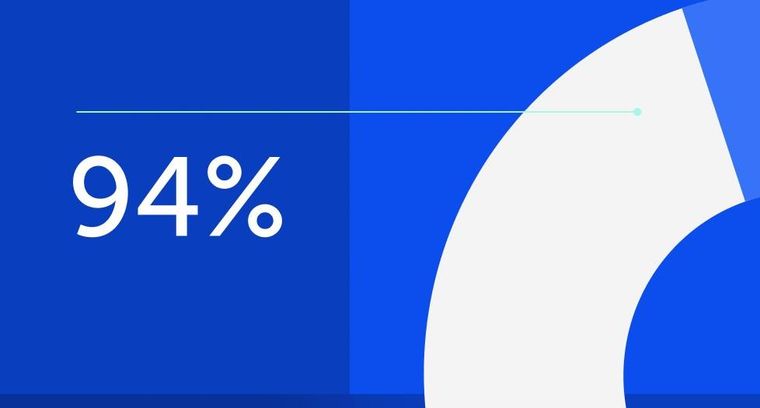
94% of researchers rate our articles as excellent or good
Learn more about the work of our research integrity team to safeguard the quality of each article we publish.
Find out more
REVIEW article
Front. Nutr., 12 June 2024
Sec. Nutrition and Food Science Technology
Volume 11 - 2024 | https://doi.org/10.3389/fnut.2024.1393357
This article is part of the Research TopicWheat: From Nutrition to Cultivation and TechnologyView all 6 articles
Crop yield and quality has increased globally during recent decades due to plant breeding, resulting in improved food security. However, climate change and shifts in human dietary habits and preferences display novel pressure on crop production to deliver enough quantity and quality to secure food for future generations. This review paper describes the current state-of-the-art and presents innovative approaches related to alien introgressions into wheat, focusing on aspects related to quality, functional characteristics, nutritional attributes, and development of novel food products. The benefits and opportunities that the novel and traditional plant breeding methods contribute to using alien germplasm in plant breeding are also discussed. In principle, gene introgressions from rye have been the most widely utilized alien gene source for wheat. Furthermore, the incorporation of novel resistance genes toward diseases and pests have been the most transferred type of genes into the wheat genome. The incorporation of novel resistance genes toward diseases and pests into the wheat genome is important in breeding for increased food security. Alien introgressions to wheat from e.g. rye and Aegilops spp. have also contributed to improved nutritional and functional quality. Recent studies have shown that introgressions to wheat of genes from chromosome 3 in rye have an impact on both yield, nutritional and functional quality, and quality stability during drought treatment, another character of high importance for food security under climate change scenarios. Additionally, the introgression of alien genes into wheat has the potential to improve the nutritional profiles of future food products, by contributing higher minerals levels or lower levels of anti-nutritional compounds into e.g., plant-based products substituting animal-based food alternatives. To conclude, the present review paper highlights great opportunities and shows a few examples of how food security and functional-nutritional quality in traditional and novel wheat products can be improved by the use of genes from alien sources, such as rye and other relatives to wheat. Novel and upcoming plant breeding methods such as genome-wide association studies, gene editing, genomic selection and speed breeding, have the potential to complement traditional technologies to keep pace with climate change and consumer eating habits.
Wheat is one of the three major crops (together with rice and maize) across the globe as related to production (yield and area) and as a staple (1, 2). Of these three crops, wheat has a specific place as a food crop with a wide range of products, and it is also the most traded of the cereals (3). Wheat thereby contributes largely to food security in various areas worldwide, where wheat is an important or major component of the daily food consumption (1, 2). Due to the high intake of wheat, its nutritional content is of particular importance to secure human health and wellbeing (4–10). Traditionally, wheat has been used for a variety of baked goods, such as breads, chapatis, naan, pancakes, wafers, biscuits, crackers, cakes etc., while pasta and more “whole seed” (e.g., breakfast cereals) are also common wheat-based products (11). Currently, novel wheat-based products are emerging, such as wheat berries (whole grain wheat to be cooked and replace, e.g., rice), seitan (gluten used to replace meat), high moisture meat analogs (HMMA, to replace meat and in these products, wheat proteins are used as building blocks), to make pace with emerging consumer and societal needs (12–15).
