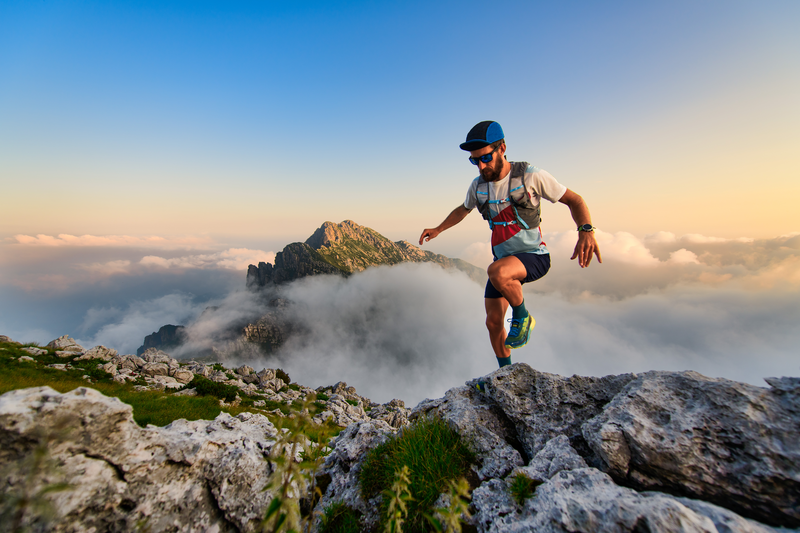
95% of researchers rate our articles as excellent or good
Learn more about the work of our research integrity team to safeguard the quality of each article we publish.
Find out more
REVIEW article
Front. Nutr. , 18 September 2024
Sec. Nutrition and Microbes
Volume 11 - 2024 | https://doi.org/10.3389/fnut.2024.1420358
In this review, we explore the effects of food additives on intestinal health. Food additives, such as preservatives, antioxidants and colorants, are widely used to improve food quality and extend shelf life. However, their effects on intestinal microecology May pose health risks. Starting from the basic functions of food additives and the importance of intestinal microecology, we analyze in detail how additives affect the diversity of intestinal flora, oxidative stress and immune responses. Additionally, we examine the association between food additives and intestinal disorders, including inflammatory bowel disease and irritable bowel syndrome, and how the timing, dosage, and individual differences affect the body’s response to additives. We also assess the safety and regulatory policies of food additives and explore the potential of natural additives. Finally, we propose future research directions, emphasizing the refinement of risk assessment methods and the creation of safer, innovative additives.
Intestinal health is crucial for overall human well-being, acting as a cornerstone of physiological health (1). Food additives, used to enhance food quality, flavor, prevent microbial contamination, and extend shelf life, are increasingly utilized due to shifts in dietary patterns and advancements in food processing technologies (2). Food additives encompass a wide variety, including thickeners, colorants, sweeteners, emulsifiers, as well as flavors and seasonings (2). While the U.S. Food and Drug Administration (FDA) has deemed food additives relatively safe, many still pose potential health risks to humans (3, 4). For instance, non-caloric artificial sweeteners are among the most widely used food additives globally. Epidemiological studies and animal experiments suggest that long-term consumption of non-caloric sweeteners, such as aspartame, saccharin, and sucralose, can disrupt hormone levels, leading to impaired glucose tolerance in both experimental animals and healthy volunteers. These sweeteners also alter the expression of reward-related genes in the brains of offspring, exacerbating obesity and impaired glucose tolerance during adolescence (5).
The widespread use of food additives has raised health concerns, particularly regarding their effects on the gastrointestinal tract (4). Although early studies have identified some links between food additives and intestinal diseases, their findings are often fragmented and fail to offer a comprehensive view of how these additives affect intestinal microecology, oxidative stress, and immune responses. Moreover, research is limited on how various types, dosages, and exposure times of food additives affect intestinal health, and how individual differences May modulate these effects.
This paper aims to elucidate the complex relationships between food additives, intestinal flora, and intestinal health, with a particular focus on their links to intestinal diseases. Additionally, it assesses the safety of food additives and the role of food safety regulation in maintaining intestinal health, providing the public, researchers, and policymakers with a comprehensive perspective and deeper understanding of these relationships. It also aims to provide scientific evidence for the development of more effective public health policies and food safety standards, as well as to guide future research directions.
The gastrointestinal (GI) tract hosts a diverse array of microbial populations (6). The intestinal microbial community consists of a diverse assembly of microorganisms—including bacteria, fungi, protozoa, and viruses—that colonize the intestinal tract (7). The major phyla of the intestinal microbiota include Bacteroidetes, Firmicutes, Clostridia, Proteobacteria, Cyanobacteria, Verrucomicrobia, and Actinobacteria (8). The healthy state of the intestinal microbiota significantly impacts overall health; however, food additives May alter the composition and function of the intestinal microbiota through various pathways. The interaction between food additives and intestinal microbiota is complex, exhibiting both beneficial and detrimental effects. In the following sections, we will describe the effects of various food additives on intestinal microbiota separately.
Preservatives, a prevalent category of food additives, possess antimicrobial properties designed to inhibit harmful bacteria, thereby extending the shelf life of food (9). Research indicates that certain antimicrobial preservatives can trigger abnormalities in glucose tolerance and interfere with the intestinal microbial community, negatively impacting intestinal health (10). Propionate, a type of preservative, can alter microbial composition and metabolism (11). Propionate is also a type of short-chain fatty acid (SCFA) that can modulate intestinal inflammation through immunomodulation and the regulation of the intestinal barrier (11). However, in patients with intestinal inflammation who are deficient in SCFAs, propionate supplementation can be beneficial (12).
Antioxidants play a critical role in preserving food freshness; however, they May also negatively impact the intestinal ecosystem. Polyphenols, a subgroup of antioxidants, are metabolized by intestinal microorganisms, thus influencing the diversity and activity of the intestinal microbiota (13). Studies have shown that polyphenols can alter the intestinal environment by modulating the metabolites of the intestinal microbiota, such as SCFAs, gasses, enzymes, etc., thereby affecting the composition and function of the microbiota. Research indicates that polyphenols May inhibit the growth of certain beneficial microorganisms, particularly lactic acid bacteria and some probiotics (14, 15), and that these inhibitory or bactericidal effects depend on the specific polyphenol structure and bacterial species. Indeed, preservatives can negatively impact the intestinal microbiota even when used within regulatory limits. For instance, certain food preservatives (e.g., benzoic acid, propionate) can reduce the diversity of the intestinal microbiota and induce mild intestinal inflammation (16).
Flavor enhancers, which include various substances, are used to improve the taste profiles of foods. The most commonly used flavor enhancer is monosodium glutamate (MSG), the sodium salt of glutamic acid (17). Xu et al. (18) investigated the intestinal structure and microbiota of mice orally administered MSG via tube feeding. The results indicated that moderate intake of MSG can increase the ratio of Bacteroidetes to Firmicutes and enhance the abundance of probiotic bacteria, promoting intestinal development and regulating the intestinal microbiota composition. However, excessive intake of MSG can reduce the abundance of certain microbiota, such as bifidobacteria (19). Conversely, Peng et al. (20) observed that MSG consumption did not significantly alter the structural or functional characteristics of the intestinal microbial community in the subjects, which May be attributed to the ingested amount or the physiological characteristics of the organism.
Artificial sweeteners, such as aspartame, acesulfame, sucralose, saccharin, neotame, and their derivatives, are commonly found in sugar-free beverages, confectioneries, and dairy products (21). Recent research has highlighted alterations in the intestinal microbiota and metabolites attributed to these sweeteners (22). Specifically, chronic low-dose aspartame consumption in mice has been shown to increase the populations of Clostridium leptum and Enterobacteriaceae (23). Additionally, aspartame enhances the adhesion, invasion, and epithelial lethality of Escherichia coli NCTC10418 and Enterococcus faecalis ATCC19433 (24). Studies by Palmnäs and Nettleton reported that aspartame intake elevates serum, fecal, and cecal concentrations of propionic and butyric acids, indicating alterations in the metabolic processing within the intestinal microbiota (5, 23). Acesulfame intake has demonstrated significant gender-specific effects, with females experiencing reduced levels of Lactobacillus and Clostridium but increased Mucispirillum, whereas males exhibited increased Bacteroides, Sutterella, and Anaerostipes (25). In pregnant and lactating mice, acesulfame intake was linked to increased levels of Bacillota and decreased levels of the potentially anti-inflammatory Akkermansia muciniphila (26). Contrasting studies on sucralose indicate no impact on intestinal microorganisms after short-term consumption (27). However, prolonged intake over 10 weeks in young individuals has been linked to increased Blautia coccoides and decreased Lactobacillus acidophilus, along with broader disturbances in intestinal flora and fluctuations in serum insulin and glucose levels (28). Elevated concentrations of saccharin in cecal contents are associated with increased aerobic flora (29), and exposure to saccharin has led to changes in intestinal microbiota composition and function, potentially promoting glucose intolerance (30). Saccharin also increases populations of Ackermannia, Corynebacterium, and Turicibacter, while decreasing Anaerostipes, Ruminococcus, and Dorea (31). However, it has been noted to inhibit bacterial growth and mitigate experimental colitis in mice (32). Lastly, neotame intake caused a sharp decline in Firmicutes and an increase in the abundance of Bacteroides, particularly Bacteroides, with significant reductions in various members of the Trichoderma and Ruminalococcaceae families, including Blautia, Dorea, Oscillospira, and Ruminalococcus spp (33).
