- 1Faculty of Medicine, School of Human Development and Health, University of Southampton, Southampton, United Kingdom
- 2NIHR Southampton Biomedical Research Centre, University Hospital Southampton NHS Foundation Trust and University of Southampton, Southampton, United Kingdom
Cardiovascular disease remains a major global health concern. The combination of the omega-3 fatty acids eicosapentaenoic acid (EPA) and docosahexaenoic acid (DHA) has been shown to beneficially modify a range of cardiovascular risk factors. However, whether EPA and DHA have differential effects or potencies is currently unclear. A systematic review of randomized controlled trials (RCTs) that compared ≥2 g/day of near pure EPA and DHA was conducted. A total of 24 publications from nine unique RCTs were included. EPA and DHA both lower triglyceride levels, with DHA most likely having a slightly greater effect. Furthermore, both EPA and DHA increase high density lipoprotein (HDL) 2 cholesterol, which is cardioprotective, with the increase being greater with DHA. DHA appears to increase low density lipoprotein (LDL) cholesterol; however, DHA also increases LDL particle size, which would render LDL less atherogenic. DHA seems more effective than EPA in decreasing heart rate and blood pressure. Both EPA and DHA alter platelet function decreasing thrombogenicity, although they may have different actions on platelets. Both EPA and DHA decrease F2-isoprostanes, interpreted as a reduction in oxidative stress. They both decrease inflammatory gene expression and promote an anti-inflammatory oxylipin profile. These are all favorable effects with regard to cardiovascular disease risk. Effects of EPA and DHA on blood glucose are inconsistent. This review is constrained by the small number of high quality RCTs that directly compare EPA to DHA and report on outcomes other than blood lipids. There is a need for additional high-quality research to assess the independent effects of EPA and DHA on cardiovascular risk factors (e.g., inflammation, blood pressure, vascular function, platelet function) in larger and more diverse study populations.
Introduction
Cardiovascular disease (CVD) remains the leading cause of mortality worldwide, with around 18.56 million deaths globally in 2019 (1). Of particular concern are the modifiable risk factors that contribute to CVD, which include (but are not limited to) elevated blood cholesterol and triglycerides, inflammation, hypertension, and diabetes. The omega-3 (n-3) polyunsaturated fatty acids eicosapentaenoic acid (EPA) and docosahexaenoic acid (DHA) have emerged as potential interventions to control these risk factors (2–4) resulting in cardioprotective properties (3–5). Although fish is the main dietary source of EPA and DHA, the study of these fatty acids has been helped by the ready availability of supplemental forms and their effects have most often been studied as the combination of EPA and DHA. This combination has been shown to lower triglycerides (6), blood pressure (7), inflammation (8–10) and heart rate (11, 12), to raise high-density lipoprotein (HDL) cholesterol (2), to improve vascular reactivity (13–15), and to reduce platelet reactivity and thrombosis (16). They may also raise low density lipoprotein (LDL) cholesterol (2) and fasting blood glucose (17). However, whether EPA and DHA have differential effects or potencies on risk factors for CVD is uncertain, in part because most trials have focussed on the effects of EPA and DHA when used in combination. It is of interest to know which is the more effective, EPA or DHA, and this information would be useful for regulators, industry and consumers. A small number of trials have been performed that directly compare the effects of pure EPA with pure DHA; these trials were subject to a systematic review published in 2018 (18) that focused on the differential effects of EPA and DHA using the findings from six randomized controlled trials (RCTs) reported in 18 publications (19–36). Knowing that there have been several more trials and publications on this topic published since then, this new systematic review aims to update the previous one and provide a comprehensive and up-to-date analysis of the current literature that specifically compares the effects of EPA and DHA on risk factors for CVD. While there is not a universally agreed-upon recommended dosage for EPA and DHA, this systematic review employed a strict inclusion criterion of ≥2 g per day due to evidence suggesting that this threshold shows favorable impacts on several relevant risk factors (37–39). Furthermore, trials included in this review needed to have used ≥90% of n-3 polyunsaturated fatty acids as either EPA or DHA in order to avoid, as best as possible, the biological effects of the “other” n-3 polyunsaturated fatty acid. These inclusion criteria are consistent with those of the previous systematic review (18).
Materials and methods
Literature search
This systematic review was conducted according to the principles of Preferred Reporting for Systematic Reviews and Meta-Analyses (PRISMA) (40). Searches were conducted in October 2023 in PubMed (2017 to October 2023), EMBASE (2017 to October 2023) and CINAHL (2017 to October 2023) databases. Earlier years were not searched because eligible publications up until 2017 had been identified in the previous systematic review (18). Search terms used included: “EPA,” “DHA,” “eicosapentaenoic acid,” “docosahexaenoic acid” together with “blood lipid,” “lipid,” “triglyceride,” “cholesterol,” “LDL,” “HDL,” “lipoprotein,” “blood pressure,” “inflamm*,” “interleukin-6,” “IL-6,” “C-reactive protein,” “CRP,” “vascular,” “heart rate,” “cardiovascular,” “cardiometabolic.” These search terms are consistent with those used in the previous systematic review (18). The full search strategies for the three databases are shown in Supplementary Table 1. This systematic review was not registered because it was conducted for educational purposes.
Publication selection
Publications had to meet the following criteria to be included in the qualitative synthesis: study must be in humans; study must compare pure or near pure EPA and DHA; study design must be a RCT; dose of EPA and DHA used must be ≥2 g per day; study has to include outcomes of interest (predefined risk factors for CVD); study must be published in the English language; study has to be available as full text. Publications from a previous systematic review (18) on the comparative effects of EPA and DHA on risk factors for CVD that included literature published up to 2017 were also included for qualitative synthesis as shown on the PRISMA flow chart (Figure 1). Publications which met the following criteria were excluded: animal studies; in vitro studies; dose of EPA and DHA used did not meet ≥2 g per day; outcomes of interest not stated or included; study was not an RCT; not published in the English language; study compared other interventions alongside EPA and DHA.
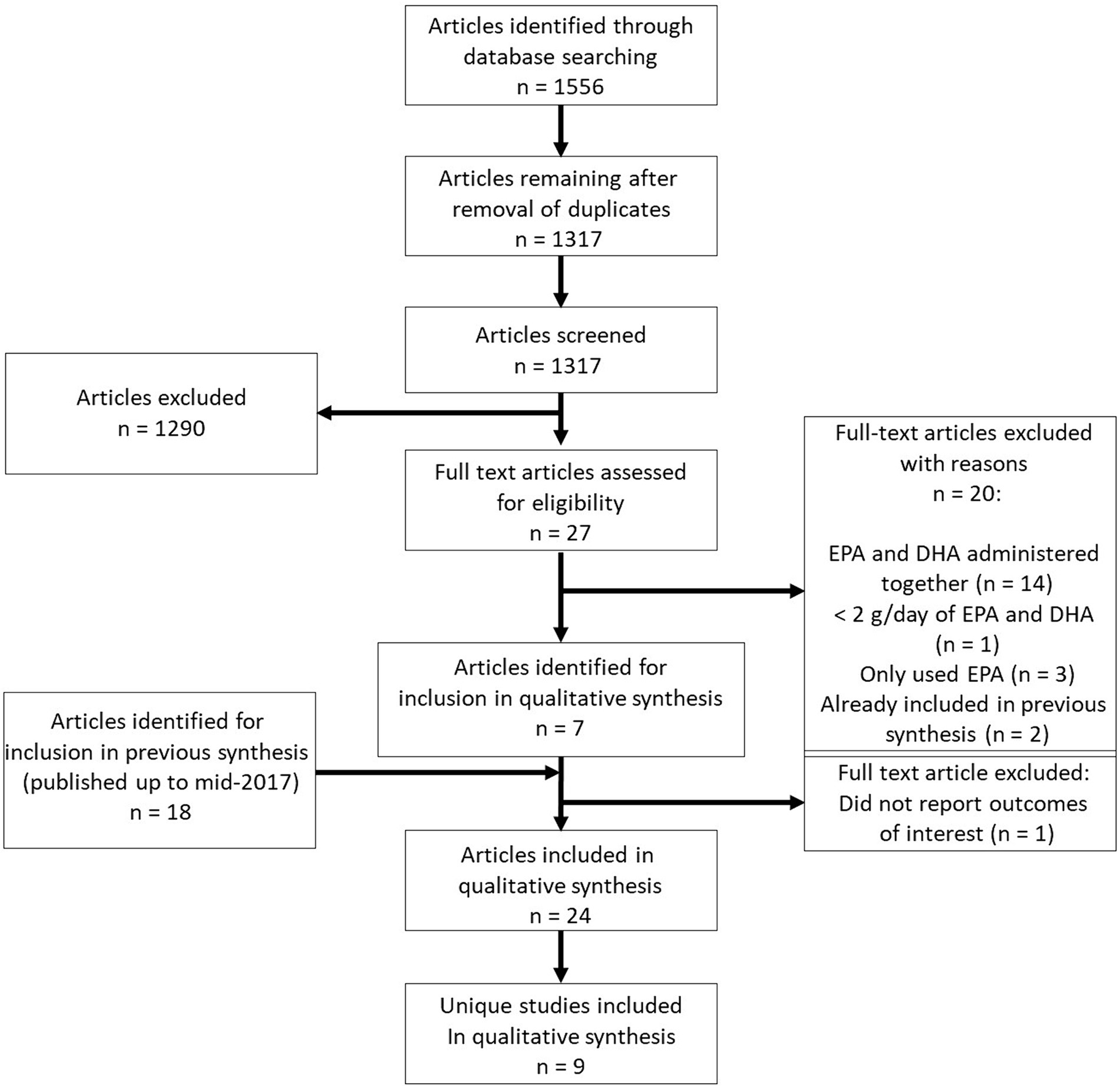
Figure 1. PRISMA flow chart showing selection of publications for inclusion in the systematic review.
Data extraction
The data extracted for each trial included the study design, study population, sample size, dosage levels of EPA, DHA and placebo, study duration and relevant outcomes measured.
Quality assessment
The “parent” publication for each trial was assessed for methodological quality and validity using the Jadad scale (41). Risk of bias was assessed for each individual publication using the Cochrane Risk of Bias tool for RCTs (42).
Results
Identification of included publications
From the electronic literature searches, 1,566 publications of potential relevance were identified. Of these, 249 were removed due to being duplicates and 1,317 were assessed for eligibility based on title and abstract. From these, 1,290 were excluded due to not meeting the eligibility criteria. Based on the abstracts of the remaining 27 publications, 20 were excluded due to the following reasons: trials described in 14 publications administered EPA and DHA together, 1 trial utilized <2 g/day of EPA and DHA, trials reported in three publications administered EPA only, and two publications had already been recorded in the previous systematic review (35, 36). One of these latter publications included in the previous review (35) did not report outcomes of relevance and so was excluded. Hence, seven newly-identified publications were included for qualitative synthesis (43–49). Two of these newly-identified publications (43, 44) report additional results from a trial with publications included in the previous review. Five of the newly-identified publications (45–49) report results from three trials not included in the previous review. From the previous systematic review, 17 publications were also included (19–34, 36). Therefore, a total of 24 publications were included in this systematic review (Figure 1). A number of these publications were from the same trials; hence a total of nine unique RCTs were identified for inclusion; results from six unique RCTs were included in the previous review.
Characteristics of included studies
Table 1 summarizes the characteristics of the nine unique trials including design, population, sample size, dose of EPA, DHA and placebo used, duration, outcomes reported and Jadad score (based on the “parent” publication).
