The results showed that higher ambient PM2.5 and NO2 were associated with higher odds of NAFLD and advanced fibrosis, and that, in addition, lowering PM2.5 and NO2 concentrations may be an effective method of preventing NAFLD, and that further research is necessary on O3 (110). From this, we concluded that reducing air pollution or actively reducing exposure to PM2.5 can effectively prevent MAFLD.
MAFLD is a liver disease with high prevalence, and currently used to diagnose MAFLD is still mainly diagnosed by imaging. This review summarizes the commonly used imaging markers (MRI-PDFF, CAP) and new evaluation markers based on the original markers (FIB-4, APRI, FLI, HSI, MAFLD-S, TyG-WC, TyG-BMI, CBST). The more studied serological markers (CHI3L1, LECT2, CTSD) are also summarized, and some serological markers in combination with imaging indices help to improve the accuracy. Combined serologic and imaging diagnosis may become an effective method for clinical diagnosis of MAFLD.
However, nowadays, indicators are generally devoted to reflecting the degree of hepatic steatosis, the degree of fibrosis, or predicting MAFLD in other metabolic disorders, and there is a lack of research on specific indicators for MAFLD, which might be useful to look for specific diagnostic indicators at the molecular level.
This review summarizes the effective methods currently used for the prevention and treatment of MAFLD in terms of diet, lifestyle habits and living environment. Dietary supplements are well suited to help in the clinic, as daily diet and lifestyle habits require a great deal of adherence from the patients themselves, and the living environment is difficult to change. Current research, based on cell and animal experiments, suggests that supplements are useful in reducing liver fat accumulation, anti-inflammatory, and antioxidant.
Current treatments targeting obesity, insulin resistance, and cardiovascular aspects are effective but lack specificity for MAFLD. This may be due to the fact that the pathomechanism of MAFLD is very complex and requires further research. It is therefore important to understand the pathogenesis of MAFLD, which may help to find drugs in the future that can both improve metabolic disorders and reduce hepatic inflammation and fibrosis, as well as provide new guidelines for finding early specific diagnostic indicators.
TC: Writing – original draft. XQ: Writing – original draft. JJ: Writing – review & editing. BH: Writing – review & editing.
The author(s) declare that financial support was received for the research, authorship, and/or publication of this article. The study is supported by the Zhejiang Provincial Natural Science Foundation of China (No. LGF22H290001) and Administration of Traditional Chinese Medicine of Zhejiang Province (No. 2023ZL419).
The authors declare that the research was conducted in the absence of any commercial or financial relationships that could be construed as a potential conflict of interest.
All claims expressed in this article are solely those of the authors and do not necessarily represent those of their affiliated organizations, or those of the publisher, the editors and the reviewers. Any product that may be evaluated in this article, or claim that may be made by its manufacturer, is not guaranteed or endorsed by the publisher.
1. Eslam, M, Sanyal, AJ, and George, J. MAFLD: a consensus-driven proposed nomenclature for metabolic associated fatty liver disease. Gastroenterology. (2020) 158:1999–2014.e1. doi: 10.1053/j.gastro.2019.11.312
2. Bajo, FR, and Shipman, KE. Reclassification of nonalcoholic fatty liver disease: a multi-society Delphi consensus statement. Clin Exp Dermatol. (2023) 48:1418–21. doi: 10.1093/ced/llad283
3. Theofilis, P, Vordoni, A, and Kalaitzidis, RG. Metabolic dysfunction-associated fatty liver disease in the National Health and nutrition examination survey 2017-2020: epidemiology, clinical correlates, and the role of diagnostic scores. Meta. (2022) 12:1070. doi: 10.3390/metabo12111070
4. Lee, H, Lee, Y-H, Kim, SU, and Kim, HC. Metabolic dysfunction-associated fatty liver disease and incident cardiovascular disease risk: a Nationwide cohort study. Clin Gastroenterol Hepatol. (2021) 19:2138–2147.e10. doi: 10.1016/j.cgh.2020.12.022
5. Ciardullo, S, and Perseghin, G. Prevalence of NAFLD, MAFLD and associated advanced fibrosis in the contemporary United States population. Liver Int. (2021) 41:1290–3. doi: 10.1111/liv.14828
6. Le, MH, Yeo, YH, Zou, B, Barnet, S, Henry, L, Cheung, R, et al. Forecasted 2040 global prevalence of nonalcoholic fatty liver disease using hierarchical bayesian approach. Clin Mol Hepatol. (2022) 28:841–50. doi: 10.3350/cmh.2022.0239
7. Gu, J, Liu, S, Du, S, Zhang, Q, Xiao, J, Dong, Q, et al. Diagnostic value of MRI-PDFF for hepatic steatosis in patients with non-alcoholic fatty liver disease: a meta-analysis. Eur Radiol. (2019) 29:3564–73. doi: 10.1007/s00330-019-06072-4
8. European Association for the Study of the Liver . EASL clinical practice guidelines on non-invasive tests for evaluation of liver disease severity and prognosis - 2021 update. J Hepatol. (2021) 75:659–89. doi: 10.1016/j.jhep.2021.05.025
9. Ramírez-Vélez, R, García-Hermoso, A, Correa-Rodríguez, M, and Izquierdo, M. Defining values for controlled attenuation parameter and liver stiffness in youth without liver disease. Pediatr Res. (2022) 91:912–20. doi: 10.1038/s41390-021-01441-6
10. Peng, T, Yi, X, Lin, Y, Dong, X, Zhang, P, Qiao, Z, et al. Controlled attenuation parameter (CAP): the clinical value based on MRI-PDFF in children with obesity. J Pediatr Endocrinol Metab. (2024). doi: 10.1515/jpem-2023-0566. [E-pub ahead of print].