Sugar alcohols are widely used in the production of chemicals, pharmaceuticals, and foods, including erythritol, xylitol, lactitol, and sorbitol (34). Erythritol has been found to increase intestinal metabolites such as butyric and valeric acids, though it does not significantly alter the structure of the intestinal microbiota (35). Conversely, xylitol May shift the rodent intestinal microbial population from Gram-negative to Gram-positive bacteria (36) and has been shown to decrease the relative abundance of Proteobacteria, Bacteroidetes, and Actinobacteria, while increasing the abundance of Bacillota and the ratio of Bacillota to Bacteroidetes in mice (37–39). Furthermore, Chen et al. (40) reported that lactitol intake significantly boosts the populations of bifidobacteria and lactobacilli while reducing the presence of Clostridium perfringens in patients with chronic viral hepatitis, noting that lactitol more effectively reduces plasma endotoxin levels than standard drug therapy. Other studies have shown that lactitol positively affects fecal flora and May alleviate symptoms such as constipation, thereby classifying it as a prebiotic (41, 42). Sorbitol is known to increase bacterial density and acetate synthesis (43). However, Li et al. (44) observed that chronic intake of sorbitol significantly decreased the relative abundance of various beneficial bacteria, including Bifidobacterium and several Lachnospiraceae members, while increasing the presence of pathogenic bacteria such as H. pylori and Prevotella, which subsequently induced glucose intolerance in mice.
Additionally, azo dyes commonly added to beverages like milk tea and ice cream, including lemon yellow, sunset yellow, and camosin (45), are metabolized by certain intestinal microorganisms such as Anabaena ovale and Enterococcus faecalis, which produce azoreductases. These enzymes convert azo dyes into the sodium salt of 1-amino-2-naphthol-6-sulfonate, a metabolite known to cause colitis (46). In their study, Wu et al. (47) used crucian carp (Carassius auratus) as an experimental model to assess the effects of lemon-yellow consumption. They demonstrated significant alterations in the intestinal microbial community of the fish, with a decrease in beneficial probiotics such as Roseomonas, Rhodococcus, and Bacillus, and an increase in pathogenic microorganisms like Vibrio vermiculiformis and Schizosaccharomyces cerevisiae, which negatively impacting fish health.
Emulsifiers, including carboxymethyl cellulose (CMC) and polysorbate 80 (P80), have been associated with a heightened prevalence of intestinal inflammation-related disorders, such as inflammatory bowel disease and metabolic syndrome. This association is attributed to the emulsifiers’ capacity to directly alter the intestinal microbiota, thereby promoting inflammation (48). Chassaing et al. (49) observed that healthy adults consuming a diet enriched with 15 grams per day of CMC exhibited changes in the fecal metabolome, notably a reduction in SCFAs and free amino acids, compared to a control group. Furthermore, two subjects in the CMC group demonstrated increased microbial invasion into the normally sterile inner mucus layer—a hallmark of intestinal inflammation—and significant shifts in microbiota composition (49). Moreover, carrageenan consumption was found to increase the presence of phyla such as Proteobacteria and Deferribacteres, while reducing Firmicutes, Actinobacteria and Bacteroidetes (50).
Conversely, not all food additives pose health risks. The International Scientific Association for Probiotics and Prebiotics (ISAPP) acknowledges prebiotics for their health-enhancing effects, emphasizing their selective use by the host’s intestinal flora to generate health-promoting metabolites such as SCFAs (51). These metabolites not only inhibit pathogen growth but also activate immune responses, helping to prevent infections and allergies (51, 52). As a prebiotic, galacto-oligosaccharides (GOS) have been shown to positively impact intestinal health, particularly in immunomodulation and the improvement of IBD. A high-purity GOS product, NeoGOS-P70, has been shown to effectively alleviate DSS-induced colitis in mice, reducing symptoms and colon shortening (53).
Ultimately, food additives exert a complex array of effects on intestinal microbiota, encompassing both potential risks and benefits. Prolonged exposure to specific food additives May disrupt microbiota structure and function, potentially leading to ecological imbalances and diseases such as ulcerative colitis and Crohn’s disease (54). Moreover, antimicrobial food additives May diminish sensitive bacterial populations while increasing others, thus altering the diversity and stability of the intestinal microbial community (55). These changes can impact the host’s immune system and overall health. However, the potential benefits of additives like propionate, monosodium glutamate, and lactitol for intestinal health cannot be overlooked. Future research ought to investigate further the precise effects of various food additives on intestinal flora and their implications for human health, with the goal of optimizing their use to enhance rather than undermine intestinal health (Table 1).
Intestinal oxidative stress occurs when the production of oxidants exceeds the antioxidant scavenging capacity within the intestinal tract, potentially leading to pathologies such as inflammation and cancer (56). Food additives May exacerbate this condition by increasing oxidant levels or impairing antioxidant functions, consequently compromising intestinal barrier integrity.
To commence, direct interactions between food additives and intestinal cells can elevate levels of reactive oxygen species (ROS) and reactive nitrogen species (RNS). For example, fructose has been shown to promote intestinal permeability through ethanol-induced cytochrome P450-2E1-mediated oxidative and nitrative stress, increasing inducible nitric oxide synthase (iNOS) in rat plasma, thereby exacerbating oxidative stress (57). Prolonged exposure to titanium dioxide (E171) induces oxidative stress and DNA base oxidation in intestinal epithelial cells (58). Additionally, diets rich in maltodextrin (MDX) disrupt the intestinal mucus barrier, induce endoplasmic reticulum stress, and heighten inflammatory responses in these cells (59). Dietary emulsifiers May also indirectly increase oxidative stress by diminishing the protective mucosal layer and promoting the growth of pro-inflammatory microbiota (60).
Subsequently, oxidative stress damages intestinal cells and disrupts mucosal barrier integrity, enhancing permeability and allowing harmful antigens to penetrate, which leads to systemic inflammation (61). Piglets on a fructose-rich diet showed reduced tight junction gene expression and altered microbiota, leading to barrier dysfunction and inflammation (62). Research also suggests that high concentrations of artificial sweeteners, such as aspartame and saccharin, can trigger apoptosis and enhance epithelial barrier permeability, disturbing tight junctions and fostering ROS production (63).
Furthermore, some food additives impair intestinal antioxidant defenses. For instance, lemon yellow exposure in crucian carp has been linked to reduced activity of key antioxidant enzymes like CAT, SOD, and GSH-Px, thereby promoting oxidative stress (64). Conversely, in a mouse model of colitis, Pingyin rose essential oil (PREO) was found to enhance the enzymatic activities of SOD and catalase (CAT), while reducing NO, malondialdehyde (MDA) and myeloperoxidase (MPO) production and restoring intestinal barrier integrity (65).
Overall, the impacts of food additives on intestinal oxidative stress are diverse, encompassing direct boosts in oxidant production, disturbance of intestinal barrier function, and impairment of intestinal antioxidant defense mechanisms. The combined effect of these actions May compromise intestinal health and increase the risk of chronic intestinal diseases. Therefore, understanding how food additives influence intestinal oxidative stress mechanisms is crucial for assessing and managing the safety of these additives (Figure 1).
Figure 1. Food additives alter physiological mechanisms such as oxidative stress and intestinal immunity by affecting intestinal microbiota. First, food additives can change the composition and metabolism of the intestinal flora. Second, food additives May exacerbate intestinal oxidative stress by increasing the production of oxidants or inhibiting antioxidant defense mechanisms. Finally, food additives can also affect the intestinal immune response by disrupting the intestinal mucosal barrier, changing the composition of the intestinal flora, and directly interfering with immune cell function.
The intestinal tract, the body’s largest immune organ, plays a critical role in maintaining the balance of the intestinal microbial community and safeguarding against harmful microorganisms (66). Diet substantially influences this balance and directly affects intestinal and immune homeostasis (67). Hence, understanding the mechanisms by which food additives affect intestinal immune responses is vital for assessing their safety. Food additives influence the intestinal immune system by disrupting the mucosal barrier, altering microbial composition, and modulating immune cell responses.
To commence, food additives compromise the intestinal mucosal barrier, the primary defense against pathogens and allergens (68). Chassaing et al. (60) showed that emulsifiers enhance the abundance of Ruminicoccus gnavus and Akkermansia muciniphila, while thinning the mucus layer, alterations that correspond with heightened intestinal permeability and increased levels of lipopolysaccharide (LPS) and circulating flagellin in mice. LPS is a major bacterial endotoxin tencountered by the immune system, and flagellin is a potent immune activator that triggers responses during infections (69). Additionally, TiO2 nanoparticles in additives are linked to inflammatory intestinal damage, which attributed to diminished mucus barrier function and elevated metabolite lipopolysaccharides, activating inflammatory pathways (70).
Subsequently, the intestinal microbial community, essential for both human immunity and intestinal health, can be significantly altered by food additives (71). Research has indicated that polyphenols can inhibit the growth of beneficial microorganisms, especially lactic acid bacteria and specific probiotics, with the impact contingent on the polyphenols’ structure and the bacterial species (14, 15). Furthermore, Li et al. (44) found that long-term sorbitol consumption markedly decreased the abundance of beneficial bacteria such as Bifidobacterium and increased levels of pathogenic bacteria such as H. pylori, leading to glucose intolerance in mice.
