- 1Institute of Food Science Research, CIAL (CSIC-UAM, CEI UAM+CSIC), Madrid, Spain
- 2Teagasc Food Research Centre, Fermoy, Ireland
- 3INRAE – Institut Agro, STLO, Rennes, France
- 4Agroscope, Bern, Switzerland
Protein is an essential macronutrient in our diet, source of nitrogen and essential amino acids, but the biological utilization of dietary protein depends on its digestibility and the absorption of amino acids and peptides in the gastrointestinal tract. The methods to define the amount and the quality of protein to meet human nutritional needs, such as the Digestible Indispensable Amino Acid Score (DIAAS), require the use of animal models or human studies. These in vivo methods are the reference in protein quality evaluation, but they are expensive and long-lasting procedures with significant ethical restrictions. Therefore, the development of rapid, reproducible and in vitro digestion methods validated with in vivo data is an old demand. This review describes the challenges of the in vitro digestion methods in the evaluation of the protein nutritional quality. In addition to the technical difficulties to simulate the complex and adaptable processes of digestion and absorption, these methods are affected by similar limitations as the in vivo procedures, i.e., analytical techniques to accurately determine bioavailable amino acids and the contribution of the endogenous nitrogen. The in vitro methods used for the evaluation of protein digestibility, with special attention on those showing comparative data, are revised, emphasizing their pros and cons. The internationally harmonized digestion protocol proposed by the INFOGEST network is being adapted to evaluate protein and amino acid digestibility. The inter-laboratory reproducibility of this protocol was demonstrated for dairy products. The in vivo/in vitro comparability results obtained to date with this protocol for several plant and animal sources are promising, but it requires an extensive validation with a wider range of foods and substrates with known in vivo digestibility. These in vitro methods will probably not be applicable to all foods, and therefore, it is important to identify their limitations, not to elude their use, but to apply them within the limits, by using the appropriate standards and references, and always as a complementary tool to in vivo tests to reduce their number.
1 Introduction
Protein is an essential macronutrient in our diet, source of nitrogen and essential amino acids. In human nutrition, the term protein nutritional quality refers to the ability of a protein to meet human requirements in essential amino acids and fulfill the physiological needs (1). The biological utilization of dietary proteins depends on their digestibility and the absorption of amino acids and di- and tri- peptides in the gastrointestinal tract. Protein digestibility is linked to its unique amino acid composition, which, in turn, determines the folding state of the protein. For instance, the gastric survival of some globular proteins, such as milk β-lactoglobulin is well known (2), as well as the intestinal resistance of proline-rich protein domains due to the limited intestinal cleavage of the amide bond of proline residues (3). Post-translational modifications, especially glycosylation and phosphorylation, also confer additional gastrointestinal resistance to the protein, as occurs for casein phosphorylated regions that have been found at different sections of the gastrointestinal tract (4, 5). In addition, proteins are often included in supramolecular structures, such as, protein bodies, micelles, fibers, or entrapped in cellular structures surrounded by non-digestible polysaccharides that limit the access of gastrointestinal enzymes (6, 7). Additionally, food products are commonly subjected to different technological processes to improve sensory properties, ensure safety or extend shelf-life, and these processes can also affect protein digestibility. While soft heat treatments denature globular proteins and inactivate anti-nutritional factors which increase digestibility, more severe treatments lead to protein aggregation, cross-linkages, or non-enzymatic browning, decreasing digestibility (8).
Given the complexity of the digestion and absorption processes and the importance to accurately define the amount and the quality of protein required to meet human nutritional needs, protein quality evaluation is being subject to numerous studies and updates. As a result of the FAO Expert Consultation on Protein Quality Evaluation in Human Nutrition held in 2011, a new protein quality index, the Digestible Indispensable Amino Acid Score (DIAAS) was proposed to replace the Protein Digestibility Corrected Amino Acid Score (PDCAAS) (9). DIAAS reflects the balance of amino acid digestibility determined at the terminal ileum, and describes protein quality better than PDCAAS (10). For the calculation of DIAAS, protein digestibility is based on the true ileal digestibility of each amino acid, preferably determined in humans, but if this is not possible, in growing pigs or in growing rats, in that order. In this report, the importance of treating each indispensable amino acid as an individual nutrient was also highlighted, and therefore, the digestibility is calculated as the oro-ileal disappearance of each amino acid. A dataset is being built based on the true or standardized ileal amino acid digestibility of a wide range of foods and ingredients. However, all these indexes, PDCAAS and DIAAS, and other previously used methods like the protein efficiency ratio (PER), include the use of animal models, or human studies. Consequently, dietary protein is the sole food macronutrient that requires animal or human testing for regulatory purposes.
These in vivo methods, although are the “gold standard” in protein quality evaluation, have important drawbacks. Animal trials are expensive and long-lasting methods with ethical restrictions. In addition to the policies on experimental animals that lead to follow the principle of the 3Rs (replacement, reduction and refinement), the social demand to reduce the number of animals for experimental purposes is currently growing, as well as, the demand for animal-free food, motivated by environmental and animal welfare reasons. In addition, because protein digestibility is affected by the food matrix and food composition and the technological treatment or the cooking conditions applied, the number of trials to be run exponentially increases, and makes the use of animal or human tests unfeasible.
Therefore, the development of rapid, reproducible and in vitro digestion methods that allow the estimation of the protein nutritional quality is an old demand. Despite the huge efforts done, especially in the field of animal nutrition [reviewed by Moughan (11)], these methods have not been sufficiently validated with appropriate in vivo data, i.e., ileal and not fecal protein digestibility, to reach sufficient confidence. The aim of this review is to present the actual status of the available in vitro methods to calculate protein digestibility with a view on past developments and special focus on the in vivo/in vitro comparability. The scope, uses and limitations of these in vitro procedures will be discussed, as well as, the work needed for the future application of these methods in routine protein quality evaluation.
2 Challenges of the in vitro methods
2.1 Simulate the in vivo digestion: a difficult task
Over the last 40 years, there has been interest in simulating human digestion in vitro, and specifically protein digestibility, since this knowledge is crucial in different areas, going from the nutritional assessment of novel foods and ingredients to the evaluation of protein allergenicity. However, human digestion and absorption are complex, multistage and adaptable processes in which several factors are involved (12). Thus, in vitro simulation of the digestion and absorption is a technically difficult, if not impossible, task. In this sense, although there are conditions that can be reproduced in vitro, with more or less success, such as, gastrointestinal enzymes, coenzymes and cofactors, pH, or temperature, there are other variables, such as, mechanical forces, regulation by gastrointestinal hormones, action of the intestinal microbiota or the participation of other organs, that are difficult to reproduce (13, 14). Furthermore, several studies have shown that the digestive capacity is adaptable, and for instance, the enzyme release at different levels of the gastrointestinal tract is regulated by the amount of ingested food (15, 16). This aspect, together with the ability of the products of digestion to modulate the intestinal function, adds extra complexity to the digestive process (17, 18). Although gut microbiota plays a critical role in digestion, its effects on protein quality evaluation could be overlooked in an in vitro approximation, since the goal of these methods is to simulate gastrointestinal digestion up to the ileum.
2.2 Simulate absorption and analysis techniques
Another important challenge of the in vitro methods to evaluate protein digestibility is the definition of the digestible-, bioavailable- or absorbable-fraction. Because the calculation of in vivo protein digestibility is based on the difference between the amount of each amino acid ingested and that non-absorbed, many in vitro methods have tried to reproduce or approximate these digestible and non-digestible fractions through dialysis, filtration or protein precipitation (14). However, other methods have estimated protein digestibility in the whole digest, like in the pH-drop or pH-stat methods, as will be described later on. However, as it will be commented, these methods based on pH measurement or monitoring were found to be susceptible to the buffering capacity of components of some food materials (19, 20).
In order to improve the evaluation of protein and amino acid digestibility, the resulting digestible and non-digestible fractions can be analyzed by using the same analytical approaches as the ones used in the in vivo assays, i.e., total nitrogen by Kjeldahl or Dumas, and determination of total amino acids by gas chromatography (GC) or HPLC. The different methodologies used for protein quantification can generate discrepancies in the data. The Kjeldahl method is considered the standard method for the estimation of nitrogen in food, because of its universality and good reproducibility in liquid and solid samples. Kjeldahl is a chemical method that determines the nitrogen concentration released during digestion with strong acids (21). Dumas is a high temperature combustion method, and the elemental nitrogen is detected by a thermal conductivity detector. However, because not all of the food nitrogen comes from proteins, these two methods do not give a measure of the true protein. Results obtained with Dumas are usually a little bit higher than those with Kjeldahl due to the detection of nitrogen compounds like nitrates, nitrites and heterocyclic compounds that are not completely quantified by Kjeldahl. In this sense, both methods might need determination of the non-protein nitrogen fraction depending on the food evaluated, and more importantly, an accurate nitrogen to protein conversion factor (NPCF) to convert the nitrogen values into protein. For many foods and ingredients, an overestimation of protein content can result from the use of the standard nitrogen correction factor 6.25 (22), and this will be translated into an underestimated protein digestibility value.
In both, the in vivo and in vitro assays, an acidic hydrolysis with 6 N HCl at 110°C has to be performed for 18–24 h prior to total amino acid determination by GC or HPLC. The effect of this acidic hydrolysis on amino acid analysis was questioned by Darragh and Moughan (23), who showed that the hydrolysis process can generate an erroneous estimate of the amino acid composition. This is due to the presence of amino acids that require times greater than 24 h to cleave the peptide bond (isoleucine, leucine, and valine) and others, considered labile amino acids, which can be partially destroyed before measurement (serine and threonine). In this context, proteins produce different rates of release and loss of amino acids, depending on their amino acid composition, causing an inaccurate quantification. Furthermore, the effect of acid hydrolysis on the chemical integrity of amino acids has been a topic of great scientific interest for years. It is well known that during thermal processing and storage, some amino acids such as methionine, cysteine, threonine, and tryptophan become unavailable, decreasing their bioavailability between 1 and 10%, as in the case of histidine (24). This aspect is especially notable for lysine amino acids, which are easily damaged during the food processing. The ɛ-amino group of lysine can react with many compounds such as reducing sugar, vitamins, fats, polyphenols, generating reactions that produce isopeptides and causing a degradation of lysine. Of these reactions, the most important occurs when, during thermal processing, the amino group reacts with the reducing sugar forming early or late Maillard compounds, which generates a decrease in the availability of lysine. When the Maillard reaction is advanced, the lysine is completely destroyed and cannot be recovered. However, during the early stages of the Maillard reaction, a portion of the structurally altered lysine (Amadori products) is partially hydrolysed in the presence of strong acids that reverse to lysine. However, such reversion does not occur during gastrointestinal digestion (25, 26). This fact leads to an overestimation of lysine quantification in processed foods caused by the acid hydrolysis step that takes place during conventional amino acid analysis. For this reason, the ultimate measure of available lysine is considered the absorbed reactive lysine and new methods, such as the isotope method or the oxidation of an indicator amino acid, have been described (27).