The sample size of the included trials varied between 21 and 224, with a total of 763 participants studied overall. Four trials included healthy participants and one, participants described as healthy with abdominal obesity and chronic inflammation. Other trials included those with overweight and hyperlipidaemia, with dyslipidaemia, with chronic inflammation or with diabetes and being treated for hypertension. The dosage of EPA and DHA given ranged from 2.8 to 4 g/day. The trial durations varied between 4 and 12 weeks (mean 7 weeks). All trials provided EPA, DHA, and placebo in capsules. Placebos used included olive oil (n = 4 trials), corn oil (n = 2), high oleic sunflower oil (n = 1), mixed oils (n = 1) and safflower oil (n = 1). Trial locations varied and included Australia (n = 4 trials), Canada (n = 2), USA (n = 2) and Norway (n = 1). Jadad scores, based on the “parent” publication, varied from 3 to 5, with 4 studies receiving the maximum score of 5. Reasons for not achieving the maximum score were lack of information on method of randomization and/or on method of blinding.
A summary of risk of bias, according to the Cochrane criteria for RCTs, is shown in Table 2. This analysis was completed for each individual publication because the details of different publications from the same trial (e.g., participant numbers) sometimes varied. Most publications, including all publications from 6 of the trials, had a low risk of bias. Reasons for concern are listed, where relevant, in Table 2.
Effects of EPA and DHA on the concentration of EPA and DHA in blood pools
Table 3 summarizes the publications that report on the EPA and DHA concentration in different blood pools. Most publications report fatty acids as % of total fatty acids in the pool, although one reports absolute concentration (19). Of the 12 publications listed, six report fatty acids in plasma or plasma or serum phospholipids (19, 21, 26, 30, 34, 48), while three report fatty acids in platelets (23, 29, 31), two - both from the same trial - in erythrocytes (45, 46) and one in whole blood (49). Supplementing with near pure EPA increases the EPA content of all pools reported on, while supplementing with near pure DHA increases the DHA content of all pools reported on (Table 3). Furthermore, most studies report that supplementing near-pure DHA increases the EPA content of the pools reported on. However, effects of near-pure EPA on DHA concentration are inconsistently observed, with some studies observing a decrease in DHA (19, 23, 26, 29), others little or no change (21, 30, 31, 34, 45, 46, 48, 49). No studies report a significant increase in DHA when EPA is supplemented.
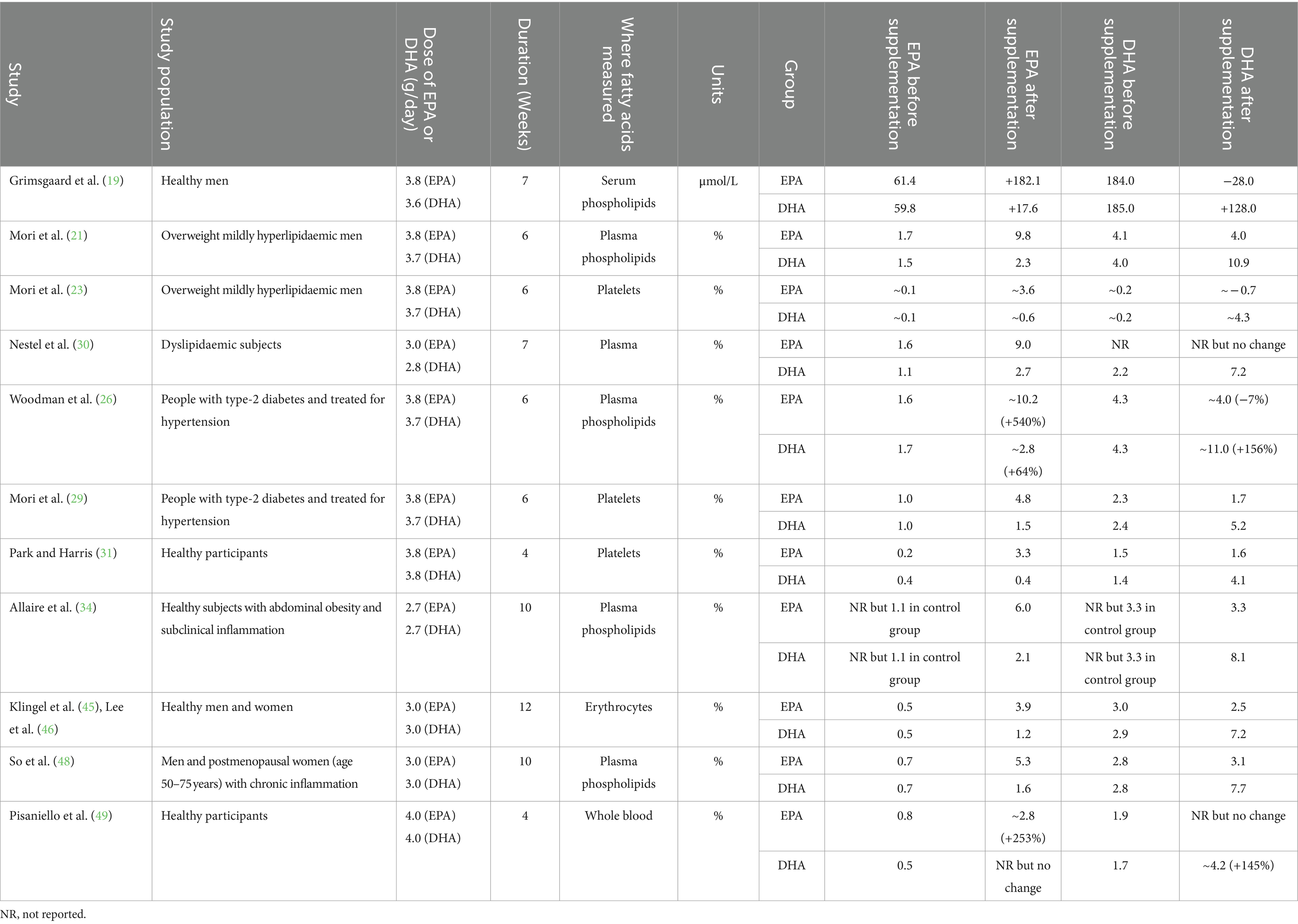
Table 3. Summary of the effects of EPA and DHA supplementation on blood and cell levels of these fatty acids.
Comparative effects of EPA and DHA on cardiovascular risk factors
Effect of EPA vs. DHA on blood lipids and lipoproteins
All nine trials (13 publications) (19, 23, 26, 28, 30, 32–34, 43–45, 48, 49) included outcomes related to the effect of EPA and DHA on blood lipids (Table 4).
The study of Grimsgaard et al. (19) in healthy men, found that both EPA (3.8 g/day) and DHA (3.6 g/day) for 7 weeks led to significant reductions in triglycerides (21 and 26%, respectively) compared to corn oil. Moreover, EPA demonstrated additional benefits by lowering total cholesterol, ApoA1 and ApoB, while DHA increased HDL cholesterol. Both EPA and DHA decreased the ratio of total to HDL cholesterol. Compared to EPA, DHA increased HDL cholesterol and showed a greater decrease in triglycerides, although the latter effect was not statistically significant.
The Mori et al. (23) study involving overweight mildly hyperlipidaemic men using 3.8 g/day of EPA or 3.7 g/day of DHA for 6 weeks demonstrated a decrease in triglycerides for both EPA and DHA (−18% and − 20%, respectively) compared to olive oil. EPA also decreased the HDL3 cholesterol subfraction by 7%, while DHA increased LDL cholesterol by 8%, HDL2 cholesterol by 29% and LDL particle size. EPA did not affect total, LDL, HDL or HDL2 cholesterol or LDL particle size, while DHA did not affect total, HDL or HDL3 cholesterol.
In the Woodman et al. (26, 28) study on hypertensive diabetics, EPA (3.8 g/day) or DHA (3.7 g/day) for 6 weeks had no impact on total, LDL or HDL cholesterol. However, there was a significant decrease in triglycerides with both EPA and DHA (−19% and − 15%, respectively) and there was an elevation in HDL2 (+16% and + 12%, respectively). EPA decreased HDL3 (−11%) but DHA had no effect. DHA but not EPA increased LDL particle size.
In the Nestel et al. (30) study involving dyslipidaemic patients that lasted 7 weeks, there was no observed change in total, LDL or HDL cholesterol levels with either EPA or DHA (3.0 and 2.8 g/day, respectively) compared to olive oil. However, both EPA and DHA were found to lower triglycerides (−23% and − 32%, respectively) and VLDL triglycerides, although there was no significant difference between the effects of the two fatty acids.
Surprisingly, in the Park and Harris study (32, 33), neither EPA nor DHA, at a dosage of 3.8 g/day for 4 weeks, had an impact on triglycerides or on total, LDL, HDL or VLDL cholesterol. This may be because of the short duration of this trial. Nevertheless, there was a significant reduction in ApoB48 and ApoB100 after a high fat challenge, suggesting more efficient handling of dietary fat. In agreement with this, both EPA and DHA increased chylomicron clearance (shorter half-life) and decreased chylomicron particle size when compared to safflower oil. Furthermore, lipoprotein lipase (LPL) activity increased following supplementation of either EPA or DHA, perhaps explaining the faster clearance of chylomicrons. The margination volume, which indicates the extent to which triglyceride-rich lipoproteins adhere to endothelial LPL, was increased with both EPA and DHA (+64% and + 53%, respectively) in the fasted state and with DHA in the fed state, consistent with more rapid triglyceride clearance.
The Allaire et al. (34, 43, 44) study conducted in healthy subjects with subclinical inflammation and abdominal obesity, showed that both EPA and DHA at 2.7 g/day for 10 weeks lowered triglycerides (−12% and − 13%, respectively), and increased LDL cholesterol (+2% and + 7%, respectively), compared to corn oil. The proportion of responders where there was a reduction in plasma triglyceride concentrations by >0.25 mmoL/L was greater with DHA than EPA (45 and 32%, respectively), although the average magnitude of triglyceride reduction was similar between DHA and EPA (0.59 and 0.57 mmol/L, respectively). Compared to control, DHA increased total cholesterol by 4%, increased HDL cholesterol by 8%, lowered the cholesterol/HDL ratio by 3% and increased ApoB by 5%. EPA did not have those effects. Compared to EPA, DHA resulted in a decrease in triglycerides, an increase in total cholesterol and LDL cholesterol (this was more prominent in men than women), an increase in HDL cholesterol and a decrease in the cholesterol/HDL cholesterol ratio. EPA decreased mean LDL particle size, while DHA increased it. Compared to EPA, DHA increased mean LDL particle size and decreased the proportion of small dense LDL by 3.2%. EPA and DHA both decreased PCSK9 concentrations (18.2 and 25% respectively). Furthermore, compared to EPA, DHA increased both the LDL ApoB100 production rate and the fractional catabolic rate.
Klingel et al. (45), found that DHA (3 g/day for 12 weeks) decreased serum triglycerides (from 0.85 ± 0.04 mmol/L to 0.65 ± 0.03 mmol/L) in healthy adults but EPA at the same dose did not. Neither EPA nor DHA significantly affected total or HDL cholesterol in this trial. Both EPA and DHA led to similar increases in serum LPL activity (from 44.1 to 49.1 mU/ml and from 42.9 to 51.5 mU/ml). In another trial involving healthy adults (49), DHA (4 g/day) was found to decrease triglycerides by an average of 27%, but there was no effect on total, LDL or HDL cholesterol or effect of EPA on blood lipids. Overall, there was no difference in effects of EPA and DHA on blood lipids including triglycerides. The duration of this study was only 4 weeks, which is shorter than other trials that show greater effects.
