- 1Department of Medical Laboratory, Guihang 300 Hospital Affiliated to Zunyi Medical University, Guiyang, China
- 2Hospital Infection Control Department, Guihang 300 Hospital Affiliated to Zunyi Medical University, Guiyang, China
- 3Department of Endocrinology, Guihang 300 Hospital Affiliated to Zunyi Medical University, Guiyang, China
Objective: This study aims to explore the relationship between dietary folate intake and serum Klotho levels in adults from aged 40 to 79 years in the United States, seeking to elucidate the intricacies of their interaction.
Methods: Analyzing data from the National Health and Nutrition Examination Survey (NHANES) spanning 2007 to 2016. The survey research determined folate intake through a 24-h dietary recall and nutrient density modeling, and assessed Klotho levels using enzyme-linked immunosorbent assay (ELISA). The relationship between folate intake and Klotho levels was evaluated using weighted linear regression, and complemented by analysis via smoothed curve models for nuanced understanding.
Results: The study encompassed 10,278 participants, with an average age of 57.64 years, revealing a noteworthy positive correlation between dietary folate and serum Klotho levels. The regression coefficient stood at 0.11 (95% confidence interval, 0.05, 0.18) post-adjustment for various covariates. When dietary folate intake was categorized into quartiles, the second, third, and fourth quartiles exhibited statistically significant differences compared to the lowest quartile. This indicates that higher folate intake correlates with increased serum Klotho levels. These findings underscore the potential benefits of elevating folate intake to enhance serum Klotho levels. Stratified analysis indicated that this association was more pronounced among males aged 60 years or older and individuals with hypertension.
Conclusion: The findings suggest a significant correlation between increased dietary folate intake and elevated serum Klotho levels in adults aged 40–79 years. Hinting at the potential nutritional influences on the aging process and associated health conditions. This calls for further exploration into the mechanisms and broader implications of this association.
1 Introduction
Aging is universally recognized as a multifaceted process influenced by an intricate interplay of genetic, environmental, and lifestyle determinants (1–3). This phenomenon spans a comprehensive spectrum of biological transformations, including diminished cellular functionality (4), the accumulation of DNA damage (5), and anomalies in amino acid metabolism (6). These transformations transcend singular biological levels, engaging in complex interactions that cumulatively precipitate the deterioration of tissue and organ function, thereby impacting the organism’s health and longevity. Consequently, it is crucial to thoroughly understand these processes. Effective interventions can slow down the aging process and enhance quality of life.
Since 1997, the Klotho protein has emerged as a focal point in anti-aging research (7, 8). It is predominantly expressed in the kidneys (9) and found in other tissues including the brain (10). Klotho plays a pivotal role in fostering longevity and health. It does so by modulating calcium and phosphorus metabolism, contributing to the antioxidant defense, and impacting critical cellular signaling pathways, notably Wnt/β-catenin and insulin-like growth factor-1 (IGF-1) (11, 12). A decline in Klotho gene expression is intricately linked to various aging-associated diseases, such as cardiovascular disease (13), osteoporosis (14), and chronic kidney disease (15). Research demonstrates that enhancing Klotho protein levels exogenously or activating its signaling pathways can mitigate these conditions and potentially slow the aging process (13, 16–19). Therefore, Klotho proteins not only serve as crucial markers for deciphering the mechanisms underlying aging, but also offer promising avenues for developing novel anti-aging therapies (8).
Folate, a critical component of the B-vitamin family, occupies a pivotal role in processes such as cell division and growth (20, 21), DNA synthesis and repair (22), and the metabolism of amino acids (23). Its significance is particularly pronounced during the initial stages of development, where it is indispensable in forestalling congenital disorders, including neural tube defects (24). Furthermore, a deficiency in folate intake can precipitate a range of health issues, encompassing anemia, cardiovascular diseases, and even cognitive impairments (25, 26). Ongoing scientific investigation continues to unveil the advantages of folate in supporting cardiovascular health, enhancing cognitive function, and contributing to anti-aging benefits (27). Therefore, it is essential to supplement folate for promoting health and preventing disease in pregnant women, those planning pregnancy, and individuals at risk of folate deficiency. However, high-dose supplementation is unnecessary for healthy individuals with normal folate levels (28).
Recent research has elucidated that particular dietary practices and the intake of specific types of food are closely linked to fluctuations in klotho protein levels (29, 30). Considering the pivotal roles that both klotho protein and folate occupy in fostering health and decelerating the aging process, understanding the dynamics of their interplay is of paramount importance.
2 Materials and methods
2.1 Study population
This investigation drew upon data from five successive cycles of the National Health and Nutrition Examination Survey (NHANES) spanning from 2007 to 2016, encompassing 87,719 participants. Following the exclusion of subjects due to incomplete data on serum Klotho levels (n = 36,824), Dietary energy (n = 38,432), dietary folate intake (n = 804), and other covariates (n = 1,381). the study’s final cohort consisted of 10,278 individuals (Figure 1). The NHANES employed a sophisticated multistage probability sampling technique, orchestrated by the National Center for Health Statistics (NCHS). Informed Consent was signed by each participant, and the methodology of data collection received approval from an ethics committee, ensuring the protection of participants. Comprehensive details regarding the survey are accessible on the NHANES website.1
2.2 Measurement of dietary folate intake
In this study, the 24-h dietary recall was conducted using a combination of face-to-face and telephone interviews. The initial interview was carried out at the Mobile Examination Center (MEC), followed by subsequent interviews conducted via telephone 3 to 10 days later. This approach employed the USDA’s Automated Multiple-Pass Method (AMPM),2 which precisely recorded the types and quantities of foods and beverages consumed by participants within the 24 h prior to the interview. The nutrient content of these consumables was then assessed through a comprehensive dietary survey. The nutrient calculations used the USDA’s Food and Nutrient Database for Dietary Studies (FNDDS 4.1; see Footnote 2). Moreover, the average of the two dietary recalls was taken, reflecting only the actual intake levels, not the habitual intake of the population. To minimize the measurement errors associated with self-reported dietary assessment tools, we standardized nutrient intake relative to total energy intake and accounted for variations in individual energy needs. The adjusted intake formula is: dietary folate intake / energy intake * 1000 (31). This method, commonly referred to as nutrient density model, allows for a more precise evaluation of dietary folate intake across diverse energy requirements.
