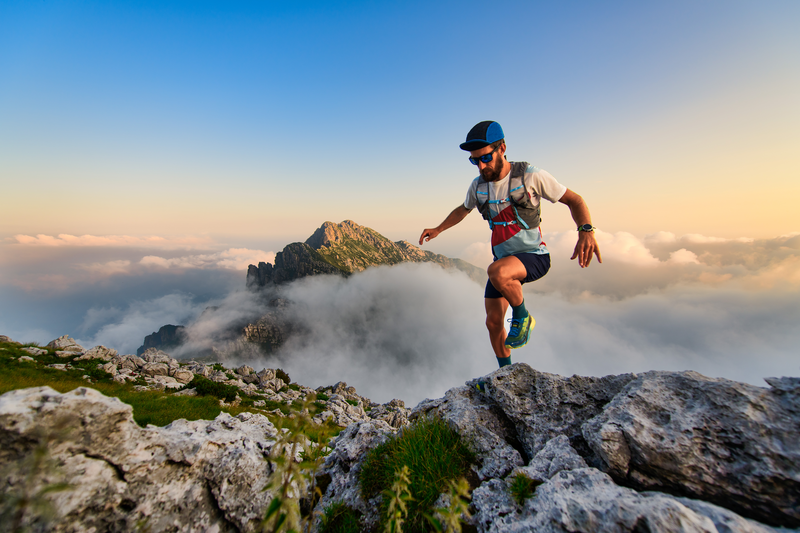
94% of researchers rate our articles as excellent or good
Learn more about the work of our research integrity team to safeguard the quality of each article we publish.
Find out more
ORIGINAL RESEARCH article
Front. Nutr. , 04 June 2024
Sec. Nutrition and Food Science Technology
Volume 11 - 2024 | https://doi.org/10.3389/fnut.2024.1403293
This article is part of the Research Topic Novel Technologies for Enrichment, Extraction, and Determination of Phenolic Compounds in Foods - Volume 2 View all 4 articles
Phenolic acids are secondary metabolites in higher plants, with antioxidant, anticancer, and anti-aging effects on the human body. Therefore, foods rich in phenolic acids are popular. Methyl jasmonate (MeJA) promoted phenolic acids accumulation but also inhibited sprout growth. Melatonin (MT) was a new type of plant hormone that not only alleviated plants’ abiotic stress, but also promoted the synthesis of plant-stimulating metabolism. This study aimed to elucidate the mechanism of exogenous MT on the growth and development, and phenolic acids metabolism of barley sprouts under MeJA treatment. The results showed that MT increased the phenolic acids content in sprouts by increasing the activities of phenylalanine ammonia-lyase and cinnamic acid 4-hydroxylase, and up-regulating the gene expression of phenylalanine ammonia-lyase, cinnamic acid 4-hydroxylase, 4-coumarate: coenzyme a ligase, and ferulic acid-5-hydroxylase. MT attenuated the growth inhibition of barley sprouts under MeJA stress by increasing the activities of regulated antioxidant enzymes and the expression of their corresponding genes. Furthermore, MT increased the NO content and induced Ca2+ burst in barley sprouts under MeJA stress. These events were inhibited by DL-4-Chlorophenylalanine. These results suggested that MT ameliorated growth inhibition and promoted the biosynthesis of phenolic acids in barley sprouts under MeJA stress.
Phenolic acids are secondary metabolites found in higher plants, primarily synthesized through the phenylpropane metabolic pathway (1). Studies indicated that phenolic acids play a significant role in plant defense systems, by limiting the generation of free radicals, reducing levels of reactive oxygen species, and enhancing antioxidant capacity (2, 3). In addition, in the human body, phenolic acids also exhibit strong antioxidant properties (4–6), effectively eliminating free radicals generated, delaying cell aging, inhibiting tumor growth, and promoting metabolism. However, phenolic acids are unable to be produced within the human body and must be obtained through external sources (7). Therefore, increasing the content of phenolic acids in plant-based foods has garnered widespread attention.
In the past few years, extensive studies have been conducted on the metabolism and accumulation of phenolic acids (6, 8). Research has found that germination under abiotic stress conditions can significantly increase the content of phenolic acids in barley (9). Methyl jasmonate (MeJA), as a plant hormone, plays various roles in plant growth metabolism, including protein synthesis, enzyme metabolism, and photosynthesis (10–12). Studies have shown that MeJA treatment can significantly increase the content of secondary metabolites in plants, especially phenolic compounds. Research by Rahmati et al. (13) found that treatment with 25 μM MeJA significantly increased the total phenolics content in Carum carvi L. Palai et al. (14) discovered that treatment with 10 μM MeJA could significantly increase the content of flavonoid compounds in grapes. In the preliminary experiments, it was found that 100 μM MeJA significantly increased phenolic acids content in barley sprouts. However, it was also discovered that MeJA treatment could inhibit sprouts’ growth and development. Therefore, it is necessary to seek a method to alleviate growth inhibition and further promote the synthesis of phenolic acids on barley sprouts under MeJA stress.