So et al. (48) found that both EPA and DHA (3.0 g/day for 10 weeks) decreased plasma triglyceride concentrations (−20% and −22%, respectively) in older adults with inflammation without changes in ApoB concentrations, resulting in a significant reduction in triglyceride/ApoB ratio with both EPA and DHA. EPA did not affect total, HDL, LDL or non-HDL cholesterol, while DHA raised LDL cholesterol. The LDL-C/ApoB and HDL-C/ApoA1 ratios were increased with both EPA and DHA but the increase of the HDL-C/ApoA1 ratio was greater with DHA. This study also reported the effects of EPA and DHA on the activities of LPL, cholesteryl ester transfer protein (CETP) and lecithin cholesterol acyl transferase (LCAT). These were all affected by both EPA and DHA but in a sex-specific way. DHA increased LPL activity and decreased LCAT activity with the latter only seen in women. EPA decreased CETP and LCAT activity with the decrease in LCAT again seen only in women.
Effect of EPA vs. DHA on inflammatory markers
Four trials (five publications) (29, 34, 36, 47, 49) included outcomes related to the effect of EPA and DHA on inflammatory markers (Table 5). The Allaire et al. (34)/Vors et al. (36) study observed a significant reduction in plasma levels of CRP, IL-6, IL-18 and TNF-α (−8, −12, −7, and − 15% respectively) and an increase in adiponectin (3%) with DHA supplementation (2.7 g/day) compared to corn oil. In contrast, EPA at the same dose only decreased plasma IL-6 (−13%). Compared to EPA, DHA resulted in a greater decrease in IL-18 and a greater increase in adiponectin. Both EPA and DHA decreased CD14 gene expression and increased PPARA gene expression. EPA also increased expression of the TRAF3 gene, while DHA increased expression of the TNFA gene. In contrast to some of these findings, Mori et al. (29) saw no effect of EPA (3.8 g/day) or DHA (3.7 g/day) on plasma CRP or IL-6, while both EPA and DHA tended to decrease plasma TNF-α, with DHA having a greater effect, although this was not formally tested statistically. So et al. (47) reported no effect of EPA or DHA (3 g/day) on plasma CRP, TNF-α, IL-6, MCP-1 or IL-10 and no difference in effect of EPA and DHA on these biomarkers of inflammation. DHA decreased monocyte secretion of TNF-α, IL-6 and MCP-1 in response to lipopolysaccharide and decreased expression of the TNFA, IL6, MCP1 and IL10 genes. EPA only decreased expression of the TNFA gene. Both EPA and DHA modified the plasma oxylipin profile; both decreased several arachidonic acid (AA)-derived oxylipins. EPA increased several EPA-derived oxylipins while DHA increased several DHA- and EPA-derived oxylipins. Finally, Pisaniello et al. (49) reported no effect of EPA or DHA (4 g/day) on serum CRP in healthy adults. Serum from those supplemented with EPA decreased CCL2 gene expression in endothelial cells. Other genes were unaffected and serum from those supplemented with DHA had no effect on any genes.
Effect of EPA vs. DHA on blood pressure, haemodynamics, and vascular function
Six trials (eight publications) (20–22, 26, 27, 30, 46, 49) included outcomes related to effects of EPA and DHA on blood pressure, haemodynamics and vascular function (Table 6). Grimsgaard et al. (20) reported an increase in heart rate (+1.9 bpm) with EPA (3.8 g/day) but a decrease in heart rate (−2.2 bpm) with DHA (3.6 g/day) in healthy men. When directly compared with each other, DHA resulted in a decreased heart rate compared to EPA. Both EPA and DHA improved left ventricular diastolic filling but there was found to be no significant effect of either EPA or DHA on blood pressure. In the Mori et al. (21, 22) study in overweight mildly hyperlipidaemic men, DHA (3.7 g/day) decreased both systolic and diastolic blood pressure compared to placebo. However, EPA (3.8 g/day) was found to have no significant effect on blood pressure. DHA decreased heart rate by around 3.5 bpm over a 24-h period compared to placebo. Furthermore, DHA increased vasodilator responses and attenuated constrictor responses in forearm blood flow compared to placebo. In the Nestel et al. (30) study in dyslipidaemic subjects, there was no effect of EPA (3 g/day) or DHA (2.8 g/day) on heart rate or blood pressure. However, there was an increase in systemic arterial compliance with EPA (+36%) and DHA (+27%) compared to placebo. There was also a non-significant lowering of vascular resistance with both EPA and DHA. There was no significant difference between the effects of EPA and DHA for any of the parameters. In the study of anti-hypertensive-treated type 2 diabetics by Woodman et al. (26, 27), there was no significant effect of EPA (3.8 g/day) or DHA (3.7 g/day) on blood pressure or vascular function. However, there was a non-significant decrease in 24 h heart rate with both EPA and DHA. In a study of healthy young men and women, Lee et al. (46) reported an increase in heart rate (4.2 beats/min) and systolic and diastolic blood pressure with EPA compared to the olive oil placebo, but no effect of DHA (both at 3 g/day); effects of EPA were different from those of DHA. DHA, but not EPA, increased cardiac muscle sympathetic nerve activity burst frequency and incidence. Finally, Pisaniello et al. (49) reported no effect of EPA (4 g/day) on heart rate or blood pressure in heathy adults. DHA (4 g/day) also did not affect heart rate or systolic blood pressure, but decreased diastolic blood pressure.
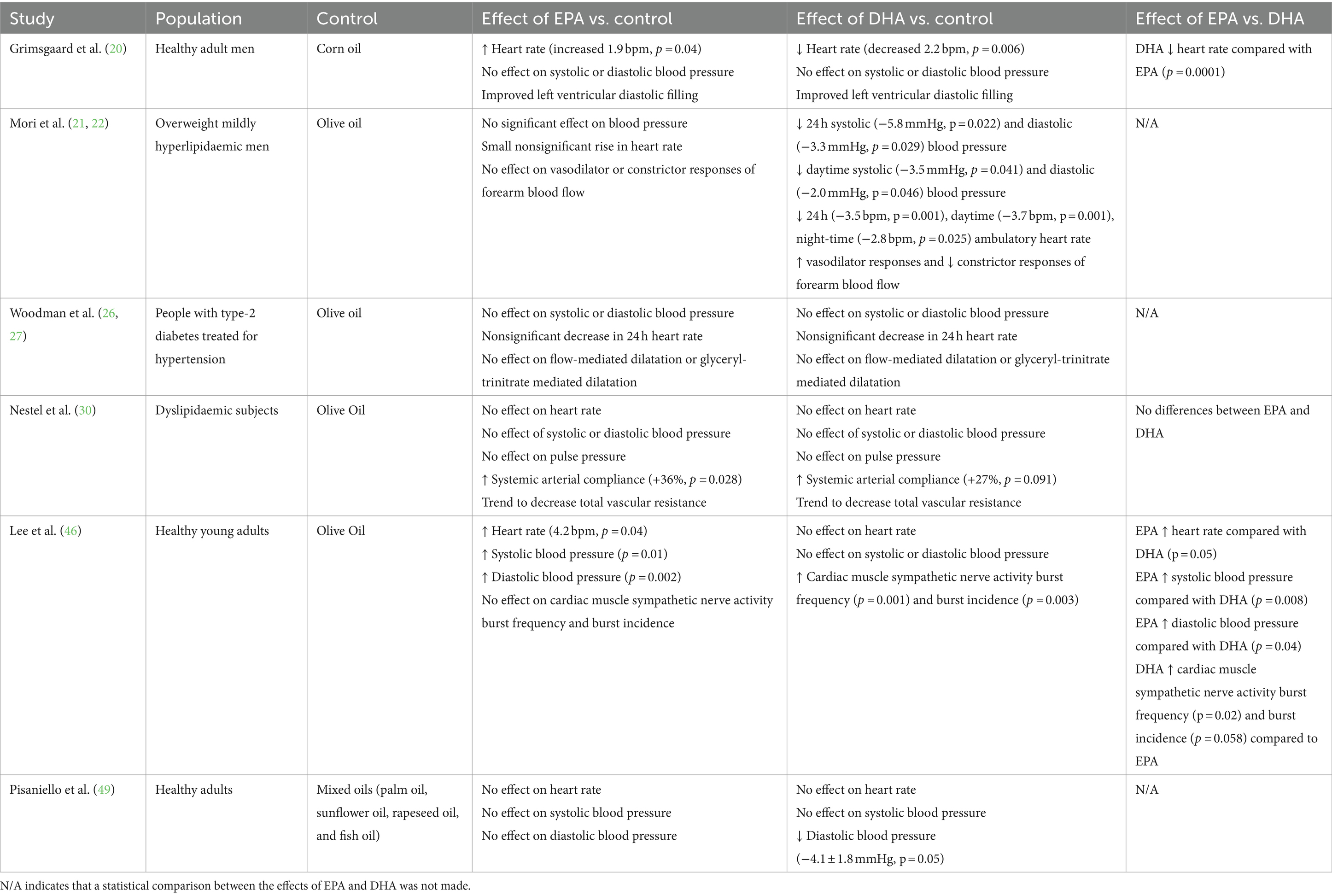
Table 6. Summary of findings related to effect of EPA and DHA on blood pressure, haemodynamics and vascular function.
Effect of EPA vs. DHA on glycaemic control
Three trials (23, 26, 45) included outcomes related to effects of EPA and DHA on glycaemic control (Table 7). In the Mori et al. (23) trial in overweight mildly hyperlipidaemic men, fasting insulin was found to be increased by both EPA (+18%) and DHA (+27%). With EPA there was also a trend toward increased fasting glucose (+4%) but there was no effect of DHA on fasting glucose. With DHA, there was a decrease in the glucose:insulin ratio, as a result of the effect on insulin. In type-2 diabetics treated for hypertension (26), both EPA and DHA increased fasting glucose, with a larger effect of EPA than DHA (+19 vs. +12%). There was no effect of either EPA or DHA on fasting insulin, glycated hemoglobin, fasting C-peptide, insulin sensitivity or insulin secretion compared to control. Klingel et al. (45) reported no effect of either EPA or DHA (3 g/day) on fasting glucose compared to olive oil in healthy participants.
Effect of EPA vs. DHA on platelet and fibrinolytic function
Two trials (28, 31) included outcomes related to effects of EPA and DHA on platelet and fibrinolytic function (Table 8). The Park and Harris (31) trial conducted in healthy subjects reported a decrease in mean platelet volume and an increase in platelet numbers with EPA (3.8 g/day) compared with safflower oil. However, with DHA (3.8 g/day), there was no effect on platelet volume or count. The effects of EPA were significantly different from those of DHA. The effects on platelet aggregation were not assessed in that trial. In the Woodman et al. (28) trial in anti-hypertensive-treated type 2 diabetics DHA, in contrast to EPA, led to a decrease in collagen-stimulated platelet aggregation (−17%) and platelet-derived thromboxane B2 release (−19%) when compared to olive oil. Thrombaxane B2 is derived from thromboxane A2 which promotes platelet aggregation and so these two observations with DHA may be related. In that trial, neither EPA nor DHA demonstrated any effect on markers of fibrinolytic function.