11. An, Z-M, Liu, Q-H, Ye, X-J, Zhang, Q, Pei, H-F, Xin, X, et al. A novel score based on controlled attenuation parameter accurately predicts hepatic steatosis in individuals with metabolic dysfunction associated Steatotic liver disease: a derivation and independent validation study. Clin Transl Gastroenterol. (2024) 15:e00680. doi: 10.14309/ctg.0000000000000680
12. Gür-Altunay, D, and Yürük-Atasoy, P. How successful are APRI and FIB-4 scores in predicting liver fibrosis in chronic hepatitis B patients? Infect Dis Clin Microbiol. (2023) 5:332–40. doi: 10.36519/idcm.2023.276
13. Xiao, G, Zhu, S, Xiao, X, Yan, L, Yang, J, and Wu, G. Comparison of laboratory tests, ultrasound, or magnetic resonance elastography to detect fibrosis in patients with nonalcoholic fatty liver disease: a meta-analysis. Hepatology. (2017) 66:1486–501. doi: 10.1002/hep.29302
14. Huang, C-F, Liang, P-C, Wang, C-W, Jang, T-Y, Hsu, P-Y, Tsai, P-C, et al. Performance of noninvasive seromarkers in predicting liver fibrosis among MAFLD patients with or without viral hepatitis. Kaohsiung J Med Sci. (2024) 40:374–83. doi: 10.1002/kjm2.12804
15. López Tórrez, SM, Ayala, CO, Ruggiro, PB, Costa, CAD, Wagner, MB, Padoin, AV, et al. Accuracy of prognostic serological biomarkers in predicting liver fibrosis severity in people with metabolic dysfunction-associated steatotic liver disease: a meta-analysis of over 40,000 participants. Front Nutr. (2024) 11:1284509. doi: 10.3389/fnut.2024.1284509
16. Han, AL, and Lee, HK. Comparison of the diagnostic performance of steatosis indices for discrimination of CT-diagnosed metabolic dysfunction-associated fatty liver disease. Meta. (2022) 12:664. doi: 10.3390/metabo12070664
17. Xu, Z, Li, H, Tian, S, Wu, J, Li, X, Liu, Z-L, et al. Blood biomarkers for the diagnosis of hepatic steatosis in metabolic dysfunction-associated fatty liver disease. J Hepatol. (2020) 73:1264–5. doi: 10.1016/j.jhep.2020.06.003
18. Ruiz-Manriquez, J, Olivas-Martinez, A, Chávez-García, LC, Fernández-Ramírez, A, Moctezuma-Velazquez, C, Kauffman-Ortega, E, et al. Prevalence of metabolic-associated fatty liver disease in Mexico and development of a screening tool: the MAFLD-S score. Gastro Hep Adv. (2022) 1:352–8. doi: 10.1016/j.gastha.2021.12.011
19. Capela, TL, Silva, VM, Freitas, M, Arieira, C, Gonçalves, TC, de Castro, FD, et al. Identifying inflammatory bowel disease patients at risk of metabolic dysfunction-associated fatty liver disease: usefulness of non-invasive steatosis predictive scores. BMC Gastroenterol. (2023) 23:437. doi: 10.1186/s12876-023-02988-w
20. Moriyama, K . Prediction and validation of metabolic dysfunction-associated fatty liver disease using fatty liver-related indices in a Japanese population. Metab Syndr Relat Disord. (2024) 22:190–8. doi: 10.1089/met.2023.0212
21. Guo, Y, Hu, Y, Yang, J, Ma, R, Zhang, X, Guo, H, et al. Validation of non-invasive indicators in the screening of metabolic dysfunction-associated fatty liver disease: a cross-sectional study among Uighurs in rural Xinjiang. Eur J Med Res. (2023) 28:555. doi: 10.1186/s40001-023-01536-2