Melatonin (MT) was initially discovered in plants in 1995 (15). MT in plant cells has various physiological effects (16, 17), including regulating plant growth, seed germination, and enhancing the photosynthetic rate of plants. Furthermore, research has indicated MT could alleviate plant stress by improving oxidative-reductive homeostasis (18), cell membrane stability (19), and photosynthetic efficiency (18). The research also found that exogenous MT serves as a stimulant for secondary metabolites in many plants. For example, it increased the synthesis of phenolic acids in tomatoes (20), citrus fruits (21), and cowpeas (22). Recent studies (23) have shown that the synergistic effect of Pseudomonas fluorescens and MT improved salt tolerance, and further increased the production of secondary metabolites in mustard sprouts under biotic stress. Similarly, in our previous studies (24, 25), it was demonstrated that MT could improve the salt tolerance of soybeans and barley sprouts, remove growth inhibition, and increase the content of isoflavones in soybean sprouts and phenolic acids in barley sprouts. These studies suggested that MT may be a promising plant hormone that can enhance biosynthesis, and maintain biomass under stress. However, current research has not yet found how MT alleviates the stress of MeJA on barley sprouts and further promotes phenolic acids synthesis.
In this study, the mechanism of exogenous MT on the development and total phenolic acids metabolism of barley sprouts under MeJA stress from physiological metabolism and gene transcription perspectives were investigated. The study objective was to elucidate the role of MT in enriching total phenolic acids in barley sprouts and unravel the underlying multifaceted mechanisms. This study served to lay the groundwork for further exploration into the synthesis and regulation mechanisms of phenolic acids in barley sprouts, offered resource reserve, and a theoretical basis for targeted plant-based foods.
Barley seeds (Su Hullless 2), supplied by Jiangsu Academy of Agricultural Sciences (Nanjing, China), were stored at −20°C. MT, MeJA, and DL-4-Chlorophenylalanine (MT inhibitor, PCPA) were bought from Sigma (Shanghai, China), and the remaining reagents were purchased from Macklin (Shanghai, China).
Barley seeds were selected, sterilized in sodium hypochlorite solution, and subsequently rinsed with deionized water to pH = 7.0. Soaked for 6 h at 25°C in deionized water. The soaked barley was placed in individual germination trays (for a single treatment group) and germinated for 6 days at 25°C in the dark. Each treatment was sprayed with 40 mL of a different solution every 12 h during germination. (1) M: sprayed with 100 μM MeJA; (2) MM: sprayed with a mixture of 100 μM MeJA and 1 mM MT (v:v = 1:1); (3) MP: sprayed with a mixture of 100 μM MeJA and 100 μM PCPA (v:v = 1:1); (4) MMP: sprayed with a mixture of 100 μM MeJA, 1 mM MT and 100 μM PCPA (v:v:v = 1:1:1). On the 4th and 6th day, the barley sprouts were collected at random, stored in a − 20°C refrigerator, and kept for measurement of relevant indicators.
Determination of sprout length and fresh weight (FW) according to Yin et al. (24) method.
The content of free amino acids in barley sprouts was determined by the method of Ma et al. (26). In detail, 1.0 g of barley sprouts were ground with 10% acetic acid to obtain a uniform slurry, then centrifuged to collect the supernatant. The supernatant was mixed with ninhydrin and vitamin C, heated for 15 min, and measured at the wavelength of 570 nm.
Determination of soluble proteins with the Assay Kit (A045-2-1, Nanjing Jiancheng Bioengineering Institute, China).
According to the method of Zhuang et al. (27) determined the malondialdehyde (MDA) and superoxide anion ( ) content. Barley sprouts were ground with trichloroacetic acid. After centrifugation, the supernatant was boiled with thiobarbituric acid for 20 min. The absorbance values of the supernatant were determined at 450 nm, 532 nm, and 600 nm, respectively.
The production of was determined by monitoring hydroxylamine nitrate in the presence of generators. Barley sprouts were ground to a homogenate with 65 mM phosphate buffer and centrifuged at 8,000 × g for 10 min. The 1 mL supernatant was mixed with 0.9 mL of 65 mM phosphate buffer (pH = 7.8) and 0.1 mL of 10 mM hydroxylammonium chloride. After incubation at 25°C for 20 min, 17 mM sulfanilic acid and 7 mM α-naphthylamine were added to the mixture, and the absorbance was measured at 530 nm after holding at 25°C for 20 min. The standard curve of sodium nitrate was used to calculate the rate of production.
Hydrogen peroxide (H2O2) content was determined using Assay Kit (A064-1-1, Nanjing Jiancheng Bioengineering Institute, China).
Total phenolics and total phenolic acids contents were determined with reference to Ma et al. (26). Specifically, barley seedling was homogenized with 50% methanol to obtain a homogenate. The homogenate was centrifuged to obtain the supernatant. The supernatant was thoroughly mixed with Folin-phenolic and sodium carbonate, then incubated at 25°C for 2 hours in the dark. The absorbance value was measured at 765 nm, and the total phenolics content was calculated based on the gallic acid standard curve.
Furthermore, the supernatant was mixed with 50% methanol, 0.3% sodium dodecyl sulfate, and 0.6% ferric chloride, then mixed thoroughly and placed in the dark for 5 min. The absorbance at 760 nm was read to determine the total phenolic acids content.
Nitric oxide (NO) content was determined using Assay Kit (XZK-15995, Zexuankang Bioengineering Institute, China).
The intracellular free calcium, H2O2, and fuorescence staining according to the method of Yin et al. (24). Germinated barley seedlings at 4 days under different treatments were collected. The root tips were cut to a length of 3 mm. The root tips were incubated in Hanks balanced salt buffer solution (HBSS) containing fluorescent dyes (Fluo-4 AM, dihydroethidium, and 2′,7′-dichlorofluorescein diacetate), and stained at 4°C for 2 h. After staining, the root tips were washed thrice with HBSS and then incubated in the dark at 25°C for 2 h. Subsequently, images were captured using an LSM 880 NLO confocal laser scanning microscope (Leica Excitation Technology Co., Ltd., Germany), with an excitation wavelength of 488 nm and an emission wavelength of 520 nm.