Furthermore, beyond affecting the intestinal barrier and microbial composition, food additives directly interfere with immune cell function. For example, high salt intake alters helper T cell function and promotes inflammation, potentially contributing to conditions such hypertension and obesity (72). High doses of titanium dioxide (TiO2) increase the production of inflammatory cytokines like IL-8, TNF-α, and IL-10 by macrophages, compromising their phagocytic activity, and disturbing the balance of dendritic and regulatory T cells (73, 74). Moreover, high fructose corn syrup (HFCS) disrupts intestinal flora, leading to a decrease in secondary bile acids and an imbalance in Th17/Treg cells, which can exacerbate intestinal inflammation (75) (Figure 1).
Firstly, food additives have been implicated in the escalation of the risk of inflammatory bowel disease (IBD). IBD is a chronic, progressive, immune-mediated inflammatory disease of the gastrointestinal tract characterized by complex pathogenesis involving environmental factors, homeostatic dysregulation, oxidative stress, and immune dysregulation (76). Food additives May increase the risk of IBD by influencing these factors. For instance, a high salt diet (HSD) was shown to exacerbate colitis in an IL-10-deficient mouse model and infectious colitis scenarios (77). This effect is attributed to HSD’s enhancement of pro-inflammatory cytokine expression, thereby identifying high dietary salt intake as a critical environmental factor in IBD inflammation (77). Aguiar et al. (78) also demonstrated that dietary salt causes intestinal inflammation, potentially by increasing intestinal permeability. Moreover, maltodextrin consumption has been linked to intestinal dysbiosis and heightened disease susceptibility, a recent study found that maltodextrin in drinking water exacerbated the inflammatory response in an intestinal disease model through mechanisms involving endoplasmic reticulum stress and mucin depletion (59). Carrageenan, a polysaccharide commonly used as a food additive, has been associated with increased inflammatory indices in patients with chronic intake, such as elevated IL-6 and fecal calreticulin (79). Dietary emulsifiers have been shown to alter intestinal microbiota composition, promote intestinal hyperpermeability, and increase inflammatory markers such as fecal lipid transport protein-2 in IL-10-deficient mice (60).
Furthermore, food additives contribute to the heightened risk of intestinal infections. TiO2, commonly used as a whitening agent in various food products, has been observed to exacerbate IBD and contribute to the development of intestinal infections in animal studies (80). The stability of the intestinal microbial community is crucial for resisting infections, and food additives can disrupt this balance, heightening infection risk (81). Benzoic acid, widely used as an antimicrobial preservative in food and feed (82), has shown benefits for growth and health by promoting intestinal functions such as digestion, absorption, and barrier function (83). However, overexposure to benzoic acid can result in toxicity and serious health and growth impairments in humans and animals, affecting organs like the liver, spleen, and lungs, and altering the intestinal mucosa, thereby increasing infection risks (84).
In addition, food additives further amplify the risk of intestinal tumors. The development of intestinal tumors, including colorectal cancer (CRC), is closely linked to factors such as intestinal flora imbalance, oxidative stress, and immune abnormalities (85, 86). Dysbiosis of intestinal flora, promoted by food additives, can enhance CRC progression through mechanisms involving inflammation, pathogenic bacteria and their virulence factors, oxidative stress, bacterial metabolites, and biofilms (87). Emulsifiers May foster intestinal and metabolic diseases by disrupting microbial community structures, impairing mucosal barriers, facilitating bacterial translocation, and triggering inflammation. These processes contribute to immune imbalances and elevated levels of circulating bacteria, significantly increasing the risk of neoplastic diseases (88). Regular consumption of dietary emulsifiers like carboxymethylcellulose or polysorbate-80 has been shown to induce microbiome alterations and low-grade inflammation that promote colon carcinogenesis (89). Additionally, emulsifiers exacerbate the development of spontaneous intestinal adenomas by affecting the proliferative state of the intestinal epithelium (90). Triclosan (TCS), an antimicrobial additive, modulates intestinal microbiota and TLR4 signaling, increasing colonic inflammation and colitis-associated colon tumorigenesis in mice (91).
Summarizing, the connection between food additives and intestinal diseases entails a multifaceted interplay among intestinal flora dysbiosis, compromised barrier function, and inflammation. While the convenience of processed foods is significant, their consumption poses potential risks to intestinal health. Moving forward, future research must concentrate on unraveling the mechanisms through which food additives impact intestinal disease development and on formulating dietary guidelines aimed at reducing these risks (Figure 2).
Figure 2. First of all, food additives aggravate the occurrence of inflammatory bowel disease by increasing the expression of pro-inflammatory cytokines, changing intestinal flora, improving intestinal permeability and other mechanisms. Secondly, food additives May impair the barrier function of the intestinal tract by disrupting the homeostasis of the intestinal microbiome, thereby increasing the risk of intestinal infections. Finally, food additives May increase the risk of intestinal tumors through the pathway of intestinal flora imbalance, oxidative stress and abnormal immunity.
The impact of food additives on intestinal diseases is determined by their type, dose, and duration of exposure. This section explores these factors in detail.
Initially, various types of food additives have been linked to different intestinal diseases. Dietary emulsifiers, for instance, May exacerbate inflammatory bowel disease (IBD) by promoting the growth of pro-inflammatory intestinal microbiota, disrupting intestinal mucus structure, increasing intestinal permeability, activating inflammatory pathways, and disrupting the cell cycle (4). Sweeteners like high fructose corn syrup (HFCS) are known to enhance intestinal tumor growth in mice, while aspartame and saccharin May elevate the incidence and recurrence of Crohn’s disease by affecting the growth of beneficial bacteria (92, 93). Food preservatives such as sulfites can induce an imbalance in biothiol levels in NCM460 colon cells, triggering intestinal inflammation in mice (94). Chronic exposure to synthetic food colorants, like Allura Red AC, exacerbates colitis models in mice through colonic 5-HT in both intestinal flora-dependent and independent pathways (95). Thickeners, such as carrageenan, have also been associated with inflammatory bowel disease (96), and xanthan gum-containing thickeners have been linked to necrotizing small bowel colitis in infants (97).
Subsequently, the dosage of food additives significantly influences intestinal disorders. For example, a study evaluating different doses of MSG on intestinal function in mice set up groups at 30 mg/kg, 300 mg/kg, and 1,500 mg/kg. The study found that a lower dose (30 mg/kg) promoted the growth of intestinal villi and enhanced absorption functions, whereas a high dose (1,500 mg/kg) decreased the villi-to-crypt ratio and increased albumin leakage, indicating impaired intestinal barrier function (18). Additionally, Yang et al. (98) found that sorbitol concentrations were significantly higher in the feces of patients with active IBD compared to those in remission or healthy controls. Higher daily consumption of lemon yellow in carp correlated with more severe histopathological changes in the intestinal tract and increased pro-inflammatory cytokines (47). Katsoudas et al.’s analysis (99) revealed that total emulsifier exposure was significantly higher in patients with inflammatory bowel disease than in healthy controls.
Furthermore, the duration of exposure to food additives also affects intestinal health. Xu et al. (100) observed that by day 120, nitrite exposure in C57BL/6 mice led to a significant increase in alpha-diversity, as indicated by higher Chao 1 and Shannon indices, and a greater number of distinct genera compared to day 70. Chronic nitrite exposure decreased the abundance of Elusimicrobium and Akkermansia, microorganisms linked to reduced obesity and inflammation (101).
In conclusion, the type, dose, and duration of exposure to food additives collectively influence intestinal health. This underscores the need to consider these factors in research, along with individual differences such as genetic background, dietary habits, and lifestyle, which can influence responses to food additives.
Individual differences play a crucial role in understanding the interaction between food additives and intestinal diseases. This underscores the need to consider these factors in research, along with individual differences such as genetic background, dietary habits, and lifestyle, which can influence responses to food additives.
Firstly, genetic factors are pivotal in determining individual responses to food additives. Specific genetic variants, such as single nucleotide polymorphisms (SNPs), significantly influence the composition and function of intestinal microbes (102), which, in turn, affect the metabolism of food additives and their impact on intestinal health. For instance, the presence of the bacterium Akkermansia muciniphila has been shown to mitigate the harmful effects of dietary emulsifiers on the microbiota and host metabolism, preventing low-grade inflammatory responses and metabolic dysregulation, thus supporting intestinal health (103). Zou et al. (104) highlighted the protective role of intestinal bacteria in mitigating the adverse effects of food and drug additives on the intestinal tract.
Subsequently, lifestyle variations, particularly in terms of exercise habits, sleep patterns, and dietary habits, profoundly influence the composition of intestinal flora, which, in turn, affects an individual’s response to food additives. For example, exercise-focused dietary strategies such as protein supplementation and carbohydrate loading have been shown to benefit the intestinal microbiota of athletes and enhance intestinal barrier function (105). Moderate exercise also promotes beneficial changes in the composition of the intestinal microbiota and the microbial metabolites produced in the gastrointestinal tract, potentially reducing intestinal permeability (106). Voigt et al. (107) reported that disturbances in circadian rhythm are associated with dysbiosis of the intestinal flora. Thus, maintaining a consistent sleep and rest routine is vital for preserving the homeostasis of the intestinal flora, enhancing resistance to the negative impacts of food additives. Moreover, food composition and dietary habits are key determinants of the intestinal microbial community (108). A study indicated that deprivation of dietary fiber compromises the colonic mucus barrier and disrupts intestinal barrier function in mice (109).