22. Okada, A, Yamada, G, Kimura, T, Hagiwara, Y, Yamaguchi, S, Kurakawa, KI, et al. Diagnostic ability using fatty liver and metabolic markers for metabolic-associated fatty liver disease stratified by metabolic/glycemic abnormalities. J Diab Invest. (2023) 14:463–78. doi: 10.1111/jdi.13966
23. Khamseh, ME, Malek, M, Jahangiri, S, Nobarani, S, Hekmatdoost, A, Salavatizadeh, M, et al. Insulin resistance/sensitivity measures as screening indicators of metabolic-associated fatty liver disease and liver fibrosis. Dig Dis Sci. (2024) 69:1430–43. doi: 10.1007/s10620-024-08309-9
24. Zou, H, Ma, X, Zhang, F, and Xie, Y. Comparison of the diagnostic performance of twelve noninvasive scores of metabolic dysfunction-associated fatty liver disease. Lipids Health Dis. (2023) 22:145. doi: 10.1186/s12944-023-01902-3
25. Kim, AH, Son, D-H, and Lee, Y-J. Modified triglyceride-glucose index indices are reliable markers for predicting risk of metabolic dysfunction-associated fatty liver disease: a cross-sectional study. Front Endocrinol (Lausanne). (2023) 14:1308265. doi: 10.3389/fendo.2023.1308265
26. Huang, H, Wu, T, Mao, J, Fang, Y, Zhang, J, Wu, L, et al. CHI3L1 is a liver-enriched, noninvasive biomarker that can be used to stage and diagnose substantial hepatic fibrosis. OMICS J Integr Biol. (2015) 19:339–45. doi: 10.1089/omi.2015.0037
27. Liao, Y, Peng, S, Huang, L, Li, Z, Hu, J, Xu, R, et al. Analytical and clinical evaluation of a Chemiluminescent immunoassay to detect serum Chitinase-3-like protein 1 in HBV-related liver diseases. Int J Anal Chem. (2024) 2024:6688819–7. doi: 10.1155/2024/6688819
28. Wang, S, Chen, S, Jin, M, Hu, M, Huang, W, Jiang, Z, et al. Diagnostic and prognostic value of serum Chitinase 3-like protein 1 in hepatocellular carcinoma. J Clin Lab Anal. (2022) 36:e24234. doi: 10.1002/jcla.24234
29. Kang, Q, Liu, JX, Tan, N, Chen, HY, Pan, JL, Han, YF, et al. Diagnostic value of novel hepatic fibrosis markers in assessing cirrhosis in patients with chronic hepatitis C. Zhonghua Gan Zang Bing Za Zhi. (2023) 31:56–64. doi: 10.3760/cma.j.cn501113-20220329-00149
30. Qiu, H, and Zhang, X. The value of serum CHI3L1 for the diagnosis of chronic liver diseases. Int J Gen Med. (2022) 15:5835–41. doi: 10.2147/IJGM.S364602
31. Nishimura, N, De Battista, D, McGivern, DR, Engle, RE, Tice, A, Fares-Gusmao, R, et al. Chitinase 3-like 1 is a profibrogenic factor overexpressed in the aging liver and in patients with liver cirrhosis. Proc Natl Acad Sci USA. (2021) 118:e2019633118. doi: 10.1073/pnas.2019633118
32. Bao, J, Ouyang, Y, Qiao, L, He, J, Liu, F, Wang, Y, et al. Serum CHI3L1 as a biomarker for non-invasive diagnosis of liver fibrosis. Discov Med. (2022) 33:41–9.
33. Zhang, F, Han, Y, Zheng, L, Liu, J, Wu, Y, Bao, Z, et al. Association of non-invasive markers with significant fibrosis in patients with nonalcoholic fatty liver disease: a cross-sectional study. Diab Metab Syndr Obes. (2023) 16:2255–68. doi: 10.2147/DMSO.S417754
34. Zhang, F, Han, Y, Zheng, L, Bao, Z, Liu, L, and Li, W. Association between chitinase-3-like protein 1 and metabolic-associated fatty liver disease in patients with type 2 diabetes mellitus. Ir J Med Sci. (2024). doi: 10.1007/s11845-024-03671-z. [E-pub ahead of print].