As for all other crops, climate change, with increased spells of extreme weather, has a large impact on the production of wheat, affecting both yield and quality (16, 17). Plant breeding has resulted in wheat material adapted to the current prevailing cultivation conditions, which will be altered due to the predicted climate change, eventually resulting in a dramatic decrease in yield (18). The focus of plant breeding has been to increase yield, resistance and end-use quality, saving lines with the best performance in the present climate, which also might have affected the genetic diversity in crops (19–22). Furthermore, plant breeding of wheat might have contributed to a decrease in the nutritional quality, e.g., minerals and phytochemicals, of the wheat, and breeding toward improved bread-making quality may have resulted in an increase in components having a negative effect on human health (allergy, coeliac disease, non-coeliac gluten sensitivity, irritable bowel syndrome) (23). However, recent studies (24, 25) have found no evidence of a lower quality of modern wheat for human health as compared to ancient wheat genotypes. Instead, some studies have indicated the modern processing methods of wheat as a risk factor of their health impact (26). Despite this, the incorporation into adapted wheat of novel genes that contribute yield and quality under climate change conditions, is extremely important (8). Genes can be incorporated into adapted wheat from various sources, including the primary (species easily crossable with wheat), secondary (related species within the same genus), tertiary (wild relatives) gene pools.
The present review paper aims to increase the understanding on how alien (wild relatives of wheat; i.e., from the tertiary gene pool) genes have the potential to contribute to increased food security as well as to improved technological and nutritional quality for traditional and novel wheat products by the use of an increasingly wide array of emerging plant breeding methods. Thus, the paper starts with a description of the current status and needs for improvements, then follows a review of opportunities with the use of alien germplasm in wheat breeding, impact on food security, quality aspects and requirements for novel products, finalizing with an overview of benefits of traditional and novel methodologies for the transfer of alien genes into adapted wheat genotypes in relation to the demands described in the other sections. The conclusion section in the end summarizes the outcomes of the present review.
Wheat contributes one-fifth of the global food calories and protein to the human population and is therefore, extremely important for global food security (1, 2). Wheat is the main staple crop in temperate zones and is thereby produced in a range of areas across the globe, with major producing areas in Europe, East-, West-, Central- and South- parts of Asia, East-, Central- and North- parts of the USA, South part of Canada, South-Central part of South America and mainly in Ethiopia and South Africa in the African continent. The highest yield (4-5 tons per Ha) is found in Europe and East Asia. Almost 30% of the wheat produced comes from low- and middle-income countries with production units of 1-3 Ha, where 80% of the production is used for food (1). Wheat is also the most traded cereal crop (3). These numbers show that wheat is extremely important for food security, feeding a large part of the human population, traded to meet requirements and needs in many areas, and also an important part for poor and small-scale farmers to supply their daily food intake.
Due to the high intake of wheat, its nutritional content has a high impact on human nutrition. Wheat is known as an important source of a number of health-related components such as protein, vitamins, dietary fiber and phytochemicals (8, 27). As an example, wheat is known to contribute 20% of the daily dietary fiber in the UK, thereby providing protection against cardio-vascular diseases, type 2 diabetes and colorectal cancer (28). Depending on genotypic differences, 1 L of wheat flour is also known to provide 100% of the daily required intake of tocopherols, carotenoids and phenolic compounds (9). Wheat genotypes with high levels of minerals, such as iron and zinc have also been reported (29, 30). The increased consumption of wheat in urban areas, together with an increase in unhealthy dietary patterns by part of the consumers in the Western world (31), calls for an increase in health-promoting components in wheat, one of the major staples worldwide. Furthermore, the predicted climate change will have an impact on both production opportunities and the quality of the wheat produced (32–34), indicating the need for the development of high-yielding and health-promoting wheat genotypes to be grown under new circumstances. Currently, we also see a change in the number of food products developed from wheat, where more traditional ones, such as bread, still have a large share in the market, while novel products, such as vegan and meat analog alternatives, are gaining shares (35, 36). Thus, future wheat genotypes need to fulfill requirements in all these aspects.
Plant breeding is used as a tool to improve the yield, resistance and quality of crops (16). With the current climate change predictions, there is increasing requirements for genes with the ability to contribute to high performance independent of climate-related stresses and diseases (37). Thus, genes from alien germplasm might be an untapped resource of genetic diversity in this novel plant breeding landscape (38). The novel and emerging techniques within plant breeding, such as speed breeding, genomic and genetic tools, phenotyping and genotyping, together with improved opportunities to handle big data, also contribute to the increased opportunities to tap and use alien germplasm in breeding programs (5). Thereby, rare and valuable gene combinations can be searched for in alien wheat lines, and modern plant breeding methods should then be used for their transfer to adapted wheat (39).