In conclusion, individual differences in response to food additives and their impact on intestinal health are influenced by genetics, lifestyle, and dietary habits. Future research should further investigate how these factors contribute to individual variances and explore strategies to mitigate the adverse effects of food additives on intestinal health.
Safety and risk assessment are foundational in the research and application of food additives. This section aims to provide an overview of the current methodologies in safety assessment and recent research findings, offering a scientific foundation for food safety management.
The safety assessment process for food additives typically includes identifying and quantifying potentially hazardous components, evaluating human exposure levels, and conducting risk analyses based on toxicological data (110). This multifaceted process integrates various factors such as the type of food additive, dosage, exposure duration, and individual differences. Advanced technologies enable rapid screening of additives in food products (111), and comprehensive databases of food ingredients have been developed to facilitate this process (112).
Recent risk assessment outcomes indicate that many food additives are safe at current usage levels. However, a meta-analysis identified that some artificial sweeteners May increase cancer risk in European populations (113). Accordingly, it is recommended that further long-term safety studies be conducted on such additives, and usage guidelines be revised as necessary.
The global food supply chain’s complexity and the diversifying types of food additives pose increasing challenges to safety assessments. International food safety organizations, such as the Joint Expert Committee on Food Additives (JECFA) of the World Health Organization (WHO) and the Food and Agriculture Organization (FAO), play critical roles in setting global food safety standards (114). Looking ahead, enhancing safety assessments for food additives will require strengthened international collaboration, greater transparency, and increased public involvement in risk assessments. Moreover, leveraging emerging technologies to improve the accuracy and efficiency of these assessments will be crucial.
In response to the potential risks associated with synthetic food additives, researchers are actively exploring alternatives, including natural additives. Promoting and applying these safer alternatives supports public intestinal health protection.
Natural colorants are substances derived from natural plants, commonly including anthocyanins and betaines (115, 116). These colorants not only possess coloring properties but also exhibit various biological activities beneficial to health (115). Anthocyanins, found in the flowers and fruits of many plants, color most red, purple, and blue botanicals (117). Beyond coloring, anthocyanin-rich plants are utilized in treating various diseases due to their anti-inflammatory, anticancer, and cardioprotective properties. Additionally, anthocyanins help regulate cholesterol, LDL, HDL, triglycerides, and mitigate neurological and cognitive alterations (118). Betaine, sourced from beets, spinach, and grains, displays antioxidant, antimicrobial, and anticancer properties (119). Its antioxidant capability is linked to its chemical structure, containing phenolic groups and cyclic amines, which effectively combat free radicals (120). This highlights natural colorants’ superior safety and nutritional value.
Traditional preservatives, some of which can be chemically hazardous, are being replaced due to potential adverse effects when intake exceeds safe limits (121). As food safety demands increase, the industry is shifting toward natural preservatives, including antimicrobials and antioxidants derived from natural sources (122). Natural antimicrobials, secondary metabolites from plants (herbs, spices), animals (eggs, milk), and microorganisms (fungi, bacteria), exhibit antimicrobial properties (123, 124) and are increasingly used in food applications due to their disease-control effects (125). Common natural antioxidants like polyphenols, vitamins, and carotenoids, known for their potent antioxidant capacity (126), are employed in treating aging, cancer, diabetes, inflammation, liver disease, cardiovascular issues, and neurodegenerative diseases due to their favorable safety profiles (127).
Despite the benefits, natural additives face significant application challenges. Extracting and maintaining the stability and activity of these ingredients, especially on a large scale, poses major technical and cost challenges. Additionally, the viability and efficacy of certain natural additives, such as probiotics, May be limited in certain conditions, necessitating further research to optimize their formulation and application conditions. Despite these hurdles, the development and application of natural additives hold substantial potential. Future research should focus on enhancing the stability, efficacy, and cost-effectiveness of these additives, ensuring their suitability across various food systems. Further clinical and epidemiological studies are crucial to fully assess the long-term impacts of natural additives on human health, particularly regarding intestinal health.
Unhealthy diets and diet-related non-communicable diseases (NCDs) can increase societal costs indirectly and directly, placing a significant burden on healthcare systems and society at large (128). Therefore, government bodies must strengthen the regulation of food production, processing, and distribution, and strictly monitor the use of food additives to ensure food safety and hygiene.
The essence of regulatory policies is the safety assessment of food additives, which involves evaluating their potential toxicity, estimating exposure, and conducting risk assessments for specific populations, such as children and pregnant women (129, 130). In recent years, there has been a heightened focus on the effects of food additives on intestinal health. Numerous regulatory policies and laws have been enacted to improve food regulatory frameworks to minimize the impact of food additives on both intestinal and systemic health (131). Moreover, preventing intestinal diseases, including food poisoning and intestinal infections, is a crucial aspect of food safety regulation (132). These diseases are often closely linked to food contamination and the misuse of food additives. Strengthening the training and management of food service employees to enhance their food safety awareness and operational skills can help prevent the occurrence of enteric diseases at the source.
Despite strict regulatory policies, numerous challenges persist in practice. The complexity of the global food supply chain necessitates greater international cooperation and information sharing to effectively regulate food additives. Additionally, as new food technologies and novel additives rapidly develop, regulatory frameworks must continually evolve to accommodate these changes. Future regulatory policy development will require more evidence-based research, particularly in evaluating the long-term effects of food additives on intestinal health. The integration of emerging technologies, such as high-throughput screening and artificial intelligence, could enhance the efficiency and accuracy of food additive assessments. Moreover, enhancing consumer education and raising public awareness about the safety of food additives is a vital component of future regulatory strategies.
In this review, we examined the influence of food additives on intestinal health, focusing on their effects on intestinal microecology, their association with intestinal oxidative stress and immunity, and their implications for intestinal diseases. Our comprehensive evaluation of existing literature reveals that food additives play a significant role in intestinal health, with both potential risks and benefits. Certain additives May adversely affect intestinal health by disrupting the intestinal barrier, altering the balance of intestinal microecology, and promoting oxidative stress and inflammation. Conversely, some additives May have positive effects under specific conditions, such as providing antioxidant protection or promoting the growth of beneficial intestinal microorganisms. These findings underscore the need for individualized assessment of the safety and benefits of food additives, considering both their potential risks and advantages.
Looking ahead, we propose several directions for future research and action. First, there is a need to improve risk assessment methods, developing more accurate models and techniques to evaluate the safety of food additives under real-life use scenarios. This includes utilizing high-throughput screening techniques, advanced in vitro and in vivo modeling, and computational simulations to predict the long-term effects of food additive intake on human health. Second, we encourage the development of new, safer additives, particularly natural food additives with lower toxicity and better health benefits. Special emphasis should be placed on additives that support intestinal health, such as prebiotics and natural antioxidants with anti-inflammatory properties. Third, regulatory policies should be continually updated to reflect emerging evidence on the effects of food additives, ensuring effective protection of public health. This includes raising public awareness about food additive safety. We recommend that consumers carefully read food labels and prioritize products with safe, natural additives. Researchers should conduct in-depth clinical and population studies to verify laboratory findings and develop safe alternatives. Policymakers should base regulatory decisions on the latest scientific evidence and promote international cooperation in food safety standards. Through these concerted efforts, we can enhance our understanding of food additives’ impact on intestinal health, develop safer and more effective additives, and ultimately achieve both food safety and public health goals.
HW: Writing – original draft. JB: Funding acquisition, Writing – original draft. PM: Writing – original draft. YW: Writing – original draft. XC: Writing – original draft. HL: Writing – original draft. YQ: Writing – original draft. MZ: Writing – original draft. YL: Writing – original draft. RT: Funding acquisition, Supervision, Writing – original draft, Writing – review & editing. XY: Funding acquisition, Writing – review & editing.
The author(s) declare that financial support was received for the research, authorship, and/or publication of this article. The authors thank the financial support provided by Project of Science and Technology Plan of Sichuan Province (2019YFS0187, 2018JY0091).
We thank all the authors for their contribution to the article.
The authors declare that the research was conducted in the absence of any commercial or financial relationships that could be construed as a potential conflict of interest.
All claims expressed in this article are solely those of the authors and do not necessarily represent those of their affiliated organizations, or those of the publisher, the editors and the reviewers. Any product that may be evaluated in this article, or claim that may be made by its manufacturer, is not guaranteed or endorsed by the publisher.