The activities determination of peroxidase (POD) and superoxide dismutase (SOD) were conducted following the method of Wang et al. (28). Barley sprouts were ground in an ice bath containing sodium phosphoric acid buffer (pH = 7.0, 50 mM). The suspensions were centrifuged, and the supernatant was used to measure the enzyme activity. A change of 0.01 at OD470 nm per minute was expressed as one unit of POD activity. A change of 0.01 at OD560 nm per minute was expressed as one unit of SOD activity.
Following the methods described in references (29), measure the 2,2′-azino-bis (3-ethylbenzothiazoline-6-sulfonic acid) (ABTS) and 2,2-diphenyl-1-picrylhydrazyl (DPPH) free radical scavenging rates in the barley. Absorbance at 734 nm was read on a spectrophotometer to determine ABTS free radical scavenging activity. DPPH scavenging was calculated by measuring absorbance at 515 nm using vitamin C as a positive control.
The activities of Phenylalanine ammonia-lyase (PAL) and cinnamic acid 4-hydroxylase (C4H) were determined by the method of Yin et al. (24). Barley sprouts and phosphate buffer were homogenized at low temperatures. The supernatant was centrifuged, and the absorbance of the supernatant and reaction mixture was measured at wavelengths 290 nm and 345 nm, respectively. A change of 0.01 per minute is one unit of enzyme activity.
Barley sprouts total RNA was isolated employing the Takara MiniBEST Plant RNA Extraction Kit (9,769, Takara, China), followed by cDNA synthesis using the PrimeScriptH RT Kit (RR092S, Takara, China). The study utilized sequence-specific primers in Table 1 for quantitative real-time PCR (qRT-PCR) analysis. Each treatment was assessed three times by qRT-PCR using the ABI 7500 Sequence Detection System (Foster City, CA, USA) and SYBR® Pre-mix Ex TaqTM (RR820A, Takara, China). Relative gene expression levels were calculated using the 2-ΔΔCt method.
The outcomes were presented as the mean ± standard deviation (SD) of duplicated determinations. Statistical analysis was performed using SPSS 18.0 software. Duncan’s multiple range test, with a significance level of 0.05 (p < 0.05), was employed to evaluate significant variances.
Compared with MeJA treatment, exogenous MT significantly increased (Figures 1A,B, p < 0.05) the length of the sprout, and the length increased by 41.9 and 27.6%, respectively. MT notably increased the fresh weight of sprouts (Figure 1C, p < 0.05). However, the PCPA (MT inhibitor) significantly reduced the fresh weight and sprout length of four-day-old sprouts, whether under MeJA treatment or MeJA plus MT treatment. Furthermore, compared with MeJA treatment, the addition of exogenous MT resulted in a substantial reduction in the content of soluble proteins and free amino acids present in the sprouts (p < 0.05, Figures 1D,E), with decreased by of 8.0 and 20.4%, respectively, at 4-day-old. Additionally, the addition of PCPA significantly increased the content of soluble proteins in sprouts under MeJA and MeJA plus MT treatments.
Figure 1. The effects of MT on growth performance(A), sprout length (B), fresh weight (C), free amino acid content (D), and soluble protein content (E) in barley sprouts under MeJA stress. Error bars indicate the standard deviations of each data point (n = 3). Lowercase letters indicate significant (p < 0.05) differences between treatments for the same germination time. M: 100 μM MeJA, MM: 100 μM MeJA plus 0.1 mM MT, MP: 100 μM MeJA plus 100 μM PCPA, MMP: 100 μM MeJA plus 0.1 mM MT plus 100 μM PCPA. The same as below.
Compared with MeJA treatment, the addition of MT significantly increased the total phenolics, total phenolic acids, and NO content in sprouts (Figure 2, p < 0.05). The total phenolics and NO content reached their maximum values on the 4th day (Figures 2A,B), which were 9.86 mg/g FW and 7.91 nmoL/g FW, respectively. The total phenolic acids content reached maximum value on the 6th day, which was 1054.08 μg/g FW. Additionally, PCPA significantly reduced the total phenolics, total phenolic acids, and NO content on the 6th day, regardless of MeJA treatment or MeJA plus MT treatment. These results suggested that NO, as a signaling molecule, mediated the promotion of phenolic acids under MT plus MeJA treatment.
Figure 2. The effects of MT on total phenolics content (A), total phenolic acids content (B), and NO content (C) in barley sprouts under MeJA stress.
The exogenous MT significantly reduced membrane damage and reactive oxygen species (ROS) content in barley sprouts under MeJA treatment (Figure 3, p < 0.05). Specifically, MT decreased the H2O2, and content in 4-day-old barley sprouts by 16.1 and 5.7%, respectively, and also significantly reduced (Figures 3B,C, p < 0.05) the MDA and H2O2 content in 6-day-old sprouts by 13.0 and 5.5% (Figures 3A,B, p < 0.05), respectively, under MeJA treatment. Furthermore, the addition of PCPA significantly increased the MDA and H2O2 content in 6-day-old sprouts under MeJA combined with MT. These results indicated that MT can serve as an antioxidant in barley sprouts under MeJA stress.
Figure 3. The effects of exogenous MT on MDA content (A), H2O2 content (B), and content (C) in barley sprout under MeJA stress.