Other methods widely used to measure protein hydrolysis degree are those based on the reaction of primary amino groups, such as the trinitrobenzenesulfonic acid (TNBS) or the o-phthaldialdehyde (OPA) procedure. However, the precision of these methods may depend on the method and the protein substrate being hydrolysed. For instance, several studies have shown that OPA and cysteine react weakly due to the sulfhydryl group of cysteine, generating an unstable product (28, 29). This aspect makes the OPA method unsuitable for quantifying the degree of hydrolysis in cysteine-rich substrates. Furthermore, TNBS or OPA do not react with secondary amino acids such as proline or hydroxyproline (30).
2.3 Enzymes and blank of enzymes
One of the critical points in the in vitro digestion protocols is the selection of the enzymes and conditions (pH, digestion times, and salt concentration) to mimic physiological digestion. To study protein digestibility, proteases of porcine origin that are commercially available, have been widely used, specifically, porcine pepsin for the gastric phase and porcine pancreatic extracts containing proteolytic, lipolytic and amylolytic activities or individual proteolytic enzymes (31). The physiological protease concentrations at different segments of the gastrointestinal tract have been revised to fix conditions in some in vitro protocols (32, 33). In addition, other methods included peptidases of bacterial origin to simulate the carboxy- and amino-peptidase activity of intestinal brush border enzymes (34). It is true that when the food contains a high fat or starch content, an insufficient digestibility of these macronutrients can affect protein digestibility, and amylolytic and lipolytic enzymes are less accessible or are available at high prices. Starch digestion starts in the oral phase and, and although it is inactivated by the low pH in the stomach, some activity may persist within the food bolus. However, oral amylase is not included in most protocols due to its high price. Similarly, lipid digestion starts in the stomach by the action of gastric lipase that reaches activities of ca 120 U/mL in gastric fluid (35). Due to the limited accessibility of human gastric lipase, and taking into account the triglycerol stereospecificity and pH stability, dog or rabbit gastric lipases have been proposed as closest substitutes (36, 37), however gastric lipase is not used in most of the in vitro methods to evaluate protein nutritional quality. Bile salts have also been reported to improve the protein hydrolysis by the action of pancreatic proteases (38), however, not all in vitro protocols include conjugated bile acids in the intestinal phase. More importantly, as detailed later on (Section 4), the key to ensure batch-to-batch and inter-laboratory reproducibility is the standardization of enzymatic activity. Most of the in vitro digestion protocols add a given amount of enzyme or fix an enzyme/substrate ratio (E/S) on weight basis. This adds an important source of variability since the enzymatic activity of commercial enzymes varies enormously from batch to batch and during prolonged or inappropriate storage.
During the simulated gastrointestinal digestion, the use of enzymes, pancreatic extracts or mucins adds a significant amount of protein that depends on the E/S. In a similar way as occurs in in vivo trials, the amount of “added nitrogen” needs to be subtracted in the final calculations of protein and amino acid digestibility. In those methods that separate the digestible and non-digestible protein fractions, this deduction should be done in one or in both fractions. Due to the high degree of autolysis of the digestive enzymes, in particular in absence or in low dietary protein concentrations (39), the use of water or simulated physiological fluids as blank will affect the calculation, underestimating digestibility.
2.4 Other factors that affect protein digestibility
The presence of antinutritional factors (ANF), i.e., lectins, saponins, polyphenols, or trypsin inhibitors, or the fiber content can also influence in vivo protein digestibility. ANF could compromise the protein digestibility by inhibiting the accessibility of the digestive enzymes to the protein or inhibiting enzyme activity (40). Trypsin inhibitors, found in field pea, peanut, wheat, lupin, and soybean, have been demonstrated to be capable of reducing the ability to bind the active site of the enzyme, while lectins may interfere with the digestion and absorption of nutrients (41). Furthermore, polyphenols have been shown to generate a complex with the digestive enzymes, inactivating them and therefore reducing the digestibility of proteins (13, 42). Moreover, fiber consumption has shown several effects on the gastrointestinal tract, from reducing enzymatic activity in the lumen and transit time to protecting the enzymes against degradation or stimulating the microbiome activity in the digestive tract. However, the effect of these compounds in the in vitro assays is still unknown and will depend on the conditions, especially the E/S used, which makes it difficult to simulate in vivo digestion with in vitro assays (43).
3 Historical overview
During the last decades, different in vitro digestion methods have been developed to evaluate protein digestibility, and thus, nutritional quality of foods and feed. The number of articles dealing with this subject is enormous with more than 5,000 publications from 1990 to date. Therefore, this historical overview will be limited to dietary proteins, and with a special emphasis on those works showing in vivo/in vitro comparative data. Most of these methods are based on enzymatic hydrolysis, performed in a one- or two-step process, by using a single or a combination of enzymes, often being gastrointestinal proteases from porcine origin. Hydrolysis conditions, pH and temperature, are generally fixed at the maximum for each enzyme with pepsin hydrolysis carried out at acidic pH (around pH 2) and pancreatic enzymes used near neutrality. Some methods to evaluate in vitro digestibility of dry matter in feedstuffs proposed to account for microbial degradation at the large intestine by adding a multienzyme step containing a wide range of carbohydrases including cellulase, hemicellulose, arabinose, xylanase and others (44, 45). Differences between approaches are given by the E/S and especially by the method employed to determine protein digestibility. Some methods are based on a measurement of pH (pH drop or pH stat) while others are based on the separation of a digestible or absorbable fraction by various procedures going from ultrafiltration or dialysis to the use of protein precipitating agents. Therefore, in this section, in vitro methods are classified by the principle used to evaluate protein digestibility. Table 1 collects different in vitro methods used to assess protein and amino acid digestibility in comparison to in vivo data where the limitations have been specified.
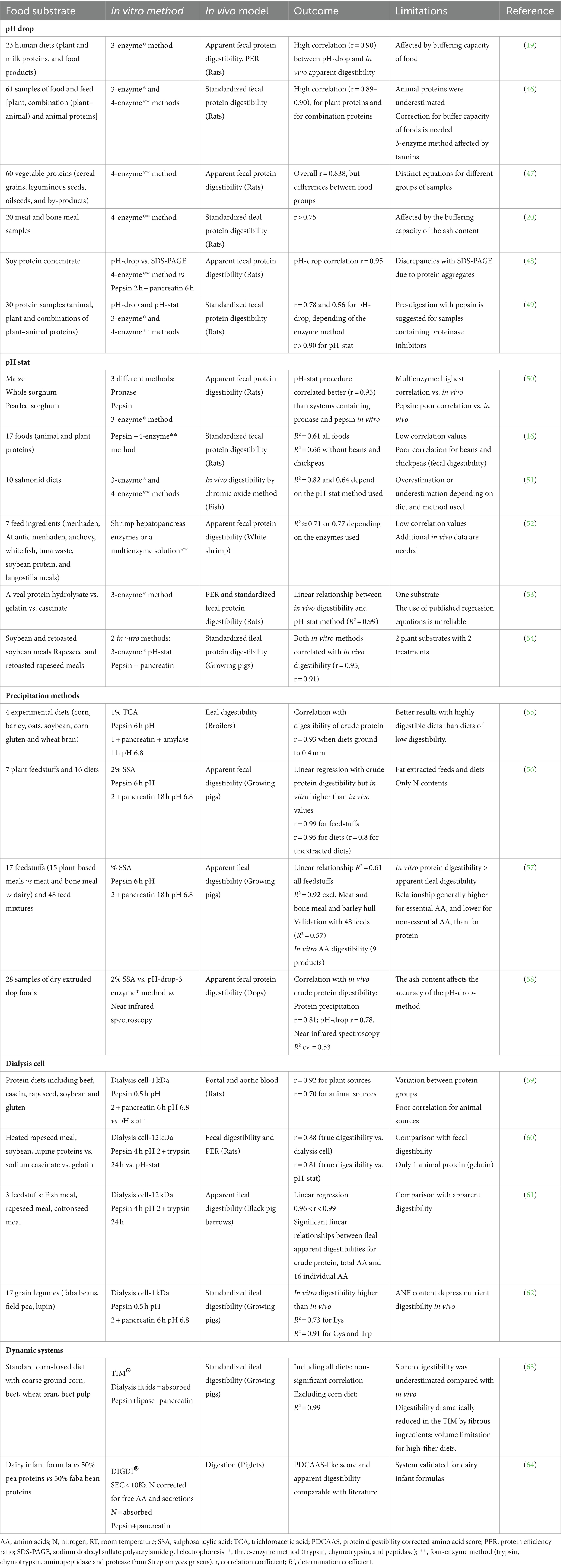
Table 1. In vitro methods used to assess protein and amino acid digestibility in comparison to in vivo data.
3.1 Methods based on pH measurement
During protein hydrolysis, release of protons and amino acids from the cleaved peptide bonds results in changes in pH. These methods lie on the correlation between the rate of hydrolysis degree and protein digestibility. Hsu et al. developed a multi-enzyme method (three-enzyme method, trypsin + chymotrypsin + peptidase) for the evaluation of protein digestibility. Specifically, they showed that the pH-drop after 10 min of digestion with the three-enzyme solution of 23 human diets, mainly vegetables and dairy foods, was highly correlated (r = 0.90) with in vivo PER values in rats. However, substances with high buffering capacities could affect the results. Despite this, the pH-drop methods was able to predict the apparent digestibility of proteins. In addition, the trypsin inhibitory activities and the effect of heat processing on digestion could be detected (19). Two years later, Satterlee et al. slightly modified the Hsu et al. protocol by including an extra 10 min of digestion with a Streptomyces griseus protease (four-enzyme method). Numerous authors have evaluated the in vitro digestion process of several food sources through the pH-drop method. In 1981, Petersen and Eggum studied the applicability of the three-enzyme (19) and four- enzyme combinations (65) on 61 samples of food and feed. Their results demonstrated a high correlation (r = 0.89–0.90) between the pH-drop results and fecal protein digestibility in rats, especially for plant proteins and for mixtures of plant and animal proteins. However, the predicted in vitro digestibility value for animal proteins significantly differed from the in vivo results (46). In 1983 the same researchers evaluated the in vitro protein digestibility of 18 protein sources using the three-enzyme method of Hsu et al. and the four-enzyme method of Satterlee et al. The results showed a greater in vitro-in vivo correlation for the three-enzyme method (r = 0.78) than for the four-enzyme method (r = 0.56). Despite the good correlations obtained, the estimations were significantly affected by the different buffering capacities of some food substances, which was considered a major drawback of the pH-drop method (49). This aspect was further demonstrated by Moughan et al. who compared the in vitro digestibility of 20 meat and bone meal samples by the pH-drop method with the values of true ileal digestibility in rats. It was concluded that pH estimation methods may be influenced or affected by the strong buffering capacity of the ash content, mainly mineral content, of food (20). For this reason, it was recommended to determine the pH-drop after a dialysis treatment to eliminate salts with buffering capacities (20). Other authors such as Kim et al. and Wolzak et al. showed in vitro-in vivo correlation values of r = 0.95 and r = 0.421 for soy protein concentrate, and 33 vegetable proteins, respectively (47, 48). However, the difference in the response for different types of food proteins made it necessary to use different regression equations to obtain realistic estimates of digestibility. This task presents a major challenge due to the complexity involved in categorizing foods in each class of food.
