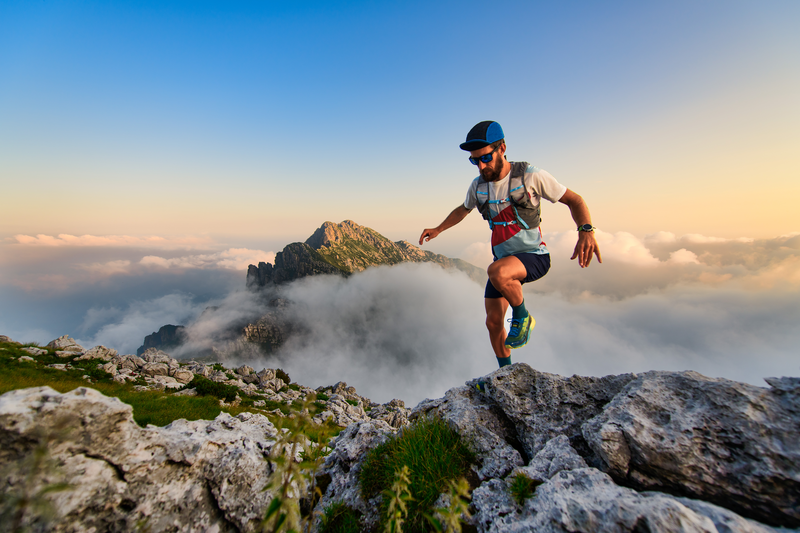
94% of researchers rate our articles as excellent or good
Learn more about the work of our research integrity team to safeguard the quality of each article we publish.
Find out more
MINI REVIEW article
Front. Nutr. , 24 May 2024
Sec. Nutritional Immunology
Volume 11 - 2024 | https://doi.org/10.3389/fnut.2024.1399687
This article is part of the Research Topic Immunomodulation by Food Components Via Dendritic Cells View all 7 articles
The concept of inflammation encompasses beneficial and detrimental aspects, which are referred to as infectious and sterile inflammations, respectively. Infectious inflammation plays a crucial role in host defense, whereas sterile inflammation encompasses allergic, autoimmune, and lifestyle-related diseases, leading to detrimental effects. Dendritic cells and macrophages, both of which are representative mononuclear phagocytes (MNPs), are essential for initiating immune responses, suggesting that the regulation of MNPs limits excessive inflammation. In this context, dietary components with immunomodulatory properties have been identified. Among them, soybean-derived compounds, including isoflavones, saponins, flavonoids, and bioactive peptides, act directly on MNPs to fine-tune immune responses. Notably, some soybean-derived compounds have demonstrated the ability to alleviate the symptom of allergy and autoimmunity in mouse models. In this review, we introduce and summarize the roles of soybean-derived compounds on MNP-mediated inflammatory responses. Understanding the mechanism by which soybean-derived molecules regulate MNPs could provide valuable insights for designing safe immunomodulators.
Inflammation serves as an immune response to infection or sterile tissue damage. Infectious inflammation is largely mediated by innate immunity against infectious agents, such as pathogen-associated molecular patterns, which contributes to the early host defense (1). In this process, mononuclear phagocyte (MNP)-derived reactive oxygen species (ROS) are essential for eliminating invading pathogens, often accompanied by collateral tissue damages. Paradoxically, upon the elimination of pathogens, inflammation promotes wound healing and tissue remodeling, leading to beneficial effects on the host (1). Conversely, sterile inflammation is mediated by both innate and adaptive immunity against self-antigens, such as altered organ-specific antigens and damage-associated molecular patterns (also known as alarmin) released by injured or dying cells, as well as harmless exogenous antigens, including dietary proteins and airborne pollens. This type of inflammation causes allergic, autoimmune, and lifestyle-related (non-communicable) diseases, which lead to long-term detrimental effects (1, 2). Therefore, the sterile chronic inflammatory diseases are thought to be impaired immune tolerance or suppression against self-antigens and harmless exogenous antigens, which are tightly regulated by MNPs.
Dendritic cells (DCs) and macrophages, both of which are representative MNPs, share the capacity of antigen-presentation to T cells. These MNPs are distributed in almost all organs and tissues, playing an essential role in inducing immunity to non-self-antigens and promoting tolerance to self-antigens as well as harmless exogenous antigens (3, 4). DCs are the specialized phenotype, stimulating antigen-specific naïve T cells and functioning in both innate and adaptive immunity. In contrast, macrophages activate DC-induced antigen-experienced effector T cells and participate in innate rather than adaptive immunity (3, 4). Therefore, the functional impairment of MNPs leads to inappropriate immune responses against antigens, contributing to the onset of various inflammatory diseases (3, 4).
Sterile inflammations are associated with genetic predispositions, which can be triggered by physical, chemical, and metabolic stimuli. Interestingly, the prevalence of sterile inflammations, especially autoimmune diseases, is much higher in Western countries than in Asian countries, highlighting the significance of the Western lifestyle as an environmental risk factor (5). Habitual intake of high-calorie Western diets, which contain low amounts of plant-derived components, such as fibers and antioxidants, is thought to exacerbate inflammatory diseases (6). In this context, although soybeans (Glycine max) are an important nutrient as a major source of plant-based protein, which are being consumed worldwide (7), fermented soybean products, including soy source, natto, miso, tofuyo, douchi, tempe, thua nao, and sufu, are preferentially consumed in Asian countries (8). Consistent with these reports, a large cohort study revealed that the habitual intake of soybeans and/or fermented soybean products can prevent the development of cardiovascular diseases, including atherosclerosis (9). Furthermore, accumulating evidence suggests that soybeans contain various immunomodularoty compounds with pro- and anti-inflammatory properties, including isoflavones, saponins, and bioactive peptides. These compounds act directly on MNPs to finely regulate immune responses in a context-dependent manner (10). Furthermore, dietary soybean-derived compounds have been demonstrated to prevent inflammatory responses in allergen- or self-antigen-sensitized mice (11–14), implying that these compounds regulate the function of MNPs that reside in the intestine, the primary site for digestion and absorption of dietary components. Notably, the intake of soybeans and some soybean-derived compounds has been shown to improve the composition and diversity of gut microbiota and increase the relative abundance of short-chain fatty acid-producing bacteria that are involved in inducing anti-inflammatory immune responses in mammals, indicating that soybean-derived compounds indirectly impact immune cells by altering the microbiota (15–17).