35. Yamagoe, S, Yamakawa, Y, Matsuo, Y, Minowada, J, Mizuno, S, and Suzuki, K. Purification and primary amino acid sequence of a novel neutrophil chemotactic factor LECT2. Immunol Lett. (1996) 52:9–13. doi: 10.1016/0165-2478(96)02572-2
36. Hwang, HJ, Jung, TW, Kim, BH, Hong, HC, Seo, JA, Kim, SG, et al. A dipeptidyl peptidase-IV inhibitor improves hepatic steatosis and insulin resistance by AMPK-dependent and JNK-dependent inhibition of LECT2 expression. Biochem Pharmacol. 98:157–66. doi: 10.1016/j.bcp.2015.08.098
37. Yoo, HJ, Hwang, SY, Choi, J-H, Lee, HJ, Chung, HS, Seo, J-A, et al. Association of leukocyte cell-derived chemotaxin 2 (LECT2) with NAFLD, metabolic syndrome, and atherosclerosis. PLoS One. (2017) 12:e0174717. doi: 10.1371/journal.pone.0174717
38. Zhao, J, Xu, X, Gou, Q, Zheng, Q, Ge, L, Chen, L, et al. TGF-β1-mediated leukocyte cell-derived Chemotaxin 2 is associated with liver fibrosis in biliary atresia. Front Pediatr. (2022) 10:901888. doi: 10.3389/fped.2022.901888
39. Zheng, X, Lu, J, Xiang, S, Zou, P, Chen, H, Liu, J, et al. Elevated serum levels of leukocyte cell-derived chemotaxin 2 are associated with the prevalence of metabolic syndrome. Acta Diabetol. (2024) 61:643–55. doi: 10.1007/s00592-024-02242-z
40. Paine-Cabrera, D, Harvey, LK, Robarts, DR, Pritchard, MT, Thyfault, J, Weinman, SA, et al. Leukocyte cell-derived chemotaxin 2 correlates with pediatric non-alcoholic fatty liver disease. Clin Transl Sci. (2023) 16:2719–28. doi: 10.1111/cts.13666
41. Fukuo, Y, Yamashina, S, Sonoue, H, Arakawa, A, Nakadera, E, Aoyama, T, et al. Abnormality of autophagic function and cathepsin expression in the liver from patients with non-alcoholic fatty liver disease. Hepatol Res. (2014) 44:1026–36. doi: 10.1111/hepr.12282
42. Khurana, P, Yadati, T, Goyal, S, Dolas, A, Houben, T, Oligschlaeger, Y, et al. Inhibiting extracellular Cathepsin D reduces hepatic steatosis in Sprague−Dawley rats (†). Biomol Ther. (2019) 9:171. doi: 10.3390/biom9050171
43. Walenbergh, SMA, Houben, T, Rensen, SS, Bieghs, V, Hendrikx, T, van Gorp, PJ, et al. Plasma cathepsin D correlates with histological classifications of fatty liver disease in adults and responds to intervention. Sci Rep. (2016) 6:38278. doi: 10.1038/srep38278
44. Meroni, M, De Caro, E, Chiappori, F, Longo, M, Paolini, E, Mosca, E, et al. Hepatic and adipose tissue transcriptome analysis highlights a commonly deregulated autophagic pathway in severe MASLD. Obesity (Silver Spring). (2024) 32:923–37. doi: 10.1002/oby.23996
45. Van De Craen, B, Declerck, PJ, and Gils, A. The biochemistry, physiology and pathological roles of PAI-1 and the requirements for PAI-1 inhibition in vivo. Thromb Res. (2012) 130:576–85. doi: 10.1016/j.thromres.2012.06.023
46. Ghosh, AK, and Vaughan, DE. PAI-1 in tissue fibrosis. J Cell Physiol. (2012) 227:493–507. doi: 10.1002/jcp.22783
47. Barbato, A, Iacone, R, Tarantino, G, Russo, O, Sorrentino, P, Avallone, S, et al. Relationships of PAI-1 levels to central obesity and liver steatosis in a sample of adult male population in southern Italy. Intern Emerg Med. (2009) 4:315–23. doi: 10.1007/s11739-009-0240-9
48. Meng, Q, Li, X, and Xiong, X. Identification of hub genes associated with non-alcoholic Steatohepatitis using integrated bioinformatics analysis. Front Genet. (2022) 13:872518. doi: 10.3389/fgene.2022.872518
49. Zhao, Y, Yakufu, M, Ma, C, Wang, B, Yang, J, and Hu, J. Transcriptomics reveal a molecular signature in the progression of nonalcoholic steatohepatitis and identifies PAI-1 and MMP-9 as biomarkers in in vivo and in vitro studies. Mol Med Rep. (2024) 29:15. doi: 10.3892/mmr.2023.13138
50. Liu, Z, and Fang, T. Association between dietary carbohydrate to fiber ratio and metabolic dysfunction associated fatty liver disease in adults: evidence from the NHANES 2017-2020. J Health Popul Nutr. (2024) 43:43. doi: 10.1186/s41043-024-00543-1
51. Hu, C, Huang, R, Li, R, Ning, N, He, Y, Zhang, J, et al. Low-carbohydrate and low-fat diet with metabolic-dysfunction-associated fatty liver disease. Nutrients. (2023) 15:4763. doi: 10.3390/nu15224763