Pedersen and Eggum revised the pH-drop method and modified it slightly in order to avoid the effect of substances present in the protein that could influence the drop in pH. In the pH-stat procedure, the pH is kept constant at pH 8 by automatic titration (0.10 M-NaOH titrant) during the incubation with enzymes. At the end of the incubation period, the amount of alkali added is recorded and the value is used as an indirect measure of protein digestibility (43, 46). Using pH-stat, Pedersen & Eggum showed an improvement in the prediction of protein digestibility of 30 samples, compared to the pH-drop. A high correlation coefficient (r > 90) with fecal digestibility in rats was obtained in pH-stat method, improving the one obtained by the pH-drop method (0.56–0.78). However, the digestibility of some foods, such as egg powder, was underestimated, because of the content of trypsin and chymotrypsin inhibitors of egg. A pretreatment with alkali to improve the correlation coefficients was then recommended (49). The pH-stat method has been widely used to evaluate the digestibility of different protein sources. Eggum et al. showed a good agreement in vitro vs. in vivo (measured by fecal protein digestibility in rats) in 17 foods, with the exception of two legumes, beans and chickpeas. The authors discussed that the discrepancies obtained for these foods, suggesting that it could be due to the high bacterial growth with the consumption of certain legumes in the diet, which caused an increase in the excretion of nitrogen in the feces. Excluding these legumes the obtained in vitro and in vivo digestibility percentages were similar (86.3–100.0% in vitro and 73.1–96.8% in vivo), although the correlation coefficient was only acceptable (R2 = 0.66) (16). Better correlation coefficients were obtained by comparing the in vitro protein digestibility of maize, whole sorghum and pearled sorghum maize (r = 0.95) and 7 specific foods (R2 ≈ 0.75) with their in vivo apparent fecal protein digestibility (50, 52). In the same way, good and significant correlations (R2 = 0.82 and 0.64) were obtained when the protein digestibility of 10 salmonid diets were estimated by two the pH-stat in vitro assay methods and compared with in vivo digestibility in fish (51). Linder et al. measured the protein digestibility of an industrial veal protein hydrolysate, used as a gelatin-replacing ingredient for human consumption. The results showed a high correlation between fecal protein digestibility measured in rats and the pH-stat method (R2 = 0.99), although already published regression equations were used (53). Recently, high correlation coefficients were obtained between in vitro protein digestibility of processed soybean meal and rapeseed meal through the pH-stat method and the results obtained from standardized ileal digestibility in growing pigs (r = 0.95) (54).
In summary, the methods based on pH measurement were shown to be suitable for predicting digestibility in many foods, with high correlations in plant substrates. The method was found to be highly reproducible across 6 laboratories that estimated protein digestibility of 17 protein sources by using the 3-enzyme method in a pH-stat (66). However, by using these methods, the results of the entire complex digestion process were evaluated based on a mere measurement of pH or pH-change. In other words, the crucial information on protein digestion that could be extracted from the use of gastrointestinal enzymes was neglected. In addition, the significant differences found for animal proteins, the use of different correlation curves for different samples, and the fact that certain physical and chemical characteristics, such as calcium content or buffering capacity, may prevent an accurate estimation of digestibility, and are important drawbacks for the use of these methods.
3.2 Methods based on protein precipitation
In the methods described in this section, enzyme incubations are followed by measurements of the insolubilized material collected after filtration, although in some cases measurements on the filtrate, or alternatively on one of the separated fractions after centrifugation are conducted. Digestibility is then related to in vitro solubility or the definition of an absorbable or bioaccessible fraction and a residue or non-absorbable fraction.
Early methods included one-step incubations giving lower digestible protein values than those obtained in vivo, and were rapidly replaced by two-step digestion. In the pepsin-jejunal fluid, a two-step incubation with pepsin digestion for 4 h followed by a further 4 h digestion with pig jejunal fluid was used (67). In vitro digestibility of protein was calculated by the determination of dry matter and crude protein on the residue after centrifugation for 10 min at 1,250 × g at 5° C, on the basis of the original protein content of the diet. A two-stage incubation with pepsin for 6 h at pH 2 followed by an incubation with pancreatin at pH 6.8 for 18 h in borate buffer was further developed (68) thus providing an animal-independent method. To calculate the digestibility, 1% TCA final concentration was used, followed by centrifugation for 1 h at 2,000 × g. This method was applied for routine analysis in quality control of feeds and feed ingredients for poultry. By reducing the particle size of the test material, passing through a 0.4 mm sieve, the accuracy of predicting in vivo digestibility was increased for all the tested diets, that included corn, barley, oats, soybean, corn gluten and wheat bran as protein sources. The correlations between ileal digestibility in broilers and in vitro estimates were high (r = 0.93 for crude protein) (55). A modification of this method was presented by Babinszky et al., where pepsin incubation was performed at pH 1 on fat-extracted feed samples, and the residue after pancreatin + amylase incubation and 1% TCA precipitation was centrifuged at 3,500 × g for 15 min, after decanting over a nylon cloth (particle size 40 μm). This method found an improved correlation with fecal digestible protein in pigs by reaching regression values for feedstuff and diets of 0.99 and 0.95, respectively (56). The additional determination of nitrogen content on the filtrate gave a similar correlation but was abandoned as it was considered to be too laborious.
With the aim to recover solubilized but not fully degraded proteins, precipitation with sulphosalicylic acid was introduced, while undigested materials were submitted to the standardized filtration equipment for measuring dietary fiber (43). Magnetic stirring was included during the enzymatic incubations in order to assure effective starch degradation. By the use of this method, prediction of individual amino acids in eight common feedstuffs showed that the in vitro digestibility of the individual amino acids was close to the in vitro digestibility of nitrogen. Hervera et al. (58) adapted the last in vitro method for estimation of digestible energy of dog foods and compared it with the pH-drop methodology. The results showed a correlation of r = 0.78 between the pH-drop with the three-enzyme method and the apparent fecal digestibility in dogs but higher accuracy, r = 0.81, was shown with the in vitro method using precipitation with sulphosalicylic acid (58). Wada and Lönnerdal determined digestibility in infant formulas by using total and non-protein nitrogen (NPN), i.e., soluble fraction in 12% final TCA concentration (69). They investigated the effect of industrial processing with in vivo digestibility using a suckling rat pup model in terms of chemical modifications and endurance of intact α-lactalbumin and β-lactoglobulin, but no direct in vivo-in vitro comparison was shown.
The role of nitrogen added in the form of enzymes was considered in further developments. When the in vitro digestibility of protein was calculated from the difference between nitrogen in the sample and the undigested residue after correction for nitrogen in the blank, it was shown that the resulting amino acid composition of the blank-derived protein was very close to reported values in the literature based on direct measurements of endogenous protein in vivo. Apparent ileal digestibility of individual amino acids was predicted in a similar way as for protein. The relationship was generally higher for essential amino acids, and generally lower for non-essential amino acids, than for protein (57). This procedure used precipitation with sulphosalicylic acid (2% final concentration) for 30 min at room temperature followed by rinsing with 1% sulphosalicylic acid of the filtered residues. A close relationship was found for the 17 single feedstuffs but meat and bone meal, and barley hull had to be excluded. The above conditions have been widely used to compare protein digestibility of different products, mainly using sulphosalicylic acid (62, 70) or TCA (71, 72) as precipitating agent.
In summary, the in vitro methods based on precipitation of a non-digestible fraction by using different agents such as TCA or sulphosalicylic acid have demonstrated good comparability with ileal digestibility in broilers and in pigs. Precipitation with sulphosalicylic acid after a 3-enzyme digestion protocol has shown higher accuracy than pH-drop when compared with dog fecal digestibility. The main advantage of these methods is the reproducibility of the precipitation step for the definition of a digestible and non-digestible fraction. However, the digestion conditions used by different authors would still require additional optimization and harmonization.
3.3 Methods using ultrafiltration or dialysis
These methods are based on the continuous removal of low-molecular-weight products from digested material by ultrafiltration or dialysis to prevent enzyme inhibition by end products.
A two step-digestion method in which the intestinal digestion products (free amino acids and low molecular weight peptides) were removed through a dialysis membrane was proposed in order to reduce enzyme inhibition by hydrolysis products (73). After a 30 min digestion step with pepsin enzyme: substrate of 1:250 (pepsin activity 3,152 units/mg protein), intestinal digestion took place with pancreatin for 6 h, at an E/S of 1:25, in a dialysis cell of a 1,000 Da molecular weight cut-off, for the continuous elimination of digested products with 10 mM sodium phosphate buffer, pH 7.5, as circulating dialysis buffer. The essential amino acids released during the intestinal phase from beef, casein, rapeseed, soybean and gluten correlated with plasma levels found in portal and aortic blood in rats fed with the same substrates (59). A good correlation was found for plant sources (r = 0.92–0.93), although lower values were reported for beef or casein (r = 0.70). This protocol was also applied to protein mixtures (74) and to 19 selected foods, showing differences between in vitro-in vivo amino acid digestibility depending on the protein source. These variations were not related to the amino acid concentration in the protein and it was proposed that the amino acid sequence as the factor leading overall protein and amino acid digestibility (75). The in vitro protein digestibility by use of a dialysis cell method and pH stat was compared with the in vivo PER and true digestibility of heated rapeseed meal, soybean and lupine proteins (60). In vivo, PDCAAS correlated with pH stat and dialysis cell values with r = 0.92 and 0.98, respectively, although PER was poorly correlated with the in vitro protein digestibility. Similar strategies but using dialysis tubes in the intestinal phase have been used to predict ileal protein digestibility of pig feedstuffs (61), obtaining linear regression equations between in vitro digestibilities and porcine ileal apparent digestibilities. Dialysis cells have been more recently used in the estimation of the protein digestibility of novel food protein sources, such as seaweeds, where the high fiber content affected protein digestibility, likely by reducing the accessibility of the proteolytic enzymes (76, 77).
In some works, the use of chromatography or ultrafiltration with different cut off membranes has been used to characterize the digestible fraction. Besides, the characterization of the non-dialyzed digest has been conducted by ion-exchange or size exclusion chromatography, and ultrafiltration. The undigested residues were separated by ion-exchange chromatography into basic-neutral, lightly acidic and acidic fractions further resolved by sequential ultrafiltration (cut-off 10 and 1 kDa). Interestingly, large proportions of leucine, lysine, arginine, phenylalanine and tyrosine were found as part of peptides smaller than 1 kDa, both in the dialysates and retentates, while glutamine, threonine, serine and asparagine appeared mostly in fractions >1 kDa, while after 6 h with pancreatin, most of the proline appeared in the basic-neutral fraction >1 kDa (78). When this procedure was applied in the comparison of casein, cod, soy and gluten proteins, animal proteins were digested at a greater rate than plant proteins, and more resistant peptides were largely rich in proline and glutamic acid (79).