Additionally, in sensitivity analyses, we extended this method to assess folate from natural foods, folic acid from fortified foods and supplements, and dietary vitamin B12. Each nutrient’s intake was similarly adjusted for energy, ensuring robustness in our analyses across different dietary sources and intake patterns.
2.3 Serum klotho levels
Blood samples from participants were transported to the Northwest Laboratory for Lipid Metabolism and Diabetes Research, adhering to predefined protocols, and subsequently preserved at −80°C for analysis. The measurement of Klotho levels was conducted utilizing an ELISA kit produced (“IBL International,” Japan). In a bid to uphold laboratory standards and mitigate potential detection bias attributable to random variance, the samples underwent duplicate analyses. The mean of these analyses was accepted contingent upon compliance with the in-lot quality control criteria. Samples demonstrating a variance exceeding 10% between the two measurements were subjected to reevaluation and duly documented.
2.4 Assessment of covariates
The study incorporated a comprehensive set of covariates, totaling 11 which included both continuous and categorical variables. These covariates are as follows: sex (categorized as male or female), age, racial/ethnic background (categorized as non-Hispanic white, non-Hispanic black, Mexican American, or other, which includes multiracial and other Latino identities), marital status (categorized as married, not currently partnered, or cohabiting with a partner), and household income relative to the poverty threshold (categorized into three groups: ≤1.30, 1.31–3.50, and > 3.50 based on the poverty income ratio [PIR]). The poor income ratio was determined based on Federal Poverty Level (FPL) information, which considers factors such as inflation and family size.3 The Body Mass Index (BMI) is calculated as weight (in kilograms) divided by the square of height (in meters; kg/m2). According to the World Health Organization (WHO), BMI is categorized into four groups: underweight (less than 18.5 kg/m2), normal weight (18.5 to less than 25 kg/m2), overweight (25 to less than 30 kg/m2), and obese (30 kg/m2 or greater).4 Hypertension was defined as having an average systolic blood pressure greater than 140 mmHg, an average diastolic blood pressure greater than 90 mmHg, a history of being diagnosed with high blood pressure, or current use of prescription medication for high blood pressure, with at least one of these criteria being met. Diabetes status was determined based on a doctor’s diagnosis. Heart disease identification relied on four indicators: a history of congestive heart failure, coronary artery disease, angina pectoris, or a heart attack, with any one of these conditions affirming the presence of heart disease. The Urine Albumin to Creatinine Ratio (UACR) was categorized as <30 mg/g for normal individuals and ≥ 30 mg/g indicating abnormal proteinuria. Glycosylated hemoglobin (HbA1c) levels were also considered, serving as an indicator of long-term glycemic control.
2.5 Statistical analysis
To obtain more representative estimates, this study applied the MEC weights recommended by the NHANES database in all analyses. All data collection and statistical analyses were performed using R4.2.05 and Empower Stats.6
The relationship between dietary folate intake and serum Klotho levels was examined using multivariate linear regression analysis, which facilitated the computation of beta values and 95% confidence intervals. This analysis was structured into three distinct models: Model 1, which did not adjust for any variables; Model 2, which adjusted for sex, age, and race; and Model 3, which incorporated adjustments for additional covariates. Serum Klotho levels were analyzed both as a continuous independent variable and categorically, divided into quartiles, to evaluate trends. To visually represent this relationship, a smoothed curve-fitting model was employed. The study also conducted stratified and interaction analyses to investigate how this association might differ across subgroups defined by each covariate. Specifically, analyses were stratified by age (comparing those under 60 years to those 60 years and older), sex, and the presence of hypertension across varying dietary folate intake.
Further, sensitivity analyses were carried out on distinct population subsets: those consuming only naturally occurring folate in foods, those whose intake included only dietary folic acid from fortified food [calculated as μg of DFEs provided = μg of natural food folate + (1.7 × μg of folic acid)] (32), and those consuming folate exclusively through supplements. The characteristics of these populations are detailed in Supplementary Table S1. These analyses aimed to assess the impact of potential outliers on the findings. To ensure the stability of the outcomes, the study excluded data points representing extreme values, this included individuals with Klotho levels above 2,500 pg./mL, those with dietary folate intake exceeding 500 μg/1000 kcal or below 100 μg/1000 kcal after energy adjustment, and individuals with energy-adjusted vitamin B12 levels greater than 6.0 μg/1000 kcal. Additionally, individuals categorized as “obese” by BMI, as well as those diagnosed with hypertension, diabetes, or heart disease, and those with a urine albumin/creatinine ratio (UACR) greater than 30 mg/g were also excluded.
3 Results
3.1 Baseline characteristics of all participants
This study enrolled a total of 10,278 participants, of whom 47.75% were male and 52.25% were female. The age range of participants spanned from 40 to 79 years, with a mean age of 57.64 years (standard deviation ±10.82 years). Participants were stratified based on the quartiles of serum Klotho levels, and the baseline characteristics are delineated in Table 1. Significant differences were observed across the quartiles of Dietary folate intake in terms of age, gender, race, marital status, Poor income ratio, body mass index (BMI), diabetes mellitus, urinary albumin-to-creatinine ratio (UACR), and glycosylated hemoglobin levels.
3.2 Association between dietary folate intake and serum klotho levels
In the multivariate regression analysis detailed in Table 2, an association was observed between dietary folate intake and serum Klotho levels. Specifically, in both Model 2 and Model 3, each 1 μg/1000 kcal increase in energy-adjusted dietary folate intake was associated with a 0.11 pg./mL increase in serum Klotho levels. Subgroup analyses in Models 2 and 3 showed significant associations for Groups Q2, Q3, and Q4 compared to Group Q1, with p-values less than 0.05 for each group. The trend difference between the groups in both Model 2 and Model 3 was significant, with p-values for trend less than 0.0022 and 0.0035, respectively.