1. Song, M, Chan, AT, and Sun, J. Influence of the gut microbiome, diet, and environment on risk of colorectal cancer. Gastroenterology. (2020) 158:322–40. doi: 10.1053/j.gastro.2019.06.048
2. Wu, L, Zhang, C, Long, Y, Chen, Q, Zhang, W, and Liu, G. Food additives: from functions to analytical methods. Crit Rev Food Sci Nutr. (2022) 62:8497–517. doi: 10.1080/10408398.2021.1929823
3. Lempart-Rapacewicz, A, Kudlek, E, Brukało, K, Rapacewicz, R, Lempart, Ł, and Dudziak, M. The threat of food additive occurrence in the environment-a case study on the example of swimming pools. Foods (Basel, Switzerland). (2023) 12:1188. doi: 10.3390/foods12061188
4. Bancil, AS, Sandall, AM, Rossi, M, Chassaing, B, Lindsay, JO, and Whelan, K. Food additive emulsifiers and their impact on gut microbiome, permeability, and inflammation: mechanistic insights in inflammatory bowel disease. J Crohns Colitis. (2021) 15:1068–79. doi: 10.1093/ecco-jcc/jjaa254
5. Nettleton, JE, Cho, NA, Klancic, T, Nicolucci, AC, Shearer, J, Borgland, SL, et al. Maternal low-dose aspartame and stevia consumption with an obesogenic diet alters metabolism, gut microbiota and mesolimbic reward system in rat dams and their offspring. Gut. (2020) 69:1807–17. doi: 10.1136/gutjnl-2018-317505
6. Sekirov, I, Russell, SL, Antunes, LC, and Finlay, BB. Gut microbiota in health and disease. Physiol Rev. (2010) 90:859–904. doi: 10.1152/physrev.00045.2009
7. Weinstock, GM. Genomic approaches to studying the human microbiota. Nature. (2012) 489:250–6. doi: 10.1038/nature11553
8. Inan-Eroglu, E, Ayaz, AJN, and Science, F. Effects of food additives on gut microbiota: friend or foe? Nutr Food Sci. (2019) 49:955–64. doi: 10.1108/NFS-02-2019-0049
9. Bensid, A, El Abed, N, Houicher, A, Regenstein, JM, and Özogul, F. Antioxidant and antimicrobial preservatives: properties, mechanism of action and applications in food - a review. Crit Rev Food Sci Nutr. (2022) 62:2985–3001. doi: 10.1080/10408398.2020.1862046
10. Li, P, Li, M, Wu, T, Song, Y, Li, Y, Huang, X, et al. Systematic evaluation of antimicrobial food preservatives on glucose metabolism and gut microbiota in healthy mice. NPJ science of food. (2022) 6:42. doi: 10.1038/s41538-022-00158-y
11. Parada Venegas, D, De la Fuente, MK, Landskron, G, González, MJ, Quera, R, Dijkstra, G, et al. Short chain fatty acids (SCFAs)-mediated gut epithelial and immune regulation and its relevance for inflammatory bowel diseases. Front Immunol. (2019) 10:277. doi: 10.3389/fimmu.2019.00277
12. Gill, PA, van Zelm, MC, Muir, JG, and Gibson, PR. Review article: short chain fatty acids as potential therapeutic agents in human gastrointestinal and inflammatory disorders. Aliment Pharmacol Ther. (2018) 48:15–34. doi: 10.1111/apt.14689
13. Makarewicz, M, Drożdż, I, Tarko, T, and Duda-Chodak, A. The interactions between polyphenols and microorganisms, Especially gut microbiota. Antioxidants (Basel, Switzerland). (2021) 10:188. doi: 10.3390/antiox10020188
14. Puupponen-Pimiä, R, Nohynek, L, Meier, C, Kähkönen, M, Heinonen, M, Hopia, A, et al. Antimicrobial properties of phenolic compounds from berries. J Appl Microbiol. (2001) 90:494–507. doi: 10.1046/j.1365-2672.2001.01271.x
15. Cueva, C, Moreno-Arribas, MV, Martín-Alvarez, PJ, Bills, G, Vicente, MF, Basilio, A, et al. Antimicrobial activity of phenolic acids against commensal, probiotic and pathogenic bacteria. Res Microbiol. (2010) 161:372–82. doi: 10.1016/j.resmic.2010.04.006
16. Hrncirova, L, Machova, V, Trckova, E, Krejsek, J, and Hrncir, T. Food preservatives induce Proteobacteria dysbiosis in human-microbiota associated Nod2-deficient mice. Microorganisms. (2019) 7:383. doi: 10.3390/microorganisms7100383
17. Chakraborty, SP. Patho-physiological and toxicological aspects of monosodium glutamate. Toxicol Mech Methods. (2019) 29:389–96. doi: 10.1080/15376516.2018.1528649
18. Xu, J, Tang, M, Liu, Y, Xu, J, Xu, XJFS, and Wellness, H. Safety assessment of monosodium glutamate based on intestinal function and flora in mice. Food Sci Human Wellness. (2022) 11:155–64. doi: 10.1016/j.fshw.2021.07.016
19. Nahok, K, Phetcharaburanin, J, Li, JV, Silsirivanit, A, Thanan, R, Boonnate, P, et al. Monosodium glutamate induces changes in hepatic and renal metabolic profiles and gut microbiome of Wistar rats. Nutrients. (2021) 13:1865. doi: 10.3390/nu13061865
20. Peng, Q, Huo, D, Ma, C, Jiang, S, Wang, L, and Zhang, J. Monosodium glutamate induces limited modulation in gut microbiota. J Funct Foods. (2018) 49:493–500. doi: 10.1016/j.jff.2018.09.015
21. Wilk, K, Korytek, W, Pelczyńska, M, Moszak, M, and Bogdański, P. The effect of artificial sweeteners use on sweet taste perception and weight loss efficacy: a review. Nutrients. (2022) 14:1261. doi: 10.3390/nu14061261
22. Suez, J, Cohen, Y, Valdés-Mas, R, Mor, U, Dori-Bachash, M, Federici, S, et al. Personalized microbiome-driven effects of non-nutritive sweeteners on human glucose tolerance. Cell. (2022) 185:3307–28.e19. doi: 10.1016/j.cell.2022.07.016
23. Palmnäs, MS, Cowan, TE, Bomhof, MR, Su, J, Reimer, RA, Vogel, HJ, et al. Low-dose aspartame consumption differentially affects gut microbiota-host metabolic interactions in the diet-induced obese rat. PLoS One. (2014) 9:e109841. doi: 10.1371/journal.pone.0109841
24. Shil, A, and Chichger, H. Artificial sweeteners negatively regulate pathogenic characteristics of two model gut bacteria, E. coli and E. faecalis. Int J Mol Sci. (2021) 22:5228. doi: 10.3390/ijms22105228
25. Bian, X, Chi, L, Gao, B, Tu, P, Ru, H, and Lu, K. The artificial sweetener acesulfame potassium affects the gut microbiome and body weight gain in CD-1 mice. PLoS One. (2017) 12:e0178426. doi: 10.1371/journal.pone.0178426
26. Olivier-Van Stichelen, S, Rother, KI, and Hanover, JA. Maternal exposure to non-nutritive sweeteners impacts Progeny's metabolism and microbiome. Front Microbiol. (2019) 10:1360. doi: 10.3389/fmicb.2019.01360
27. Thomson, P, Santibañez, R, Aguirre, C, Galgani, JE, and Garrido, D. Short-term impact of sucralose consumption on the metabolic response and gut microbiome of healthy adults. Br J Nutr. (2019) 122:856–62. doi: 10.1017/S0007114519001570
28. Méndez-García, LA, Bueno-Hernández, N, Cid-Soto, MA, De León, KL, Mendoza-Martínez, VM, Espinosa-Flores, AJ, et al. Ten-week sucralose consumption induces gut dysbiosis and altered glucose and insulin levels in healthy young adults. Microorganisms. (2022) 10:434. doi: 10.3390/microorganisms10020434
29. Anderson, RL, and Kirkland, JJ. The effect of sodium saccharin in the diet on caecal microflora. Food Cosmet Toxicol. (1980) 18:353–5. doi: 10.1016/0015-6264(80)90188-1
30. Suez, J, Korem, T, Zeevi, D, Zilberman-Schapira, G, Thaiss, CA, Maza, O, et al. Artificial sweeteners induce glucose intolerance by altering the gut microbiota. Nature. (2014) 514:181–6. doi: 10.1038/nature13793
31. Bian, X, Tu, P, Chi, L, Gao, B, Ru, H, and Lu, K. Saccharin induced liver inflammation in mice by altering the gut microbiota and its metabolic functions. Food Chem Toxicol. (2017) 107:530–9. doi: 10.1016/j.fct.2017.04.045
32. Sünderhauf, A, Pagel, R, Künstner, A, Wagner, AE, Rupp, J, Ibrahim, SM, et al. Saccharin supplementation inhibits bacterial growth and reduces experimental colitis in mice. Nutrients. (2020) 12:1122. doi: 10.3390/nu12041122
33. Chi, L, Bian, X, Gao, B, Tu, P, Lai, Y, Ru, H, et al. Effects of the artificial sweetener neotame on the gut microbiome and fecal metabolites in mice. Molecules (Basel, Switzerland). (2018) 23:367. doi: 10.3390/molecules23020367
34. Liang, P, Cao, M, Li, J, Wang, Q, and Dai, Z. Expanding sugar alcohol industry: microbial production of sugar alcohols and associated chemocatalytic derivatives. Biotechnol Adv. (2023) 64:108105. doi: 10.1016/j.biotechadv.2023.108105
35. Mahalak, KK, Firrman, J, Tomasula, PM, Nuñez, A, Lee, JJ, Bittinger, K, et al. Impact of Steviol glycosides and Erythritol on the human and Cebus apella gut microbiome. J Agric Food Chem. (2020) 68:13093–101. doi: 10.1021/acs.jafc.9b06181