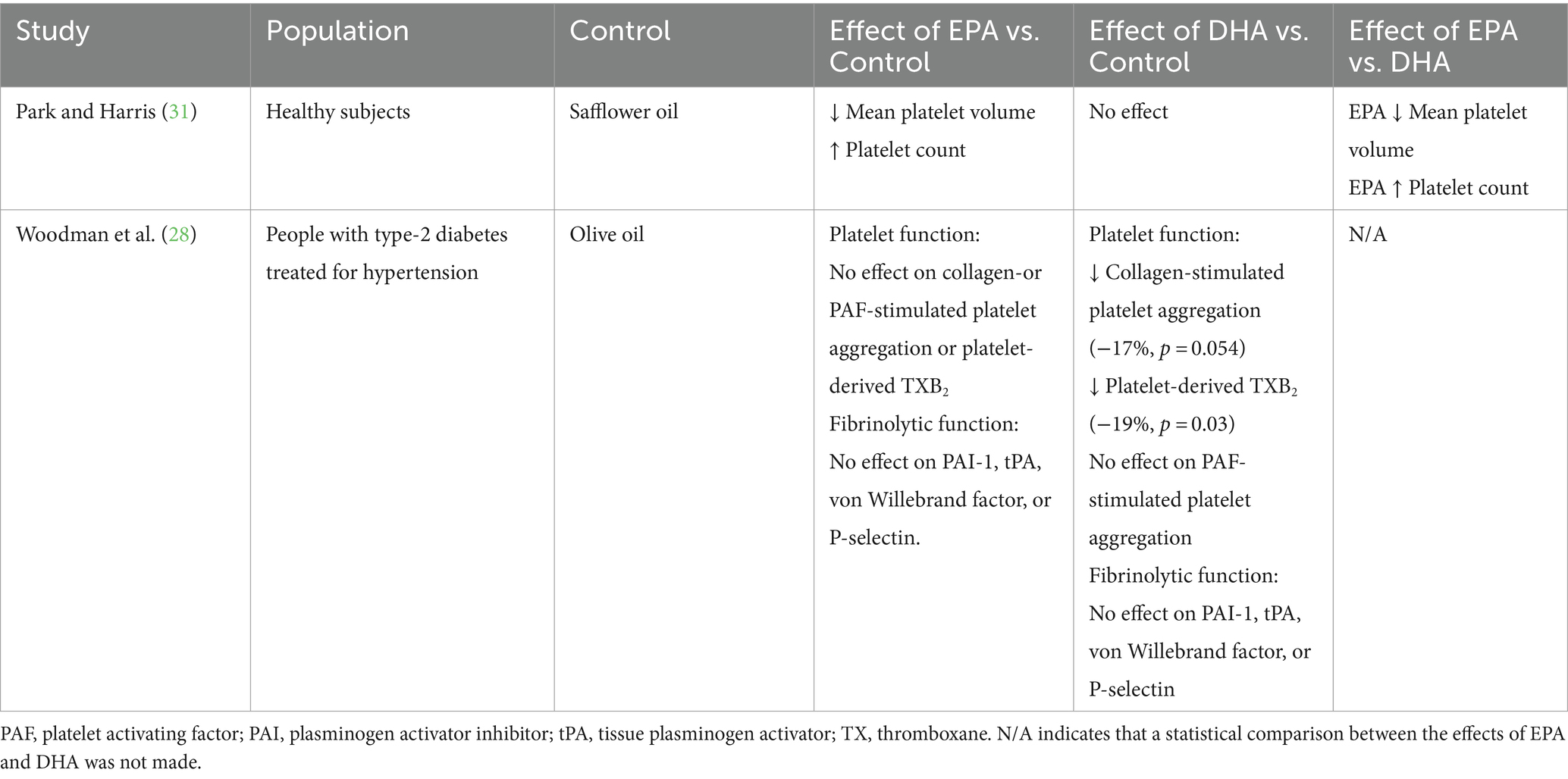
Table 8. Summary of findings related to effect of EPA and DHA on platelet and fibrinolytic function.
Effect of EPA vs. DHA on oxidative stress
Two trials (three publications) (24, 25, 29) included outcomes related to effects of EPA and DHA on oxidative stress as assessed by measuring F2 isoprostances in urine and plasma (Table 9). In a trial in overweight mildly hyperlipidaemic men (24, 25), both EPA and DHA significantly decreased urinary F2 isoprostanes (by 27 and 26% respectively) and plasma F2 isoprostanes (by 24 and 14% respectively) compared to olive oil. Likewise, in a trial in type 2 diabetics treated for hypertension (25, 29), both EPA and DHA significantly decreased urinary F2 isoprostanes (by 19 and 20% respectively) and plasma F2 isoprostanes (by 19 and 23% respectively) compared to olive oil. The effects of EPA and DHA were not formally compared but DHA tended to have greater effects than EPA on F2 isoprostanes as a marker of oxidative stress.
Discussion
Context of this systematic review
Higher dietary intakes and higher blood and tissue status of the omega-3 PUFAs EPA and DHA are associated with lower risk of developing CVD and mortality from CVD, including coronary heart disease (3, 4) and some studies have reported that intervention with these fatty acids decreases adverse cardiovascular outcomes in at risk patients (50, 51). EPA and DHA act through beneficial effects on multiple cardiovascular risk factors, as demonstrated in numerous trials and meta-analyses of RCTs (2–4, 6–16). An important question that is not fully resolved is whether EPA and DHA have the same or unique actions (52, 53). A systematic review of RCTs that compared the effect of ≥2 g/day of near pure EPA and DHA on cardiovascular risk factors was published in 2018 (18). It included 18 publications from 6 unique RCTs and concluded that EPA and DHA do appear to have differential effects on at least some risk factors for CVD. New information on this topic has been published since mid-2017 when the literature search for that systematic review was conducted. Therefore, the search was updated and this systematic review produced. The new search identified an additional seven publications including five publications from 3 new RCTs (45–49) and 2 from one of the previously included RCTs (43, 44). New data on plasma lipids and lipoproteins (43–45, 47, 48), inflammatory biomarkers (47, 49), blood pressure, haemodynamics and vascular function (46, 49) and glycaemic control (45) are included.
Summary of effects of EPA vs. DHA on cardiovascular risk factors
Six out of nine trials reported that EPA lowered triglycerides compared with placebo, while seven out of nine trials reported that DHA lowered triglycerides. Although some trials suggest a greater triglyceride lowering effect of DHA than EPA, the difference sometimes appears to be small, and is not apparent in some trials. Effects of EPA and DHA on cholesterol, LDL and HDL are less consistent. Most trials report no effect of EPA on LDL cholesterol, although one reported an increase (34). Although some trials report no effect of DHA on LDL cholesterol, 3 reported an increase (23, 34, 48). No included trials reported that EPA affects HDL cholesterol and, while most trials also report no effect of DHA, two did report increased HDL cholesterol with DHA (19, 34). There may be effects of EPA and DHA on HDL subfractions: two trials reported that EPA lowers HDL3 (26, 34) while one reported higher HDL2 (26). Two trials reported that DHA increases HDL2 (23, 26). Regarding LDL particle size, two trials reported no effect of EPA (23, 28), while one reported a decrease in size (43). Three trials reported that DHA increased LDL particle size (23, 28, 43). The trials of Park and Harris (32, 33) and So et al. (48) indicate that EPA and DHA can have effects on the enzymes that metabolize lipoproteins and control the transfer of lipid moieties between lipoproteins. Thus, high dose EPA and DHA lower triglycerides, with DHA possibly being more potent. EPA has little impact on either LDL or HDL cholesterol, but may lower the level of the harmful HDL3 subfraction. DHA can raise both LDL and HDL cholesterol, may raise the level of the protective HDL2 subfraction, and increases LDL particle size, perhaps rendering LDL less atherogenic.
Regarding inflammatory markers, all four included trials reported that EPA did not alter CRP levels; 3 out of the 4 trials also report no effect of DHA, but one (34) reported that DHA lowered CRP. No included trial reported that EPA altered any of the cytokines assessed (TNF-α, IL-6, IL-10, MCP-1, IL-18, adiponectin), but these were mostly only measured in one trial. One trial did report a trend to lower TNF-α with EPA (29). The trial that reported that DHA lowered CRP also found lower TNF-α, IL-6 and IL-18 and higher adiponectin with DHA (34). Another trial (29) reported a trend to lower TNF-α with DHA. Both EPA and DHA decreased CD14 gene expression and increased PPARA gene expression in one trial (36). Both EPA and DHA decreased the inflammatory response seen with LPS stimulation of monocytes studied ex vivo, with a stronger effect of DHA (47). Both EPA and DHA modified the plasma oxylipin profile (47) Thus, both EPA and DHA promoted an anti-inflammatory gene expression profile and reduced the response of monocytes to an inflammatory stimulus; however circulating biomarkers of inflammation like CRP and IL-6 are little impacted by EPA, but these may be lowered by DHA suggesting that DHA has stronger anti-inflammatory actions. Both EPA and DHA foster a less inflammatory plasma profile of oxylipins.
Five out of 6 trials reported no effect of EPA on blood pressure, 4 out of 6 reported no effect on heart rate and 2 out of 3 reported no effect on vascular function. One trial reported that EPA increased systolic and diastolic blood pressure in healthy young adults compared to the olive oil placebo (46). Two trials reported that EPA increased heart rate in healthy adult men (20, 46), while another reported a trend to decreased heart rate in people with diabetes being treated for hypertension (26). One trial reported that EPA improved arterial compliance in people with dyslipidaemia (30). Four out of 6 trials reported no effect of DHA on blood pressure, 3 out of 6 reported no effect on heart rate and 1 out of 3 reported no effect on vascular function. One trial reported that DHA decreased systolic and diastolic blood pressure in overweight mildly hyperlipidaemic men (21) while another reported lower diastolic, but not systolic, blood pressure in healthy adults (49). Two trials reported that DHA lowered heart rate (20, 21), while a third trial reported a trend for this (26). Two trials reported that DHA improved vascular function (22, 30). Overall, DHA appears to have stronger and more favorable effects on blood pressure, heart rate and vascular function than EPA.
One trial reported that EPA increased fasting glucose (26); another reported a trend for this outcome (23) but a third found no effect of EPA (45). One trial reported that EPA increased fasting insulin (23) but a second trial did not see this (26). That trial found no other effects of EPA on markers of glucose homeostasis including insulin sensitivity. One trial reported that DHA increased fasting glucose (26); two others found no effect of DHA (23, 45). One trial reported that DHA increased fasting insulin (23) but a second trial did not see this (26). That trial found no other effects of DHA on markers of glucose homeostasis including insulin sensitivity. Overall, there seems to be little impact of EPA and DHA on glucose homeostasis.
One trial reported that EPA decreases mean platelet volume and increases platelet number, with DHA not having these effects (31). A second trial reported that DHA decreased collagen-stimulated platelet aggregation and thromboxane B2 generation, but EPA did not have these effects (28). There was no effect of EPA or DHA on markers of fibrinolytic function in the one trial that reported these (28).
Both EPA and DHA were found to decrease urinary and plasma F2 isoprostanes assessed as markers of oxidative stress, with little difference in potency (24, 25, 29).
Discussion of the findings
This systematic review suggests that EPA and DHA have quantitatively different effects on CVD risk factors such as blood lipids including triglycerides, blood pressure, heart rate, vascular function, platelet function, and inflammatory markers. The trials included in this review show that DHA has a more favorable impact on several of these parameters.