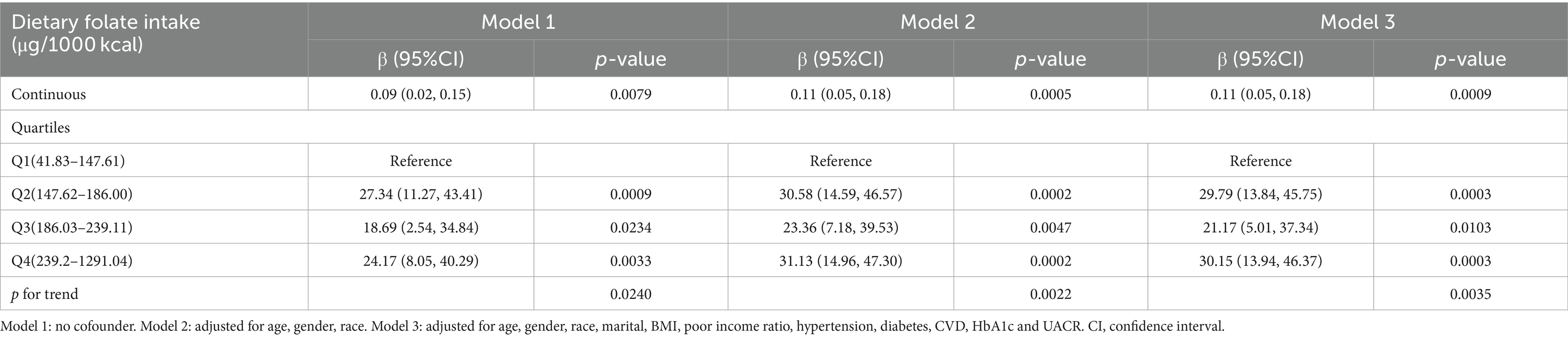
Table 2. Association between dietary folate intake and serum klotho levels among adults in NHANES 2007–2016.
We used smoothed curve fitting to show the complex, non-linear relationship between folate intake and Klotho levels in the blood (Figure 2). This technique helps highlight how these two variables interact beyond straightforward linear patterns.
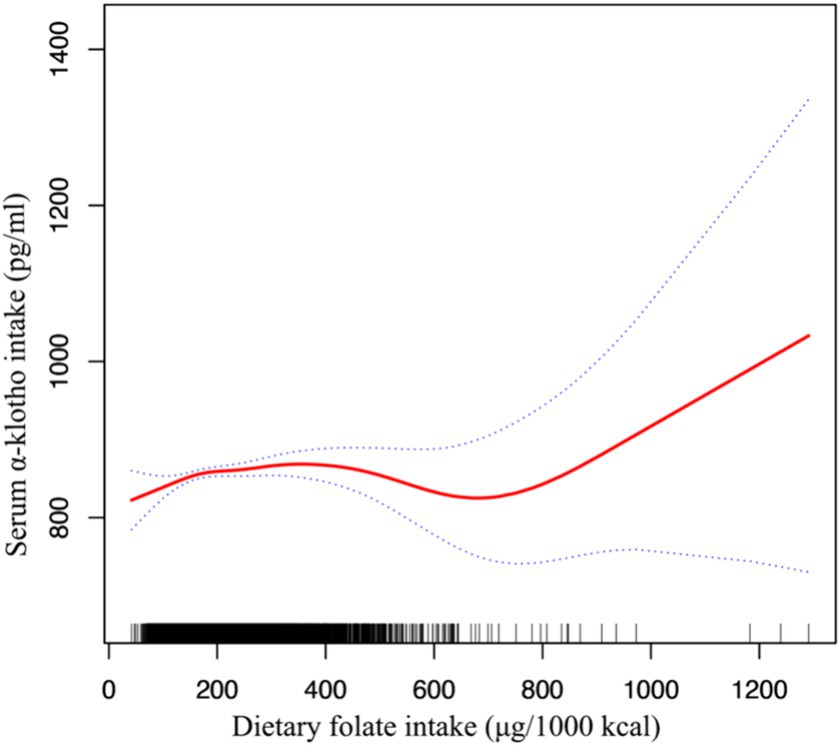
Figure 2. Association between dietary folate intake and serum Klotho levels adjusted for age, gender, race, marital, body mass index, poor income ratio, hypertension, diabetes, CVD, HbA1c and UACR.
3.3 Subgroup analysis and sensitivity analysis
In the subgroup analysis examining the relationship between dietary folate intake and Klotho levels (Table 3), the study population was stratified into quartiles based on their dietary folate intake. Following adjustments for potential confounding variables, the correlation appeared to be more pronounced among individuals aged 60 years or older, within the male demographic, and among participants with a history of hypertension.