52. Lee, JH, Lee, HS, Jeon, S, Lee, J-H, and Kwon, Y-J. Association between dairy-rich dietary pattern and metabolic dysfunction-associated steatotic liver disease: findings from the Korean genome and epidemiology study. Dig Liver Dis. (2024) S1590-8658:00247–0. doi: 10.1016/j.dld.2024.01.200
53. Bae, J, and Lee, B-W. Association between impaired Ketogenesis and metabolic-associated fatty liver disease. Biomol Ther. (2023) 13:1506. doi: 10.3390/biom13101506
54. Ravaut, G, Carneiro, A, and Mounier, C. Exploring the impacts of ketogenic diet on reversible hepatic steatosis: initial analysis in male mice. Front Nutr. (2024) 11:1290540. doi: 10.3389/fnut.2024.1290540
55. De Nucci, S, Bonfiglio, C, Donvito, R, Di Chito, M, Cerabino, N, Rinaldi, R, et al. Effects of an eight week very low-calorie ketogenic diet (VLCKD) on white blood cell and platelet counts in relation to metabolic dysfunction-associated Steatotic liver disease (MASLD) in subjects with overweight and obesity. Nutrients. (2023) 15:4468. doi: 10.3390/nu15204468
56. Xiao, Y, Zhang, X, Yi, D, Qiu, F, Wu, L, Tang, Y, et al. Mediterranean diet affects the metabolic outcome of metabolic dysfunction-associated fatty liver disease. Front Nutr. (2023) 10:1225946. doi: 10.3389/fnut.2023.1225946
57. Mokhtare, M, Abdi, A, Sadeghian, AM, Sotoudeheian, M, Namazi, A, and Khalighi, SM. Investigation about the correlation between the severity of metabolic-associated fatty liver disease and adherence to the Mediterranean diet. Clin Nutr ESPEN. (2023) 58:221–7. doi: 10.1016/j.clnesp.2023.10.001
58. Cano Contreras, AD, García Carvajal, M, Martínez Pérez, GP, Ortiz Lorenzo, AA, Francisco, MDR, Ordaz Álvarez, H, et al. Efficacy of the regional Mexican diet versus the Mediterranean diet in patients with MASLD: a 24-week non-inferiority trial. Rev Esp Enferm Dig. (2024). doi: 10.17235/reed.2024.10392/2024. [E-pub ahead of print].
59. Zhu, X, Qucuo, N, Zhang, N, Tang, D, Hu, Y, Xie, X, et al. Dietary patterns and metabolic dysfunction-associated fatty liver disease in China’s multi-ethnic regions. J Health Popul Nutr. (2023) 42:141. doi: 10.1186/s41043-023-00485-0
60. Chiu, TH, Lin, M-N, Pan, W-H, Chen, Y-C, and Lin, C-L. Vegetarian diet, food substitution, and nonalcoholic fatty liver. Tzu Chi Med J. (2018) 30:102. doi: 10.4103/tcmj.tcmj_109_17
61. Moss, K, Gitman, V, Pinto Sanchez, MI, Oczkowski, S, Armstrong, D, Jayakumar, S, et al. Evidence related to a vegetarian diet and metabolic dysfunction-associated steatotic liver disease: protocol for a scoping review. BMJ Open. (2024) 14:e079750. doi: 10.1136/bmjopen-2023-079750
62. Steinbrenner, H, Speckmann, B, Pinto, A, and Sies, H. High selenium intake and increased diabetes risk: experimental evidence for interplay between selenium and carbohydrate metabolism. J Clin Biochem Nutr. (2011) 48:40–5. doi: 10.3164/jcbn.11-002FR
63. Guo, P, and Yu, J. Association of multiple serum minerals and vitamins with metabolic dysfunction-associated fatty liver disease in US adults: National Health and nutrition examination survey 2017-2018. Front Nutr. (2024) 11:1335831. doi: 10.3389/fnut.2024.1335831
64. Niu, Z, Liu, J, Peng, H, Wu, X, Zheng, X, Yao, S, et al. Dietary composition and its association with metabolic dysfunction-associated fatty liver disease among Chinese adults: a cross-sectional study. Arab J Gastroenterol. (2024) 25:205–13. doi: 10.1016/j.ajg.2024.02.003
65. Hou, J-Z, Wu, Q-W, and Zhang, L. Association between micronutrients intake and metabolic-associated fatty liver disease: a cross-sectional study based on the National Health and nutrition examination survey. J Nutr Sci. (2023) 12:e117. doi: 10.1017/jns.2023.99
66. Lee, S-B, Jin, MH, and Yoon, J-H. The contribution of vitamin D insufficiency to the onset of steatotic liver disease among individuals with metabolic dysfunction. Sci Rep. (2024) 14:6714. doi: 10.1038/s41598-024-57380-9