It is suggested that the plasma triglyceride lowering effect of both EPA and DHA is due to a number of factors, including an inhibitory effect on hepatic triglyceride synthesis and VLDL assembly and secretion and an enhanced triglyceride hydrolysis and clearance of VLDL by LPL (54). This was supported by the Klingel et al. (45) trial that showed that both EPA and DHA increased LPL activity. DHA had a greater effect on LPL activity, which might account for its (slightly) greater triglyceride lowering action. The decrease in hepatic triglyceride synthesis and VLDL secretion is thought to be due to the decrease in synthesis of ApoB100, which is required for VLDL assembly. A decrease in hepatic VLDL assembly and secretion is thought to be beneficial for lowering blood triglyceride levels, as VLDL is the main lipoprotein that transports triglycerides (54). Interestingly, Allaire et al. (44) reported an increase in the VLDL ApoB100 catabolism rate which is the rate at which VLDL is cleared from the bloodstream, which further supports the decrease in triglyceride levels observed with EPA and DHA. VLDL catabolism generates LDL, so this could be part of the mechanism for the elevated LDL-cholesterol reported in some studies of DHA.
In terms of the results with other blood lipids and blood lipid-related factors, one of the newer trials identified a decrease in PCSK9 levels with both EPA and DHA (43). PCSK9 degrades the cell surface LDL receptors responsible for clearing circulating LDL and therefore contributes to elevated LDL-cholesterol (55). An EPA- or DHA-mediated decrease in PCSK9 would be anticipated to result in better LDL clearance and so lower LDL-cholesterol. Contrary to this expectation, some trials report elevated LDL-cholesterol, especially with DHA (23, 34, 48). Apart from an increase in LDL levels, some trials reported an increase in LDL particle size with DHA supplementation (23, 28, 43). Allaire et al. (43) suggest that the reason for this could be due to the decrease in ApoCIII secretion from the liver through the regulation of transcriptions factors and binding proteins. This apparent reduction in ApoCIII production after DHA leads to increased conversion of VLDL to LDL and the formation of larger LDL particles compared to EPA, as reported in several trials. In this regard DHA also decreased the proportion of pro-atherogenic small dense LDL particles (43). The increase in HDL cholesterol that is reported with DHA could be explained by altered activity of lipid transfer proteins such as CETP (48) which results in the transfer of cholesteryl esters from HDL toward more triglyceride-rich lipoproteins.
Regarding the effects of EPA and DHA on inflammation, there is some inconsistency in the findings of the included trials. In terms of their actions on inflammation, EPA and DHA are thought to have anti-inflammatory effects through the replacement of, and competition with, AA in the cell membrane which results in decreased production of eicosanoids from AA which tend to be more inflammatory (such as prostaglandin E2 and leukotriene B4) and increased production of eicosanoids from EPA (leukotriene B5 and prostaglandin E3) which are less inflammatory (56, 57). Furthermore, EPA and DHA both give rise to lipid mediators termed specialized pro-resolving mediators (58–60). Interestingly, So et al. (47) reported a greater reduction in plasma phospholipid AA with DHA than EPA linked with a greater reduction in AA derivatives such as prostaglandin E2 and thromboxane B2. There was also an elevation in some of the EPA- and DHA-derived oxylipins (47). EPA and DHA are also known to have a role in regulating inflammatory gene expression (56, 57), effects seen in the studies reported in Allaire et al. (34, 36) and So et al. (47). Markers of inflammation such as CRP, IL-6, IL-1, and TNF-α are linked with an increased likelihood of CVD and cardiovascular events (61, 62). Furthermore, there is a relationship between the inflammatory markers themselves, for example IL-6 triggers CRP to be synthesized in the liver. Despite the reported effects on oxylipins and gene expression in the included trials (34, 36, 47), there were few effects on circulating markers of inflammation, although Allaire et al. (34) reported a decrease in plasma CRP, TNF-α, IL-6, and IL-18 with DHA and a decrease in IL-6 with EPA.
With respect to the limited trials that show a decrease in blood pressure with EPA and DHA, it is suggested that the mechanism for this is related to a decrease in systemic vascular resistance due to the increase in nitric oxide production (63), decreased response to angiotensin II and noradrenalin (64, 65) and an increase in arterial compliance (66) leading to a reduction in systolic and diastolic pressure. The changes in heart rate observed with DHA can be explained by the beneficial impacts on cardiac muscle cell function and the likely changes in membrane fluidity, which alter the conductive properties of ion channels within those membranes (67) resulting in lowered heart rate.
Regarding glycaemic control, the results are limited, but some trials report that EPA and DHA may increase fasting glucose and fasting insulin, which may be seen as a deleterious effect. These effects may be due to an increase in hepatic glucose output and an increase in plasma glucagon concentrations. It is worth noting that the recent trial by Klingel et al. (45) reported no effect of either EPA or DHA on blood glucose.
EPA and DHA appear to have different effects on platelet function with EPA reducing mean platelet volume and DHA reducing collagen-induced platelet aggregation. The mechanism behind effects on platelet aggregation is similar to the effects on inflammation, in that EPA and DHA displace AA from the platelet membrane leading to a decrease in thromboxane A2 which is a platelet aggregator and an increase in EPA-derived prostacyclin PGI3 which is an inhibitor of platelet aggregation (68). Regarding platelet volume, an increase in platelet volume would suggest a more pro-atherogenic environment; therefore, EPA may reduce the incidence of atherogenic events via the reduction of mean platelet volume; DHA seems not to have this effect.
In the context of oxidative stress, both plasma and urinary F2-isoprostanes have been established as biomarkers indicative of in vivo lipid peroxidative damage (69). AA is the precursor for the synthesis of F2-isoprostanes (69). The reduction in F2-isoprostanes by EPA and DHA is interpreted to indicate reduced oxidative stress. However, since AA is the precursor to F2-isoprostanes, it may be that lower F2-isoprostanes also partly reflect the lowering of AA that is a feature of increased intake of EPA and DHA. Nevertheless, Mas et al. (25) reported that the effects of EPA and DHA on plasma F2-isoprostanes are retained when the data are adjusted for the change in plasma AA.
The broader context
Irrespective of whether there are differences in their quantitative effects, the beneficial impact of both EPA and DHA on a range of recognized and emerging risk factors for CVD suggests that they play an important role in disease prevention. This is supported by multiple epidemiological studies which evaluate the association between intake or blood or tissue levels of EPA and DHA and incident disease during a, usually long, follow-up period. Chowdhury et al. (70) aggregated such prospective studies investigating risk of coronary outcomes. Data from 16 studies, including over 422,000 subjects, showed a 13% reduction in risk for those in the top third of dietary intake of EPA + DHA than those in the lower third of intake. Data from 13 studies with over 20,000 participants showed a 22, 21, and 25% reduction in risk of coronary outcomes for those in the top third of blood levels of EPA, DHA, or EPA + DHA, respectively, compared to those in the lower third (70). Using data from 17 prospective cohort studies, Alexander et al. (71) reported an 18% lower risk for any coronary heart disease event for subjects with higher dietary intake of EPA + DHA than for those with lower intake. There were also significant reductions of 19, 23, and 47% in the risk for fatal coronary death, coronary events, and sudden cardiac death, respectively. Another study pooled data from 19 trials investigating the association between EPA or DHA concentration in a body pool, such as serum, plasma, red blood cells, or adipose tissue, and risk of future coronary heart disease in adults who were healthy at study entry (72). Both EPA and DHA were independently associated with a reduction in the risk of fatal coronary heart disease, with about a 10% reduced risk for each one standard-deviation increase in either EPA or DHA. Harris et al. (73) gathered together 10 cohort studies and found a 15% lower risk of fatal coronary heart disease for each one-standard-deviation increase in the omega-3 index (i.e., the sum of EPA + DHA in red blood cells). A de novo pooled analysis of 17 prospective cohort studies with 42,466 individuals confirmed the association between a lower risk for death from CVD in those with the highest vs. the lowest quintile of circulating EPA, DHA, and EPA + DHA (74). These analyses support a clear role for EPA and DHA in the primary prevention of coronary heart disease and, perhaps more widely, of CVD, as discussed elsewhere (3, 4), findings that underpin current dietary recommendations for intake of these fatty acids (75–78). The Vitamin D and Omega-3 (VITAL) trial also provides some support for these recommendations. This was an RCT conducted as a two-by-two factorial design of vitamin D3 (at a dose of 50 μg/day) and EPA + DHA (1 g/day) among 25,871 healthy participants aged over 50 years for the primary prevention of CVD and cancer (79). After a median follow-up of 5.3 years, there was no statistically significant difference between the groups receiving EPA + DHA or placebo in the primary outcome of major cardiovascular events (a composite of myocardial infarction, stroke, or death from cardiovascular causes). However, an analysis of the individual components of the composite showed a significant reduction in the EPA + DHA arm for myocardial infarction (28% reduction) and coronary heart disease (17% reduction). Correspondingly, there was also a lower risk of death from these two non-prespecified outcomes (50% for myocardial infarction and 24% for coronary heart disease), although the effect on coronary heart disease mortality was not significant. There was a significant reduction in major adverse cardiovascular events (19%) and risk of myocardial infarction (40%) for those who consumed fewer than 1.5 fish meals per week and then supplemented with EPA + DHA. Although there was no effect on the primary outcome (first serious vascular event) between EPA + DHA (840 mg/day) and placebo groups over a median follow-up on 7.4 years in A Study of Cardiovascular Events in Diabetes (ASCEND), a study of 15,480 people living with diabetes but with no evidence of CVD, there were 19% fewer deaths from vascular events in the EPA + DHA arm as well as a trend toward reduced risk of death (21%) from coronary heart disease (80).