Considering the pivotal role of MNPs in the onset of various inflammatory diseases and the regulatory effect of soybean-derived compounds on MNPs, these compounds may serve as novel immunomodulators targeting MNPs. Here, we present a comprehensive review and discussion of the current understanding of the immunomodulation via MNPs by soybean-derived compounds, largely based on the knowledge obtained from studies on mouse models.
MNPs comprise DCs, macrophages, and monocytes. Among them, DCs and macrophages share common functionalities in host defense, such as antigen recognition, uptake, processing, and presentation. However, MNPs exhibit differences in their origin, differentiation, phenotype, and localization, comprising heterogeneous populations.
DCs consist of two subsets: FMS-like tyrosine kinase ligand-dependent DCs and colony-stimulating factor (CSF) 1/2-dependent monocyte-derived DCs. The former includes CD11chiMHC class II+ conventional DCs (cDCs) and CD11cintMHC class IIlo plasmacytoid DCs (pDCs) (3). Furthermore, cDCs can be divided into type 1 (cDC1) and type 2 cDCs (cDC2) based on the expression of cell surface markers and transcription factors, as described elsewhere (4).
Upon engulfing antigens, including bacteria, cDCs migrate into the regional lymph nodes through afferent lymphatic ducts in a chemokine (C-C motif) receptor 7 (CCR7)-dependent manner. After arriving at the lymph nodes, cDCs prime antigen-specific naive T cells via antigen presentation and costimulation (3, 4). During this process, “immunogenic” cDCs secrete various cytokines that induce the differentiation of naïve CD4+ T cells into mature helper T (Th) cells. For example, interleukin (IL)-12, IL-4, and IL-23 selectively induce the differentiation into Th1, Th2, and Th17, respectively. Furthermore, cDC-induced antigen-specific Th cells promote the differentiation of B cells into antibody-producing plasma cells, facilitating antigen eliminations. In contrast, “tolerogenic” regulatory cDCs conditioned under an anti-inflammatory environment secrete transforming growth factor (TGF)-β1 and IL-10 to induce regulatory T (Treg) cells. Unlike cDCs, pDCs sensed antigens, including viruses, and then migrate into the lymph nodes through the bloodstream in a CCR9-dependent manner. Despite their lower antigen-presenting capacity compared to that of cDCs, pDCs can secrete substantial amounts of type-I interferons (IFNs) to eliminate viruses and virus-infected cells (3, 4).
Monocyte-derived DCs spread into inflamed tissues in a CCR2-dependent manner. Upon infection with Listeria monocytogenes, tumors necrosis factor (TNF)-α and inducible nitric oxide (NO) synthase-producing DCs (Tip-DCs), a subset of monocyte-derived DCs, are predominantly induced at the site of infection in a CCR2- and myeloid differentiation primary response 88 (MyD88)-dependent manner, leading to the initiation of Th1-type immune responses to eliminate the bacteria (18, 19). Interestingly, we found that, under steady-state conditions, “naturally occurring” Tip-DCs that promote immunoglobulin A production are induced in the small intestine by toll-like receptor (TLR)-dependent recognition of microbiota (20). This subset also originates from monocytes, which are recruited into the intestine in a CCR2- and MyD88-dependent manner (20, 21).
Macrophages are divided into tissue-resident and monocyte-derived subsets based on their ontogeny, localization, and functions (22). Tissue-resident macrophages, which originate from progenitors in the embryonic yolk sac and fetal liver, populate various organs, including the brain, dermis, liver, kidney, lung, and intestine, before birth. These tissue-resident subsets perpetuate their numbers through self-renewal to maintain tissue homeostasis (22). On the other hand, monocyte-derived subsets originate from common monocyte progenitors in the bone marrow after birth (23). Notably, under stady-state conditions, some tissue-resident macrophages in the organs, excluding the brain, are replaced by monocyte-derived macrophages during aging in a CSF1-dependent manner (22). In inflammatory contexts, monocytes selectively migrate into inflamed tissues in a CCR1/CCR2/CCR5/CX3CR1-dependent manner, where they generally differentiate into M1 macrophages, as described below.
Numerous culture experiments have demonstrated that F4/80+CD11b+ activated macrophages are divided into “classically” M1 and “alternatively” M2 subsets based on their functional and phenotypic properties (24). M1 macrophages induced by stimulation with IFN-γ and lipopolysaccharides (LPS), a cell wall component from Gram-negative bacteria, represent a pro-inflammatory “immunogenic” phenotype. This subset initiates immune responses against invading pathogens or tumors by producing pro-inflammatory factors, such as TNF-α, NO, and ROS, in a nuclear factor kappa B (NF-κB) signaling-dependent manner (24, 25). In contrast, M2 macrophages induced by Th2 cell-derived cytokines, such as IL-4 and IL-10, represent an anti-inflammatory “tolerogenic” phenotype. This subset promotes the remodeling of damaged tissues by producing anti-inflammatory mediators, such as TGF-β1 and IL-10, thereby resolving inflammation (24). Simultaneously, M2 subsets promote the remodeling of damaged tissues by producing ornithine, a substrate of polyamine synthesis. Nowadays, anti-inflammatory M2 macrophages can be categorized into at least four subsets (M2a, M2b, M2c, and M2d) based on the expression of transcription factors (26). Given that M2 macrophages play a role in repairing M1 macrophage-mediated tissue damages, their appearance in damaged tissues is likely attributable to be either the reprogramming from M1 to M2 subsets or the replenishment of M2 subsets during the recovery process. Although the concept of reprogramming seems plausible, the mechanisms underlying the reprogramming toward M2 subsets remain unclear.