The impact of cooking on animal and plant protein digestion has been evidenced by the use of this strategy. The increase in protein digestibility of white and brown beans (Phaseolus vulgaris) after cooking was found to be related to a higher extent of proteolysis, as monitored by SDS-PAGE and recovery of low molecular weight peptides (< 30 kDa) after ultrafiltration of the digests (80). On the contrary, meat protein digestion in a microreactor fitted with a 10 kDa cut-off membrane in the gastric compartment and 1 kDa cut-off dialysis membrane in the intestinal compartment showed a decrease of protein digestibility with meat cooking (81). This study showed superior precision with the use of a semi-automatic flow procedure in comparison with the test tube method. Analytical size exclusion chromatography has been used to determine digestibility of casein vs modified casein with the glycation product pyrraline. The size pattern was used to show that the digestibility decreased with increasing pyrraline concentration of the peptide mixtures. Moreover, further ultrafiltration of digests using 1 kDa cut-off indicated that 50–60% of pyrraline was included in peptides (82).
The methods based on in vitro digestion and dialysis or ultrafiltration have shown good correlation in the prediction of ileal protein digestibility of food and feed. Some of these approaches have been used to characterize the gastrointestinal digests in combination with chromatographic methods. Main weaknesses of these methods would derive from the limited reproducibility of the use of ultrafiltration devices and the unspecific bound of protein material to the membrane material.
3.4 Dynamic systems
Dynamic systems have been proposed as in vitro alternatives for human or animal studies as physiologically relevant, including peristaltic mixing of food, computer- controlled pH values and realistic gastrointestinal transit times. Moreover, small molecules are removed from the digesta with hollow fiber membranes. The TNO-developed TIM® system was tested to predict the true ileal digestibility of proteins including dairy, meat, wheat, faba bean or barley, and a linear relationship versus pig or calf data was obtained (83). A standard corn-based diet was compared with the same diet with coarse ground corn, 8% sugar beet pulp, 10% wheat bran, or 8% sugar beet pulp and 10% wheat bran. The dynamic model yielded digestibility coefficients comparable with in vivo ileal digestibility in growing pigs for the standard and coarse ground corn but the values were considerably affected by the incorporation of the fibrous ingredients. The linear fitting between the in vitro and the in vivo results for crude protein digestibility was not significant but resulted in R2 = 0.99 when the coarse ground corn diet was excluded from the regression (63).
Using the tiny-TIM, digestibilities of ovalbumin, cooked and raw chicken egg white, and casein showed similar values to values reported in humans (R2 = 0.96). The true ileal protein and amino acid digestibilities were used by the authors to estimate the DIAAS for immature herring egg proteins (84). More recently, cumulative true ileal digestibility of nitrogen data has been reported during 5 h tiny-TIM, expressed as the percentage of the exogenous nitrogen intake, correcting for nitrogen in gastric residue (85). These values served to calculate DIAAS for different protein ingredients, alongside the corresponding limiting amino acid. The DIAAS values for rice, whey, and pea-based proteins were in agreement with those collected from literature, using pig ileal data. However, for soy and a second source of pea protein with different processing, the values were significantly lower than those previously described in literature. This was ascribed to treatments applied to these specific ingredients during processing, including alkaline or heat treatment, leading to protein aggregation or structural changes. An alternative source of discrepancy was related to differences in the innate protein features due to cultivar of growing conditions. A low (under 50%) bioavailability of the majority of amino acids and low N digestibility was found for the last two products. Isolates with lower DIAAS also showed lower protein solubility and increased protein aggregation, which was identified as a potential cause inhibiting digestion. Indeed, DIAAS positively correlated to protein solubility and N-bioaccessibility. The dynamic system developed at INRAE, DIDGI® was set up to mimic infant digestion upon an extensive analysis of literature on infant physiology and validated with piglet digestion (86). This system provided comparable results in vitro/in vivo for a reference dairy infant formula in terms of limiting essential amino acid, PDCAAS-like score and in vitro apparent digestibility. The last parameter was determined based on the soluble N lower than 10 kDa, as measured in the peptides by size exclusion chromatography and cumulated to the free amino acid nitrogen (64).
The use of dynamic systems to determine protein digestibility is still limited. Although these systems allow monitoring the progress and digestion kinetics, the calculation of the nitrogen mass balance could be more complex than in static systems. In addition to the difficulties to harmonize conditions in different apparatus, the availability of this sophisticated equipment could be an additional limitation to the extensive use of these methods.
4 INFOGEST static protocol applied to protein digestibility and protein quality analysis (in vitro DIAAS)
The INFOGEST static digestion protocol was developed during the COST Action INFOGEST1 with the main goal to harmonize the highly variable protocols used within the research laboratories interested in food digestion. The first INFOGEST consensus method (32), was followed by an improved and more detailed protocol in 2019 (33). Digestion parameters were based on currently available physiological data. The resultant peptides from the in vitro digestion with the INFOGEST protocol have been compared with human and pig peptidomic analysis showing comparable results for milk proteins (Figure 1). Compared to previous published protocols, the following points can be highlighted as the most important advantages, which helped to reduce experimental variability and improve reproducibility (87). Firstly, the protocol includes specific enzyme activity assays in order to harmonize the addition of enzymes based on their activity and not based on weight, as in previous published protocols. Secondly, due to the variable buffering capacity of different foods, the protocol requests to perform a pH test tube where the volumes of HCl to add in the gastric phase (to reach pH 3) and the volume of NaOH (to reach pH 7) in the intestinal phase, are tested for each food sample. And thirdly, the protocol provides indications on how the enzyme activities can be stopped after the gastric and intestinal phase of digestion, depending on the downstream analyses. The protocol was shown to be reproducible and robust in inter-laboratory experiments (87) and the results at the end of the intestinal phase were comparable to in vivo results (5, 88) although this has been proved so far only for dairy proteins.
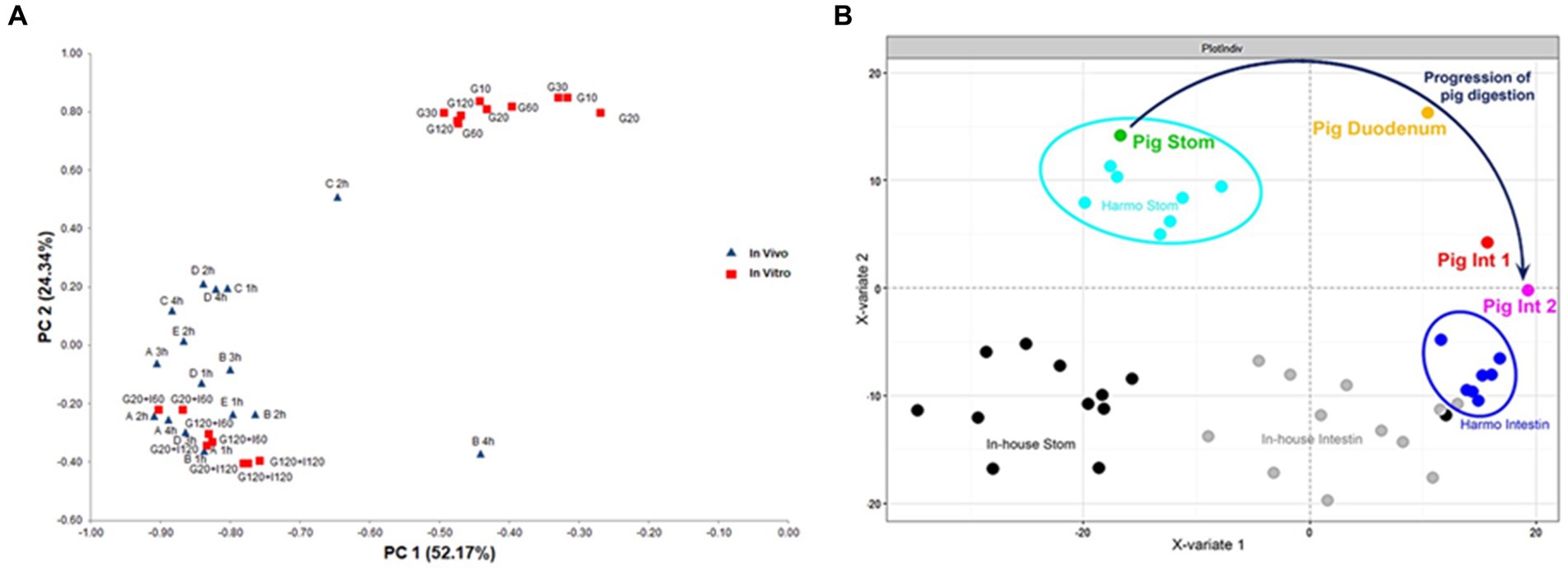
Figure 1. Comparison of in vitro digestion (INFOGEST protocol) vs in vivo (A: human jejunal digests; B: pig digests). (A) Principal component analysis score plot calculated with the frequency of appearance of each amino acid identified as part of a peptide from β-casein and αs1-casein. Different human subjects (blue triangles) are referred to with capital letters from A to E followed by the time of jejunal sampling (1, 2, 3, and 4 h). In vitro digests are represented with red squares. G, gastric; I, intestinal, followed by the time expressed in minutes of in vitro digestion. Reprinted with permission from Elsevier, by Sanchón et al. (5); (B) Partial least square analysis over all peptide patterns identified in the five most abundant milk proteins (β-, αs1-, αs2-, κ-casein, β-lactoglobulin). The average of eight pig samples is shown versus the harmonized or in-house digestion protocol, from previous interlaboratory studies. The arrow indicates the progression of digestion in the pig samples from Stom (stomach)-, Duodenum, Int 1 (proximal jejunum)-, to Int. 2 (median jejunum)- phases (B). Reprinted with permission from Taylor & Francis, by Bohn et al. (17).
Although the INFOGEST protocol increased harmonization of digestion experiments, critical steps in the protocol and further adaptations were proposed. The INFOGEST sub-group (WG4) tested lipase activity in several collaborative studies and found a high variability due to unprecise descriptions in the original protocol. The detailed protocol elaborated by this group led to a significant reduction in variability and for the study of lipid digestion, therefore these recommendations should be considered (89). A similar work focusing on amylase activity is currently ongoing and in the near future an improved protocol for amylase activity will be proposed and published. Moreover, in order to better simulate digestion in different age groups, both protocols, the static and the semi-dynamic protocol were and are further adapted to infant and elderly conditions.
The static INFOGEST in vitro digestion protocol represents a good starting point on which the quantification of the protein digestibility could be based. Several recent publications based on the INFOGEST static protocol are focusing on the quantification of protein digestibility and are listed chronologically in Table 2. In this table, the main adaptations with regard to the original INFOGEST method, the amount of protein input, the separation of non-digestible from digestible material, the use of an enzyme blank, and the calculations of digestibility are compiled. The approaches to overcome the main challenges of the in vitro methods are discussed below.