Despite the consistent evidence for EPA and DHA reducing risk of CVD, findings from trials using these fatty acids therapeutically in those already with high risk or with advanced disease have proven to be inconsistent (3, 4). This has led to discussion about the relative impact of EPA and DHA, since different therapeutic trials have used different formulations. In the GISSI-Prevenzione study (81) involving survivors of recent myocardial infarction (≤ 3 months since myocardial infarction), treatment with EPA + DHA (840 mg/day) significantly reduced the composite primary outcomes (−15% and − 20%, respectively) and several secondary outcomes, including cardiovascular death by 30%, sudden death by 45%, and total fatal events by 20%. In the GISSI-HF trial (82), patients with chronic heart failure received EPA + DHA (840 mg/day) or placebo for approximately 4 years, and there a small (9%) but significant reduction in all-cause mortality. These trials suggest that the combination of EPA + DHA may be effective therapeutically. The randomized, open-label Japan EPA Lipid Intervention Study (JELIS) included patients with hypercholesterolemia who were assigned to receive either a statin alone or a statin along with highly purified EPA (1.8 g/day EPA) with a 5-year follow-up (83). The primary outcome was any major coronary event, including sudden cardiac death, fatal and nonfatal myocardial infarction, and other nonfatal events, including unstable angina pectoris, angioplasty, stenting, and coronary artery bypass grafting. Long-term use of EPA-ethyl ester as an addition to statin therapy had no effect over statin alone on the primary outcome in the primary prevention arm of the trial, but in the secondary prevention arm, EPA supplementation resulted in a significant 19% reduction in nonfatal coronary events vs. statin alone (83). JELIS highlights that EPA may be effective in the absence of DHA. This latter conclusion is supported by more recent trials. In the Reduction of Cardiovascular Events with Icosapent Ethyl Intervention Trial (REDUCE-IT) (50), 8,179 high risk patients received 3.6 g/day of EPA as ethyl ester or mineral oil as placebo with a median follow-up of 4.9 years. The primary outcome (a composite of cardiovascular death, nonfatal myocardial infarction, nonfatal stroke, coronary revascularization, or unstable angina) was reduced by 25% in patients who received EPA-ethyl ester compared to placebo. The key prespecified secondary outcome (a composite of cardiovascular death, nonfatal MI, or nonfatal stroke) was also significantly reduced in the EPA-ethyl ester group as were a whole range of other clinical outcomes (50). Another positive EPA-ethyl ester trial was Effect of Vascepa on Improving Coronary Atherosclerosis in People with High Triglycerides Taking Statin Therapy (EVAPORATE) (84). This study involved 80 patients with known angiographic coronary artery disease taking statins and with no history of myocardial infarction, stroke, or life-threatening arrhythmia within the prior 6 months. The same EPA-ethyl ester preparation and the same dose were used as in REDUCE-IT. EVAPORATE demonstrated that EPA might directly promote atherosclerotic plaque attenuation in hypertriglyceridemic individuals at 18 months (84). In contrast to this series of trials demonstrating significant therapeutic benefit of EPA provided in the absence of DHA (50, 83, 84), trials of the combination of EPA + DHA conducted since the two GISSI trials provide inconsistent findings. The Outcome Reduction with an Initial Glargine Intervention (ORIGIN) trial with 840 mg/day EPA + DHA in 12,536 dysglycemic patients with recent myocardial infarction or heart failure and a median follow-up of 6.2 years was null (85), as was the Risk and Prevention Study which assessed the effect of 840 mg/day EPA + DHA in 12,513 patients at high cardiovascular risk but with no myocardial infarction for a median of 5 years (86). However, in a prespecified subgroup analysis, compared with placebo, EPA + DHA resulted in an 18% lower incidence of the revised primary outcome among women (composite of the time to death from cardiovascular causes or first hospital admission for cardiovascular causes). Also, admissions for heart failure were significantly lower in the long-chain omega-3 fatty acid group. It is worth noting that the trials of EPA + DHA have used a lower dose (840 mg/day) than trials of pure EPA (1.8 or 3.6 g/day), so any difference in findings of these trials could relate to dosing.
One trial that has questioned the impact of the combination of EPA + DHA is the Long Term Outcomes Study to Assess Statin Residual Risk with Epanova in High Cardiovascular Risk Patients with Hypertriglyceridemia (STRENGTH) trial (87). In this study, patients with hypertriglyceridemia and high cardiovascular risk on statin therapy were treated with 4 g/day of an oil containing EPA and DHA (as free fatty acids); this provided about 2.2 g EPA and 0.8 g DHA daily. Corn oil was used as placebo. There was no significant difference in a composite outcome of major adverse cardiovascular events among patients who received additional omega-3 fatty acids to usual background therapies vs. control, and the trial was stopped early (87). The Omega-3 Fatty Acids in Elderly with Myocardial Infarction (OMEMI) trial randomized a total of 1,027 patients with a recent myocardial infarction (in the previous 2–8 weeks) to receive approximately 1.6 g/day of EPA + DHA (930 mg EPA and 660 mg DHA as triglycerides) or corn oil (placebo) as an addition to standard care (88). After 2 years of follow-up, there was no significant difference between the two groups in the primary composite cardiovascular outcome.
Thus, REDUCE-IT and EVAPORATE both report benefits of pure EPA while STRENGTH and OMEMI report no benefit of the combination of EPA + DHA. The reasons for this discrepancy between REDUCE-IT and STRENGTH have been discussed elsewhere (89, 90) and include choice of placebo, formulation of omega-3 fatty acids (ethyl ester vs. free fatty acids) and exact omega-3 dose (3.6 vs. 3 g/day). Another possibility is that DHA negates the benefits of EPA so that the combination of EPA + DHA is less effective than EPA alone, although the mechanisms for how this would occur are unclear. There are no long-term studies comparing the therapeutic effect of pure EPA, pure DHA and the combination of EPA + DHA on hard cardiovascular endpoints.
Conclusion
The results of this systematic review suggest that EPA and DHA have some similar and some different effects on cardiovascular risk factors. EPA and DHA both lower triglyceride levels with DHA most likely having a slightly greater effect. Furthermore, both EPA and DHA increase HDL2 cholesterol, which is cardioprotective, with the increase being greater with DHA. DHA appears to increase LDL cholesterol and LDL particle size which would render LDL less atherogenic. From the more limited study data, both EPA and DHA decreased some inflammatory markers and pro-inflammatory gene expression, with DHA having stronger effects. DHA may be more effective than EPA in decreasing heart rate and blood pressure. Both EPA and DHA alter platelet function decreasing thrombogenicity, although they have different actions on platelets. Both EPA and DHA decrease F2-isoprostanes, interpreted as a reduction in oxidative stress. They both decrease inflammatory gene expression and promote an anti-inflammatory oxylipin profile. These are all favorable effects with regard to cardiovascular risk. Reported effects of EPA and DHA on blood glucose are inconsistent.
Although the new data on the effects of EPA and DHA on blood lipids including triglycerides may create a clearer picture of those effects, the overall data around whether the two omega-3 fatty acids have differential effects on other cardiovascular risk factors is still inconsistent, but generally speaking there is a signal that DHA has a stronger impact than EPA. However, this updated systematic review is constrained by the small number of high quality RCTs that directly compare EPA to DHA and report on outcomes other than blood lipids. Therefore, there is a need for additional high-quality research to assess the independent effects of EPA and DHA on a range of cardiovascular risk factors (e.g., inflammation, blood pressure, vascular function, platelet function) in larger and more diverse study populations.
Data availability statement
The original contributions presented in the study are included in the article/Supplementary material, further inquiries can be directed to the corresponding author.
Author contributions
GC: Data curation, Formal analysis, Investigation, Methodology, Writing – original draft. PC: Conceptualization, Project administration, Supervision, Writing – review & editing.
Funding
The author(s) declare that no financial support was received for the research, authorship, and/or publication of this article.
Conflict of interest
The authors declare that the research was conducted in the absence of any commercial or financial relationships that could be construed as a potential conflict of interest.
The author(s) declared that they were an editorial board member of Frontiers, at the time of submission. This had no impact on the peer review process and the final decision.
Publisher’s note
All claims expressed in this article are solely those of the authors and do not necessarily represent those of their affiliated organizations, or those of the publisher, the editors and the reviewers. Any product that may be evaluated in this article, or claim that may be made by its manufacturer, is not guaranteed or endorsed by the publisher.
Supplementary material
The Supplementary material for this article can be found online at: https://www.frontiersin.org/articles/10.3389/fnut.2024.1423228/full#supplementary-material
References
1. Li, Y, Cao, GY, Jing, WZ, Liu, J, and Liu, M. Global trends and regional differences in incidence and mortality of cardiovascular disease, 1990-2019: findings from 2019 global burden of disease study. Eur J Prev Cardiol. (2023) 30:276–86. doi: 10.1093/eurjpc/zwac285
2. AbuMweis, S, Jew, S, Tayyem, R, and Agraib, L. Eicosapentaenoic acid and docosahexaenoic acid containing supplements modulate risk factors for cardiovascular disease: a meta-analysis of randomized placebo-control human clinical trials. J Hum Nutr Diet. (2018) 31:67–84. doi: 10.1111/jhn.12493
3. Innes, JK, and Calder, PC. Marine omega-3 (n-3) fatty acids for cardiovascular health: an update for 2020. Int J Mol Sci. (2020) 21:1362. doi: 10.3390/ijms21041362
4. Djuricic, I, and Calder, PC. Pros and cons of long-chain omega-3 polyunsaturated fatty acids in cardiovascular health. Annu Rev Pharmacol Toxicol. (2023) 63:383–406. doi: 10.1146/annurev-pharmtox-051921-090208