The root tips of all experimental samples were subjected to staining with fluorescence for free calcium, H2O2, and . The MT plus MeJA treatment exhibited the highest intracellular free calcium (green) fluorescence intensity, compared to the other treatments. The application of MT reduced the fluorescence intensity of H2O2 (red) and (blue) at the root tips compared to MeJA stress. These findings suggested that the accumulation of ROS was alleviated under the MT plus MeJA treatment.
The addition of exogenous MT significantly increased (Figures 4A,B, p < 0.05) the activities of POD and SOD in sprouts under MeJA treatment. The activities of POD and SOD in 6-day-old sprouts reached maximum values, at 1782.5 U/g and 40.2 U/g, respectively. On the 4th day, compared to MeJA treatment, the addition of MT significantly up-regulated (Figures 4C,D, p < 0.05) the expression levels of HvPOD and HvSOD, increased by 44.74- and 4.42-time, respectively. PCPA significantly reduced (Figures 4A,B, p < 0.05) the activities of POD and SOD in four-day-old sprouts under MM treatment, as well as the expression of HvPOD and HvSOD levels were down-regulated. Furthermore, MT not only significantly increased the clearance rates of DPPH and ABTS (Figures 4E,F, p < 0.05) under MeJA treatment, but also increased the clearance rates of DPPH and ABTS in sprouts under MeJA plus PCPA treatment. These results indicated that exogenous MT increased the clearance rate of free radicals by enhancing the antioxidant system.
Figure 4. Effects of MT on the activities of POD (A) and SOD (B), and the gene expression levels of HvPOD (C) and HvSOD (D) in barley sprouts under MeJA stress. The clearance rate ABTS (E) and DPPH (F) of barley sprouts were measured.
MT significantly increased barley sprouts’ PAL and C4H activities under MeJA treatment (Figures 5A,B, p < 0.05). Specifically, on the 4th day, PAL and C4H activities, under MT plus MeJA treatment, reached maximum values, at 949.35 U/g and 137.9 U/g, respectively, which were 1.3- and 1.2-fold higher than the MeJA treatment. The addition of PCPA significantly reduced the PAL and C4H activities in four-day-old sprouts under MM treatment and the C4H activity in six-day-old sprouts. Compared to MeJA treatment, the MT significantly up-regulated the gene expression levels of HvPAL, HvC4H, 4-coumarate: coenzyme a ligase (Hv4CL), and ferulic acid-5-hydroxylase (HvF5H) (Figures 5C–E, G, p < 0.05). Specifically, at the 4th of sprouts under MM treatment, the expression levels were 18.2-, 3.6-, 26.2-, and 12.7-fold higher than the MeJA treatment. At 6th of sprouts under MM treatment, the expression levels were 2.7-, 4.7-, 1.1-, and 4.4-fold higher than MeJA treatment. Similarly, the exogenous MT significantly increased the expression levels of HvC4H and caffeic acid O-methyltransferase (HvCOMT) in sprouts under MeJA combined with PCPA treatment (Figures 5D,H, p < 0.05). These results suggested that exogenous MT promoted total phenolic acids synthesis by upregulating gene expression levels.
Figure 5. Effects of MT on the activities of PAL (A) and C4H (B), and the gene expression levels of HvPAL (C), HvC4H (D), Hv4CL (E), HvC3H (F), HvF5H (G), and HvCOMT (H) in barley sprouts under MeJA stress.
Sprouted barley, as a new form of food, possesses a nutritional value far superior to that of barley itself. In recent years, with the pursuit of healthy diets, barley has been widely used in breakfast products, salads, soups, and baked goods due to its unique nutritional value and flavor (30, 31). However, how to further improve the nutritional of barley, especially to increase the total phenolic acids content, has been the direction of researchers’ efforts. Studies have shown that germination under abiotic stress increases the content of secondary metabolites in the plant (9). In this study, it was found that MT combined with MeJA treatment significantly increased the total phenolic acids content in barley sprouts (Figure 2B). This study will increase the nutritional value and health functions of existing barley sprout-related products such as barley green juice and barley sprouts powder. Based on this study, barley sprout products with richer nutrients and better health functions can be developed to meet consumer demand for health, flavor, and nutrition.
MeJA, as a phytohormone, promotes total phenolic acids content in plants (13), but reduces sprouts length and fresh weight, induces the production of ROS. In this study, it was found that the addition of 0.1 mM exogenous MT under MeJA treatment increased the shoot length and fresh weight (Figures 1B,C), decreased MDA, H2O2, and content (Figures 3A–C). Conversely, the addition of MT inhibitor PCPA resulted in more severe oxidative damage to the sprouts, leading to a significant decrease in shoot length and fresh weight. However, the addition of MT could reverse the adverse effects caused by PCPA, and protect the integrity of the cell membrane structure. As a new type of plant hormone, MT not only has the function of alleviating damage caused by various abiotic stresses to plants (18, 19), but also can induce the production of some secondary metabolites (20, 21), including total phenols, total phenolic acids, and flavonoids. In this study, the addition of MT significantly increased the content of total phenolics and total phenolic acids (Figures 2A,B) in sprouts treated with MeJA. These results suggested that MT could act not only as an effective plant antioxidant to alleviate oxidative damage to barley sprouts caused by MeJA, but also further increase the content of phenolic acids under MeJA treatment. This research will provide a new perspective for enriching barley phenolic acids and enhancing the development of plant-based foods.