MNPs recognize various antigens through pattern-recognition receptor TLRs and scavenger receptor C-type lectins. These receptors activate ubiquitous transcription factor NF-κB via various intracellular signaling pathways, including MyD88, mitogen-activated protein kinase (MAPK), and phosphoinositide 3-kinase (PI3K)/Akt (also known as protein kinase B) (25). Once activated, NF-κB is recruited into the nucleus, binding to the promoter regions of genes encoding inflammatory factors, including pro-inflammatory cytokines and the NOD-like receptor family, pyrin domain-containing 3 inflammasome complex, leading to the induction of immunogenic MNPs (25). Therefore, the regulation of ubiquitous transcription factor in MNPs could prevent aberrant inflammation. In this section, we describe the significance of MNPs in inflammatory diseases, drawing insights obtained from studies on mice with MNP-specific disruption of the NF-κB family, which is the master regulator of immune responses.
Macrophage-specific deficiency of inhibitory κB kinase β (IKKβ), an NF-κB activating kinase, protected mice from myelin oligodendrocyte glycoprotein-induced experimental autoimmune encephalomyelitis (EAE), a model of multiple sclerosis, due to a reduced number of Th1 and Th17 cells, along with an increased number of Treg cells in the spinal cord (27). Macrophage-specific deletion of IKKβ in low-density lipoprotein receptor-deficient mice led to reduced high-fat diet-induced atherosclerosis (28). These findings indicate that, upon recognition of self-antigens, prolonged NF-κB activation in MNPs induces inflammatory diseases through the activation of pathogenic T cell subsets. Conversely, macrophages-specific IKKβ- or p65-deficient mice showed increased sensitivity to LPS-induced shock due to elevated production of pro-inflammatory cytokines, including IL-1β, IL-6, and TNF-α (29, 30). These findings suggest that NF-κB signaling in MNPs limits the aberrant inflammation against infectious agents. Therefore, the regulation of MNPs with dual roles in inducing immunity to non-self-antigens and tolerance to self-antigens is dependent on NF-κB signaling. This signaling pathway serves as a target for soybean-derived compounds, as described below.
Soybeans have long been recognized for their nutritional value and health benefits (7–9). In addition to their well-established effects on cardiovascular health (31) and cholesterol levels (32), soybean-derived compounds influence on the immune system (10, 33). Among these compounds, isoflavones, saponins, flavonoids, peptides, proteins, lipids, vitamins, and minerals have been confirmed to have immunomodulatory effects. This section focuses on the interplay between the various soy compounds and MNPs (Table 1), highlighting the potential immunomodulatory effects that may contribute to overall health.
Among well-studied soybean-derived compounds, isoflavones standout bioactive compounds. Genistein and daidzein, the representative soy isoflavone aglycones, are synthesized from their glycosides genistin and daidzin, respectively, by gut microbial b-galactosidase (52). Notably, these isoflavone aglycones function as phytoestrogens with structural similarities to mammalian estrogens, acting as estrogen receptor modulators (53, 54) (Figure 1). However, recent studies have expanded their role beyond estrogen modulation, revealing their impact on MNPs, such as DCs and macrophages (11, 34, 35, 55, 56) (Table 1). Upon stimulation with LPS, these compounds regulate the production of inflammatory cytokines (IL-1β, IL-6, IL-12, and TNF-α), mediators (NO, prostaglandin E2 [PGE2], and ROS), phagocytosis, and differentiation in human monocyte-derived DCs and the murine macrophage cell line RAW264.7 cells, suggesting that isoflavones prevent the generation of immunogenic MNP subsets (11, 34, 35, 55, 56). Notably, dietary genistein skewed murine macrophage polarization toward M2-like subsets and improved clinical symptoms in a dextran sulfate sodium-induced colitis model (35, 56). Mechanistically, the immunomodulatory effect of isoflavone glycosides in water-soluble soybean extracts on murine MNPs is thought to be mediated by inhibiting NF-κB-signaling pathways through suppression of IKKα/β phosphorylation (35) (Table 1). Understanding the intricate interaction between soy isoflavones and MNPs is pivotal for elucidating the immunological mechanisms underlying soy isoflavone-mediated health effects and their potential applications in therapeutic interventions for various inflammatory diseases.
Figure 1. Chemical structures of representative soybean-derived compounds with immunomodulatory properties. The structures of isoflavone aglycones (A) and soyasaponins (B) is shown for comparison.
Soy saponin is general term for oleanane triterpene (aglycone basic skelton) glycosides, of which there are more than 50 different molecular species. These diverse species originate from differences in aglycone structure, sugar chain, and sugar acetylation (57) (Figure 1). Soy saponins are naturally occurring compounds with diverse biological activities (57, 58). Some studies showed that soy saponins regulate the production of pro-inflammatory cytokines and mediators by murine macrophages (37, 38) (Table 1). For example, soy saponins inhibit the production of inflammatory mediators, such as IL-1β, TNF-α, NO, PGE2, and ROS, in LPS-stimulated peritoneal macrophages and RAW264.7 cells (37, 38), possibly prompting to polarization toward M2-like subsets. The soy saponin-induced polarization toward M2-like subsets is dependent on the suppression of TLR4-mediated activation of NF-κB, MAPK, and PI3K/Akt (37–39) (Table 1). Therefore, dietary saponins may contribute to the regulation of excessive immune responses.