5. Bernasconi, AA, Wiest, MM, Lavie, CJ, Milani, RV, and Laukkanen, JA. Effect of omega-3 dosage on cardiovascular outcomes: an updated meta-analysis and meta-regression of interventional trials. Mayo Clin Proc. (2021) 96:304–13. doi: 10.1016/j.mayocp.2020.08.034
6. Eslick, GD, Howe, PR, Smith, C, Priest, R, and Bensoussan, A. Benefits of fish oil supplementation in hyperlipidemia: a systematic review and meta-analysis. Int J Cardiol. (2009) 136:4–16. doi: 10.1016/j.ijcard.2008.03.092
7. Miller, PE, Van Elswyk, M, and Alexander, DD. Long-chain omega-3 fatty acids eicosapentaenoic acid and docosahexaenoic acid and blood pressure: a meta-analysis of randomized controlled trials. Am J Hypertens. (2014) 27:885–96. doi: 10.1093/ajh/hpu024
8. Lin, N, Shi, JJ, Li, YM, Zhang, XY, Chen, Y, Calder, PC, et al. What is the impact of n-3 PUFAs on inflammation markers in type 2 diabetic mellitus populations?: a systematic review and meta-analysis of randomized controlled trials. Lipids Health Dis. (2016) 15:133. doi: 10.1186/s12944-016-0303-7
9. O’Mahoney, LL, Matu, J, Price, OJ, Birch, KM, Ajjan, RA, Farrar, D, et al. Omega-3 polyunsaturated fatty acids favourably modulate cardiometabolic biomarkers in type 2 diabetes: a meta-analysis and meta-regression of randomized controlled trials. Cardiovasc Diabetologia. (2018) 17:98. doi: 10.1186/s12933-018-0740-x
10. Li, K, Huang, T, Zheng, J, Wu, K, and Li, D. Effect of marine-derived n-3 polyunsaturated fatty acids on C-reactive protein, interleukin 6 and tumor necrosis factor alpha: a meta-analysis. PLoS One. (2014) 9:e88103. doi: 10.1371/journal.pone.0088103
11. Hidayat, K, Yang, J, Zhang, Z, Chen, GC, Qin, LQ, Eggersdorfer, M, et al. Effect of omega-3 long-chain polyunsaturated fatty acid supplementation on heart rate: a meta-analysis of randomized controlled trials. Eur J Clin Nutr. (2018) 72:805–17. doi: 10.1038/s41430-017-0052-3
12. Mozaffarian, D, Geelen, A, Brouwer, IA, Geleijnse, JM, Zock, PL, and Katan, MB. Effect of fish oil on heart rate in humans: a meta-analysis of randomized controlled trials. Circulation. (2005) 112:1945–52. doi: 10.1161/CIRCULATIONAHA.105.556886
13. Xin, W, Wei, W, and Li, X. Effect of fish oil supplementation on fasting vascular endothelial function in humans: a meta-analysis of randomized controlled trials. PLoS One. (2012) 7:e46028. doi: 10.1371/journal.pone.0046028
14. Wang, Q, Liang, X, Wang, L, Lu, X, Huang, J, Cao, J, et al. Effect of omega-3 fatty acids supplementation on endothelial function: a meta-analysis of randomized controlled trials. Atherosclerosis. (2012) 221:536–43. doi: 10.1016/j.atherosclerosis.2012.01.006
15. Pase, MP, Grima, NA, and Sarris, J. Do long-chain n-3 fatty acids reduce arterial stiffness? A meta-analysis of randomised controlled trials. Br J Nutr. (2011) 106:974–80. doi: 10.1017/S0007114511002819
16. Gao, L, Cao, J, Mao, QX, Lu, XX, Zhou, XL, and Fan, L. Influence of omega-3 polyunsaturated fatty acid-supplementation on platelet aggregation in humans: a meta-analysis of randomized controlled trials. Atherosclerosis. (2013) 226:328–34. doi: 10.1016/j.atherosclerosis.2012.10.056
17. Balk, EM, Lichtenstein, AH, Chung, M, Kupelnick, B, Chew, P, and Lau, J. Effects of omega-3 fatty acids on serum markers of cardiovascular disease risk: a systematic review. Atherosclerosis. (2006) 189:19–30. doi: 10.1016/j.atherosclerosis.2006.02.012
18. Innes, JK, and Calder, PC. The differential effects of eicosapentaenoic acid and docosahexaenoic acid on cardiometabolic risk factors: a systematic review. Int J Mol Sci. (2018) 19:532. doi: 10.3390/ijms19020532
19. Grimsgaard, S, Bonaa, KH, Hansen, JB, and Nordøy, A. Highly purified eicosapentaenoic acid and docosahexaenoic acid in humans have similar triacylglycerol-lowering effects but divergent effects on serum fatty acids. Am J Clin Nutr. (1997) 66:649–59. doi: 10.1093/ajcn/66.3.649
20. Grimsgaard, S, Bønaa, KH, Hansen, JB, and Myhre, ES. Effects of highly purified eicosapentaenoic acid and docosahexaenoic acid on hemodynamics in humans. Am J Clin Nutr. (1998) 68:52–9. doi: 10.1093/ajcn/68.1.52
21. Mori, TA, Bao, DQ, Burke, V, Puddey, IB, and Beilin, LJ. Docosahexaenoic acid but not eicosapentaenoic acid lowers ambulatory blood pressure and heart rate in humans. Hypertension. (1999) 34:253–60. doi: 10.1161/01.HYP.34.2.253
22. Mori, TA, Watts, GF, Burke, V, Hilme, E, Puddey, IB, and Beilin, LJ. Differential effects of eicosapentaenoic acid and docosahexaenoic acid on vascular reactivity of the forearm microcirculation in hyperlipidemic, overweight men. Circulation. (2000a) 102:1264–9. doi: 10.1161/01.CIR.102.11.1264
23. Mori, TA, Burke, V, Puddey, IB, Watts, GF, O'Neal, DN, Best, JD, et al. Purified eicosapentaenoic and docosahexaenoic acids have differential effects on serum lipids and lipoproteins, LDL particle size, glucose, and insulin in mildly hyperlipidemic men. Am J Clin Nutr. (2000b) 71:1085–94. doi: 10.1093/ajcn/71.5.1085
24. Mori, TA, Puddey, IB, Burke, V, Croft, KD, Dunstan, DW, Rivera, JH, et al. Effect of omega 3 fatty acids on oxidative stress in humans: GC-MS measurement of urinary F2-isoprostane excretion. Redox Rep. (2000c) 5:45–6. doi: 10.1179/rer.2000.5.1.45
25. Mas, E, Woodman, RJ, Burke, V, Puddey, IB, Beilin, LJ, Durand, T, et al. The omega-3 fatty acids EPA and DHA decrease plasma F(2)-isoprostanes: results from two placebo-controlled interventions. Free Radic Res. (2010) 44:983–90. doi: 10.3109/10715762.2010.492830
26. Woodman, RJ, Mori, TA, Burke, V, Puddey, IB, Watts, GF, and Beilin, LJ. Effects of purified eicosapentaenoic and docosahexaenoic acids on glycemic control, blood pressure, and serum lipids in type 2 diabetic patients with treated hypertension. Am J Clin Nutr. (2002) 76:1007–15. doi: 10.1093/ajcn/76.5.1007
27. Woodman, RJ, Mori, TA, Burke, V, Puddey, IB, Barden, A, Watts, GF, et al. Effects of purified eicosapentaenoic acid and docosahexaenoic acid on platelet, fibrinolytic and vascular function in hypertensive type 2 diabetic patients. Atherosclerosis. (2003a) 166:85–93. doi: 10.1016/S0021-9150(02)00307-6
28. Woodman, RJ, Mori, TA, Burke, V, Puddey, IB, Watts, GF, Best, JD, et al. Docosahexaenoic acid but not eicosapentaenoic acid increases LDL particle size in treated hypertensive type 2 diabetic patients. Diabetes Care. (2003b) 26:253. doi: 10.2337/diacare.26.1.253
29. Mori, TA, Woodman, RJ, Burke, V, Puddey, IB, Croft, KD, and Beilin, LJ. Effect of eicosapentaenoic acid and docosahexaenoic acid on oxidative stress and inflammatory markers in treated-hypertensive type 2 diabetic subjects. Free Radic Biol Med. (2003) 35:772–81. doi: 10.1016/S0891-5849(03)00407-6
30. Nestel, P, Shige, H, Pomeroy, S, Cehun, M, Abbey, M, and Raederstorff, D. The n-3 fatty acids eicosapentaenoic acid and docosahexaenoic acid increase systemic arterial compliance in humans. Am J Clin Nutr. (2002) 76:326–30. doi: 10.1093/ajcn/76.2.326
31. Park, Y, and Harris, W. EPA, but not DHA, decreases mean platelet volume in normal subjects. Lipids. (2002) 37:941–6. doi: 10.1007/s11745-006-0984-1
32. Park, Y, and Harris, WS. Omega-3 fatty acid supplementation accelerates chylomicron triglyceride clearance. J Lipid Res. (2003) 44:455–63. doi: 10.1194/jlr.M200282-JLR200
33. Park, Y, Jones, PG, and Harris, WS. Triacylglycerol-rich lipoprotein margination: a potential surrogate for whole-body lipoprotein lipase activity and effects of eicosapentaenoic and docosahexaenoic acids. Am J Clin Nutr. (2004) 80:45–50. doi: 10.1093/ajcn/80.1.45
34. Allaire, J, Couture, P, Leclerc, M, Charest, A, Marin, J, Lépine, MC, et al. A randomized, crossover, head-to-head comparison of eicosapentaenoic acid and docosahexaenoic acid supplementation to reduce inflammation markers in men and women: the comparing EPA to DHA (ComparED) study. Am J Clin Nutr. (2016) 104:280–7. doi: 10.3945/ajcn.116.131896
35. Allaire, J, Harris, WS, Vors, C, Charest, A, Marin, J, Jackson, KH, et al. Supplementation with high-dose docosahexaenoic acid increases the Omega-3 index more than high-dose eicosapentaenoic acid. Prostaglandins Leukot Essent Fatty Acids. (2017) 120:8–14. doi: 10.1016/j.plefa.2017.03.008
36. Vors, C, Allaire, J, Marin, J, Lépine, MC, Charest, A, Tchernof, A, et al. Inflammatory gene expression in whole blood cells after EPA vs. DHA supplementation: results from the ComparED study. Atherosclerosis. (2017) 257:116–22. doi: 10.1016/j.atherosclerosis.2017.01.025
37. Calder, PC . Omega-3 polyunsaturated fatty acids and inflammatory processes: nutrition or pharmacology? Br J Clin Pharmacol. (2013) 75:645–62. doi: 10.1111/j.1365-2125.2012.04374.x
38. Wang, T, Zhang, X, Zhou, N, Shen, Y, Li, B, Chen, BE, et al. Association between omega-3 fatty acid intake and dyslipidemia: a continuous dose-response meta-analysis of randomized controlled trials. J Am Heart Assoc. (2023) 12:e029512. doi: 10.1161/JAHA.123.029512
39. Zhang, X, Ritonja, JA, Zhou, N, Chen, BE, and Li, X. Omega-3 polyunsaturated fatty acids intake and blood pressure: a dose-response meta-analysis of randomized controlled trials. J Am Heart Assoc. (2022) 11:e025071. doi: 10.1161/JAHA.121.025071
40. Page, MJ, McKenzie, JE, Bossuyt, PM, Boutron, I, Hoffmann, TC, Mulrow, CD, et al. The PRISMA 2020 statement: an updated guideline for reporting systematic reviews. BMJ. (2021) 372:n71. doi: 10.1136/bmj.n71
41. Jadad, AR, Moore, RA, Carroll, D, Jenkinson, C, Reynolds, DJM, Gavaghan, DJ, et al. Assessing the quality of reports of randomized clinical trials: is blinding necessary? Control Clin Trials. (1996) 17:1–12. doi: 10.1016/0197-2456(95)00134-4
42. Sterne, JAC, Savović, J, Page, MJ, Elbers, RG, Blencowe, NS, Boutron, I, et al. RoB 2: a revised tool for assessing risk of bias in randomised trials. BMJ. (2019) 366:l4898. doi: 10.1136/bmj.l4898
43. Allaire, J, Vors, C, Tremblay, AJ, Marin, J, Charest, A, Tchernof, A, et al. High-dose DHA has more profound effects on LDL-related features than high-dose EPA: the ComparED study. J Clin Endocrinol Metab. (2018) 103:2909–17. doi: 10.1210/jc.2017-02745
44. Allaire, J, Vors, C, Harris, WS, Jackson, KH, Tchernof, A, Couture, P, et al. Comparing the serum TAG response to high-dose supplementation of either DHA or EPA among individuals with increased cardiovascular risk: the ComparED study. Br J Nutr. (2019) 121:1223–34. doi: 10.1017/S0007114519000552
45. Klingel, SL, Metherel, AH, Irfan, M, Rajna, A, Chabowski, A, Bazinet, RP, et al. EPA and DHA have divergent effects on serum triglycerides and lipogenesis, but similar effects on lipoprotein lipase activity: a randomized controlled trial. Am J Clin Nutr. (2019) 110:1502–9. doi: 10.1093/ajcn/nqz234