The alien resource most commonly used in wheat breeding is rye, from which a number of genes have been transferred (40), while the most commonly utilized Aegilops species is A. tauschii (41). The transfer of genes from the rye gene pool has resulted in a great increase of the yield potential in wheat (42, 43). Besides the widely explored rye genome, a range of other alien sources have been explored for their opportunities to contribute genes for wheat improvement (40). Thus, Goatgrass (Aegilops spp.), i.e., the donor of the D genome to common wheat, has been used to widen the genetic diversity of wheat (44); tall wheatgrass (Agropyron elongatum) has been used in wheat breeding through distant hybridization (45); A. caudata (46), Thinopyron bessarabicum (47), Amblyopyrum muticum (48), Triticum timopheevii (49), and T. urartu (50) are additional alien species evaluated and found to carry potential interesting characters for introgressions into the wheat genome.
The predicted climate change is expected to have a large impact on food security for the global human population (18, 51). A global temperature increase is part of the prediction, which will result in fewer opportunities to grow crops in areas that are already hot and dry (52), while cold areas might be increasingly beneficial for crop production. However, such a change in cultivation environments will require adaptation of plants to daylight changes and other environmental (e.g., soil) differences between current and future cultivation areas (53). Furthermore, the predicted climate change is expected to result in an increased number of events with extreme weather events (54). As a result, heat, drought and flooding will become more common, and the timing of these events might be more unpredictable (54). Such events of extreme abiotic stress will potentially induce massive yield loss in wheat and other crops (18, 55–57), and especially drought is known as a major threat to food security due to its severe impact on wheat yield (55–57). Thus, for increased food security, alien genetic materials should be used in wheat breeding to fortify tolerance to abiotic stress (32) and to increase disease resistance (38, 58, 59), thereby securing high yield (Table 1).
Table 1. Examples of the utilization of alien germplasm to improve (a) quality and yield related traits and (b) resistance traits
Modern wheat usually demonstrates a high yield potential and performance, although, incorporation of alien chromosomes in the wheat genome, i.e., from rye, has in previous studies been shown to increase the yield potential of wheat (42, 43). Thus, 1R and 1RS translocation lines were found with a higher yield than non-translocated lines (43, 88), and 1RS has been reported to contribute to a lower yield reduction compared to non-translocation wheat under drought conditions (89). Furthermore, 1R and 1RS have been found to improve root length and biomass in wheat, benefiting better accessibility to water in deep layers when the surface soil dries up (32, 85, 90). In general, root traits are known to play a key role in drought tolerance (91, 92). Furthermore, chromosome 3R has recently been shown to contribute to drought tolerance in wheat at vegetative growth stages under climate chamber conditions (32).
In addition to the widely explored rye genome, there are other alien species exploited to enhance wheat resilience for dynamic climates, such as the D genome donor goatgrass (Aegilops spp.) (44). Several wheat-aegilops lines have been reported to hold increased drought (67, 93) and heat tolerance (94) as well as with improved nutrient use efficiency (95). A. tauschii have in several studies been found to contribute yield related traits when introgressed into wheat (68, 69). Furthermore, tall wheatgrass (Agropyron elongatum) is a perennial crop known to withstand different abiotic stresses. Several wheat cultivars developed through distant hybridization with tall wheatgrass showed increased tolerance to heat, strong light, and hot-dry wind (45). The introgression of the Lr19 gene from tall wheatgrass has been reported to be responsible for a significant yield increase in wheat (73). A summary of the incorporations of various alien chromosomes into wheat and their effect on wheat improvement is shown in Table 1.
Quality is a concept describing how good or bad something is, and for wheat it attributes to (i) the end-use quality, (ii) health and wellbeing through consumption, and (iii) how the wheat is produced (96). The present review is focusing on the functional and nutritional aspects, where the functionality is directly related to the end-use quality, while the nutritional aspects are directly connected to health and wellbeing. A few of these characters are encoded by major genes (as described below), but the majority are encoded by quantitative traits loci (QTL) (38). In general, the use of alien introgressions for transfer of improvements of a trait will be more complicated when the trait is determined by QTL instead of major genes. Previous work has shown the success of the transfer of resistance genes encoded by a single major gene, into an adapted genetic background [(e.g., 39)]. The fact that introgression of single major genes are easier and more common are depicted in Table 1, showing examples of disease resistance genes transferred as major genes, while introgressions effecting quality is often the result of QTL and impact on several traits.