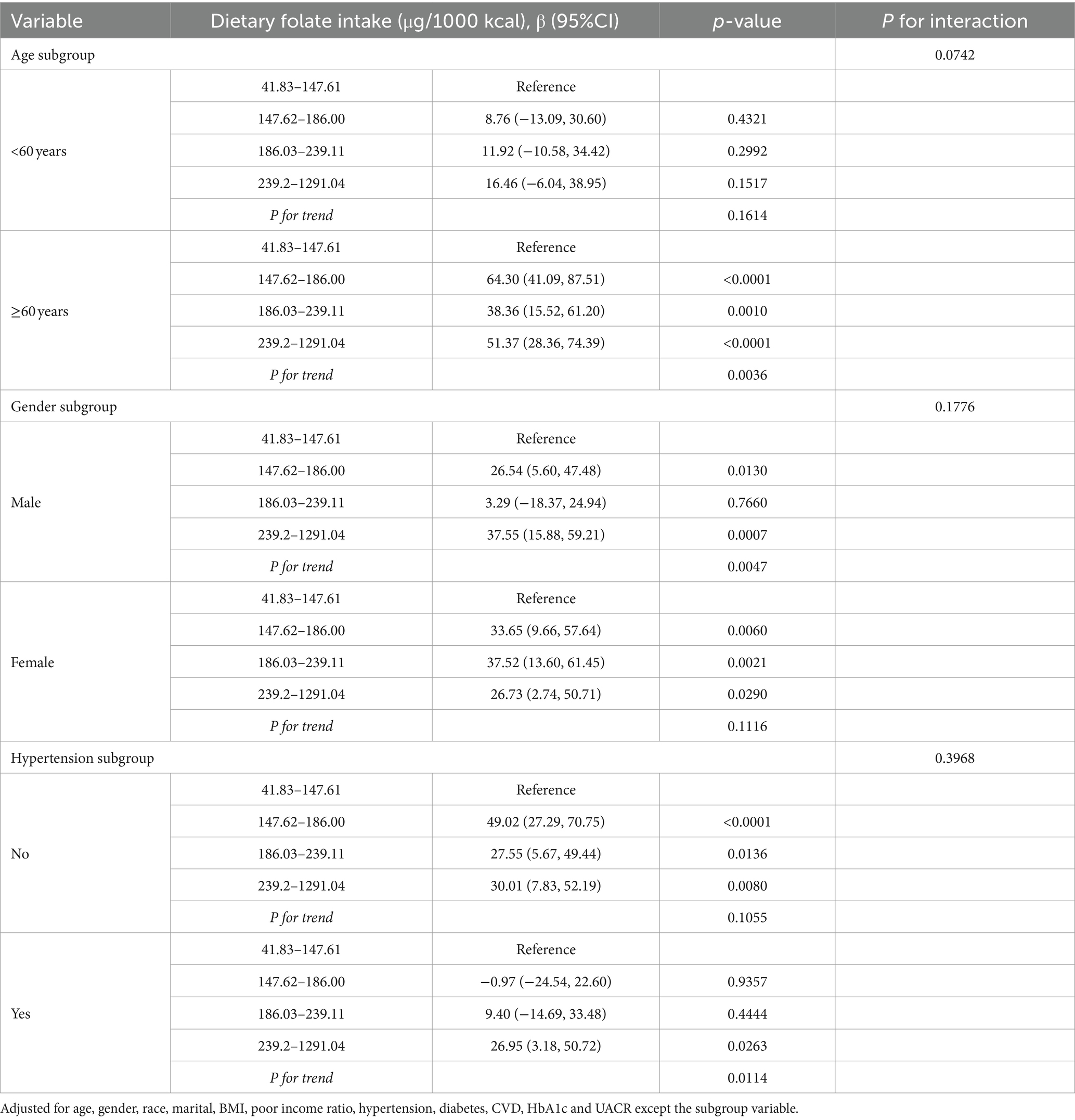
Table 3. Stratified analyses of association between dietary folate intake and serum klotho levels in NHANES 2007–2016.
Furthermore, extensive sensitivity analyses were conducted in this study to assess the stability of the results (refer to Table 4): (1) only samples deriving from natural food folate intake were included; (2) Inclusion was limited to samples containing dietary folic acid from fortified foods; (3) the analysis included only samples with intake of folate supplements; (4) samples exhibiting Klotho levels >2,500 pg./mL were excluded; (5) Excluding samples with dietary folate intake exceeding 500 μg/1000 kcal; (6) exclusion of samples with <100 μg/1000 kcal of dietary folate intake; (7) samples with dietary vitamin B12 intake >6.0 μg/1000 kcal were also excluded; (8) given the potential influence of hyperlipidemia on Klotho levels, samples with a BMI categorization of “obese” were excluded (33); (9) considering the association of hypertension prevalence with lower Klotho levels, samples with a hypertension status of “yes” were excluded (34); (10) due to the nonlinear relationship between serum Klotho levels and the prevalence of diabetes mellitus, samples with a diabetes mellitus status of “yes” were excluded (35); (11) recognizing the significant correlations of serum Klotho with heart failure (36) and myocardial infarction (37), samples with a cardiac status of “yes” were excluded; (12) considering the association between Klotho levels and urinary albumin excretion rate (38) as well as chronic kidney disease albuminuria (15) samples with UACR results ≥30 were excluded. These results imply that folate intake from natural sources significantly impacts serum Klotho levels, whereas factors such as dietary fortification with folic acid and the prevalence of hypertension appear to have a negligible influence on this relationship.
4 Discussion
In our study, we explored the relationship between dietary folate intake and serum Klotho levels, finding a nuanced interplay. This connection was stronger in individuals over 60, males, and those with hypertension, highlighting folate’s role in regulating the anti-aging factor Klotho. After adjusting for energy intake, each additional unit of dietary folate intake was associated with a 0.11 pg./mL increase in Klotho levels. This correlation may play a positive role in promoting healthy aging and preventing age-related diseases. Additionally, it lays the foundation for pioneering new health strategies focused on dietary interventions.
Folate intake has been found to exert significant effects on multiple signaling pathways, notably the mTOR pathway, the insulin/IGF-1 signaling pathway, and the Wnt/β-catenin signaling pathway. For instance, the research conducted by Fredrick J. Rosario and colleagues illustrates that maternal folate deficiency can impair fetal growth by inhibiting placental mTOR signaling, thereby establishing a direct connection between folate levels and mTOR signaling (39). Furthermore, Elena Silva’s studies have shown that folate deficiency influences cellular functions through innovative sensing mechanisms, which in turn activate the mTOR signaling pathway, affecting processes such as nutrient transport and protein synthesis (40). Additionally, Andrea Annibal and co-authors have discovered through high-resolution mass spectrometry analysis of the metabolome of the nematode Cryptomeria hidrobatidis, that one-carbon metabolism and the folate cycle are integral in jointly regulating lifespan, with insulin/IGF signaling playing a comparable regulatory role across different species’ longevity models. This suggests that certain metabolic nodes are pivotal in promoting healthy aging (41). Meanwhile, research by Wen-Chi L. Chang and others on the impact of folic acid supplementation in the development of colitis-associated colorectal cancer indicated that high doses of folic acid can encourage cancer formation by modifying the epigenetic field effects of the Wnt/β-catenin and MAPK signaling pathways (42). Despite the compelling evidence linking folate intake with mechanisms of anti-aging, a gap remains in the direct, controlled trials demonstrating a connection with Klotho, highlighting a promising avenue for future research.