Soy saponins exhibit structural diversity, as they are a diverse group of amphiphilic glycosides with distinctive chemical structures (Figure 1). Therefore, their immunomodulatory effects may vary depending on differences in chemical compositions. This is evident in the distinct actions of soyasoponin Ab compared to that of soyasaponin I in regulating macrophage function. Specifically, soyasaponin Ab inhibits the binding of LPS to the cell surface receptor TLR4 (37), whereas soyasaponin I directly inhibit the phosphorylation of IκBα (39) (Table 1). Understanding the structure–activity relationships of soy saponins is crucial for elucidating the molecular mechanism through which they influence the regulation of immune responses.
Soy flavonoids, a diverse group of phytochemicals abundantly present in soy-based products, have garnered significant attention owing to their potential health benefits (59). Soy flavones, including apigenin and chrysin, prevent LPS-induced production of NO and TNF-α in RAW264.7 cells (40). In contrast, black soybean-derived anthocyanines prevent the production of pro-inflammatory cytokines (IL-6 and TNF-α), chemokines (MCP-1), and mediators (NO, PGE2, and ROS) by inhibiting MAPK/JNK/ERK/p38, upstream molecules of NF-κB, in LPS-stimulated murine macrophages (41, 42) (Table 1). In an adipocyte-macrophage coculture experiment, anthocyanins prevented murine macrophage-stimulated release of free-fatty acids and improved insulin resistance in adipocytes (42). These findings suggest that isoflavone-mediated polarization toward M2-like subsets improves sterile inflammatory diseases, including type 2 diabetes.
Soy proteins, abundant in essential amino acids and bioactive peptides, play important roles in immune regulation (10). In an experiment using RAW264.7 cells, soy protein-derived crude low-molecular weight peptides inhibited LPS-induced production of M1-type cytokines (IL-1β, IL-6, and TNF-α) by suppressing MAPK/JUN pathways and IκB phosphorylation in NF-κB pathways (43). Recent findings revealed that hydrolyzed soy protein containing bioactive lunasin, a 43-amino acid peptide, inhibited LPS-induced secretion of M1-type mediators (IL-1β, IL-6, and NO) through suppressing NF-κB nuclear translocation in RAW264.7 cells (44). Contrary, lunasin enhances phagocytosis and induces the production of M1-type mediators in unstimulated RAW264.7 cells, contributing to the activation of immune responses (45, 46) (Table 1). Lunasin also exerts diverse effects on human monocyte-derived DCs, influencing their maturation, M1-type cytokine production, and antigen presentation, which potentiate adaptive immune responses as an adjuvant (47). These findings suggest that the regulation of macrophages by lunasin in the presence of LPS leads to opposing immune consequences.
Soybean-derived lipids, including phospholipids and sterols, have been investigated for their immunomodulatory effects (60). A human study revealed that individuals on a soy oil-supplemented diet showed a tendency toward lower LPS-induced production of IL-6 and TNF-α in peripheral blood mononuclear cells than those on a control diet (61). This finding suggests the presence of unique fatty acids with immunomodulatory effects. Soy lipids contain a diverse array of compounds, including phospholipids, glycolipids, and triglycerides (60). Among them, soy phospholipids, particularly phosphatidylinositol, inhibit LPS-induced expression of IL-1β and MCP-1 through the suppression of IKK phosphorylation in the human monocytic cell line THP-1 (48). Additionally, soy lecithin (also known as phosphatidylcholine) influences murine macrophage function, affecting their phagocytic and antigen-processing abilities (49) (Table 1). Collectively, soy lipids presumably regulate NF-κB pathways in MNPs, influencing the expression of genes involved in inflammatory responses. The interplay between soy lipids and MNPs presents a fascinating avenue for research in the field of immunonutrition.
Polysaccharides derived from legumes exhibit various immunomodulatory functions (62). Particularly, soy polysaccharides induce the production of pro-inflammatory cytokines (IL-1β, IL-6, IL-12, and TNF-α) and mediators (NO and ROS) in unstimulated RAW264.7 cells (50, 51). Notably, the immunostimulatory effects are dependent on JUN/ERK/p38-mediated activation of NF-kB signaling (51) (Table 1). These findings suggest that soybean-derived immunostimulatory agents targeting MNPs offer protective effects against infections. Additionally, soybeans represent an abundant source of vitamins (vitamin E, D, and folate) and minerals (zinc), essential for maintaining immune responses (63–65). These micronutrients may directly or indirectly influence MNP functions by supporting cellular processes critical for antigen presentation and immune response regulation.
The higher prevalence of inflammatory diseases in Western countries than in Asian countries correlates well with the observed habitual intake of soybeans and their products (8). Soybean-derived compounds, including genistein, daidzein, soyasaponin, anthocyanins, phospholipids, and peptides, prevent LPS-induced production of inflammatory mediators in MNPs (34, 37, 38, 41–43, 48, 55), possibly achieved through the suppression of NF-κB pathways as described above. In this context, dietary isoflavones, including genistein and daidzein, reduce the anaphylactic symptoms in peanut antigen-sensitized mice, due to reduced DC maturation (11).