MT can uphold cellular osmotic equilibrium by suppressing the content of H2O2, and , while diminishing lipid peroxidation of the cell membrane, consequently mitigating the adverse impacts of abiotic stress on plant physiology (32). Our study demonstrated that the exogenous MT significantly reduces the accumulation of H2O2 and MDA under MeJA treatment (Figures 3A–C, p < 0.05), enhances the ability to scavenge DPPH and ABTS free radicals (Figures 4E,F, p < 0.05), as well as the fluorescence intensity of H2O2, and (Figure 6). Similar results were reported in soybean (33) and barley (24) sprouts. Under stress, plants experience an overabundance of ROS, leading to oxidative harm and the initiation of antioxidative protection mechanisms. In the realm of plant stress responses, the enzymes, POD and SOD, play essential roles. Our investigation revealed that MT effectively preserved the integrity of cell membranes by mitigating the buildup of ROS within cells, achieved through the enhancement activities of POD and SOD. Studies have shown that the decrease in H2O2, and content is positively correlated with POD, SOD, and CAT activity and gene expression level (34). In addition, some studies (35, 36) have shown that the application of MT up-regulated the gene expression levels of POD, CAT, and APX, in plants under abiotic stress. Similarly, in our study, the exogenous MT significantly up-regulated the expression levels of HvPOD and HvSOD in sprouts under MeJA treatment (Figures 4C,D). Conversely, the addition of PCPA reversed the above results. These findings suggested that MT enhanced the antioxidant properties of barley sprouts by increasing POD and SOD enzyme activities and up-regulating related gene expression.
Figure 6. The effect of MT on root tip staining of barley sprouts under MeJA stress on the 4th day. The horizontal axis is treatment, the vertical axis is intracellular free calcium (green), H2O2 (red), and (blue), and the scale length is 100 μm.
Both NO and Ca2+ are the signaling in plant cells (37, 38). NO could induce the opening of plasma membrane Ca2+ channels, increased cytoplasmic Ca2+ concentration, and the transmission of external information (39). The elevation of Ca2+ levels stimulated the production of NO, triggered programmed cell death in the infected area, and limited pathogen growth (40, 41). Additionally, the study has shown (42) that transient increases in Ca2+ concentration induced by oligosaccharides of galacturonic acid in Arabidopsis thaliana sprouts induced the expression of genes associated with phenolic compound synthesis, including AoCHS, AoGST, AoPAL, and AoPR-1. Our research has revealed that under MeJA treatment, the addition of exogenous MT significantly enhanced the Ca2+ fluorescence intensity and NO content in sprout root tips. Furthermore, the addition of exogenous MT upregulated the expression levels of HvPAL, HvC4H, and Hv4CL and increased in total phenolic content. In future studies, we will further strengthen the research on the interaction between Ca2+ and NO, providing a theoretical basis for exploring the alleviation of MeJA stress by MT and promoting phenolic acids accumulation.
In higher plants, the synthesis of phenolic acids is mainly through the phenylpropane metabolic pathway, where acids such as phenolic acids are produced under the action of enzymes like PAL and C4H (43, 44). The investigation demonstrated that upon exposure to MeJA, the application of exogenous MT notably enhanced the PAL and C4H activities (Figures 4A,B). Conversely, the introduction of PCPA effectively suppressed the PAL and C4H activities, suggested the participation of MT in modulating crucial enzyme functions in phenolic acids synthesis in barley sprouts subjected to MeJA-induced stress. Exogenous MT significantly increased the total phenolic and phenolic acids contents in barley sprouts (Figures 2A,B). PCPA inhibited the synthesis of total phenolics and phenolic acids in four-day-old sprouts, while MT treatment reversed the inhibitory effect of PCPA. This outcome is positively correlated with changes in the enzyme activities and contents of phenolic acids in barley sprouts, possibly due to the introduction of MT under MeJA stress mediating the synthesis of phenolic acids, thereby enhancing the key enzyme activity involved in soybean sprout phenolic substance synthesis and promoting phenolic substance synthesis. Conversely, PCPA treatment can inhibit MT synthesis, reducing phenolic substance content. The addition of MT after PCPA alleviates the inhibition, restoring some enzyme activity and content levels involved in phenolic substance synthesis. These results indicated that MT positively regulates phenolic acids synthesis in barley sprouts under MeJA stress. Numerous research studies (45, 46) have indicated that the upregulation expression levels of PAL, C4H, and 4CL could contributes to the elevation of phenolic levels. In this study, it was observed that the MT not only up-regulated the expression levels of HvPAL, HvC4H, and Hv4CL but also up-regulated the expression levels of HvF5H. However, this is in contrast to previous findings (24), that exogenous MT promoted phenolic acids enrichment and inhibited HvF5H expression in sprouts under NaCl-induced stress. Our analysis suggested that the underlying cause of this discrepancy may stem from variations in gene transcription triggered by differences in stress factors or the spatiotemporal dynamics of protein translation. These results revealed that MT plus MeJA had a positive impact on the functions and levels of key enzymes involved in the phenylpropane metabolic pathway in barley, thereby increasing the phenolic acids content.
The findings presented above support the speculative molecular mechanism depicted in Figure 7, illustrating how exogenous MT mitigates the stress induced by MeJA and enhances total phenolic acids biosynthesis in barley sprouts. Exogenous MT (0.1 mM) mitigated the adverse impact of MeJA stress (100 μM) on barley sprouts. This was achieved through enhancing the functionality of antioxidant enzymes, reduced the generation of ROS, and enhanced cell membrane stability. Furthermore, MT was observed to elevate the transcription of genes HvPAL, HvC4H, Hv4CL, and HvF5H involved in phenylpropane metabolism, thus promoting the accumulation of phenolic acids. This study provided a reliable material basis for the production of phenolic acid-rich barley sprouts processed products.