The primary dietary sources of folate include green leafy vegetables (for instance, spinach and broccoli), citrus fruits, legumes, nuts, and whole grain products (43–47). To alleviate health issues caused by folate deficiency, since 1998, the United States and Canada have mandated the addition of dietary folic acid from fortified food to flour and grain products. Common foods containing fortified folic acid include flour, bread, breakfast cereals, pasta, and cornmeal (48–50). Our sensitivity analysis unveiled a noteworthy observation: a significant correlation was identified exclusively between folate intake from natural sources and serum Klotho levels, whereas folic acid obtained through fortified foods and supplements did not exhibit a similar association, paralleling the discoveries of Mengyi Liu et al. (51). This prompts us to suggest that there exists an “optimal” range for folate intake, with the intake of folate from natural foods generally falling precisely within this range (52). The excessive intake of folic acid may precipitate adverse effects, notably when surpassing the recommended daily allowance (53). Such overconsumption bears the risk of concealing the diagnosis of vitamin B12 deficiency and merely ameliorating anemia symptoms without addressing the potential for neurological harm (54–56). Furthermore, certain research suggests that a long-term, high-dose regimen of folic acid could correlate with an elevated risk of specific cancers (57). Consequently, it is imperative to regulate folic acid intake meticulously to prevent excessive ingestion (58).
Subgroup analyses have elucidated a broad decline in metabolic function and nutrient absorption capabilities as individuals age (59–61). Notably, research focusing on adults aged 60 and above has highlighted a correlation between folate intake and serum Klotho levels, particularly pronounced among those seniors with elevated folate intake (p < 0.0001, trend p-value = 0.0036). These findings underscore the significance of maintaining a moderate intake of folate within aging populations to support cardiovascular health, bone integrity, and other health facets (62, 63). Conversely, in individuals younger than 60 years, the link between folate intake and Klotho levels did not prove to be significant. This suggests that the demand for folate, along with its contribution to physical well-being, assumes greater importance as one ages, highlighting the potential benefits of targeted nutritional supplementation to meet the specific needs posed by aging (2, 26).
Moreover, we observed pronounced differences between genders regarding the association between dietary folate intake and serum Klotho levels among men and women (64). In men, a notable correlation was observed between folate intake and serum Klotho levels, particularly within the moderate to high folate intake category (p-values = 0.0007 and a trend p-value of 0.0047). Similarly, in females, dietary folate intake was significantly associated with serum Klotho levels (p-values ranging from 0.0021 to 0.0290, trend p-value = 0.1116). Although some literature suggests the potential influence of testosterone levels on Klotho protein expression in men (65–67), our current dataset does not directly investigate this hypothesis at either a physiological or molecular level. Thus, these findings underscore the critical need for subsequent research to more comprehensively explore how gender differences affect the interplay between folate intake and Klotho levels.
The potential beneficial impact of folate on the expression and functionality of Klotho proteins assumes critical importance, particularly in light of Klotho’s pivotal role in preserving vascular health and regulating the equilibrium of blood pressure (20, 62). This mode of action not only underscores the profound importance of folate in combating cardiovascular diseases but also illuminates its prospective utility in the realm of anti-aging (41).
This study is subject to certain limitations. Firstly, the NHANES cohort might not accurately reflect the global population, particularly in countries and regions where food is not fortified with folic acid. Thus, incorporating additional samples from a variety of centers could enhance the study’s validity. Secondly, like all dietary assessment methods, the 24-h dietary recall used in this study has inherent limitations. These include recall bias, as it relies on participants’ ability to accurately remember and report their food intake, and response bias, where participants might alter their reported intake to align with perceived social norms or to avoid negative judgment. Additionally, the 24-h recall provides a snapshot of a single day’s intake, which may not accurately represent usual dietary patterns. Moreover, while data from cross-sectional studies can establish associations, they are insufficient for determining causality, necessitating further evidence to elucidate the cause-and-effect relationship.
5 Conclusion
In summary, among a nationally representative population of American adults, our study identified a significant association between dietary folate intake and serum Klotho levels, especially prominent in men, individuals aged 60 and above, and those with hypertension. These findings suggest a potential role for dietary folate in supporting healthy aging and underscore the need for further research to understand its mechanisms and broader health implications.
Data availability statement
Publicly available datasets were analyzed in this study. This data can be found at: https://wwwn.cdc.gov/nchs/nhanes/Default.aspx.
Ethics statement
The studies involving humans were approved by NCHS Ethics Review Board (ERB) Approval. The studies were conducted in accordance with the local legislation and institutional requirements. The participants provided their written informed consent to participate in this study.
Author contributions
YL: Data curation, Writing – original draft, Writing – review & editing. CZ: Conceptualization, Writing – review & editing. RS: Data curation, Writing – review & editing. AW: Conceptualization, Writing – review & editing. TZ: Writing – review & editing. ZC: Conceptualization, Writing – review & editing.
Funding
The author(s) declare that no financial support was received for the research, authorship, and/or publication of this article.
Acknowledgments
We sincerely thank the NHANES team and participants for their dedication and contributions, essential to the success of this study.
Conflict of interest
The authors declare that the research was conducted in the absence of any commercial or financial relationships that could be construed as a potential conflict of interest.
Publisher’s note
All claims expressed in this article are solely those of the authors and do not necessarily represent those of their affiliated organizations, or those of the publisher, the editors and the reviewers. Any product that may be evaluated in this article, or claim that may be made by its manufacturer, is not guaranteed or endorsed by the publisher.