36. Tamura, M, Hoshi, C, and Hori, S. Xylitol affects the intestinal microbiota and metabolism of daidzein in adult male mice. Int J Mol Sci. (2013) 14:23993–4007. doi: 10.3390/ijms141223993
37. Kong, F, Kang, S, Zhang, J, Zhao, H, Peng, Y, Yang, M, et al. Whey protein and xylitol complex alleviate type 2 diabetes in C57BL/6 mice by regulating the intestinal microbiota. Food Res Int (Ottawa, ON). (2022) 157:111454. doi: 10.1016/j.foodres.2022.111454
38. Uebanso, T, Kano, S, Yoshimoto, A, Naito, C, Shimohata, T, Mawatari, K, et al. Effects of consuming xylitol on gut microbiota and lipid metabolism in mice. Nutrients. (2017) 9:756. doi: 10.3390/nu9070756
39. Zuo, QL, Cai, X, Zheng, XY, Chen, DS, Li, M, Liu, ZQ, et al. Influences of xylitol consumption at different dosages on intestinal tissues and gut microbiota in rats. J Agric Food Chem. (2021) 69:12002–11. doi: 10.1021/acs.jafc.1c03720
40. Chen, C, Li, L, Wu, Z, Chen, H, and Fu, S. Effects of lactitol on intestinal microflora and plasma endotoxin in patients with chronic viral hepatitis. J Infect. (2007) 54:98–102. doi: 10.1016/j.jinf.2005.11.013
41. Li, XQ, Zhang, XM, Wu, X, Lan, Y, Xu, L, Meng, XC, et al. Beneficial effects of lactitol on the composition of gut microbiota in constipated patients. J Dig Dis. (2020) 21:445–53. doi: 10.1111/1751-2980.12912
42. Finney, M, Smullen, J, Foster, HA, Brokx, S, and Storey, DM. Effects of low doses of lactitol on faecal microflora, pH, short chain fatty acids and gastrointestinal symptomology. Eur J Nutr. (2007) 46:307–14. doi: 10.1007/s00394-007-0666-7
43. Van den Abbeele, P, Poppe, J, Deyaert, S, Laurie, I, Otto Gravert, TK, Abrahamsson, A, et al. Low-no-calorie sweeteners exert marked compound-specific impact on the human gut microbiota ex vivo. Int J Food Sci Nutr. (2023) 74:630–44. doi: 10.1080/09637486.2023.2240037
44. Li, CH, Wang, CT, Lin, YJ, Kuo, HY, Wu, JS, Hong, TC, et al. Long-term consumption of the sugar substitute sorbitol alters gut microbiome and induces glucose intolerance in mice. Life Sci. (2022) 305:120770. doi: 10.1016/j.lfs.2022.120770
45. Barciela, P, Perez-Vazquez, A, and Prieto, MA. Azo dyes in the food industry: features, classification, toxicity, alternatives, and regulation. Food Chem Toxicol. (2023) 178:113935. doi: 10.1016/j.fct.2023.113935
46. Zahran, SA, Ali-Tammam, M, Hashem, AM, Aziz, RK, and Ali, AE. Azoreductase activity of dye-decolorizing bacteria isolated from the human gut microbiota. Sci Rep. (2019) 9:5508. doi: 10.1038/s41598-019-41894-8
47. Wu, L, Xu, Y, Lv, X, Chang, X, Ma, X, Tian, X, et al. Impacts of an azo food dye tartrazine uptake on intestinal barrier, oxidative stress, inflammatory response and intestinal microbiome in crucian carp (Carassius auratus). Ecotoxicol Environ Saf. (2021) 223:112551. doi: 10.1016/j.ecoenv.2021.112551
48. Naimi, S, Viennois, E, Gewirtz, AT, and Chassaing, B. Direct impact of commonly used dietary emulsifiers on human gut microbiota. Microbiome. (2021) 9:66. doi: 10.1186/s40168-020-00996-6
49. Chassaing, B, Compher, C, Bonhomme, B, Liu, Q, Tian, Y, Walters, W, et al. Randomized controlled-feeding study of dietary emulsifier Carboxymethylcellulose reveals detrimental impacts on the gut microbiota and metabolome. Gastroenterology. (2022) 162:743–56. doi: 10.1053/j.gastro.2021.11.006
50. Munyaka, PM, Sepehri, S, Ghia, JE, and Khafipour, E. Carrageenan gum and adherent invasive Escherichia coli in a piglet model of inflammatory bowel disease: impact on intestinal mucosa-associated microbiota. Front Microbiol. (2016) 7:462. doi: 10.3389/fmicb.2016.00462
51. Gibson, GR, Hutkins, R, Sanders, ME, Prescott, SL, Reimer, RA, Salminen, SJ, et al. Expert consensus document: the international scientific Association for Probiotics and Prebiotics (ISAPP) consensus statement on the definition and scope of prebiotics. Nat Rev Gastroenterol Hepatol. (2017) 14:491–502. doi: 10.1038/nrgastro.2017.75
52. Khangwal, I, and Shukla, P. Prospecting prebiotics, innovative evaluation methods, and their health applications: a review. 3 Biotech. (2019) 9:187. doi: 10.1007/s13205-019-1716-6
53. Park, HR, Eom, DH, Kim, JH, Shin, JC, Shin, MS, and Shin, KS. Composition analysis and oral administered effects on dextran sulfate sodium-induced colitis of galactooligosaccharides bioconverted by Bacillus circulans. Carbohydr Polym. (2021) 270:118389. doi: 10.1016/j.carbpol.2021.118389
54. Abiega-Franyutti, P, and Freyre-Fonseca, V. Chronic consumption of food-additives lead to changes via microbiota gut-brain axis. Toxicology. (2021) 464:153001. doi: 10.1016/j.tox.2021.153001
55. Hrncirova, L, Hudcovic, T, Sukova, E, Machova, V, Trckova, E, Krejsek, J, et al. Human gut microbes are susceptible to antimicrobial food additives in vitro. Folia Microbiol. (2019) 64:497–508. doi: 10.1007/s12223-018-00674-z
56. Circu, ML, and Aw, TY. Intestinal redox biology and oxidative stress. Semin Cell Dev Biol. (2012) 23:729–37. doi: 10.1016/j.semcdb.2012.03.014
57. Cho, YE, Kim, DK, Seo, W, Gao, B, Yoo, SH, and Song, BJ. Fructose promotes leaky gut, Endotoxemia, and liver fibrosis through ethanol-inducible cytochrome P450-2E1-mediated oxidative and Nitrative stress. Hepatology (Baltimore, Md). (2021) 73:2180–95. doi: 10.1002/hep.30652
58. Dorier, M, Béal, D, Marie-Desvergne, C, Dubosson, M, Barreau, F, Houdeau, E, et al. Continuous in vitro exposure of intestinal epithelial cells to E171 food additive causes oxidative stress, inducing oxidation of DNA bases but no endoplasmic reticulum stress. Nanotoxicology. (2017) 11:751–61. doi: 10.1080/17435390.2017.1349203
59. Laudisi, F, Di Fusco, D, Dinallo, V, Stolfi, C, Di Grazia, A, Marafini, I, et al. The food additive maltodextrin promotes endoplasmic reticulum stress-driven mucus depletion and exacerbates intestinal inflammation. Cell Mol Gastroenterol Hepatol. (2019) 7:457–73. doi: 10.1016/j.jcmgh.2018.09.002
60. Chassaing, B, Koren, O, Goodrich, JK, Poole, AC, Srinivasan, S, Ley, RE, et al. Dietary emulsifiers impact the mouse gut microbiota promoting colitis and metabolic syndrome. Nature. (2015) 519:92–6. doi: 10.1038/nature14232
61. Di Vincenzo, F, Del Gaudio, A, Petito, V, Lopetuso, LR, and Scaldaferri, F. Gut microbiota, intestinal permeability, and systemic inflammation: a narrative review. Intern Emerg Med. (2024) 19:275–93. doi: 10.1007/s11739-023-03374-w
62. Guo, P, Wang, H, Ji, L, Song, P, and Ma, X. Impacts of fructose on intestinal barrier function, inflammation and microbiota in a piglet model. Nutrients. (2021) 13:3515. doi: 10.3390/nu13103515
63. Shil, A, Olusanya, O, Ghufoor, Z, Forson, B, Marks, J, and Chichger, H. Artificial sweeteners disrupt tight junctions and barrier function in the intestinal epithelium through activation of the sweet taste receptor, T1R3. Nutrients. (2020) 12:1862. doi: 10.3390/nu12061862
64. Wu, L, Lv, X, Zhang, Y, Xin, Q, Zou, Y, and Li, X. Tartrazine exposure results in histological damage, oxidative stress, immune disorders and gut microbiota dysbiosis in juvenile crucian carp (Carassius carassius). Aquat Toxicol (Amsterdam, Netherlands). (2021) 241:105998. doi: 10.1016/j.aquatox.2021.105998
65. Raka, RN, Xiao, J, Wu, H, Lv, W, Ding, Z, Cao, Y, et al. Pingyin rose essential oil restores intestinal barrier integrity in DSS-induced mice colitis model. Food Res Int (Ottawa, ON). (2023) 164:112362. doi: 10.1016/j.foodres.2022.112362
66. Takiishi, T, Fenero, CIM, and Câmara, NOS. Intestinal barrier and gut microbiota: shaping our immune responses throughout life. Tissue Barriers. (2017) 5:e1373208. doi: 10.1080/21688370.2017.1373208
67. Rinninella, E, Raoul, P, Cintoni, M, Franceschi, F, Miggiano, GAD, Gasbarrini, A, et al. What is the healthy gut microbiota composition? A changing ecosystem across age, environment, diet, and diseases. Microorganisms. (2019) 7:14. doi: 10.3390/microorganisms7010014
68. Turner, JR. Intestinal mucosal barrier function in health and disease. Nat Rev Immunol. (2009) 9:799–809. doi: 10.1038/nri2653