Figure 7. The putative molecular mechanism of MT relieving MeJA stress and stimulating total phenolic acids biosynthesis in barley sprouts.
The datasets presented in this study can be found in online repositories. The names of the repository/repositories and accession number(s) can be found in the article/Supplementary material.
XT: Writing – original draft. RZ: Data curation, Writing – review & editing. ZY: Validation, Writing – review & editing. JZ: Data curation, Writing – review & editing. WF: Supervision, Writing – review & editing. RY: Writing – review & editing. YY: Writing – review & editing.
The author(s) declare that financial support was received for the research, authorship, and/or publication of this article. This research was supported by Postgraduate Research & Practice Innovation Program of Jiangsu Province (Yangzhou University KYCX24_3872).
The authors declare that the research was conducted in the absence of any commercial or financial relationships that could be construed as a potential conflict of interest.
All claims expressed in this article are solely those of the authors and do not necessarily represent those of their affiliated organizations, or those of the publisher, the editors and the reviewers. Any product that may be evaluated in this article, or claim that may be made by its manufacturer, is not guaranteed or endorsed by the publisher.
The Supplementary material for this article can be found online at: https://www.frontiersin.org/articles/10.3389/fnut.2024.1403293/full#supplementary-material
1. Martínez-Silvestre, K, Santiz-Gómez, J, Luján-Hidalgo, M, Ruiz-Lau, N, Sánchez-Roque, Y, and Gutiérrez-Miceli, F. Effect of UV-B radiation on flavonoids and phenols accumulation in tempisque (Sideroxylon capiri Pittier) callus. Plants. (2022) 11:473. doi: 10.3390/plants11040473
2. Tian, W, Hu, R, Chen, G, Zhang, Y, Wang, W, and Li, Y. Potential bioaccessibility of phenolic acids in whole wheat products during in vitro gastrointestinal digestion and probiotic fermentation. Food Chem. (2021) 362:130135. doi: 10.1016/j.foodchem.2021.130135
3. Shahrajabian, M, Cheng, Q, and Sun, W. The effects of amino acids, phenols and protein hydrolysates as biostimulants on sustainable crop production and alleviated stress. Recent Pat Biotechnol. (2022) 16:319–28. doi: 10.2174/1872208316666220412133749
4. Huang, M, Han, Y, Li, L, Rakariyatham, K, Wu, X, Gao, Z, et al. Protective effects of non-extractable phenolics from strawberry against inflammation and colon cancer in vitro. Food Chem. (2022) 374:131759. doi: 10.1016/j.foodchem.2021.131759
5. Bento-Silva, A, Koistinen, V, Mena, P, Bronze, M, Hanhineva, K, Sahlstrøm, S, et al. Factors affecting intake, metabolism and health benefits of phenolic acids: do we understand individual variability? Eur J Nutr. (2020) 59:1275–93. doi: 10.1007/s00394-019-01987-6
6. Wani, M, Ganie, N, Wani, D, Wani, A, Dar, S, Khan, A, et al. The phenolic components extracted from mulberry fruits as bioactive compounds against cancer: a review. Phytother Res. (2023) 37:1136–52. doi: 10.1002/ptr.7713
7. Tyagi, A, Shabbir, U, Chen, X, Chelliah, R, Elahi, F, Ham, H, et al. Phytochemical profiling and cellular antioxidant efficacy of different rice varieties in colorectal adenocarcinoma cells exposed to oxidative stress. PLoS One. (2022) 17:e0269403. doi: 10.1371/journal.pone.0269403
8. Wang, L, Li, X, Gao, F, Liu, Y, Lang, S, and Wang, C. Effects of pretreatment with a combination of ultrasound and γ-aminobutyric acid on polyphenol metabolites and metabolic pathways in mung bean sprouts. Front Nutr. (2022) 9:1081351. doi: 10.3389/fnut.2022.1081351
9. Xie, C, Zhu, Y, Leng, C, Wang, Q, Wang, P, and Yang, R. Investigation into the relationship between spermidine degradation and phenolic compounds biosynthesis in barley seedlings under ultraviolet-B stress. Plants. (2023) 12:3533. doi: 10.3390/plants12203533
10. Duric, M, Subotic, A, Prokic, L, Trifunovic-Momcilov, M, and Milosevic, S. Foliar application of methyl jasmonate affects impatiens walleriana growth and leaf physiology under drought stress. Plant Signal Behav. (2023) 18:2219936. doi: 10.1080/15592324.2023.2219936
11. Ndiaye, A, Diallo, A, Fall, N, Diouf, R, Diouf, D, and Kane, N. Transcriptomic analysis of methyl jasmonate treatment reveals gene networks involved in drought tolerance in pearl millet. Sci Rep. (2022) 12:5158. doi: 10.1038/s41598-022-09152-6
12. Verma, G, Srivastava, D, Narayan, S, Shirke, PA, and Chakrabarty, D. Exogenous application of methyl jasmonate alleviates arsenic toxicity by modulating its uptake and translocation in rice (Oryza sativa L.). Ecotoxicol Environ Saf. (2020) 201:110735. doi: 10.1016/j.ecoenv.2020.110735
13. Rahmati, M, Golkar, P, and Tarkesh, M. Effects of methyl jasmonate elicitation on the carvone and limonene contents, phenolic compounds and antioxidant activity in caraway (Carum carvi L.) callus cultures. Nat Prod Res. (2023) 37:4221–6. doi: 10.1080/14786419.2023.2169862
14. Palai, G, and D'Onofrio, C. Berry secondary metabolites and leaf physiological parameters are independently regulated by exogenous methyl jasmonate application in Sangiovese grapevines (Vitis vinifera L.). Plant Physiol Biochem. (2024) 207:108378. doi: 10.1016/j.plaphy.2024.108378
15. Hattori, A, Migitaka, H, Iigo, M, Itoh, M, Yamamoto, K, Ohtani-Kaneko, R, et al. Identification of melatonin in plants and its effects on plasma melatonin levels and binding to melatonin receptors in vertebrates. Biochem Mol Biol Int. (1995) 35:627–34.