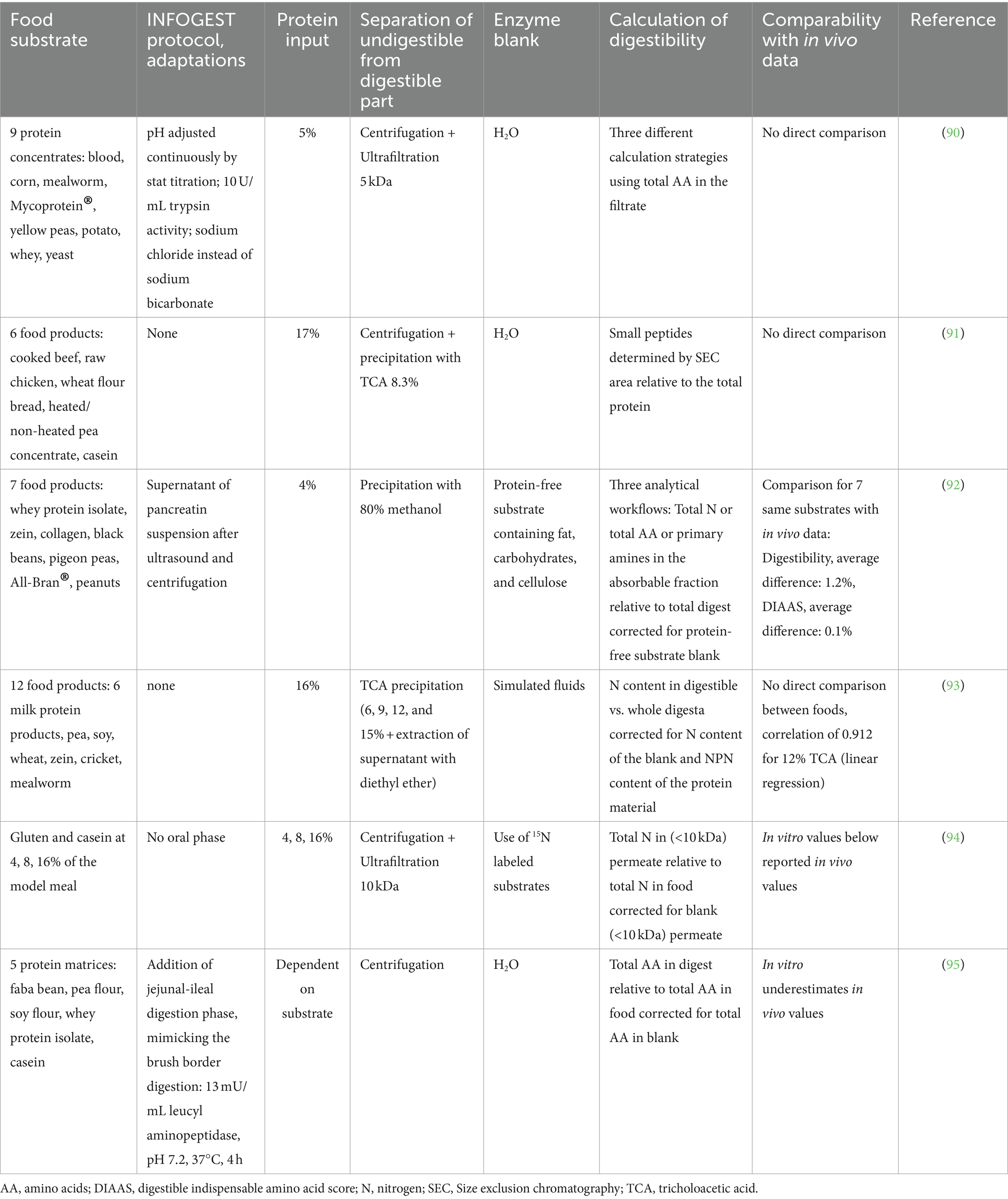
Table 2. In vitro methods based on the INFOGEST digestion protocol applied to the evaluation of protein and amino acid digestibility.
4.1 Enzyme/substrate ratio
The original INFOGEST protocol proposed for each digestion step a 1:1 ratio (w:w) between food and simulated fluid, ending up with a final ratio of 1:8 of food in digesta. No recommendation of nutrient normalization was proposed. However, in order to compare protein digestibility of different foods, a normalization may be needed. In four of the listed publications, protein input was normalized between 4 and 16% in the foods subjected to digestion. Increasing the amount of protein entering into the system reduced digestibility, as was observed in the case of different amounts of TCA soluble casein after size exclusion chromatography (91) and for casein and gluten digestibility, testing 4, 8, and 16% of protein input (94). Another approach to increase the food to enzyme ratio is the adaptation of digestive enzymes as proposed by Ariëns et al. (90) to reduce the background of enzymes. The authors reduced the addition of pancreatin from 100 U/mL of digesta by a factor of 10 to 10 U/mL and observed no impact on released NH2 during digestion of whey protein isolate, which represents a highly digestible substrate. It would be interesting to test if this observation is also correct for substrates with lower digestibility. Alternatively, a reduction in enzyme background was achieved by Sousa et al., by using the supernatant of the pancreatin suspension after solubilization with ultrasound and subsequent centrifugation (92). This procedure did not reduce the trypsin activity in the pancreatin supernatant.
4.2 Separation of digestible from non-digestible material
At the end of the intestinal phase of the original INFOGEST protocol, all products are in the same container. In order to assess protein digestibility, digestible and non-digestible fractions need to be separated. Different approaches were used by various authors, such as centrifugation (95), or ultrafiltration at different cut-off sizes, such as 5 or 10 kDa (90, 94), corresponding to peptides of 45–90 amino acids in length, assuming an average weight of 110 Da per amino acid. The choice of the rather high molecular weight cut-off compared to in vivo (500 Da) was justified by the lack of brush border enzymes in the system (94). Moreover, the use of ultrafiltration could as well lead to loss of material, impacting the mass-balance and in consequence the digestibility of the tested substrates (90). A second approach applied in the different protocols was a precipitation step either with different concentrations of TCA (6–12%) (91, 93) or with MeOH (80%) (92). Depending on the downstream analysis, the precipitation agent could disturb the measurements and it was removed by extraction with diethyl ether (93) or simply be evaporated in the case of MeOH (92).
4.3 Consideration of the enzyme background
In both, in vivo and in vitro situations, the endogenous enzymes and background proteins need to be considered. Different solutions to this challenge have been suggested. Probably the most precise way of differentiating endogenous material from the food of interest represents the use of isotopically labeled food sources as has been used by Ménard et al. (94). In this study, 15N isotopically labeled casein and gluten were digested at different concentrations. Unfortunately, the generation of isotopically labeled substrates is not always possible, in addition to being time consuming and expensive, and therefore other solutions are requested. However, for experiments of proof of principle and validation, isotopic labeling would be the method of choice. As an alternative, the enzyme background was subtracted by performing a parallel digestion with H2O (90, 93–95) or using a protein-free food (92). However, as explained in Section 2, in the absence of substrate, a higher enzyme autolysis may occur (39, 94), which would cause an underestimated digestibility value.
4.4 Validation and standardization of in vitro protein digestibility protocols
Comparisons between in vitro and in vivo data were performed by four of the above-mentioned publications (Table 2). A high correlation between in vitro and in vivo DIAAS, as well as an agreement in limiting amino acid (DIAA) was demonstrated for the investigated substrates, although the in vitro digestibility values were below in vivo digestibilities in these studies (93, 95). In the same direction, a lower true in vitro digestibility value compared to in vivo was found for the two investigated substrates, casein and gluten (94). A direct comparison between in vivo and in vitro digestibility was performed for seven substrates (Figure 2, WPI (A) and black bean (B), representing two of the investigated substrates). The results showed a comparable digestibility with an average bias of 1.2% for all essential amino acids of the assayed substrates (Figure 2C). The DIAAS values were comparable with an average bias of 0.1% and a correlation of r = 0.96 between in vivo and in vitro results (92). It has to be highlighted that this latter study was carried out in vivo and in vitro by using identical substrates. In view of the published data, an increased number of substrates of different nature is needed to validate this in vitro model for digestibility.
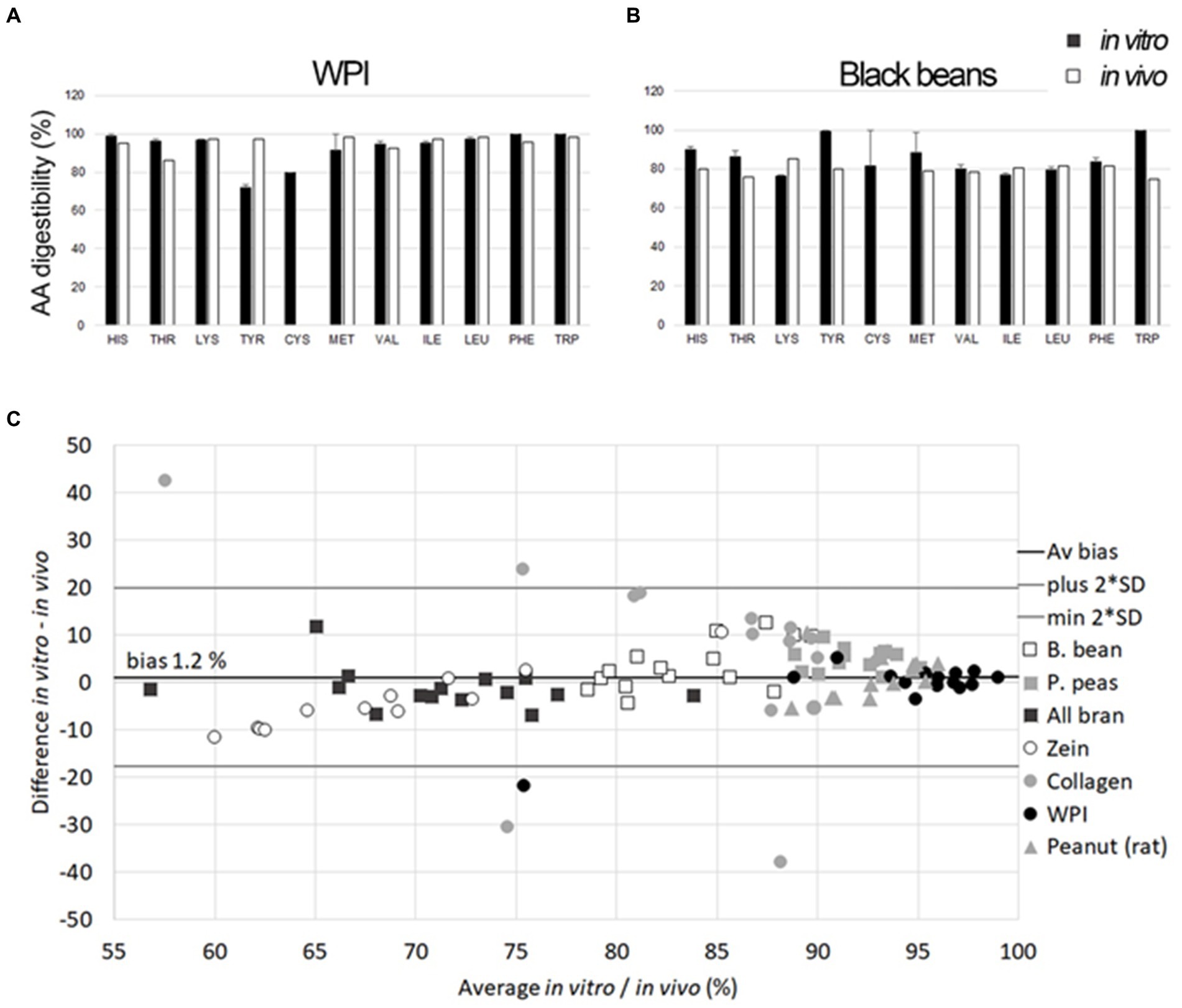
Figure 2. In vitro digestibility (y-axis: %) of individual amino acids (black) after IVD compared with in vivo data for whey protein isolate (WPI) (A) and B. bean (B) (mean pig and human values, white). Error bars are SEM of three individual in vitro experiments; Statistical comparison between in vitro and in vivo digestibility of essential amino acids AA (C), according to previous work (96), show the average digestibility of in vitro and in vivo results (x-axis) versus the differences between in vitro and in vivo digestibility (y-axis) of all essential amino acids of the comparisons of B. bean, P. peas, All bran, Zein, Collagen, WPI, and Peanut [in vivo rat data, (97)]. The mean bias between methods was 1.2% and upper and lower limits indicate ±2 * SD of the average difference. The comparison with in vivo DIAAR for SAA could not be calculated due to missing in vivo cysteine values.