Our research revealed that a hot water extract of green soybeans significantly reduces the level of antigen-specific IgE in an ovalbumin-sensitized allergic mouse model and suppresses nasal secretion in a 2, 4-toluene diisocyanate-induced guinea pig rhinitis model (12). The human trial study consisted of two open-label treatment phases. In Part 1, participants were administered a daily single dose of green soybean extracts (3 g) over a 6-week period. In a trial study involving 16 patients with cedar pollinosis (8 men and 8 women aged 30 to 60 years) living in the Kanto region of Japan, those who continued to consume green soybeans after pollen dispersal experienced less severe symptoms of cedar pollinosis than those who had ceased consumption before pollen dispersal (12). This result suggests that green soybean extract holds considerable potential as an orally effective immunomodulator for treating various allergic diseases. Soybeans contain several bioactive compounds, such as genistein, daidzein, and saponins, which have been independently reported to possess anti-inflammatory and immunomodulatory properties. These compounds may partially explain the bioactivities of green soybean extracts. However, green soybeans have slightly higher levels of these compounds than in yellow soybeans (36). Additionally, there may be other unknown compounds present in green soybean extracts that contribute to their full range of bioactivities. Furthermore, we screened visible light-irradiated soybean extracts (LIEGS) inhibiting the production of cytokines. LIEGS strongly suppressed IL-2 production in Jurkat cells, with the effect being specific to green soybeans but not to other colored soybeans (36). LIEGS also suppressed LPS-induced expression of IL-6, IL-12, and TNF-α in THP-1 cells in a dose-dependent manner. Furthermore, dietary LIEGS reduced dermatitis scores and improved skin damages in NC/Nga mice, an atopic dermatitis model, possibly due to the suppression of pro-inflammatory cytokine production by MNPs (36). Finally, we successfully identified protodaidzeone, an isoflavone from LIEGS, as a novel dietary immunomodulator (36).
The multifaceted components of soybeans exert a complex yet intriguing influence on MNPs. The interactions between MNPs and isoflavones, proteins, saponins, lipids, and micronutrients contribute to the immunomodulatory potential of soybeans. A comprehensive understanding of these mechanisms may offer insights for developing dietary strategies to finely regulate immune responses and promote overall health. Immunomodulatory research centered on soybean-derived compounds holds significant potential for enhancing human health and combating various inflammatory diseases. Therefore, continued exploration of the mechanisms underlying the immunomodulatory effects of soybean-derived compounds and their therapeutic applications is crucial for harnessing their full potential. Although substantial preclinical research supports the immunomodulatory potential of soybean-derived compounds, further clinical trials and human studies are essential to validate these findings and assess their efficacy, safety, and optimal dosing regimens in diverse populations.
HT: Conceptualization, Funding acquisition, Project administration, Supervision, Validation, Writing – original draft, Writing – review & editing. SI: Conceptualization, Validation, Writing – original draft, Writing – review & editing.
The author(s) declare that financial support was received for the research, authorship, and/or publication of this article. This work was supported by Aichi Kidney Foundation (HT).
We thank Nao Shimoga for redrawing the Table 1, Yui Shibata for drafting Figure 1, and Chikako Hibiya for secretarial support. We apologize for omitting the references due to space limitations.
The authors declare that the research was conducted in the absence of any commercial or financial relationships that could be construed as a potential conflict of interests.
All claims expressed in this article are solely those of the authors and do not necessarily represent those of their affiliated organizations, or those of the publisher, the editors and the reviewers. Any product that may be evaluated in this article, or claim that may be made by its manufacturer, is not guaranteed or endorsed by the publisher.
DCs, dendritic cells; MNPs, mononuclear phagocytes.
1. Zindel, J, and Kubes, P. DAMPs, PAMPs, and LAMPs in immunity and sterile inflammation. Annu Rev Pathol. (2020) 15:493–518. doi: 10.1146/annurev-pathmechdis-012419-032847
2. Zitvogel, L, Pietrocola, F, and Kroemer, G. Nutrition, inflammation and cancer. Nat Immunol. (2017) 18:843–50. doi: 10.1038/ni.3754
3. Chow, A, Brown, BD, and Merad, M. Studying the mononuclear phagocyte system in the molecular age. Nat Rev Immunol. (2011) 11:788–98. doi: 10.1038/nri3087