46. Lee, JB, Notay, K, Klingel, SL, Chabowski, A, Mutch, DM, and Millar, PJ. Docosahexaenoic acid reduces resting blood pressure but increases muscle sympathetic outflow compared with eicosapentaenoic acid in healthy men and women. Am J Physiol Heart Circ Physiol. (2019) 316:H873–81. doi: 10.1152/ajpheart.00677.2018
47. So, J, Wu, D, Lichtenstein, AH, Tai, AK, Matthan, NR, Maddipati, KR, et al. EPA and DHA differentially modulate monocyte inflammatory response in subjects with chronic inflammation in part via plasma specialized pro-resolving lipid mediators: a randomized, double-blind, crossover study. Atherosclerosis. (2021) 316:90–8. doi: 10.1016/j.atherosclerosis.2020.11.018
48. So, J, Asztalos, BF, Horvath, K, and Lamon-Fava, S. Ethyl EPA and ethyl DHA cause similar and differential changes in plasma lipid concentrations and lipid metabolism in subjects with low-grade chronic inflammation. J Clin Lipidol. (2022) 16:887–94. doi: 10.1016/j.jacl.2022.10.002
49. Pisaniello, AD, Psaltis, PJ, King, PM, Liu, G, Gibson, RA, Tan, JT, et al. Omega-3 fatty acids ameliorate vascular inflammation: a rationale for their atheroprotective effects. Atherosclerosis. (2021) 324:27–37. doi: 10.1016/j.atherosclerosis.2021.03.003
50. Bhatt, DL, Steg, PG, Miller, M, Brinton, EA, Jacobson, TA, Ketchum, SB, et al. Cardiovascular risk reduction with icosapent ethyl for hypertriglyceridemia. N Engl J Med. (2019) 380:11–22. doi: 10.1056/NEJMoa1812792
51. Hu, Y, Hu, FB, and Manson, JE. Marine omega-3 supplementation and cardiovascular disease: an updated meta-analysis of 13 randomized controlled trials involving 127,477 participants. J Am Heart Assoc. (2019) 8:e013543. doi: 10.1161/JAHA.119.013543
52. Mozaffarian, D, and Wu, JH. (n-3) fatty acids and cardiovascular health: are effects of EPA and DHA shared or complementary? J Nutr. (2012) 142:614S–25S. doi: 10.3945/jn.111.149633
53. Kelsey, MD, and Pagidipati, NJ. Should we "RESPECT EPA" more now? EPA and DHA for cardiovascular risk reduction. Curr Cardiol Rep. (2023) 25:1601–9. doi: 10.1007/s11886-023-01972-w
54. Shearer, GC, Savinova, OV, and Harris, WS. Fish oil — how does it reduce plasma triglycerides? Biochim Biophys Acta. (2012) 1821:843–51. doi: 10.1016/j.bbalip.2011.10.011
55. Peterson, AS, Fong, LG, and Young, SG. PCSK9 function and physiology. J Lipid Res. (2008) 49:1152–6. doi: 10.1194/jlr.E800008-JLR200
56. Calder, PC . Marine omega-3 fatty acids and inflammatory processes: effects, mechanisms and clinical relevance. Biochim Biophys Acta Mol Cell Biol Lipids. (2015) 1851:469–84. doi: 10.1016/j.bbalip.2014.08.010
57. Calder, PC . N-3 PUFA and inflammation: from membrane to nucleus and from bench to bedside. Proc Nutr Soc. (2020) 79:404–16. doi: 10.1017/S0029665120007077
58. Serhan, CN . Discovery of specialized pro-resolving mediators marks the dawn of resolution physiology and pharmacology. Mol Asp Med. (2017) 58:1–11. doi: 10.1016/j.mam.2017.03.001
59. Chiang, N, and Serhan, CN. Structural elucidation and physiologic functions of specialized pro-resolving mediators and their receptors. Mol Asp Med. (2017) 58:114–29. doi: 10.1016/j.mam.2017.03.005
60. Calder, PC . Eicosapentaenoic and docosahexaenoic acid derived specialised pro-resolving mediators: concentrations in humans and the effects of age, sex, disease and increased omega-3 fatty acid intake. Biochimie. (2020) 178:105–23. doi: 10.1016/j.biochi.2020.08.015
61. Glass, CK, and Witztum, JL. Atherosclerosis: the road ahead. Cell. (2001) 104:503–16. doi: 10.1016/S0092-8674(01)00238-0
62. Hansson, GK . Inflammation, atherosclerosis, and coronary artery disease. N Engl J Med. (2005) 352:1685–95. doi: 10.1056/NEJMra043430
63. Harris, WS, Rambjør, GS, Windsor, SL, and Diederich, D. N-3 fatty acids and urinary excretion of nitric oxide metabolites in humans. Am J Clin Nutr. (1997) 65:459–64. doi: 10.1093/ajcn/65.2.459
64. Kenny, D, Warltier, DC, Pleuss, JA, Hoffmann, RG, Goodfriend, TL, and Egan, BM. Effect of omega-3 fatty acids on the vascular response to angiotensin in normotensive men. Am J Cardiol. (1992) 70:1347–52. doi: 10.1016/0002-9149(92)90773-R
65. Chin, JP, Gust, AP, Nestel, PJ, and Dart, AM. Marine oils dose-dependently inhibit vasoconstriction of forearm resistance vessels in humans. Hypertension. (1993) 21:22–8. doi: 10.1161/01.HYP.21.1.22
66. McVeigh, GE, Brennan, GM, Cohn, JN, Finkelstein, SM, Hayes, RJ, and Johnston, GD. Fish oil improves arterial compliance in non-insulin-dependent diabetes mellitus. Arterioscler Thromb. (1994) 14:1425–9. doi: 10.1161/01.ATV.14.9.1425
67. DiNicolantonio, JJ, and O’Keefe, J. The benefits of marine omega-3s for preventing arrhythmias. Open. Heart. (2020) 7:e000904. doi: 10.1136/openhrt-2018-000904
69. Lawson, JA, Rokach, J, and FitzGerald, GA. Isoprostanes: formation, analysis and use as indices of lipid peroxidation in vivo. J Biol Chem. (1999) 274:24441–4. doi: 10.1074/jbc.274.35.24441
70. Chowdhury, R, Warnakula, S, Kunutsor, S, Crowe, F, Ward, HA, Johnson, L, et al. Association of dietary, circulating, and supplement fatty acids with coronary risk: a systematic review and meta-analysis. Ann Intern Med. (2014) 160:398–406. doi: 10.7326/M13-1788
71. Alexander, DD, Miller, PE, Van Elswyk, ME, Kuratko, CN, and Bylsma, LC. A meta-analysis of randomized controlled trials and prospective cohort studies of eicosapentaenoic and docosahexaenoic long-chain omega-3 fatty acids and coronary heart disease risk. Mayo Clin Proc. (2017) 92:15–29. doi: 10.1016/j.mayocp.2016.10.018
72. Del Gobbo, LC, Imamura, F, Aslibekyan, S, Marklund, M, Virtanen, JK, Wennberg, M, et al. ω-3 Polyunsaturated fatty acid biomarkers and coronary heart disease: pooling project of 19 cohort studies. JAMA Intern Med. (2016) 176:1155–66. doi: 10.1001/jamainternmed.2016.2925
73. Harris, WS, Del Gobbo, L, and Tintle, NL. The Omega-3 index and relative risk for coronary heart disease mortality: estimation from 10 cohort studies. Atherosclerosis. (2017) 262:51–4. doi: 10.1016/j.atherosclerosis.2017.05.007
74. Harris, WS, Tintle, NL, Imamura, F, Qian, F, Korat, AVA, Marklund, M, et al. Fatty acids and outcomes research consortium (FORCE). Blood n-3 fatty acid levels and total and cause-specific mortality from 17 prospective studies. Nat Commun. (2021) 12:2329. doi: 10.1038/s41467-021-22370-2
75. Scientific Advisory Committee on Nutrition and Committee on Toxicology . Advice on fish consumption: Benefits and risks. London: TSO (2004).
76. International Society for the Study of Fatty Acids and Lipids . Recommendations for intake of polyunsaturated fatty acids in healthy adults - policy statement. Washington, DC: ISSFAL (2004).
77. Food and Agricultural Organisation of the United Nations . Fat and fatty acids in human nutrition: Report of an expert consultation. Rome: FAO (2010).
78. European Food Safety Authority . Scientific opinion on dietary reference values for fats, including saturated fatty acids, polyunsaturated fatty acids, monounsaturated fatty acids, trans fatty acids and cholesterol. EFSA J. (2010) 8:1461. doi: 10.2903/j.efsa.2010.1507
79. Manson, JE, Cook, NR, Lee, IM, Christen, W, Bassuk, SS, Mora, S, et al. Marine n-3 fatty acids and prevention of cardiovascular disease and cancer. N Engl J Med. (2019) 380:23–32. doi: 10.1056/NEJMoa1811403
80. ASCEND Study Collaborative Group . Effects of n-3 fatty acid supplements in diabetes mellitus. N Engl J Med. (2018) 379:1540–50. doi: 10.1056/NEJMoa1804989
81. GISSI-Prevenzione Investigators dietary supplementation with n-3 polyunsaturated fatty acids and vitamin E after myocardial infarction: results of the GISSI-Prevenzione trial. Lancet. (1999) 354:447–55. doi: 10.1016/S0140-6736(99)07072-5
82. Tavazzi, L, Maggioni, AP, Marchioli, R, Barlera, S, Franzosi, MG, Latini, R, et al. Effect of n-3 polyunsaturated fatty acids in patients with chronic heart failure (the GISSI-HF trial): a randomised, double-blind, placebo-controlled trial. Lancet. (2008) 372:1223–30. doi: 10.1016/S0140-6736(08)61239-8
83. Yokoyama, M, Origasa, H, Matsuzaki, M, Matsuzawa, Y, Saito, Y, Ishikawa, Y, et al. Japan EPA lipid intervention study (JELIS) Investigators. Effects of eicosapentaenoic acid on major coronary events in hypercholesterolaemic patients (JELIS): a randomised open-label, blinded endpoint analysis. Lancet. (2007) 369:1090–8. doi: 10.1016/S0140-6736(07)60527-3
84. Budoff, MJ, Bhatt, DL, Kinninger, A, Lakshmanan, S, Muhlestein, JB, Le, VT, et al. Effect of icosapent ethyl on progression of coronary atherosclerosis in patients with elevated triglycerides on statin therapy: final results of the EVAPORATE trial. Eur Heart J. (2020) 41:3925–32. doi: 10.1093/eurheartj/ehaa652
85. ORIGIN Trial Investigators . N-3 fatty acids and cardiovascular outcomes in patients with dysglycemia. N Engl J Med. (2012) 367:309–18. doi: 10.1056/NEJMoa1203859
86. Risk and Prevention Study Collaborative Group . N-3 fatty acids in patients with multiple cardiovascular risk factors. N Engl J Med. (2013) 368:1800–8. doi: 10.1056/NEJMoa1205409
87. Nicholls, SJ, Lincoff, AM, Garcia, M, Bash, D, Ballantyne, CM, Barter, PJ, et al. Effect of high-dose omega-3 fatty acids versus corn oil on major adverse cardiovascular events in patients at high cardiovascular risk: the STRENGTH randomized clinical trial. JAMA. (2020) 324:2268–80. doi: 10.1001/jama.2020.22258
88. Kalstad, AA, Myhre, PL, Laake, K, Tveit, SH, Schmidt, EB, Nilsen, DWT, et al. Effects of n-3 fatty acid supplements in elderly patients after myocardial infarction: a randomized, controlled trial. Circulation. (2021) 143:528–39. doi: 10.1161/CIRCULATIONAHA.120.052209
89. Sharma, G, Martin, SS, and Bluementhal, RS. Effects of omega-3 fatty acids on major adverse cardiovascular events: what matters most: the drug, the dose, or the placebo? JAMA. (2020) 324:2262–4. doi: 10.1001/jama.2020.22387
Keywords: blood lipids, triglycerides, cholesterol, blood pressure, inflammation
Citation: Choi GY and Calder PC (2024) The differential effects of eicosapentaenoic acid and docosahexaenoic acid on cardiovascular risk factors: an updated systematic review of randomized controlled trials. Front. Nutr. 11:1423228. doi: 10.3389/fnut.2024.1423228
Edited by:
Emma Derbyshire, Nutritional Insight Limited, United KingdomReviewed by:
Marika Massaro, National Research Council (CNR), ItalyBenjamin D. Horne, Intermountain Healthcare, United States
Copyright © 2024 Choi and Calder. This is an open-access article distributed under the terms of the Creative Commons Attribution License (CC BY). The use, distribution or reproduction in other forums is permitted, provided the original author(s) and the copyright owner(s) are credited and that the original publication in this journal is cited, in accordance with accepted academic practice. No use, distribution or reproduction is permitted which does not comply with these terms.
*Correspondence: Philip C. Calder, pcc@soton.ac.uk