4.5 Method repeatability, reproducibility, and standardization
Until recently, a major drawback of in vitro methods was the lack of comparability between different laboratories. In consequence, one of the major achievements of the INFOGEST network was to establish harmonized digestion protocols with satisfactory inter-laboratory reproducibility. Within the same INFOGEST network, protein digestibility is currently tested with several dairy products (SMP, whole milk powder, whey protein isolate, yogurt, and gruyere cheese) and with two plant sources (soy protein isolate, chickpea), applying the analytical workflow published by Sousa et al. (92). In parallel to these collaborative studies, a standardization of the method within the International Dairy Federation (IDF) and International Standardization Organization (ISO) was launched. The precision data (repeatability, reproducibility) obtained in the inter-laboratory trials will be included in the future IDF/ISO standard method, with the final goal to obtain a robust and validated protocol allowing the analysis of protein digestibility.
5 Conclusions and future prospects
Several in vitro methods to be applied in the assessment of the protein nutritional quality have been developed during the last 40 years. In vitro digestion models have been shown to provide a good estimation of protein digestibility and of the nutritional scores, such as the DIAAS, and appear to be a realistic alternative to animal trials in the near future. Some of them have demonstrated good agreement with in vivo digestibility data with high correlation coefficients or close protein and amino acid digestibility values. It is important to note that most of these correlations were established protein fecal protein digestibility, while these methods do not take into account the action of microbiota, and thus, when possible, the comparison with standardized or true ileal digestibility data is preferred.
Despite the huge effort done, in vitro methods have not reached sufficient confidence to be used for the routine evaluation of protein and amino acid digestibility due to discrepancies in certain substrates. The conditions of static in vitro methods are fixed, in the most optimal situation by mimicking as closely as possible the digestive conditions: enzyme/substrate ratios, standardized enzymatic activity, and a digestion time, etc. However, it is highly unlikely that the in vitro conditions will be able to simulate all types of foods, matrices, and ingredients without adaptations. For instance, the work performed to date with the INFOGEST method has already detected the need to test protein isolates the same as done in vivo, i.e., incorporated in a protein-free food matrix. Similarly, substrates with low protein content or having a high content of trypsin inhibitors will require protocol adaptations. Therefore, it is crucial to carry out in vitro protein and amino acid digestibilities of a wide range of substrates with previously measured ileal digestibilities in order to identify limitations and propose adaptations to the in vitro protocols. In this sense, new in vivo data obtained on biological fluids are needed to refine these in vitro digestion conditions. Such work is currently being completed in the frame of a cooperation between INFOGEST and the UNGAP network on drug absorption. Moreover, a large proportion of the studies comparing in vitro to in vivo values has been made on protein ingredients from animal, plant or alternative sources, although humans do not consume ingredients, but food that adds complexity. More work is needed to apply in vitro models to determine protein digestibility on real food where the other constituents of the food matrix can interact with each other, especially when they are processed or ultra-processed, and can limit the accessibility of digestive enzymes to their protein substrates.
Static in vitro digestion models are relatively simple techniques with a huge potential for assessing protein digestibility. However, based on the experience within the INFOGEST network, even with protocols extensively described step by step, some slight differences may lead to significant discrepancies. It is important that the validation of these in vitro methods is run in different laboratories to generate enough reproducibility and repeatability data. A huge effort is being done in INFOGEST to train people on how to use the model in a proper way and training schools organized in Europe, South America, Australia and Canada. Videos showing the different steps of the model, the digestive enzyme calibration or the quantification of bile salts have been made available on the INFOGEST YouTube channel.2 All these events and tools will highly improve the reproducibility of the model, leading to more robust interlaboratory data.
Author contributions
GS-S: Writing – original draft, Writing – review & editing, Investigation, Formal analysis. BM: Writing – original draft, Writing – review & editing, Resources. AB: Writing – original draft, Writing – review & editing. DD: Writing – original draft, Writing – review & editing. LE: Writing – original draft, Writing – review & editing. IR: Writing – original draft, Writing – review & editing, Conceptualization, Funding acquisition, Supervision, Project administration.
Funding
The author(s) declare financial support was received for the research, authorship, and/or publication of this article. This work has received financial support from grant PID2019-107663RB-I00 (MCIN/AEI/10.13039/501100011033) and project i-Link22018 financed by CSIC.
Conflict of interest
The authors declare that the research was conducted in the absence of any commercial or financial relationships that could be construed as a potential conflict of interest.
Publisher’s note
All claims expressed in this article are solely those of the authors and do not necessarily represent those of their affiliated organizations, or those of the publisher, the editors and the reviewers. Any product that may be evaluated in this article, or claim that may be made by its manufacturer, is not guaranteed or endorsed by the publisher.
Footnotes
References
1. Kurpad, AV. Protein: quality and sources. Encycl Hum Nutr. (2013) 123–30. doi: 10.1016/B978-0-12-375083-9.00241-5
2. Kitabatake, N, and Kinekawa, Y-I. Digestibility of bovine milk whey protein and β-lactoglobulin in vitro and in vivo. J Agric Food Chem. (1998) 46:4917–23. doi: 10.1021/jf9710903
3. Hausch, F, Shan, L, Santiago, NA, Gray, GM, and Khosla, C. Intestinal digestive resistance of immunodominant gliadin peptides. Am J Physiol Gastrointest Liver Physiol. (2002) 283:G996–G1003. doi: 10.1152/ajpgi.00136.2002
4. Meisel, H, and Frister, H. Chemical characterization of a caseinophosphopeptide isolated from in vivo digests of a casein diet. Biol Chem Hoppe Seyler. (1988) 369:1275–9. doi: 10.1515/bchm3.1988.369.2.1275
5. Sanchón, J, Fernández-Tomé, S, Miralles, B, Hernández-Ledesma, B, Tomé, D, Gaudichon, C, et al. Protein degradation and peptide release from milk proteins in human jejunum. Comparison with in vitro gastrointestinal simulation. Food Chem. (2018) 239:486–94. doi: 10.1016/j.foodchem.2017.06.134
6. Bhattarai, RR, Dhital, S, Wu, P, Chen, XD, and Gidley, MJ. Digestion of isolated legume cells in a stomach-duodenum model: three mechanisms limit starch and protein hydrolysis. Food Funct. (2017) 8:2573–82. doi: 10.1039/C7FO00086C
7. Joye, I. Protein digestibility of cereal products. Food Secur. (2019) 8:199. doi: 10.3390/foods8060199
8. Dallas, DC, Sanctuary, MR, Qu, Y, Khajavi, SH, Van Zandt, AE, Dyandra, M, et al. Personalizing protein nourishment. Crit Rev Food Sci Nutr. (2017) 57:3313–31. doi: 10.1080/10408398.2015.1117412
9. Consultation FAOE. Dietary protein quality evaluation in human nutrition. Report of an FAQ Expert Consultation. FAO Food Nutr Pap. (2013) 92:1.