4. Cabeza-Cabrerizo, M, Cardoso, A, Minutti, CM, Pereira da Costa, M, Reis e Sousa, C, and Sousa, C. Dendritic cells revisited. Annu Rev Immunol. (2021) 39:131–66. doi: 10.1146/annurev-immunol-061020-053707
5. Cao, F, Liu, Y-C, Ni, Q-Y, Chen, Y, Wan, C-H, Liu, S-Y, et al. Temporal trends in the prevalence of autoimmune diseases from 1990 to 2019. Autoimmun Rev. (2023) 22:103359. doi: 10.1016/j.autrev.2023.103359
6. Christ, A, Lauterbach, M, and Latz, E. Western diet and the immune system: an inflammatory connection. Immunity. (2019) 51:794–811. doi: 10.1016/j.immuni.2019.09.020
7. Tsugane, S. Why has Japan become the world’s most long-lived country: insights from a food and nutrition perspective. Eur J Clin Nutr. (2021) 75:921–8. doi: 10.1038/s41430-020-0677-5
8. do Prado, FG, Pagnoncelli, MGB, de Melo Pereira, GV, Karp, SG, and Soccol, CR. Fermented soy products and their potential health benefits: a review. Microorganisms. (2022) 10:1606. doi: 10.3390/microorganisms10081606
9. Nozue, M, Shimazu, T, Charvat, H, Mori, N, Mutoh, M, Sawada, N, et al. Fermented soy products intake and risk of cardiovascular disease and total cancer incidence: the Japan public health center-based prospective study. Eur J Clin Nutr. (2021) 75:954–68. doi: 10.1038/s41430-020-00732-1
10. Tezuka, H, and Imai, S. Immunomodulatory effects of soybeans and processed soy food compounds. Recent Pat Food Nutr Agric. (2015) 7:92–9. doi: 10.2174/2212798407666150629123957
11. Masilamani, M, Wei, J, Byatt, S, Paul, M, Yakir, S, and Sampson, HA. Soybean isoflavones regulate dendritic cell function and suppress allergic sensitization to peanut. J Allergy Clin Immunol. (2011) 128:1242–1250.e1. doi: 10.1016/j.jaci.2011.05.009
12. Katayanagi, Y, Yasui, K, Ikemoto, H, Taguchi, K, Fukutomi, R, Isemura, M, et al. The clinical and immunomodulatory effects of green soybean extracts. Food Chem. (2013) 138:2300–5. doi: 10.1016/j.foodchem.2012.12.014
13. Verdregh, M, Jonsson, IM, Holmdahl, R, and Tarkowski, A. Genistein as an anti-inflammatory agent. Inflamm Res. (2003) 52:341–6. doi: 10.1007/s00011-003-1182-8
14. Yang, X, Zhu, J, Tung, CY, Gardiner, G, Wang, Q, Chang, HC, et al. Lunasin alleviates allergic airway inflammation while increases antigen-specific Tregs. PLoS One. (2015) 10:e0115330. doi: 10.1371/journal.pone.0115330
15. Huang, H, Krishnan, HB, Pham, Q, Yu, LL, and Wang, TTY. Soy and gut microbiota: interaction and implication for human health. J Agric Food Chem. (2016) 64:8695–709. doi: 10.1021/acs.jafc.6b03725
16. Huang, L, Zheng, T, Hui, H, and Xie, G. Soybean isoflavones modulate gut microbiota to benefit the health weight and metabolism. Front Cell Infect Microbiol. (2022) 12:1004765. doi: 10.3389/fcimb.2022.1004765
17. Kayama, H, Okumura, R, and Takeda, K. Interaction between the microbiota, epithelia, and immune cells in the intestine. Annu Rev Immunol. (2020) 38:23–48. doi: 10.1146/annurev-immunol-070119-115104
18. Serbina, NV, Salazar-Mather, TP, Biron, CA, Kuziel, WA, and Pamer, EG. TNF/iNOS-producing dendritic cells mediate innate immune defense against bacteria infection. Immunity. (2003) 19:59–70. doi: 10.1016/s1074-7613(03)00171-7
19. Serbina, NV, Kuziel, W, Flavell, R, Akira, S, Rollins, B, and Pamer, EG. Sequential MyD88-independent and-dependent activation of innate immune responses to intracellular bacteria infection. Immunity. (2003) 19:891–901. doi: 10.1016/s1074-7613(03)00330-3
20. Tezuka, H, Abe, Y, Iwata, M, Takeuchi, H, Ishikawa, H, Matsushita, M, et al. Regulation of IgA production by naturally occurring TNF/iNOS-producing dendritic cells. Nature. (2007) 448:929–33. doi: 10.1038/nature06033
21. Tezuka, H, and Ohteki, T. Regulation of intestinal homeostasis by dendritic cells. Immunol Rev. (2010) 234:247–58. doi: 10.1111/j.0105-2896.2009.00872.x
22. Mass, E, Nimmerjahn, F, Kierdorf, K, and Schlitzer, A. Tissue-specific macrophages: how they develop and choreograph tissue biology. Nat Rev Immunol. (2023) 23:563–79. doi: 10.1038/s41577-023-00848-y
23. Kawamura, S, and Ohteki, T. Monopoiesis in human and mouse. Int Immunol. (2018) 30:503–9. doi: 10.1093/intimm/dxy063
24. Murray, PJ. Macrophage polarization. Annu Rev Physiol. (2017) 79:541–66. doi: 10.1146/annurev-physiol-022516-034339
25. Mussbacher, M, Derler, M, Basilio, J, and Schmid, JA. NF-κB in monocytes and macrophages – an inflammatory master regulator in multitalented immune cells. Front Immunol. (2023) 14:1134661. doi: 10.3389/fimmu.2023.1134661
26. Strizova, Z, Benesova, I, Bartolini, R, Novysedlak, R, Cecrdlova, E, Foley, LK, et al. M1/M2 macrophages and their overlaps – myth or reality? Clin Sci. (2023) 137:1067–93. doi: 10.1042/cs20220531
27. Lee, MJ, Bing, SJ, Choi, J, Jang, M, Lee, G, Lee, H, et al. IKKβ-mediated inflammatory myeloid cell activation exacerbates experimental autoimmune encephalomyelitis by potentiality Th1/Th17 cell activation and compromising blood brain barrier. Mol Neurodegener. (2016) 11:54–74. doi: 10.1186/s13024-016-0116-1