67. Ebrahimpour-Koujan, S, Sohrabpour, AA, Giovannucci, E, Vatannejad, A, and Esmaillzadeh, A. Effects of vitamin D supplementation on liver fibrogenic factors, vitamin D receptor and liver fibrogenic microRNAs in metabolic dysfunction-associated steatotic liver disease (MASLD) patients: an exploratory randomized clinical trial. Nutr J. (2024) 23:24. doi: 10.1186/s12937-024-00911-x
68. Abboud, KY, Reis, SK, Martelli, ME, Zordão, OP, Tannihão, F, de Souza, AZZ, et al. Oral glutamine supplementation reduces obesity, pro-inflammatory markers, and improves insulin sensitivity in DIO Wistar rats and reduces waist circumference in overweight and obese humans. Nutrients. (2019) 11:536. doi: 10.3390/nu11030536
69. Zhang, Y, Wang, Y, Liao, X, Liu, T, Yang, F, Yang, K, et al. Glutamine prevents high-fat diet-induced hepatic lipid accumulation in mice by modulating lipolysis and oxidative stress. Nutr Metab (Lond). (2024) 21:12. doi: 10.1186/s12986-024-00784-1
70. Mastron, JK, Siveen, KS, Sethi, G, and Bishayee, A. Silymarin and hepatocellular carcinoma: a systematic, comprehensive, and critical review. Anti-Cancer Drugs. (2015) 26:475–86. doi: 10.1097/CAD.0000000000000211
71. Sozen, H, Celik, OI, Cetin, ES, Yilmaz, N, Aksozek, A, Topal, Y, et al. Evaluation of the protective effect of silibinin in rats with liver damage caused by itraconazole. Cell Biochem Biophys. (2015) 71:1215–23. doi: 10.1007/s12013-014-0331-8
72. Perez-Araluce, M, Jüngst, T, Sanmartin, C, Prosper, F, Plano, D, and Mazo, MM. Biomaterials-based antioxidant strategies for the treatment of oxidative stress diseases. Biomimetics (Basel). (2024) 9:23. doi: 10.3390/biomimetics9010023
73. Wang, X, Jin, Y, Di, C, Zeng, Y, Zhou, Y, Chen, Y, et al. Supplementation of Silymarin alone or in combination with Salvianolic acids B and Puerarin regulates gut microbiota and its metabolism to improve high-fat diet-induced NAFLD in mice. Nutrients. (2024) 16:1169. doi: 10.3390/nu16081169
74. Deng, A, Liu, F, Tang, X, Wang, Y, Xie, P, Yang, Q, et al. Water extract from artichoke ameliorates high-fat diet-induced non-alcoholic fatty liver disease in rats. BMC Complement Med Ther. (2022) 22:308. doi: 10.1186/s12906-022-03794-9
75. Deng, A, Wang, Y, Huang, K, Xie, P, Mo, P, Liu, F, et al. Artichoke (Cynara scolymus L.) water extract alleviates palmitate-induced insulin resistance in HepG2 hepatocytes via the activation of IRS1/PI3K/AKT/FoxO1 and GSK-3β signaling pathway. BMC Complement Med Ther. (2023) 23:460. doi: 10.1186/s12906-023-04275-3
76. Adorisio, S, Muscari, I, Fierabracci, A, Thi Thuy, T, Marchetti, MC, Ayroldi, E, et al. Biological effects of bergamot and its potential therapeutic use as an anti-inflammatory, antioxidant, and anticancer agent. Pharm Biol. (2023) 61:639–46. doi: 10.1080/13880209.2023.2197010
77. Liu, H, Liang, J, Liang, C, Liang, G, Lai, J, Zhang, R, et al. Physicochemical properties of dietary fiber of bergamot and its effect on diabetic mice. Front Nutr. (2022) 9:1040825. doi: 10.3389/fnut.2022.1040825
78. Maurotti, S, Pujia, R, Ferro, Y, Mare, R, Russo, R, Coppola, A, et al. A nutraceutical with Citrus bergamia and Cynara cardunculus improves endothelial function in adults with non-alcoholic fatty liver disease. Nutrition. (2024) 118:112294. doi: 10.1016/j.nut.2023.112294
79. Xin, M, Wang, H, Wang, M, Yang, B, Liang, S, Xu, X, et al. Attenuating effect of Polygala tenuifolia Willd. Seed oil on progression of MAFLD. Front Pharmacol. (2023) 14:1253715. doi: 10.3389/fphar.2023.1253715
80. Suzuki, Y, Miyoshi, N, and Isemura, M. Health-promoting effects of green tea. Proc Japan Acad Ser B. (2012) 88:88–101. doi: 10.2183/pjab.88.88
81. Xia, Y, Wang, X, Zhang, S, Zhang, Q, Liu, L, Meng, G, et al. Daily tea drinking is not associated with newly diagnosed non-alcoholic fatty liver disease in Chinese adults: the Tianjin chronic low-grade systemic inflammation and health cohort study. Nutr J. (2019) 18:71. doi: 10.1186/s12937-019-0502-y
82. Mahmoodi, M, Hosseini, R, Kazemi, A, Ofori-Asenso, R, Mazidi, M, and Mazloomi, SM. Effects of green tea or green tea catechin on liver enzymes in healthy individuals and people with nonalcoholic fatty liver disease: a systematic review and meta-analysis of randomized clinical trials. Phytother Res. (2020) 34:1587–98. doi: 10.1002/ptr.6637