Supplementary material
The Supplementary material for this article can be found online at: https://www.frontiersin.org/articles/10.3389/fnut.2024.1420087/full#supplementary-material
Footnotes
1. ^https://www.cdc.gov/nchs/nhanes/about_nhanes.htm
2. ^http://www.ars.usda.gov/ba/bhnrc/fsrg
3. ^https://aspe.hhs.gov/prior-hhs-poverty-guidelines-and-federal-register-references
4. ^https://www.who.int/data/gho/data/themes/topics/topic-details/GHO/body-mass-index
References
1. Bjørklund, G, Shanaida, M, Lysiuk, R, Butnariu, M, Peana, M, Sarac, I, et al. Natural compounds and products from an anti-aging perspective. Molecules. (2022) 27:7084. doi: 10.3390/molecules27207084
2. Yousefzadeh, M, Henpita, C, Vyas, R, Soto-Palma, C, Robbins, P, and Niedernhofer, L. DNA damage-how and why we age? eLife. (2021) 10:10. doi: 10.7554/eLife.62852
3. Benayoun, BA, Pollina, EA, and Brunet, A. Epigenetic regulation of ageing: linking environmental inputs to genomic stability. Nat Rev Mol Cell Biol. (2015) 16:593–610. doi: 10.1038/nrm4048
4. Castaldi, A, Dodia, RM, Orogo, AM, Zambrano, CM, Najor, RH, Gustafsson, ÅB, et al. Decline in cellular function of aged mouse C-kit(+) cardiac progenitor cells. J Physiol. (2017) 595:6249–62. doi: 10.1113/jp274775
5. Schumacher, B, Pothof, J, Vijg, J, and Hoeijmakers, JHJ. The central role of DNA damage in the ageing process. Nature. (2021) 592:695–703. doi: 10.1038/s41586-021-03307-7
6. Johnson, AA, and Cuellar, TL. Glycine and aging: evidence and mechanisms. Ageing Res Rev. (2023) 87:101922. doi: 10.1016/j.arr.2023.101922
7. Kuro-o, M, Matsumura, Y, Aizawa, H, Kawaguchi, H, Suga, T, Utsugi, T, et al. Mutation of the mouse klotho gene leads to a syndrome resembling ageing. Nature. (1997) 390:45–51. doi: 10.1038/36285
8. Abraham, CR, and Li, A. Aging-suppressor klotho: prospects in diagnostics and therapeutics. Ageing Res Rev. (2022) 82:101766. doi: 10.1016/j.arr.2022.101766
9. Buchanan, S, Combet, E, Stenvinkel, P, and Shiels, PG. Klotho, aging, and the failing kidney. Front Endocrinol (Lausanne). (2020) 11:560. doi: 10.3389/fendo.2020.00560
10. Semba, RD, Moghekar, AR, Hu, J, Sun, K, Turner, R, Ferrucci, L, et al. Klotho in the cerebrospinal fluid of adults with and without Alzheimer's disease. Neurosci Lett. (2014) 558:37–40. doi: 10.1016/j.neulet.2013.10.058
11. Miao, J, Liu, J, Niu, J, Zhang, Y, Shen, W, Luo, C, et al. Wnt/Β-catenin/Ras signaling mediates age-related renal fibrosis and is associated with mitochondrial dysfunction. Aging Cell. (2019) 18:e13004. doi: 10.1111/acel.13004
12. Sopjani, M, Rinnerthaler, M, Kruja, J, and Dermaku-Sopjani, M. Intracellular signaling of the aging suppressor protein klotho. Curr Mol Med. (2015) 15:27–37. doi: 10.2174/1566524015666150114111258
13. Chen, K, Wang, S, Sun, QW, Zhang, B, Ullah, M, and Sun, Z. Klotho deficiency causes heart aging via impairing the Nrf2-gr pathway. Circ Res. (2021) 128:492–507. doi: 10.1161/circresaha.120.317348
14. Torres, PU, Prié, D, Molina-Blétry, V, Beck, L, Silve, C, and Friedlander, G. Klotho: an antiaging protein involved in mineral and vitamin D metabolism. Kidney Int. (2007) 71:730–7. doi: 10.1038/sj.ki.5002163
15. Zhang, J, and Zhang, A. Relationships between serum klotho concentrations and cognitive performance among older chronic kidney disease patients with albuminuria in Nhanes 2011-2014. Front Endocrinol (Lausanne). (2023) 14:1215977. doi: 10.3389/fendo.2023.1215977
16. Zhou, H, Pu, S, Zhou, H, and Guo, Y. Klotho as potential autophagy regulator and therapeutic target. Front Pharmacol. (2021) 12:755366. doi: 10.3389/fphar.2021.755366
17. Yi, YY, Chen, H, Zhang, SB, Xu, HW, Fang, XY, and Wang, SJ. Exogenous klotho ameliorates extracellular matrix degradation and angiogenesis in intervertebral disc degeneration via inhibition of the Rac1/Pak1/Mmp-2 signaling Axis. Mech Ageing Dev. (2022) 207:111715. doi: 10.1016/j.mad.2022.111715
18. Castner, SA, Gupta, S, Wang, D, Moreno, AJ, Park, C, Chen, C, et al. Longevity factor klotho enhances cognition in aged nonhuman Primates. Nat Aging. (2023) 3:931–7. doi: 10.1038/s43587-023-00441-x
19. Kale, A, Sankrityayan, H, Anders, HJ, and Gaikwad, AB. Epigenetic and non-epigenetic regulation of klotho in kidney disease. Life Sci. (2021) 264:118644. doi: 10.1016/j.lfs.2020.118644
20. Zheng, Y, and Cantley, LC. Toward a better understanding of folate metabolism in health and disease. J Exp Med. (2019) 216:253–66. doi: 10.1084/jem.20181965
21. Clare, CE, Brassington, AH, Kwong, WY, and Sinclair, KD. One-carbon metabolism: linking nutritional biochemistry to epigenetic programming of long-term development. Annu Rev Anim Biosci. (2019) 7:263–87. doi: 10.1146/annurev-animal-020518-115206
22. Koury, MJ, and Ponka, P. New insights into erythropoiesis: the roles of folate, vitamin B12, and Iron. Annu Rev Nutr. (2004) 24:105–31. doi: 10.1146/annurev.nutr.24.012003.132306
23. Stanhewicz, AE, and Kenney, WL. Role of folic acid in nitric oxide bioavailability and vascular endothelial function. Nutr Rev. (2017) 75:61–70. doi: 10.1093/nutrit/nuw053
24. Copp, AJ, and Greene, ND. Neural tube defects: prevention by folic acid and other vitamins. Indian J Pediatr. (2000) 67:915–21. doi: 10.1007/bf02723958
25. Tamura, T, and Picciano, MF. Folate and human reproduction. Am J Clin Nutr. (2006) 83:993–1016. doi: 10.1093/ajcn/83.5.993
26. Liu, Y, Geng, T, Wan, Z, Lu, Q, Zhang, X, Qiu, Z, et al. Associations of serum folate and vitamin B12 levels with cardiovascular disease mortality among patients with type 2 diabetes. JAMA Netw Open. (2022) 5:e2146124. doi: 10.1001/jamanetworkopen.2021.46124
27. Ashraf, MJ, Cook, JR, and Rothberg, MB. Clinical utility of folic acid testing for patients with Anemia or dementia. J Gen Intern Med. (2008) 23:824–6. doi: 10.1007/s11606-008-0615-z
28. Paniz, C, Bertinato, JF, Lucena, MR, De Carli, E, Amorim, P, Gomes, GW, et al. A daily dose of 5 mg folic acid for 90 days is associated with increased serum Unmetabolized folic acid and reduced natural killer cell cytotoxicity in healthy Brazilian adults. J Nutr. (2017) 147:1677–85. doi: 10.3945/jn.117.247445
29. Wu, SE, Chen, YJ, and Chen, WL. Adherence to Mediterranean diet and soluble klotho level: the value of food synergy in aging. Nutrients. (2022) 14:3910. doi: 10.3390/nu14193910
30. Liu, S, Wu, M, Wang, Y, Xiang, L, Luo, G, Lin, Q, et al. The association between dietary Fiber intake and serum klotho levels in Americans: a cross-sectional study from the National Health and nutrition examination survey. Nutrients. (2023) 15:3147. doi: 10.3390/nu15143147
31. Willett, WC, Howe, GR, and Kushi, LH. Adjustment for Total energy intake in epidemiologic studies. Am J Clin Nutr. (1997) 65:1220S–8S. doi: 10.1093/ajcn/65.4.1220S
32. Institute of Medicine Standing Committee on the Scientific Evaluation of Dietary Reference I, its Panel on Folate OBV, Choline . Dietary Reference Intakes for Thiamin, Riboflavin, Niacin, Vitamin B(6), Folate, Vitamin B(12), Pantothenic Acid, Biotin, and Choline. Washington (DC): National Academies Press (1998).