69. Hajam, IA, Dar, PA, Shahnawaz, I, Jaume, JC, and Lee, JH. Bacterial flagellin-a potent immunomodulatory agent. Exp Mol Med. (2017) 49:e373. doi: 10.1038/emm.2017.172
70. Yan, J, Wang, D, Li, K, Chen, Q, Lai, W, Tian, L, et al. Toxic effects of the food additives titanium dioxide and silica on the murine intestinal tract: mechanisms related to intestinal barrier dysfunction involved by gut microbiota. Environ Toxicol Pharmacol. (2020) 80:103485. doi: 10.1016/j.etap.2020.103485
71. de Vos, WM, Tilg, H, Van Hul, M, and Cani, PD. Gut microbiome and health: mechanistic insights. Gut. (2022) 71:1020–32. doi: 10.1136/gutjnl-2021-326789
72. Wilck, N, Matus, MG, Kearney, SM, Olesen, SW, Forslund, K, Bartolomaeus, H, et al. Salt-responsive gut commensal modulates T(H)17 axis and disease. Nature. (2017) 551:585–9. doi: 10.1038/nature24628
73. Butler, M, Boyle, JJ, Powell, JJ, Playford, RJ, and Ghosh, S. Dietary microparticles implicated in Crohn's disease can impair macrophage phagocytic activity and act as adjuvants in the presence of bacterial stimuli. Inflam Res. (2007) 56:353–61. doi: 10.1007/s00011-007-7068-4
74. Bettini, S, Boutet-Robinet, E, Cartier, C, Coméra, C, Gaultier, E, Dupuy, J, et al. Food-grade TiO(2) impairs intestinal and systemic immune homeostasis, initiates preneoplastic lesions and promotes aberrant crypt development in the rat colon. Sci Rep. (2017) 7:40373. doi: 10.1038/srep40373
75. Zhou, M, Liu, X, He, J, Xu, X, Ju, C, Luo, S, et al. High-fructose corn syrup aggravates colitis via microbiota dysbiosis-mediated Th17/Treg imbalance. Clin Sci (Lond). (2023) 137:1619–35. doi: 10.1042/CS20230788
76. Flynn, S, and Eisenstein, S. Inflammatory bowel disease presentation and diagnosis. Surg Clin North Am. (2019) 99:1051–62. doi: 10.1016/j.suc.2019.08.001
77. Tubbs, AL, Liu, B, Rogers, TD, Sartor, RB, and Miao, EA. Dietary salt exacerbates experimental colitis. J Immunol (Baltimore, Md: 1950). (2017) 199:1051–9. doi: 10.4049/jimmunol.1700356
78. Aguiar, SLF, Miranda, MCG, Guimarães, MAF, Santiago, HC, Queiroz, CP, Cunha, PDS, et al. High-salt diet induces IL-17-dependent gut inflammation and exacerbates colitis in mice. Front Immunol. (2017) 8:1969. doi: 10.3389/fimmu.2017.01969
79. Bhattacharyya, S, Shumard, T, Xie, H, Dodda, A, Varady, KA, Feferman, L, et al. A randomized trial of the effects of the no-carrageenan diet on ulcerative colitis disease activity. Nutr Healthy Aging. (2017) 4:181–92. doi: 10.3233/NHA-170023
80. Ruiz, PA, Morón, B, Becker, HM, Lang, S, Atrott, K, Spalinger, MR, et al. Titanium dioxide nanoparticles exacerbate DSS-induced colitis: role of the NLRP3 inflammasome. Gut. (2017) 66:1216–24. doi: 10.1136/gutjnl-2015-310297
81. Su, Y, and Ding, T. Targeting microbial quorum sensing: the next frontier to hinder bacterial driven gastrointestinal infections. Gut Microbes. (2023) 15:2252780. doi: 10.1080/19490976.2023.2252780
82. Rouxel, M, Barthe, M, Marchand, P, Juin, C, Mondamert, L, Berges, T, et al. Characterization of antifungal compounds produced by lactobacilli in cheese-mimicking matrix: comparison between active and inactive strains. Int J Food Microbiol. (2020) 333:108798. doi: 10.1016/j.ijfoodmicro.2020.108798
83. Mao, X, Yang, Q, Chen, D, Yu, B, and He, J. Benzoic acid used as food and feed additives can regulate gut functions. Biomed Res Int. (2019) 2019:1–6. doi: 10.1155/2019/5721585
84. Shu, Y, Yu, B, He, J, Yu, J, Zheng, P, Yuan, Z, et al. Excess of dietary benzoic acid supplementation leads to growth retardation, hematological abnormality and organ injury of piglets. Livestock Sci. (2016) 190:94–103. doi: 10.1016/j.livsci.2016.06.010
85. Requena, DO, and Garcia-Buitrago, M. Molecular insights into colorectal carcinoma. Arch Med Res. (2020) 51:839–44. doi: 10.1016/j.arcmed.2020.09.014
86. Jasti, R, and Carucci, LR. Small bowel neoplasms: a pictorial review. Radiographics. (2020) 40:1020–38. doi: 10.1148/rg.2020200011
87. Cheng, Y, Ling, Z, and Li, L. The intestinal microbiota and colorectal Cancer. Front Immunol. (2020) 11:615056. doi: 10.3389/fimmu.2020.615056
88. Partridge, D, Lloyd, KA, Rhodes, JM, Walker, AW, Johnstone, AM, and Campbell, BJ. Food additives: assessing the impact of exposure to permitted emulsifiers on bowel and metabolic health - introducing the FADiets study. Nutr Bull. (2019) 44:329–49. doi: 10.1111/nbu.12408
89. Viennois, E, Merlin, D, Gewirtz, AT, and Chassaing, B. Dietary emulsifier-induced low-grade inflammation promotes Colon carcinogenesis. Cancer Res. (2017) 77:27–40. doi: 10.1158/0008-5472.CAN-16-1359
90. Viennois, E, and Chassaing, B. Consumption of select dietary emulsifiers exacerbates the development of spontaneous intestinal adenoma. Int J Mol Sci. (2021) 22:2602. doi: 10.3390/ijms22052602
91. Yang, H, Wang, W, Romano, KA, Gu, M, Sanidad, KZ, Kim, D, et al. A common antimicrobial additive increases colonic inflammation and colitis-associated colon tumorigenesis in mice. Sci Transl Med. (2018) 10:eaan4116. doi: 10.1126/scitranslmed.aan4116
92. Loayza, JJJ, Kang, S, Schooth, L, Teh, JJ, de Klerk, A, Noon, EK, et al. Effect of food additives on key bacterial taxa and the mucosa-associated microbiota in Crohn's disease. The ENIGMA study. Gut Microb. (2023) 15:2172670. doi: 10.1080/19490976.2023.2172670
93. Trakman, GL, Lin, WYY, Hamilton, AL, Wilson-O'Brien, AL, Stanley, A, Ching, JY, et al. Processed food as a risk factor for the development and perpetuation of Crohn's disease-the ENIGMA study. Nutrients. (2022) 14:3627. doi: 10.3390/nu14173627
94. Wu, W, Fu, G, Xuan, R, Zhai, L, Lu, Y, Tang, M, et al. Food additive sodium bisulfite induces intracellular imbalance of biothiols levels in NCM460 colonic cells to trigger intestinal inflammation in mice. Toxicol Lett. (2022) 359:73–83. doi: 10.1016/j.toxlet.2022.01.019
95. Kwon, YH, Banskota, S, Wang, H, Rossi, L, Grondin, JA, Syed, SA, et al. Chronic exposure to synthetic food colorant Allura red AC promotes susceptibility to experimental colitis via intestinal serotonin in mice. Nat Commun. (2022) 13:7617. doi: 10.1038/s41467-022-35309-y
96. Borsani, B, De Santis, R, Perico, V, Penagini, F, Pendezza, E, Dilillo, D, et al. The role of carrageenan in inflammatory bowel diseases and allergic reactions: where do we stand? Nutrients. (2021) 13:3402. doi: 10.3390/nu13103402
97. Beal, J, Silverman, B, Bellant, J, Young, TE, and Klontz, K. Late onset necrotizing enterocolitis in infants following use of a xanthan gum-containing thickening agent. J Pediatr. (2012) 161:354–6. doi: 10.1016/j.jpeds.2012.03.054
98. Yang, Z, Qin, J, Zhao, L, Chen, T, Huang, Q, Jian, Y, et al. Host sorbitol and bacterial sorbitol utilization promote Clostridioides difficile infection in inflammatory bowel disease. Gastroenterology. (2023) 164:1189–201.e13. doi: 10.1053/j.gastro.2023.02.046
99. Katsoudas, N, Tavakoli, P, Wu, N, Shapiro, A, Leach, ST, Williams, AJ, et al. Dietary emulsifier exposure in people with inflammatory bowel disease compared with healthy controls: is there a cause for concern? Inflamm Bowel Dis. (2024) 30:1241–50. doi: 10.1093/ibd/izad318
100. Xu, J, Wang, M, Liu, Q, Lin, X, Pu, K, and He, Z. Gut microbiota mediated the toxicity of high concentration of dietary nitrite in C57BL/6 mice. Ecotoxicol Environ Saf. (2022) 231:113224. doi: 10.1016/j.ecoenv.2022.113224
101. Gómez-Gallego, C, Pohl, S, Salminen, S, De Vos, WM, and Kneifel, W. Akkermansia muciniphila: a novel functional microbe with probiotic properties. Benefic Microbes. (2016) 7:571–84. doi: 10.3920/BM2016.0009
102. Rothschild, D, Weissbrod, O, Barkan, E, Kurilshikov, A, Korem, T, Zeevi, D, et al. Environment dominates over host genetics in shaping human gut microbiota. Nature. (2018) 555:210–5. doi: 10.1038/nature25973
103. Daniel, N, Gewirtz, AT, and Chassaing, B. Akkermansia muciniphila counteracts the deleterious effects of dietary emulsifiers on microbiota and host metabolism. Gut. (2023) 72:906–17. doi: 10.1136/gutjnl-2021-326835