83. Mansour, A, Mohajeri-Tehrani, MR, Samadi, M, Qorbani, M, Merat, S, Adibi, H, et al. Effects of supplementation with main coffee components including caffeine and/or chlorogenic acid on hepatic, metabolic, and inflammatory indices in patients with non-alcoholic fatty liver disease and type 2 diabetes: a randomized, double-blind, placebo-controlled, clinical trial. Nutr J. (2021) 20:35. doi: 10.1186/s12937-021-00694-5
84. Coelho, M, Patarrão, RS, Sousa-Lima, I, Ribeiro, RT, Meneses, MJ, Andrade, R, et al. Increased intake of both caffeine and non-caffeine coffee components is associated with reduced NAFLD severity in subjects with type 2 diabetes. Nutrients. (2023) 15:4. doi: 10.3390/nu15010004
85. Kaur, M, Murugesan, S, Singh, S, Uy, KN, Kaur, J, Mann, N, et al. The influence of coffee on reducing metabolic dysfunction-associated Steatotic liver disease in patients with type 2 diabetes: a review. Cureus. (2023) 15:e50118. doi: 10.7759/cureus.50118
86. Wu, Y, Tan, Z, Zhen, J, Liu, C, Zhang, J, Liao, F, et al. Association between diet soft drink consumption and metabolic dysfunction-associated steatotic liver disease: findings from the NHANES. BMC Public Health. (2023) 23:2286. doi: 10.1186/s12889-023-17223-0
87. Kim, D, Murag, S, Cholankeril, G, Cheung, A, Harrison, SA, Younossi, ZM, et al. Physical activity, measured objectively, is associated with lower mortality in patients with nonalcoholic fatty liver disease. Clin Gastroenterol Hepatol. (2021) 19:1240–1247.e5. doi: 10.1016/j.cgh.2020.07.023
88. Wang, S, Xia, BX, Luo, T, and Wang, P. Association between physical activity and diet quality of obese and non-obese MAFLD. Nutr Metab Cardiovasc Dis. (2024) 34:75–89. doi: 10.1016/j.numecd.2023.07.022
89. Li, Y, Guo, Y, and Tan, S. Independent and joint association of physical activity and adequate weekday sleep duration with metabolic dysfunction-associated steatotic liver disease. Clin Res Hepatol Gastroenterol. (2024) 48:102320. doi: 10.1016/j.clinre.2024.102320
90. Hao, X-Y, Zhang, K, Huang, X-Y, Yang, F, and Sun, S-Y. Muscle strength and non-alcoholic fatty liver disease/metabolic-associated fatty liver disease. World J Gastroenterol. (2024) 30:636–43. doi: 10.3748/wjg.v30.i7.636
91. Chang, Q, Zhang, Y, Zhang, T, Liu, Z, Cao, L, Zhang, Q, et al. Healthy lifestyle and the risk of metabolic dysfunction-associated fatty liver disease: a large prospective cohort study. Diabetes Metab J. (2024). doi: 10.4093/dmj.2023.0133. [E-pub ahead of print].
92. Lei, L, Li, J, Wang, W, Yu, Y, Pu, B, Peng, Y, et al. The associations of “weekend warrior” and regularly active physical activity with abdominal and general adiposity in US adults. Obesity (Silver Spring). (2024) 32:822–33. doi: 10.1002/oby.23986
93. Kim, C-W, Yun, KE, Jung, H-S, Chang, Y, Choi, E-S, Kwon, M-J, et al. Sleep duration and quality in relation to non-alcoholic fatty liver disease in middle-aged workers and their spouses. J Hepatol. (2013) 59:351–7. doi: 10.1016/j.jhep.2013.03.035
94. Imaizumi, H, Takahashi, A, Tanji, N, Abe, K, Sato, Y, Anzai, Y, et al. The association between sleep duration and non-alcoholic fatty liver disease among Japanese men and women. Obes Facts. (2015) 8:234–42. doi: 10.1159/000436997
95. Trovato, FM, Martines, GF, Brischetto, D, Catalano, D, Musumeci, G, and Trovato, GM. Fatty liver disease and lifestyle in youngsters: diet, food intake frequency, exercise, sleep shortage and fashion. Liver Int. (2016) 36:427–33. doi: 10.1111/liv.12957
96. Wei, Y-T, Lee, P-Y, Lin, C-Y, Chen, H-J, Lin, C-C, Wu, J-S, et al. Non-alcoholic fatty liver disease among patients with sleep disorders: a Nationwide study of Taiwan. BMC Gastroenterol. (2020) 20:32. doi: 10.1186/s12876-020-1178-7
97. Li, Y, and Tan, S. Sleep factors were associated with a higher risk of MAFLD and significant fibrosis. Sleep Breath. (2024). doi: 10.1007/s11325-024-03017-0. [E-pub ahead of print].