33. Yan, S, Luo, W, Lei, L, Zhang, Q, and Xiu, J. Association between serum klotho concentration and hyperlipidemia in adults: a cross-sectional study from Nhanes 2007-2016. Front Endocrinol (Lausanne). (2023) 14:1280873. doi: 10.3389/fendo.2023.1280873
34. Yan, Y, and Chen, J. Association between serum klotho concentration and all-cause and cardiovascular mortality among American individuals with hypertension. Front Cardiovasc Med. (2022) 9:1013747. doi: 10.3389/fcvm.2022.1013747
35. Wang, K, Mao, Y, Lu, M, Liu, X, Sun, Y, Li, Z, et al. Association between serum klotho levels and the prevalence of diabetes among adults in the United States. Front Endocrinol (Lausanne). (2022) 13:1005553. doi: 10.3389/fendo.2022.1005553
36. Cai, J, Zhang, L, Chen, C, Ge, J, Li, M, Zhang, Y, et al. Association between serum klotho concentration and heart failure in adults, a cross-sectional study from Nhanes 2007-2016. Int J Cardiol. (2023) 370:236–43. doi: 10.1016/j.ijcard.2022.11.010
37. Xu, JP, Zeng, RX, He, MH, Lin, SS, Guo, LH, and Zhang, MZ. Associations between serum soluble Α-klotho and the prevalence of specific cardiovascular disease. Front Cardiovasc Med. (2022) 9:899307. doi: 10.3389/fcvm.2022.899307
38. Chang, K, Li, Y, Qin, Z, Zhang, Z, Wang, L, Yang, Q, et al. Association between serum soluble Α-klotho and urinary albumin excretion in middle-aged and older us adults: Nhanes 2007-2016. J Clin Med. (2023) 12:637. doi: 10.3390/jcm12020637
39. Rosario, FJ, Nathanielsz, PW, Powell, TL, and Jansson, T. Maternal folate deficiency causes inhibition of Mtor signaling, Down-regulation of placental amino acid transporters and fetal growth restriction in mice. Sci Rep. (2017) 7:3982. doi: 10.1038/s41598-017-03888-2
40. Silva, E, Rosario, FJ, Powell, TL, and Jansson, T. Mechanistic target of rapamycin is a novel molecular mechanism linking folate availability and cell function. J Nutr. (2017) 147:1237–42. doi: 10.3945/jn.117.248823
41. Annibal, A, Tharyan, RG, Schonewolff, MF, Tam, H, Latza, C, Auler, MMK, et al. Regulation of the one carbon folate cycle as a shared metabolic signature of longevity. Nat Commun. (2021) 12:3486. doi: 10.1038/s41467-021-23856-9
42. Chang, WL, Ghosh, J, Cooper, HS, Vanderveer, L, Schultz, B, Zhou, Y, et al. Folic acid supplementation promotes Hypomethylation in both the inflamed colonic mucosa and colitis-associated dysplasia. Cancers (Basel). (2023) 15:2949. doi: 10.3390/cancers15112949
43. Morris, MC, Wang, Y, Barnes, LL, Bennett, DA, Dawson-Hughes, B, and Booth, SL. Nutrients and bioactives in green leafy vegetables and cognitive decline: prospective study. Neurology. (2018) 90:e214–22. doi: 10.1212/wnl.0000000000004815
44. Saini, RK, Nile, SH, and Park, SW. Carotenoids from fruits and vegetables: chemistry, analysis, occurrence, bioavailability and biological activities. Food Res Int. (2015) 76:735–50. doi: 10.1016/j.foodres.2015.07.047
45. Miles, EA, and Calder, PC. Effects of Citrus fruit juices and their bioactive components on inflammation and immunity: a narrative review. Front Immunol. (2021) 12:712608. doi: 10.3389/fimmu.2021.712608
46. Thomas, PM, Flanagan, VP, and Pawlosky, RJ. Determination of 5-Methyltetrahydrofolic acid and folic acid in Citrus juices using stable isotope dilution-mass spectrometry. J Agric Food Chem. (2003) 51:1293–6. doi: 10.1021/jf020902e
47. Liang, Q, Wang, K, Shariful, I, Ye, X, and Zhang, C. Folate content and retention in wheat grains and wheat-based foods: effects of storage, processing, and cooking methods. Food Chem. (2020) 333:127459. doi: 10.1016/j.foodchem.2020.127459
48. Crider, KS, Bailey, LB, and Berry, RJ. Folic acid food fortification-its history, effect, concerns, and future directions. Nutrients. (2011) 3:370–84. doi: 10.3390/nu3030370
49. Centeno Tablante, E, Pachón, H, Guetterman, HM, and Finkelstein, JL. Fortification of wheat and maize flour with folic acid for population health outcomes. Cochrane Database Syst Rev. (2019) 7:CD012150. doi: 10.1002/14651858.CD012150.pub2
50. Wang, A, Rose, CE, Qi, YP, Williams, JL, Pfeiffer, CM, and Crider, KS. Impact of voluntary folic acid fortification of corn Masa flour on Rbc folate concentrations in the U.S. (Nhanes 2011-2018). Nutrients. (2021) 13:1325. doi: 10.3390/nu13041325
51. Liu, M, Ye, Z, Yang, S, Zhang, Y, Zhang, Y, He, P, et al. Relationship of dietary intake of food folate and synthetic folic acid intake from fortified foods with all-cause mortality in individuals with chronic kidney disease. Food Funct. (2024) 15:559–68. doi: 10.1039/d3fo03927g