16. Wang, K, Xing, Q, Ahammed, GJ, and Zhou, J. Functions and prospects of melatonin in plant growth, yield, and quality. J Exp Bot. (2022) 73:5928–46. doi: 10.1093/jxb/erac233
17. Arnao, M, and Hernández-Ruiz, J. Melatonin in flowering, fruit set and fruit ripening. Plant Reprod. (2020) 33:77–87. doi: 10.1007/s00497-020-00388-8
18. Liu, G, Hu, Q, Zhang, X, Jiang, J, Zhang, Y, and Zhang, Z. Melatonin biosynthesis and signal transduction in plants in response to environmental conditions. J Exp Bot. (2022) 73:5818–27. doi: 10.1093/jxb/erac196
19. Maity, S, Guchhait, R, and Pramanick, K. Melatonin mediated activation of MAP kinase pathway may reduce DNA damage stress in plants: a review. Biofactors. (2022) 48:965–71. doi: 10.1002/biof.1882
20. Dou, J, Wang, J, Tang, Z, Yu, J, Wu, Y, Liu, Z, et al. Application of exogenous melatonin improves tomato fruit quality by promoting the accumulation of primary and secondary metabolites. Food Secur. (2022) 11:4097. doi: 10.3390/foods11244097
21. Jafari, M, and Shahsavar, A. The effect of foliar application of melatonin on changes in secondary metabolite contents in two citrus species under drought stress conditions. Front Plant Sci. (2021) 12:692735. doi: 10.3389/fpls.2021.692735
22. Song, Z, Yang, Q, Dong, B, Li, N, Wang, M, Du, T, et al. Melatonin enhances stress tolerance in pigeon pea by promoting flavonoid enrichment, particularly luteolin in response to salt stress. J Exp Bot. (2022) 73:5992–6008. doi: 10.1093/jxb/erac276
23. Khan, V, Umar, S, and Iqbal, N. Synergistic action of Pseudomonas fluorescens with melatonin attenuates salt toxicity in mustard by regulating antioxidant system and flavonoid profile. Physiol Plant. (2023) 175:e14092. doi: 10.1111/ppl.14092
24. Yin, Y, Xu, J, He, X, Yang, Z, Fang, W, and Tao, J. Role of exogenous melatonin involved in phenolic acid metabolism of germinated hulless barley under NaCl stress. Plant Physiol Biochem. (2022) 170:14–22. doi: 10.1016/j.plaphy.2021.11.036
25. Yin, Y, Tian, X, He, X, Yang, J, Yang, Z, and Fang, W. Exogenous melatonin stimulated isoflavone biosynthesis in NaCl-stressed germinating soybean (Glycine max L.). Plant Physiol Biochem. (2022) 185:123–31. doi: 10.1016/j.plaphy.2022.05.033
26. Ma, Y, Wang, P, Zhou, T, Chen, ZJ, Gu, ZX, and Yang, RQ. Role of Ca2+ in phenolic compound metabolism of barley (Hordeum vulgare L.) sprouts under NaCl stress. J. Sci. Food Agri. (2019) 99:5176–86. doi: 10.1002/jsfa.9764
27. Zhuang, L, Xu, K, Zhu, Y, Wang, F, Xiao, J, and Guo, L. Calcium affects glucoraphanin metabolism in broccoli sprouts under ZnSO4 stress. Food Chem. (2021) 334:127520. doi: 10.1016/j.foodchem.2020.127520
28. Wang, Y, Tian, S, and Xu, Y. Effects of high oxygen concentration on pro- and anti-oxidant enzymes in peach fruits during postharvest periods. Food Chem. (2005) 91:99–104. doi: 10.1016/j.foodchem.2004.05.053
29. Gu, Y, Qiu, Y, Wei, X, Li, Z, Hu, Z, Gu, Y, et al. Characterization of selenium-containing polysaccharides isolated from selenium-enriched tea and its bioactivities. Food Chem. (2020) 316:126371. doi: 10.1016/j.foodchem.2020.126371
30. Tian, W, Ehmke, L, Miller, R, and Li, Y. Changes in bread quality, antioxidant activity, and phenolic acid composition of wheats during early-stage germination. J Food Sci. (2019) 84:457–65. doi: 10.1111/1750-3841.14463
31. Yang, B, Yin, Y, Liu, C, Zhao, Z, and Guo, M. Effect of germination time on the compositional, functional and antioxidant properties of whole wheat malt and its end-use evaluation in cookie-making. Food Chem. (2021) 349:129125. doi: 10.1016/j.foodchem.2021.129125
32. Zhang, P, Hu, Y, Zhou, R, Zhang, X, Hu, H, and Lang, D. The antioxidant system response to drought-stressed Diospyros lotus treated with exogenous melatonin. PeerJ. (2022) 10:e13936. doi: 10.7717/peerj.13936
33. Wang, M, Liu, G, Guo, T, Xie, C, Wang, P, and Yang, R. UV-B radiation enhances isoflavone accumulation and antioxidant capacity of soybean calluses. Front Nutr. (2023) 10:1139698. doi: 10.3389/fnut.2023.1139698