98. Li, C, Xing, J-J, Shan, A-Q, Leng, L, Liu, J-C, Yue, S, et al. Increased risk of nonalcoholic fatty liver disease with occupational stress in Chinese policemen: a 4-year cohort study. Medicine (Baltimore). (2016) 95:e5359. doi: 10.1097/MD.0000000000005359
99. Stewart, KE, and Levenson, JL. Psychological and psychiatric aspects of treatment of obesity and nonalcoholic fatty liver disease. Clin Liver Dis. (2012) 16:615–29. doi: 10.1016/j.cld.2012.05.007
100. Han, AL . Association between non-alcoholic fatty liver disease and dietary habits, stress, and health-related quality of life in Korean adults. Nutrients. (2020) 12:1555. doi: 10.3390/nu12061555
101. Kang, D, Zhao, D, Ryu, S, Guallar, E, Cho, J, Lazo, M, et al. Perceived stress and non-alcoholic fatty liver disease in apparently healthy men and women. Sci Rep. (2020) 10:38. doi: 10.1038/s41598-019-57036-z
102. Kong, Y, Yao, Z, Ren, L, Zhou, L, Zhao, J, Qian, Y, et al. Depression and hepatobiliary diseases: a bidirectional Mendelian randomization study. Front Psych. (2024) 15:1366509. doi: 10.3389/fpsyt.2024.1366509
103. Leung, C, Rivera, L, Furness, JB, and Angus, PW. The role of the gut microbiota in NAFLD. Nat Rev Gastroenterol Hepatol. (2016) 13:412–25. doi: 10.1038/nrgastro.2016.85
104. Yang, K, Zeng, J, Wu, H, Liu, H, Ding, Z, Liang, W, et al. Nonalcoholic fatty liver disease: changes in gut microbiota and blood lipids. J Clin Transl Hepatol. (2024) 12:333–45. doi: 10.14218/JCTH.2023.00199
105. Zhu, L, Ying, N, Hao, L, Fu, A, Ding, Q, Cao, F, et al. Probiotic yogurt regulates gut microbiota homeostasis and alleviates hepatic steatosis and liver injury induced by high-fat diet in golden hamsters. Food Sci Nutr. (2024) 12:2488–501. doi: 10.1002/fsn3.3930
106. Xu, M-X, Ge, C-X, Qin, Y-T, Gu, T-T, Lou, D-S, Li, Q, et al. Prolonged PM2.5 exposure elevates risk of oxidative stress-driven nonalcoholic fatty liver disease by triggering increase of dyslipidemia. Free Radic Biol Med. (2019) 130:542–56. doi: 10.1016/j.freeradbiomed.2018.11.016
107. Guo, B, Huang, S, Li, S, Han, X, Lin, H, Li, Y, et al. Long-term exposure to ambient PM2.5 and its constituents is associated with MAFLD. JHEP Rep. (2023) 5:100912. doi: 10.1016/j.jhepr.2023.100912
108. Li, F-R, Liao, J, Zhu, B, Li, X, Cheng, Z, Jin, C, et al. Long-term exposure to air pollution and incident non-alcoholic fatty liver disease and cirrhosis: a cohort study. Liver Int. (2023) 43:299–307. doi: 10.1111/liv.15416
109. Jang, T-Y, Ho, C-C, Liang, P-C, Wu, C-D, Wei, Y-J, Tsai, P-C, et al. Air pollution associate with advanced hepatic fibrosis among patients with chronic liver disease. Kaohsiung J Med Sci. (2024) 40:304–14. doi: 10.1002/kjm2.12781
Keywords: metabolic dysfunction-associated fatty liver disease, biomarker, lifestyle interventions, diet, activity
Citation: Chen T, Qin X, Jiang J and He B (2024) Diagnostic indicators and lifestyle interventions of metabolic-associated fatty liver disease. Front. Nutr. 11:1424246. doi: 10.3389/fnut.2024.1424246
Received: 27 April 2024; Accepted: 05 June 2024;
Published: 14 June 2024.
Edited by:
Claudia Tovar-Palacio, National Institute of Medical Sciences and Nutrition Salvador Zubirán, MexicoReviewed by:
Elisa Mazza, Magna Græcia University, ItalyCopyright © 2024 Chen, Qin, Jiang and He. This is an open-access article distributed under the terms of the Creative Commons Attribution License (CC BY). The use, distribution or reproduction in other forums is permitted, provided the original author(s) and the copyright owner(s) are credited and that the original publication in this journal is cited, in accordance with accepted academic practice. No use, distribution or reproduction is permitted which does not comply with these terms.
*Correspondence: Jianping Jiang, amlhbmdqcEBoemN1LmVkdS5jbg==; Beihui He, Z3JhZjMwM0BzaW5hLmNvbQ==
†These authors have contributed equally to this work
Disclaimer: All claims expressed in this article are solely those of the authors and do not necessarily represent those of their affiliated organizations, or those of the publisher, the editors and the reviewers. Any product that may be evaluated in this article or claim that may be made by its manufacturer is not guaranteed or endorsed by the publisher.
Research integrity at Frontiers
Learn more about the work of our research integrity team to safeguard the quality of each article we publish.