52. Gomes, S, Lopes, C, and Pinto, E. Folate and folic acid in the Periconceptional period: recommendations from official health organizations in thirty-six countries worldwide and who. Public Health Nutr. (2016) 19:176–89. doi: 10.1017/s1368980015000555
53. Patel, KR, and Sobczyńska-Malefora, A. The adverse effects of an excessive folic acid intake. Eur J Clin Nutr. (2017) 71:159–63. doi: 10.1038/ejcn.2016.194
54. Henry, CJ, Nemkov, T, Casás-Selves, M, Bilousova, G, Zaberezhnyy, V, Higa, KC, et al. Folate dietary insufficiency and folic acid supplementation similarly impair metabolism and compromise hematopoiesis. Haematologica. (2017) 102:1985–94. doi: 10.3324/haematol.2017.171074
55. Zhao, G, Deng, J, Shen, Y, Zhang, P, Dong, H, Xie, Z, et al. Hyperhomocysteinemia is key for increased susceptibility to Pnd in aged mice. Ann Clin Transl Neurol. (2019) 6:1435–44. doi: 10.1002/acn3.50838
56. Gil Martínez, V, Avedillo Salas, A, and Santander, BS. Vitamin supplementation and dementia: a systematic review. Nutrients. (2022) 14:1033. doi: 10.3390/nu14051033
57. Vegrim, HM, Dreier, JW, Alvestad, S, Gilhus, NE, Gissler, M, Igland, J, et al. Cancer risk in children of mothers with epilepsy and high-dose folic acid use during pregnancy. JAMA Neurol. (2022) 79:1130–8. doi: 10.1001/jamaneurol.2022.2977
58. Fardous, AM, and Heydari, AR. Uncovering the hidden dangers and molecular mechanisms of excess folate: a narrative review. Nutrients. (2023) 15:4699. doi: 10.3390/nu15214699
59. Mattson, MP, and Arumugam, TV. Hallmarks of brain aging: adaptive and pathological modification by metabolic states. Cell Metab. (2018) 27:1176–99. doi: 10.1016/j.cmet.2018.05.011
60. Xie, S, Xu, SC, Deng, W, and Tang, Q. Metabolic landscape in cardiac aging: insights into molecular biology and therapeutic implications. Signal Transduct Target Ther. (2023) 8:114. doi: 10.1038/s41392-023-01378-8
61. López-Otín, C, Blasco, MA, Partridge, L, Serrano, M, and Kroemer, G. The hallmarks of aging. Cell. (2013) 153:1194–217. doi: 10.1016/j.cell.2013.05.039
62. Zhou, YH, Tang, JY, Wu, MJ, Lu, J, Wei, X, Qin, YY, et al. Effect of folic acid supplementation on cardiovascular outcomes: a systematic review and Meta-analysis. PLoS One. (2011) 6:e25142. doi: 10.1371/journal.pone.0025142
63. Fratoni, V, and Brandi, ML. B vitamins, homocysteine and bone health. Nutrients. (2015) 7:2176–92. doi: 10.3390/nu7042176
64. Espuch-Oliver, A, Vázquez-Lorente, H, Jurado-Fasoli, L, de Haro-Muñoz, T, Díaz-Alberola, I, López-Velez, MDS, et al. References values of soluble Α-klotho serum levels using an enzyme-linked immunosorbent assay in healthy adults aged 18-85 years. J Clin Med. (2022) 11:2415. doi: 10.3390/jcm11092415
65. Hsu, SC, Huang, SM, Lin, SH, Ka, SM, Chen, A, Shih, MF, et al. Testosterone increases renal anti-aging klotho gene expression via the androgen receptor-mediated pathway. Biochem J. (2014) 464:221–9. doi: 10.1042/bj20140739
66. Dote-Montero, M, Amaro-Gahete, FJ, De-la, OA, Jurado-Fasoli, L, Gutierrez, A, and Castillo, MJ. Study of the Association of Dheas, testosterone and cortisol with S-klotho plasma levels in healthy sedentary middle-aged adults. Exp Gerontol. (2019) 121:55–61. doi: 10.1016/j.exger.2019.03.010
Keywords: klotho, folate, dietary, intake, aging, NHANES
Citation: Liu Y, Zhou C, Shen R, Wang A, Zhang T and Cao Z (2024) Dietary folate intake and serum klotho levels in adults aged 40–79 years: a cross-sectional study from the national health and nutrition examination survey 2007–2016. Front. Nutr. 11:1420087. doi: 10.3389/fnut.2024.1420087
Edited by:
Julius Liobikas, Lithuanian University of Health Sciences, LithuaniaReviewed by:
Cecilia Zanin Palchetti, University of São Paulo, BrazilXu Zhu, Hunan University of Chinese Medicine, China
Copyright © 2024 Liu, Zhou, Shen, Wang, Zhang and Cao. This is an open-access article distributed under the terms of the Creative Commons Attribution License (CC BY). The use, distribution or reproduction in other forums is permitted, provided the original author(s) and the copyright owner(s) are credited and that the original publication in this journal is cited, in accordance with accepted academic practice. No use, distribution or reproduction is permitted which does not comply with these terms.
*Correspondence: Zhengyuan Cao, MTg5ODUxNzIzMzJAMTYzLmNvbQ==