34. Imran, M, Latif Khan, A, Shahzad, R, Aaqil Khan, M, Bilal, S, Khan, A, et al. Exogenous melatonin induces drought stress tolerance by promoting plant growth and antioxidant defence system of soybean plants. Aob Plants. (2021) 13:026. doi: 10.1093/aobpla/plab026
35. Yuan, X, An, J, Zheng, T, and Liu, W. Exogenous melatonin improves salt tolerance mainly by regulating the antioxidant system in cyanobacterium Nostoc flagelliforme. PeerJ. (2022) 10:e14479. doi: 10.7717/peerj.14479
36. Luo, C, Min, W, Akhtar, M, Lu, X, Bai, X, Zhang, Y, et al. Melatonin enhances drought tolerance in rice seedlings by modulating antioxidant systems, osmoregulation, and corresponding gene expression. Int J Mol Sci. (2022) 23:12075. doi: 10.3390/ijms232012075
37. Yuan, P, Luo, F, Gleason, C, and Poovaiah, B. Calcium/calmodulin-mediated microbial symbiotic interactions in plants. Front Plant Sci. (2022) 13:984909. doi: 10.3389/fpls.2022.984909
38. Ciacka, K, Staszek, P, Sobczynska, K, Krasuska, U, and Gniazdowska, A. Nitric oxide in seed biology. Int J Mol Sci. (2022) 23:14951. doi: 10.3390/ijms232314951
39. Perez-Zoghbi, J, Bai, Y, and Sanderson, M. Nitric oxide induces airway smooth muscle cell relaxation by decreasing the frequency of agonist-induced Ca2+ oscillations. J Gen Physiol. (2010) 135:247–59. doi: 10.1085/jgp.200910365
40. Corpas, F, and Barroso, J. Peroxisomal plant metabolism-an update on nitric oxide, Ca2+ and the NADPH recycling network. J Cell Sci. (2018) 131:202978. doi: 10.1242/jcs.202978
41. Maksoud, M, Tellios, V, Xiang, Y, and Lu, W. Nitric oxide displays a biphasic effect on calcium dynamics in microglia. Nitric Oxide. (2021) 108:28–39. doi: 10.1016/j.niox.2021.01.001
42. Moscatiello, R, Mariani, P, Sanders, D, and Maathuis, F. Transcriptional analysis of calcium-dependent and calcium-independent signalling pathways induced by oligogalacturonides. J Exp Bot. (2006) 57:2847–65. doi: 10.1093/jxb/erl043
43. Sharma, A, Shahzad, B, Rehman, A, Bhardwaj, R, Landi, M, and Zheng, B. Response of phenylpropanoid pathway and the role of polyphenols in plants under abiotic stress. Molecules. (2019) 24:2452. doi: 10.3390/molecules24132452
44. Lin, M, Han, P, Li, Y, Wang, W, Lai, D, and Zhou, L. Quinoa secondary metabolites and their biological activities or functions. Molecules. (2019) 24:2512. doi: 10.3390/molecules24132512
45. Ma, Y, Wang, P, Chen, Z, Gu, Z, and Yang, R. NaCl stress on physio-biochemical metabolism and antioxidant capacity in germinated hulless barley (Hordeum vulgare L.). J Sci Food Agric. (2019) 99:1755–64. doi: 10.1002/jsfa.9365
Keywords: phenolic acids, barley sprouts, methyl jasmonate, melatonin, gene expression
Citation: Tian X, Zhang R, Yang Z, Zhu J, Fang W, Yang R and Yin Y (2024) Melatonin mediates phenolic acids accumulation in barley sprouts under MeJA stress. Front. Nutr. 11:1403293. doi: 10.3389/fnut.2024.1403293
Received: 19 March 2024; Accepted: 20 May 2024;
Published: 04 June 2024.
Edited by:
Yifen Lin, Fujian Agriculture and Forestry University, ChinaReviewed by:
Sen Cao, Guiyang University, ChinaCopyright © 2024 Tian, Zhang, Yang, Zhu, Fang, Yang and Yin. This is an open-access article distributed under the terms of the Creative Commons Attribution License (CC BY). The use, distribution or reproduction in other forums is permitted, provided the original author(s) and the copyright owner(s) are credited and that the original publication in this journal is cited, in accordance with accepted academic practice. No use, distribution or reproduction is permitted which does not comply with these terms.
*Correspondence: Runqiang Yang, eWFuZ3JxQG5qYXUuZWR1LmNu; Yongqi Yin, eXF5aW5AeXp1LmVkdS5jbg==
†These authors have contributed equally to this work
Disclaimer: All claims expressed in this article are solely those of the authors and do not necessarily represent those of their affiliated organizations, or those of the publisher, the editors and the reviewers. Any product that may be evaluated in this article or claim that may be made by its manufacturer is not guaranteed or endorsed by the publisher.
Research integrity at Frontiers
Learn more about the work of our research integrity team to safeguard the quality of each article we publish.