104. Zou, L, Spanogiannopoulos, P, Pieper, LM, Chien, HC, Cai, W, Khuri, N, et al. Bacterial metabolism rescues the inhibition of intestinal drug absorption by food and drug additives. Proc Natl Acad Sci USA. (2020) 117:16009–18. doi: 10.1073/pnas.1920483117
105. Hughes, RL, and Holscher, HD. Fueling gut microbes: a review of the interaction between diet, exercise, and the gut microbiota in athletes. Adv Nutr (Bethesda, MD). (2021) 12:2190–215. doi: 10.1093/advances/nmab077
106. Clauss, M, Gérard, P, Mosca, A, and Leclerc, M. Interplay between exercise and gut microbiome in the context of human health and performance. Front Nutr. (2021) 8:637010. doi: 10.3389/fnut.2021.637010
107. Voigt, RM, Forsyth, CB, Green, SJ, Mutlu, E, Engen, P, Vitaterna, MH, et al. Circadian disorganization alters intestinal microbiota. PLoS One. (2014) 9:e97500. doi: 10.1371/journal.pone.0097500
108. Rinninella, E, Cintoni, M, Raoul, P, Lopetuso, LR, Scaldaferri, F, Pulcini, G, et al. Food components and dietary habits: keys for a healthy gut microbiota composition. Nutrients. (2019) 11:2393. doi: 10.3390/nu11102393
109. Desai, MS, Seekatz, AM, Koropatkin, NM, Kamada, N, Hickey, CA, Wolter, M, et al. A dietary Fiber-deprived gut microbiota degrades the colonic mucus barrier and enhances pathogen susceptibility. Cell. (2016) 167:1339–53.e21. doi: 10.1016/j.cell.2016.10.043
110. Jansen, T, Claassen, L, van Kamp, I, and Timmermans, DRM. ‘All chemical substances are harmful.’ public appraisal of uncertain risks of food additives and contaminants. Food Chem Toxicol. (2020) 136:110959. doi: 10.1016/j.fct.2019.110959
111. Wang, X, Huang, M, Li, X, Dai, W, and Liang, J. Rapid screening of illegal additives in functional food using atmospheric pressure solids analysis probe coupled to a portable mass spectrometer. J Pharm Biomed Anal. (2022) 214:114722. doi: 10.1016/j.jpba.2022.114722
112. Hinojosa-Nogueira, D, Muros, JJ, Navajas-Porras, B, Delgado-Osorio, A, Pérez-Burillo, S, Pastoriza, S, et al. Development of a food composition database of different food contaminants CONT11 and estimation of dietary exposure in children of southern Spain. Food Chem Toxicol. (2023) 177:113843. doi: 10.1016/j.fct.2023.113843
113. Yan, S, Yan, F, Liu, L, Li, B, Liu, S, and Cui, W. Can artificial sweeteners increase the risk of Cancer incidence and mortality: evidence from prospective studies. Nutrients. (2022) 14:3742. doi: 10.3390/nu14183742
114. World Health Organization. Evaluation of certain food additives. World Health Organ Tech Rep Ser. (2017) 1–162.
115. Sigurdson, GT, Tang, P, and Giusti, MM. Natural colorants: food colorants from natural sources. Annu Rev Food Sci Technol. (2017) 8:261–80. doi: 10.1146/annurev-food-030216-025923
116. Martins, N, Roriz, CL, Morales, P, Barros, L, and Ferreira, I. Coloring attributes of betalains: a key emphasis on stability and future applications. Food Funct. (2017) 8:1357–72. doi: 10.1039/C7FO00144D
117. Wallace, TC, and Giusti, MM. Anthocyanins. Adv Nutr (Bethesda, Md). (2015) 6:620–2. doi: 10.3945/an.115.009233
118. Khoo, HE, Azlan, A, Tang, ST, and Lim, SM. Anthocyanidins and anthocyanins: colored pigments as food, pharmaceutical ingredients, and the potential health benefits. Food Nutr Res. (2017) 61:1361779. doi: 10.1080/16546628.2017.1361779
119. Dobrijević, D, Pastor, K, Nastić, N, Özogul, F, Krulj, J, Kokić, B, et al. Betaine as a functional ingredient: metabolism, health-promoting attributes, food sources, applications and analysis methods. Mol (Basel, Switzerland). (2023) 28:4824. doi: 10.3390/molecules28124824
120. Sadowska-Bartosz, I, and Bartosz, G. Biological properties and applications of Betalains. Molecules (Basel, Switzerland). (2021) 26:2520. doi: 10.3390/molecules26092520
121. Vandenberg, LN, and Bugos, J. Assessing the public health implications of the food preservative Propylparaben: has this chemical been safely used for decades. Curr Environ Health Rep. (2021) 8:54–70. doi: 10.1007/s40572-020-00300-6
122. Novais, C, Molina, AK, Abreu, RMV, Santo-Buelga, C, Ferreira, I, Pereira, C, et al. Natural food colorants and preservatives: a review, a demand, and a challenge. J Agric Food Chem. (2022) 70:2789–805. doi: 10.1021/acs.jafc.1c07533
123. Medema, MH, Blin, K, Cimermancic, P, de Jager, V, Zakrzewski, P, Fischbach, MA, et al. antiSMASH: rapid identification, annotation and analysis of secondary metabolite biosynthesis gene clusters in bacterial and fungal genome sequences. Nucleic Acids Res. (2011) 39:W339–46. doi: 10.1093/nar/gkr466
124. Jalosinska, M, and JJPJof, W. Sciences n. influence of plant extracts on the microbiological shelf life of meat products. Pol J Food Nutr Sci. (2009) 59:303–8.
125. Atarés, L, De Jesús, C, Talens, P, and Chiralt, A. Characterization of SPI-based edible films incorporated with cinnamon or ginger essential oils (2010) 99:384–91. doi: 10.1016/j.jfoodeng.2010.03.004,
127. Neha, K, Haider, MR, Pathak, A, and Yar, MS. Medicinal prospects of antioxidants: a review. Eur J Med Chem. (2019) 178:687–704. doi: 10.1016/j.ejmech.2019.06.010
128. Peters, R, Ee, N, Peters, J, Beckett, N, Booth, A, Rockwood, K, et al. Common risk factors for major noncommunicable disease, a systematic overview of reviews and commentary: the implied potential for targeted risk reduction. Ther Adv Chronic Dis. (2019) 10:204062231988039. doi: 10.1177/2040622319880392
129. Ramos-Souza, C, Bandoni, DH, Bragotto, APA, and De Rosso, VV. Risk assessment of azo dyes as food additives: revision and discussion of data gaps toward their improvement. Compr Rev Food Sci Food Saf. (2023) 22:380–407. doi: 10.1111/1541-4337.13072
130. Bearth, A, Cousin, ME, and Siegrist, M. "the dose makes the poison": informing consumers about the scientific risk assessment of food additives. Risk Anal. (2016) 36:130–44. doi: 10.1111/risa.12410
131. Lehto, S, Buchweitz, M, Klimm, A, Straßburger, R, Bechtold, C, and Ulberth, F. Comparison of food colour regulations in the EU and the US: a review of current provisions. Food Addit Contam Part A Chem Anal Control Expo Risk Assess. (2017) 34:335–55. doi: 10.1080/19440049.2016.1274431
Keywords: food additives, intestinal health, intestinal microecology, intestinal diseases, food safety regulation
Citation: Wang H, Bai J, Miao P, Wei Y, Chen X, Lan H, Qing Y, Zhao M, Li Y, Tang R and Yang X (2024) The key to intestinal health: a review and perspective on food additives. Front. Nutr. 11:1420358. doi: 10.3389/fnut.2024.1420358
Received: 25 April 2024; Accepted: 09 September 2024;
Published: 18 September 2024.
Edited by:
Philippe Gérard, Institut National de Recherche pour l’Agriculture, l’Alimentation et l’Environnement (INRAE), FranceReviewed by:
Jie Peng, Fudan University, ChinaCopyright © 2024 Wang, Bai, Miao, Wei, Chen, Lan, Qing, Zhao, Li, Tang and Yang. This is an open-access article distributed under the terms of the Creative Commons Attribution License (CC BY). The use, distribution or reproduction in other forums is permitted, provided the original author(s) and the copyright owner(s) are credited and that the original publication in this journal is cited, in accordance with accepted academic practice. No use, distribution or reproduction is permitted which does not comply with these terms.
*Correspondence: Rui Tang, MjM5NjI1ODIwNkBxcS5jb20=; Xiangdong Yang, eS14ZEB2aXAuMTYzLmNvbQ==
†These authors have contributed equally to this work and share first authorship
Disclaimer: All claims expressed in this article are solely those of the authors and do not necessarily represent those of their affiliated organizations, or those of the publisher, the editors and the reviewers. Any product that may be evaluated in this article or claim that may be made by its manufacturer is not guaranteed or endorsed by the publisher.
Research integrity at Frontiers
Learn more about the work of our research integrity team to safeguard the quality of each article we publish.