28. Park, S-H, Sui, Y, Gizard, F, Xu, J, Rios-Pilier, J, Helsley, RN, et al. Myeloid-specific IkB kinase β deficiency decreases atherosclerosis in low-density lipoprotein receptor-deficient mice. Arterioscler Thromb Vasc Biol. (2012) 32:2869–76. doi: 10.1161/ATVBAHA.112.254573
29. Vanoni, S, Tsai, Y-T, Waddell, A, Waggoner, L, Klarquist, J, Divanovic, S, et al. Myeloid-derived NF-κB negative regulation of PU.1 and c/EBP-β-driven pro-inflammatory cytokine production restrains LPS-induced shock. Innate Immun. (2017) 23:175–87. doi: 10.1177/1753425916681444
30. Green, FR, Arkan, MC, Bollrath, J, Hsu, L-C, Goode, J, Miething, C, et al. NF-kappaB is negative regulator of IL-1 beta secretion as revealed by genetic and phamacological inhibition of IKKbeta. Cell. (2007) 130:918–31. doi: 10.1016/j.cell.2007.07.009
31. Clarkson, TB. Soy, soy phytoestrogens and cardiovascular disease. J Nutr. (2002) 132:566S–9S. doi: 10.1093/jn/132.3.566S
32. Messina, M, Duncan, A, Messina, V, Lynch, H, Kiel, J, and Erdman, JW Jr. The health effects of soy: a reference guide for health professionals. Front Nutr. (2022) 9:970364. doi: 10.3389/fnut.2022.970364
33. Messina, M, and Messina, V. The role of soy in preventing and treating chronic disease. J American College of Nutr. (1991) 10:406–19. doi: 10.1080/07315724.1991.10718186
34. Wei, J, Bhatt, S, Chang, LM, Sampson, HA, and Masilamani, M. Isoflavones, genistein, and daidzein, regulate mucosal immune response by suppressing dendritic cell function. PLoS One. (2012) 7:e47979. doi: 10.1371/journal.pone.0047979
35. Kim, S-E, Kawaguchi, K, Hayashi, H, Furusho, K, and Maruyama, M. Remission effect of dietary soybean. Isoflavones on DSS-induced murine colitis and LPS-activated macrophages cell line. Nutrients. (2019) 11:1746. doi: 10.3390/nu11081746
36. Tanaka, K, Ohgo, Y, Katayanagi, Y, Yasui, K, Hiramoto, S, Ikemoto, H, et al. Anti-inflammatory effects of green soybean extract irradiated with visible light. Sci Rep. (2014) 4:4732. doi: 10.1038/srep04732
37. Lee, IA, Park, YJ, Joh, EH, and Kim, DH. Soyasaponin Ab ameliorates colitis by inhibiting the binding of lipopolysaccharide (LPS) to toll-like receptor (TLR)4 on macrophages. J Agric Food Chem. (2011) 59:13165–72. doi: 10.1021/jf2033818
38. Zha, L, Chen, J, Sun, S, Mao, L, Chu, X, Deng, H, et al. Soyasaponins can blunt inflammation by inhibiting the reactive oxygen species-mediated activation of PI3K/Akt/NF-κB pathway. PLoS One. (2014) 9:e107655. doi: 10.1371/journal.pone.0107655
39. Lee, IA, Park, YJ, Yeo, HK, Han, MJ, and Kim, DH. Soyasaponin I attenuates TNBS-induced colitis in mice by inhibiting NF-κB pathway. J Agric Food Chem. (2010) 58:10929–34. doi: 10.1021/jf102296y
40. Lyu, SY, and Park, WB. Production of cytokine and NO by RAW 264.7 macrophages and PBMC in vitro incubation with flavonoids. Arch Pharm Res. (2005) 28:573–81. doi: 10.1007/BF02977761
41. Kim, J-N, Han, SN, Ha, TJ, and Kim, H-K. Black soybean anthocyanins attenuate inflammatory responses by suppressing reactive oxygen species production and mitogen activated protein kinases signaling in lipopolysaccharide-stimulated macrophages. Nutr Res Pract. (2017) 11:357–64. doi: 10.4162/nrp.2017.11.5.357
42. Kim, J-N, Han, SN, and Kim, H-K. Anti-inflammatory and anti-diabetic effect of black soybean anthocyanines: data from a dual cooperative cellular system. Molecules. (2021) 26:3363. doi: 10.3390/molecules26113363
43. Yi, G, Li, H, Liu, M, Ying, Z, Zhang, J, and Liu, X. Soybean protein-derived peptides inhibit inflammation in LPS-induced RAW264.7 macrophages via the suppression of TLR4-mediated MAPK-JUN and NF-kappa B activation. Food Biochem. (2020) 44:e13289. doi: 10.1111/jfbc.13289
44. de Mejia, EG, and Dia, VP. Lunasin and lunasin-like peptides inhibit inflammation through suppression of NF-κB pathway in the macrophages. Peptides. (2009) 30:2388–98. doi: 10.1016/j.peptides.2009.08.005
45. Hsieh, L-S, Lu, M-S, and Chiang, W-D. Identification and characterization of immunomodulatory peptides from soy protein hydrolysates. Bioresour Bioprocess. (2022) 9:39. doi: 10.1186/s40643-022-00526-2
46. Paterson, S, Frenadez-Tome, S, Galvez, A, and Hernandez-Ledesma, B. Evaluation of the multifunctionality of soybean proteins and peptides in immune cell models. Nutrients. (2023) 15:1220. doi: 10.3390/nu15051220
47. Tung, C-Y, Lewis, DE, Han, L, Java, M, Yao, S, Li, F, et al. Activation of dendritic cell function by soypeptide lunasin as a novel vaccine adjuvant. Vaccine. (2014) 32:5411–9. doi: 10.1016/j.vaccine.2014.07.103
48. Ohta, S, Asanoma, M, Irie, N, Tachibana, N, and Kohno, M. Soy phospholipids exert a renoprotective effect by inhibiting the nuclear factor kappa B pathway in macrophages. Meta. (2022) 12:330. doi: 10.3390/metabo12040330
49. Miranda, DTSZ, Batista, VG, Grando, FCC, Paula, FM, Felício, CA, Rubbo, GFS, et al. Soy lecithin supplementation alters macrophage phagocytosis and lymphocyte response to concanavalin a: a study in alloxan-induced diabetic rats. Cell Biochem Funct. (2008) 26:859–65. doi: 10.1002/cbf.1517