10. Mathai, JK, Liu, Y, and Stein, HH. Values for digestible indispensable amino acid scores (DIAAS) for some dairy and plant proteins may better describe protein quality than values calculated using the concept for protein digestibility-corrected amino acid scores (PDCAAS). Br J Nutr. (2017) 117:490–9. doi: 10.1017/S0007114517000125
11. Moughan, PJ. In vitro techniques for the assessment of the nutritive value of feed grains for pigs: a review. Aust J Agric Res. (1999) 50:871. doi: 10.1071/AR98172
12. Somaratne, G, Ferrua, MJ, Ye, A, Nau, F, Floury, J, Dupont, D, et al. Food material properties as determining factors in nutrient release during human gastric digestion: a review. Crit Rev Food Sci Nutr. (2020) 60:3753–69. doi: 10.1080/10408398.2019.1707770
13. Gu, J, Bk, A, Wu, H, Lu, P, Nawaz, MA, Barrow, CJ, et al. Impact of processing and storage on protein digestibility and bioavailability of legumes. Food Rev Intl. (2023) 39:4697–724. doi: 10.1080/87559129.2022.2039690
14. Butts, CA, Monro, JA, and Moughan, PJ. In vitro determination of dietary protein and amino acid digestibility for humans. Br J Nutr. (2012) 108:S282–7. doi: 10.1017/S0007114512002310
15. Corring, T. The adaptation of digestive enzymes to the diet: its physiological significance. Reprod Nutr Dev. (1980) 20:1217–35. doi: 10.1051/rnd:19800713
16. Eggum, BO, Hansen, I, and Larsen, T. Protein quality and digestible energy of selected foods determined in balance trials with rats. Plant Foods Hum Nutr. (1989) 39:13–21. doi: 10.1007/BF01092397
17. Bohn, T, Carrière, F, Day, L, Deglaire, A, Egger, L, Freitas, D, et al. Correlation between in vitro and in vivo data on food digestion. What can we predict with static in vitro digestion models? Crit Rev Food Sci Nutr. (2018) 58:2239–61. doi: 10.1080/10408398.2017.1315362
18. Santos-Hernández, M, Miralles, B, Amigo, L, and Recio, I. Intestinal signaling of proteins and digestion-derived products relevant to satiety. J Agric Food Chem. (2018) 66:10123–31. doi: 10.1021/acs.jafc.8b02355
19. Hsu, HW, Vavak, DL, Satterlee, L, and Miller, GA. A multienzyme technique for estimating protein digestibility. J Food Sci. (1977) 42:1269–73. doi: 10.1111/j.1365-2621.1977.tb14476.x
20. Moughan, PJ, Schrama, J, Skilton, GA, and Smith, WC. In-vitro determination of nitrogen digestibility and lysine availability in meat and bone meals and comparison with in-vivo ileal digestibility estimates. J Sci Food Agric. (1989) 47:281–92. doi: 10.1002/jsfa.2740470304
21. Sáez-Plaza, P, Michałowski, T, Navas, MJ, Asuero, AG, and Wybraniec, S. An overview of the Kjeldahl method of nitrogen determination. Part I. Early history, chemistry of the procedure, and titrimetric finish. Crit Rev Anal Chem. (2013) 43:178–223. doi: 10.1080/10408347.2012.751786
22. Hayes, M. Measuring protein content in food: an overview of methods. Food Secur. (2020) 9:1340. doi: 10.3390/foods9101340
23. Darragh, AJ, and Moughan, PJ. The effect of hydrolysis time on amino acid analysis. J AOAC Int. (2005) 88:888–93. doi: 10.1093/jaoac/88.3.888
24. Moughan, PJ, and Rutherfurd, SM. A new method for determining digestible reactive lysine in foods. J Agric Food Chem. (1996) 44:2202–9. doi: 10.1021/jf950032j
25. Fuller, MF, and Tomé, D. In vivo determination of amino acid bioavailability in humans and model animals. J AOAC Int. (2005) 88:923–34. doi: 10.1093/jaoac/88.3.923
26. Moughan, PJ, and Rutherfurd, SM. Available lysine in foods: a brief historical overview. J AOAC Int. (2008) 91:901–6. doi: 10.1093/jaoac/91.4.901
27. Moughan, PJ, and Wolfe, RR. Determination of dietary amino acid digestibility in humans. J Nutr. (2019) 149:2101–9. doi: 10.1093/jn/nxz211
28. Church, FC, Porter, DH, Catignani, GL, and Swaisgood, HE. An o-phthalaldehyde spectrophotometric assay for proteinases. Anal Biochem. (1985) 146:343–8. doi: 10.1016/0003-2697(85)90549-4
29. Spellman, D, McEvoy, E, O’Cuinn, G, and FitzGerald, RJ. Proteinase and exopeptidase hydrolysis of whey protein: comparison of the TNBS, OPA and pH stat methods for quantification of degree of hydrolysis. Int Dairy J. (2003) 13:447–53. doi: 10.1016/S0958-6946(03)00053-0
30. Herbert, P, Santos, L, and Alves, A. Simultaneous quantification of primary, secondary amino acids, and biogenic amines in musts and wines using OPA/3-MPA/FMOC-CI fluorescent derivatives. J Food Sci. (2001) 66:1319–25. doi: 10.1111/j.1365-2621.2001.tb15208.x
31. Savoie, L, and Charbonneau, R. Specific role of endopeptidases in modulating the nature of protein digestion products. Plant Foods Hum Nutr. (1990) 40:233–42. doi: 10.1007/BF02193846
32. Minekus, M, Alminger, M, Alvito, P, Ballance, S, Bohn, T, Bourlieu, C, et al. A standardised static in vitro digestion method suitable for food–an international consensus. Food Funct. (2014) 5:1113–24. doi: 10.1039/C3FO60702J
33. Brodkorb, A, Egger, L, Alminger, M, Alvito, P, Assunção, R, Ballance, S, et al. INFOGEST static in vitro simulation of gastrointestinal food digestion. Nat Protoc. (2019) 14:991–1014. doi: 10.1038/s41596-018-0119-1
34. Picariello, G, Miralles, B, Mamone, G, Sánchez-Rivera, L, Recio, I, Addeo, F, et al. Role of intestinal brush border peptidases in the simulated digestion of milk proteins. Mol Nutr Food Res. (2015) 59:948–56. doi: 10.1002/mnfr.201400856
35. Carriere, F, Barrowman, JA, Verger, R, and René, L. Secretion and contribution to lipolysis of gastric and pancreatic lipases during a test meal in humans. Gastroenterology. (1993) 105:876–88. doi: 10.1016/0016-5085(93)90908-U
36. Bakala-N’Goma, J-C, Williams, HD, Sassene, PJ, Kleberg, K, Calderone, M, Jannin, V, et al. Toward the establishment of standardized in vitro tests for lipid-based formulations. 5. Lipolysis of representative formulations by gastric lipase. Pharm Res. (2015) 32:1279–87. doi: 10.1007/s11095-014-1532-y
37. Capolino, P, Guérin, C, Paume, J, Giallo, J, Ballester, J-M, Cavalier, J-F, et al. In vitro gastrointestinal lipolysis: replacement of human digestive lipases by a combination of rabbit gastric and porcine pancreatic extracts. Food Dig. (2011) 2:43–51. doi: 10.1007/s13228-011-0014-5
38. Gass, J, Vora, H, Hofmann, AF, Gray, GM, and Khosla, C. Enhancement of dietary protein digestion by conjugated bile acids. Gastroenterology. (2007) 133:16–23. doi: 10.1053/j.gastro.2007.04.008
39. Stewart, RJC, Morton, H, Coad, J, and Pedley, KC. In vitro digestion for assessing micronutrient bioavailability: the importance of digestion duration. Int J Food Sci Nutr. (2019) 70:71–7. doi: 10.1080/09637486.2018.1481200
40. Orlien, V, Aalaei, K, Poojary, MM, Nielsen, DS, Ahrné, L, and Carrascal, JR. Effect of processing on in vitro digestibility (IVPD) of food proteins. Crit Rev Food Sci Nutr. (2023) 63:2790–839. doi: 10.1080/10408398.2021.1980763
41. Pusztai, A. Biological effects of dietary lectins. Recent advances of research in antinutritional factors in legume seeds, Wageningen, The Netherlands. (1989).
42. Cirkovic Velickovic, TD, and Stanic-Vucinic, DJ. The role of dietary phenolic compounds in protein digestion and processing technologies to improve their antinutritive properties. Compr Rev Food Sci Food Saf. (2018) 17:82–103. doi: 10.1111/1541-4337.12320
43. Boisen, S, and Eggum, BO. Critical evaluation of in vitro methods for estimating digestibility in simple-stomach animals. Nutr Res Rev. (1991) 4:141–62. doi: 10.1079/NRR19910012
44. Boisen, S, and Fernández, JA. In vitro digestion as a basis for the prediction of energy and protein value in pig feeds. Annual Meeting of European Association of Animal Production (1991) 42:
45. Boisen, S, and Fernández, JA. In vitro digestibility of energy and amino acids in pig feeds. Dig Physiol Pigs. (1991) 54:231–6.
46. Pedersen, B, and Eggum, BO. Prediction of protein digestibility by in vitro procedures based on two multi-enzyme systems. Z Tierphysiol Tierernahr Futtermittelkd. (1981) 45:190–200. doi: 10.1111/j.1439-0396.1981.tb01319.x
47. Wolzak, A, Bressani, R, and Gomez, BR. A comparison of in vivo and in vitro estimates of protein digestibility of native and thermally processed vegetable proteins. Plant Foods Hum Nutr. (1981) 31:31–43. doi: 10.1007/BF01093886
48. Kim, YA, and Barbeau, WE. Evaluation of SDS-PAGE method for estimating protein digestibility. J Food Sci. (1991) 56:1082–6. doi: 10.1111/j.1365-2621.1991.tb14647.x
49. Pedersen, B, and Eggum, BO. Prediction of protein digestibility by an in vitro enzymatic pH-stat procedure. Z Tierphysiol Tierernahr Futtermittelkd. (1983) 49:265–77. doi: 10.1111/j.1439-0396.1983.tb00808.x
50. Serna-Saldivar, SO. Nutritional evaluation of sorghum and maize tortillas. United States: Texas A&M University (1984).
51. Dimes, LE, and Haard, NF. Estimation of protein digestibility—I. Development of an in vitro method for estimating protein digestibility in salmonids (Salmo gairdneri). Comp Biochem Physiol A Physiol. (1994) 108:349–62. doi: 10.1016/0300-9629(94)90106-6
52. Ezquerra, JM, García-Carreño, FL, Civera, R, and Haard, NF. pH-stat method to predict protein digestibility in white shrimp (Penaeus vannamei). Aquaculture. (1997) 157:251–62. doi: 10.1016/S0044-8486(97)00058-6
53. Linder, M, Rozan, P, El Kossori, RL, Fanni, J, Villaume, C, Mejean, L, et al. Nutritional value of veal bone hydrolysate. J Food Sci. (1997) 62:183–9. doi: 10.1111/j.1365-2621.1997.tb04396.x
54. Salazar-Villanea, S, Hulshof, TG, Van der Poel, AFB, Bruininx, E, and Bikker, P. Predicting the standardized ileal protein digestibility of processed soybean meal and rapeseed meal in growing pigs using two in vitro methods. J Anim Sci. (2016) 94:202–6. doi: 10.2527/jas.2015-9743
55. Clunies, M, and Leeson, S. In vitro estimation of dry matter and crude protein digestibility. Poult Sci. (1984) 63:89–96. doi: 10.3382/ps.0630089
56. Babinszky, L, Van Der Meer, JM, Boer, H, and Den Hartog, LA. An in-vitro method for prediction of the digestible crude protein content in pig feeds. J Sci Food Agric. (1990) 50:173–8. doi: 10.1002/jsfa.2740500205
57. Boisen, S, and Fernández, JA. Prediction of the apparent ileal digestibility of protein and amino acids in feedstuffs and feed mixtures for pigs by in vitro analyses. Anim Feed Sci Technol. (1995) 51:29–43. doi: 10.1016/0377-8401(94)00686-4
58. Hervera, M, Baucells, MD, González, G, Pérez, E, and Castrillo, C. Prediction of digestible protein content of dry extruded dog foods: comparison of methods. J Anim Physiol Anim Nutr (Berl). (2009) 93:366–72. doi: 10.1111/j.1439-0396.2008.00870.x
59. Galibois, I, and Savoie, L. Relationship between amino acid intestinal effluent in rat and in vitro protein digestion products. Nutr Res. (1987) 7:65–79. doi: 10.1016/S0271-5317(87)80192-6
60. Rozan, P, Lamghari, R, Linder, M, Villaume, C, Fanni, J, Parmentier, M, et al. In vivo and in vitro digestibility of soybean, lupine, and rapeseed meal proteins after various technological processes. J Agric Food Chem. (1997) 45:1762–9. doi: 10.1021/jf960723v
61. Huang, R-L, Tan, Z-L, Xing, T-X, Pan, Y-F, and Li, T-J. An in vitro method for the estimation of ileal crude protein and amino acids digestibility using the dialysis tubing for pig feedstuffs. Anim Feed Sci Technol. (2000) 88:79–89. doi: 10.1016/S0377-8401(00)00209-1
62. Jezierny, D, Mosenthin, R, Sauer, N, and Eklund, M. In vitro prediction of standardised ileal crude protein and amino acid digestibilities in grain legumes for growing pigs. Animal. (2010) 4:1987–96. doi: 10.1017/S1751731110001114
63. Meunier, JP, Manzanilla, EG, Anguita, M, Denis, S, Pérez, JF, Gasa, J, et al. Evaluation of a dynamic in vitro model to simulate the porcine ileal digestion of diets differing in carbohydrate composition1. J Anim Sci. (2008) 86:1156–63. doi: 10.2527/jas.2007-0145
64. Le Roux, L, Ménard, O, Chacon, R, Dupont, D, Jeantet, R, Deglaire, A, et al. Are Faba Bean and Pea Proteins potential whey protein substitutes in infant formulas? An in vitro dynamic digestion approach. Foods. (2020) 9:362. doi: 10.3390/foods9030362
65. Satterlee, LD, Marshall, HF, and Tennyson, JM. Measuring protein quality. J Am Oil Chem Soc. (1979) 56:103. doi: 10.1007/BF02671431
66. Mcdonough, FE, Sarwar, G, Steinke, FH, Slump, P, Garcia, S, and Boisen, S. In vitro assay for protein digestibility: Interlaboratory study. J Assoc Off Anal Chem. (1990) 73:622–5. doi: 10.1093/jaoac/73.4.622
67. Furuya, S, Sakamoto, K, and Takahashi, S. A new in vitro method for the estimation of digestibility using the intestinal fluid of the pig. Br J Nutr. (1979) 41:511–20. doi: 10.1079/BJN19790066
68. Büchmann, NB. Variation in in vitro digestibility of barley protein. J Sci Food Agric. (1979) 30:590–6. doi: 10.1002/jsfa.2740300607
69. Wada, Y, and Lönnerdal, B. Effects of different industrial heating processes of Milk on site-specific protein modifications and their relationship to in vitro and in vivo digestibility. J Agric Food Chem. (2014) 62:4175–85. doi: 10.1021/jf501617s
70. Son, J, and Kim, BG. Nutrient digestibility of soybean meal products based on in vitro procedures for pigs. Agriculture. (2023) 13:1631. doi: 10.3390/agriculture13081631
71. Hernández-Olivas, E, Muñoz-Pina, S, García-Hernández, J, Andrés, A, and Heredia, A. Impact of common gastrointestinal disorders in elderly on in vitro meat protein digestibility and related properties. Food Biosci. (2022) 46:101560. doi: 10.1016/j.fbio.2022.101560
72. Potin, F, Goure, E, Lubbers, S, Husson, F, and Saurel, R. Functional properties of hemp protein concentrate obtained by alkaline extraction and successive ultrafiltration and spray-drying. Int J Food Sci Technol. (2022) 57:436–46. doi: 10.1111/ijfs.15425
73. Gauthier, SF, Vachon, C, and Savoie, L. Enzymatic conditions of an in vitro method to study protein digestion. J Food Sci. (1986) 51:960–4. doi: 10.1111/j.1365-2621.1986.tb11208.x
74. Brulé, D, and Savoie, L. In vitro digestibility of protein and amino acids in protein mixtures. J Sci Food Agric. (1988) 43:361–72. doi: 10.1002/jsfa.2740430409
75. Savoie, L, Charbonneau, R, and Parent, G. In vitro amino acid digestibility of food proteins as measured by the digestion cell technique. Plant Foods Hum Nutr. (1989) 39:93–107. doi: 10.1007/BF01092406
76. Galland-Irmouli, A-V, Fleurence, J, Lamghari, R, Luçon, M, Rouxel, C, Barbaroux, O, et al. Nutritional value of proteins from edible seaweed Palmaria palmata (Dulse). J Nutr Biochem. (1999) 10:353–9. doi: 10.1016/s0955-2863(99)00014-5
77. Marrion, O, Fleurence, J, Schwertz, A, Guéant, J-L, Mamelouk, L, Ksouri, J, et al. Evaluation of protein in vitro digestibility of Palmaria palmata and Gracilaria verrucosa. J Appl Phycol. (2005) 17:99–102. doi: 10.1007/s10811-005-5154-y
78. Agudelo, RA, Gauthier, SF, Pouliot, Y, Marin, J, and Savoie, L. Kinetics of peptide fraction release during in vitro digestion of casein. J Sci Food Agric. (2004) 84:325–32. doi: 10.1002/jsfa.1662
79. Savoie, L, Agudelo, R, Gauthier, S, Marin, J, and Pouliot, Y. In vitro determination of the release kinetics of peptides and free amino acids during the digestion of food proteins. J AOAC Int. (2005) 88:935–48. doi: 10.1093/jaoac/88.3.935
80. Marina, C, Luisa, M, and Emilia, C. Factors affecting cystine reactivity in proteolytic digests of Phaseolus vulgaris. J Agric Food Chem. (1992) 40:169–74. doi: 10.1021/jf00014a001
81. Gatellier, P, and Santé-Lhoutellier, V. Digestion study of proteins from cooked meat using an enzymatic microreactor. Meat Sci. (2009) 81:405–9. doi: 10.1016/j.meatsci.2008.09.002
82. Hellwig, M, and Henle, T. Release of pyrraline in absorbable peptides during simulated digestion of casein glycated by 3-deoxyglucosone. Eur Food Res Technol. (2013) 237:47–55. doi: 10.1007/s00217-013-2027-5
83. Schaafsma, G. The protein digestibility-corrected amino acid score (PDCAAS)—a concept for describing protein quality in foods and food ingredients: a critical review. J AOAC Int. (2005) 88:988–94. doi: 10.1093/jaoac/88.3.988
84. Havenaar, R, Maathuis, A, de Jong, A, Mancinelli, D, Berger, A, and Bellmann, S. Herring roe protein has a high digestible indispensable amino acid score (DIAAS) using a dynamic in vitro gastrointestinal model. Nutr Res. (2016) 36:798–807. doi: 10.1016/j.nutres.2016.05.004
85. Jaeger, A, Ahern, N, Sahin, AW, Nyhan, L, Mes, JJ, van der Aa, C, et al. Dynamic in-vitro system indicates good digestibility characteristics for novel upcycled plant protein; correlation to techno-functional properties. Innovative Food Sci Emerg Technol. (2024) 92:103571. doi: 10.1016/j.ifset.2024.103571
86. Ménard, O, Cattenoz, T, Guillemin, H, Souchon, I, Deglaire, A, Dupont, D, et al. Validation of a new in vitro dynamic system to simulate infant digestion. Food Chem. (2014) 145:1039–45. doi: 10.1016/j.foodchem.2013.09.036
87. Egger, L, Ménard, O, Delgado-Andrade, C, Alvito, P, Assunção, R, Balance, S, et al. The harmonized INFOGEST in vitro digestion method: from knowledge to action. Food Res Int. (2016) 88:217–25. doi: 10.1016/j.foodres.2015.12.006
88. Egger, L, Schlegel, P, Baumann, C, Stoffers, H, Guggisberg, D, Brügger, C, et al. Physiological comparability of the harmonized INFOGEST in vitro digestion method to in vivo pig digestion. Food Res Int. (2017) 102:567–74. doi: 10.1016/j.foodres.2017.09.047
89. Grundy, MML, Abrahamse, E, Almgren, A, Alminger, M, Andres, A, Ariëns, RMC, et al. INFOGEST inter-laboratory recommendations for assaying gastric and pancreatic lipases activities prior to in vitro digestion studies. J Funct Foods. (2021) 82:104497. doi: 10.1016/j.jff.2021.104497
90. Ariëns, RMC, Bastiaan-Net, S, van de Berg-Somhorst, DBPM, El Bachrioui, K, Boudewijn, A, van den Dool, RTM, et al. Comparing nutritional and digestibility aspects of sustainable proteins using the INFOGEST digestion protocol. J Funct Foods. (2021) 87:104748. doi: 10.1016/j.jff.2021.104748
91. Rieder, A, Afseth, NK, Böcker, U, Knutsen, SH, Kirkhus, B, Mæhre, HK, et al. Improved estimation of in vitro protein digestibility of different foods using size exclusion chromatography. Food Chem. (2021) 358:129830. doi: 10.1016/j.foodchem.2021.129830
92. Sousa, R, Recio, I, Heimo, D, Dubois, S, Moughan, PJ, Hodgkinson, SM, et al. In vitro digestibility of dietary proteins and in vitro DIAAS analytical workflow based on the INFOGEST static protocol and its validation with in vivo data. Food Chem. (2023) 404:134720. doi: 10.1016/j.foodchem.2022.134720
93. Komatsu, Y, Tsuda, M, Wada, Y, Shibasaki, T, Nakamura, H, and Miyaji, K. Nutritional evaluation of Milk-, plant-, and insect-based protein materials by protein digestibility using the INFOGEST digestion method. J Agric Food Chem. (2023) 71:2503–13. doi: 10.1021/acs.jafc.2c07273
94. Ménard, O, Chauvet, L, Henry, G, Dupont, D, Gaudichon, C, Calvez, J, et al. The use of 15N-labelled protein to account for the endogenous nitrogen contribution to in vitro protein digestibility measurement. Food Res Int. (2023) 173:113242. doi: 10.1016/j.foodres.2023.113242
95. Martineau-Côté, D, Achouri, A, Pitre, M, Karboune, S, and L’Hocine, L. Improved in vitro gastrointestinal digestion protocol mimicking brush border digestion for the determination of the digestible indispensable amino acid score (DIAAS) of different food matrices. Food Res Int. (2024) 178:113932. doi: 10.1016/j.foodres.2024.113932
96. Martin Bland, J, and DouglasG, A. Statistical methods for assessing agreement between two methods of clinical measurement. Lancet. (1986) 327:307–10. doi: 10.1016/S0140-6736(86)90837-8
Keywords: in vitro protein digestibility, protein nutritional quality, in vitro DIAAS, simulated gastrointestinal digestion, INFOGEST
Citation: Santos-Sánchez G, Miralles B, Brodkorb A, Dupont D, Egger L and Recio I (2024) Current advances for in vitro protein digestibility. Front. Nutr. 11:1404538. doi: 10.3389/fnut.2024.1404538
Edited by:
Sylvia Chungchunlam, Massey University, New ZealandReviewed by:
Mutamed Ayyash, United Arab Emirates University, United Arab EmiratesVibeke Orlien, University of Copenhagen, Denmark
Copyright © 2024 Santos-Sánchez, Miralles, Brodkorb, Dupont, Egger and Recio. This is an open-access article distributed under the terms of the Creative Commons Attribution License (CC BY). The use, distribution or reproduction in other forums is permitted, provided the original author(s) and the copyright owner(s) are credited and that the original publication in this journal is cited, in accordance with accepted academic practice. No use, distribution or reproduction is permitted which does not comply with these terms.
*Correspondence: Isidra Recio, i.recio@csic.es