50. Wang, M, Zhang, M, Zhang, Y, and Cao, L. Immunostimulatory activity of soybean hull polysaccharide on macrophages. Exp Ther Med. (2022) 23:389. doi: 10.3892/etm.2022.11316
51. Shin, K-S, Seo, H, Lee, SJ, Sung, S, and Hwang, D. Polysaccharide isolated from Korean-style soy sauce activates macrophages via the MAPK and NF-κB pathways. Food Sci Technol. (2021) 41:817–24. doi: 10.1590/fst.06121
52. Hur, HG, Lay, JO Jr, Beger, RD, Freeman, JP, and Rafii, F. Isolation of human intestinal bacteria metabolizing the natural isoflavone glycosides daidzin and genistin. Arch Microbiol. (2000) 174:422–8. doi: 10.1007/s002030000222
53. Setchell, KDR, and Adlercreutz, H. Mammalian lignans and phytoestrogens. Recent Adv Phytochem. (1988) 22:303–46. doi: 10.1016/S0079-9920(08)60268-5
54. Setchell, KDR. Phytoestrogens: the biochemistry, physiology, and implications for human health of soy isoflavones. Am J Clin Nutr. (1998) 68:1333S–46S. doi: 10.1093/ajcn/68.6.1333S
55. Dia, VP, Berhow, MA, and Gonzalez De Mejia, E. Bowman-Birk inhibitor and genistein among soy compounds that synergistically inhibit nitric oxide and prostaglandin E2 pathways in lipopolysaccharide-induced macrophages. J Argic Food Chem. (2008) 56:11707–17. doi: 10.1021/jf802475z
56. Abron, JD, Singh, NP, Price, RL, Nagarkatti, M, Nagarkatti, PS, and Singh, UP. Genistein induces macrophage polarization and systemic cytokine to ameliorate experimental colitis. PLoS One. (2018) 13:e0199631. doi: 10.1371/journal.pone.0199631
57. Yoshiki, Y, Kudou, S, and Okubo, K. Relationship between chemical structures and biological activities of triterpenoid saponins from soybean. Biosci Biotechnol Biochem. (1998) 62:2291–9. doi: 10.1271/bbb.62.2291
58. Kerwin, SM. Soy saponins and the anticancer effects of soybeans and soy-based foods. Curr Med Chem Anticancer Agents. (2004) 4:263–72. doi: 10.2174/1568011043352993
59. Hooper, L, Kroon, PA, Rimm, EB, Cohn, JS, Harvey, I, Le Cornu, KA, et al. Flavonois, flavonoid-rich foods, and cardiovascular risk: a meta-analysis of randomized controlled trials. Am J Clin Nutr. (2008) 88:38–50. doi: 10.1093/ajcn/88.1.38
60. Messina, M, Shearer, G, and Peterson, K. Soybean oil lowers circulating cholesterol levels and coronary heart disease risk, and has no effect on markers of inflammation and oxidation. Nutrition. (2021) 89:111343. doi: 10.1016/j.nut.2021.111343
61. Han, SN, Leka, LS, Lichtenstein, AH, Ausman, LM, Schaefer, EJ, and Meydani, SN. Effect of hydrogenated and saturated, relative to polysatureted, fat on immune and inflammatory responses of adults with moderate hypercholesterolemia. J Lipid Res. (2002) 43:445–52. doi: 10.1016/S0022-2275(20)30151-6
62. Zhu, Y, Feng, X, Guo, J, Wang, L, Guo, X, and Zhu, X. A review of extraction, purification, structural properties and biological activities of legumes polysaccharides. Front Nutr. (2022) 9:1021448. doi: 10.3389/fnut.2022.1021448
63. Ghaseminejad-Raeini, A, Ghaderi, A, Sharafi, A, Nematollahi-Sani, B, Moossavi, M, Derakhshani, A, et al. Immunomodulatory actions of vitamin D in various immune-related disoeders; a comprehensive review. Front Immunol. (2023) 14:950465. doi: 10.3389/fimmu.2023.950465
64. Lee, GY, and Han, SN. The role of vitamin E in immunity. Nutrients. (2018) 10:1614. doi: 10.3390/nu10111614
Keywords: allergy, anti-inflammation, dendritic cells, macrophages, mononuclear phagocytes, phytochemicals, soybeans
Citation: Tezuka H and Imai S (2024) Fine-tuning of mononuclear phagocytes for improved inflammatory responses: role of soybean-derived immunomodulatory compounds. Front. Nutr. 11:1399687. doi: 10.3389/fnut.2024.1399687
Received: 12 March 2024; Accepted: 29 April 2024;
Published: 24 May 2024.
Edited by:
Chiharu Nishiyama, Tokyo University of Science, JapanReviewed by:
Masako Toda, Tohoku University, JapanCopyright © 2024 Tezuka and Imai. This is an open-access article distributed under the terms of the Creative Commons Attribution License (CC BY). The use, distribution or reproduction in other forums is permitted, provided the original author(s) and the copyright owner(s) are credited and that the original publication in this journal is cited, in accordance with accepted academic practice. No use, distribution or reproduction is permitted which does not comply with these terms.
*Correspondence: Hiroyuki Tezuka, dGV6dWthQGZ1aml0YS1odS5hYy5qcA==
†These authors have contributed equally to this work
Disclaimer: All claims expressed in this article are solely those of the authors and do not necessarily represent those of their affiliated organizations, or those of the publisher, the editors and the reviewers. Any product that may be evaluated in this article or claim that may be made by its manufacturer is not guaranteed or endorsed by the publisher.
Research integrity at Frontiers
Learn more about the work of our research integrity team to safeguard the quality of each article we publish.