End-use quality requirements of wheat differs, as wheat is consumed in various forms around the world, e.g., as bread, pasta, noodles, biscuits and pastries, and type of product and processing conditions is largely affecting the functional aspects (16, 97). Several characteristics, such as grain size and hardness, hue, and, to a large extent, protein content and composition, especially of the gluten proteins, determine the functional quality of wheat (8, 98). The gluten proteins, i.e., the glutenins and gliadins, accounting for 80% of the grain proteins in the mature wheat seed, have a significant impact on the bread-making quality as they confer the viscoelastic properties (99, 100). The gluten proteins have been largely studied, they have been found encoded by major genes and the encoding genes have been determined and designated (101). However, the ability of the gluten proteins to polymerize, in the wheat grain and during processing, has also been found a major determinant of the functionality (97) and this character is most likely determined by QTL as it correlates with weather aspects and developmental stages of the wheat plant (102). Several studies have reported that baking and bread-making qualities of wheat flour is affected by the introgression of rye chromosomes into wheat. The most well-known transfer is the 1BL/1RS translocation, known to contribute a yield increase in wheat but also sticky dough performance due to the exchange of some high molecular weight (HMW)-glutenin subunits (GS) encoded on the 1B wheat chromosome to some secalins encoded on the 1R rye chromosome (103). Thus, to retain the functional quality of wheat, introgressions that includes the deletion of major gluten protein genes should be avoided. However, recent studies have shown improved functionality when 1BL/1RS translocation lines where used and gluten protein genes were restored in these (65). Additionally, introgressions from the 3R chromosome has been shown to contribute positively to baking quality stability across various drought treatments (33). Major genes with positive effects on functional quality have been transferred to wheat from other species than rye, e.g., Aegilops sharonensis (82), T. timopheevii (84), and Aegilops triuncialis (104). Additionally, introgressions from Aegilops spp., Agropyron spp., Thinopyrum spp., and Leymus spp., contributing major genes or quantitative traits loci (QTL), have been found to contribute positive effects to the functional properties of wheat (38, 105–108).
Nutritional quality of wheat encompasses the content of a range of different elements including protein, micronutrients such as iron (Fe) and zinc (Zn), as well as various vitamins and phytochemicals (8, 9). Micronutrient deficiency (hidden hunger), especially of Fe and Zn, is a major challenge for more than 3 billion people, with women and children mostly affected (7, 109–111). This problem is present in all countries, but with the highest share in developing countries, where the daily diets for most people are cereal crops, often without any additions (111–113). In developed countries nutrient deficiency is instead often related to the intake of a diet high in sugar and fat and low in essential nutrients (114). Considering economics and sustainability, one of the best strategies to address food nutrient deficiency is through biofortification, i.e., increase of nutrient density in food crops (115–118). As for nutritional characters, much less is known than for the gluten proteins in relation to encoding genes and several of them are encoded by QTL and not by major genes. Alien germplasm has been found to harbor a wealth of essential genes associated with levels of these elements (5, 79, 109, 110, 119). The grain protein content (GPC) is known determined by QTL in wheat and also influenced by e.g., starch accumulation in the grain and stressors (abiotic and biotic) during the grain accumulation (8). However, wild wheat relatives have been identified with high GPC values (120, 121). Furthermore, major genes, e.g., NAM-B1 and Gpc-2, have been identified and introgressed into wheat from T. dicoccoides (122), and T. timopheevii (84), respectively. Also, through the development of synthetic lines, QTL was transferred from A. tauschii which contributed increased GPC (105). Elevated Fe and Zn concentrations have been reported in wheat grains with introgressions of 1R, 2R, 3R and 5R (34, 38). Also, in some wheat genotypes bred through hybridization between the wheat landrace Chinese Spring and Aegilops kotschyi a significant increase in grain Fe and Zn concentration were reported as a result of the presence of the A. kotschyi chromosomes 2S and 7U (77). Similarly, grain Fe and Zn concentration enhancements have been reported in wheat lines carrying genes from chromosome groups 4 and 7 of A. peregrina (78). A recent mega QTL analysis (123) have, correspondingly to previous results using recombinant inbred lines [RILs; (124)], identified candidate areas from T. turgidum ssp. dicoccoides, with genes positively impacting quality traits such as mineral content and abiotic stress tolerance.
Alien germplasms are also reported to have elevated levels of phenolic compounds, beta-glucans and vitamins, compounds that have been reported to have positive effects on human health (80, 107). Dietary fiber, which is an important food component that enhances digestion and regular movement of the bowl has significant variability in alien germplasms that can be exploited and introduced into elite cultivars (75). Similarly, alien material with a high content of bioactive compounds can be selected and used in breeding to increase the nutritional value of wheat (125).
Wheat, with its gluten protein (glutenins and gliadins), holds certain quality attributes, which makes it outstanding as a food ingredient and quality contributor. The wheat gluten can easily be texturized e.g., by the use of high moisture extrusion processing (≥40%), resulting in elastic, tough and uniform textures of significance for a variety of food products with different flavors (126). Twin-screw extrusion is commonly used in the process to create novel products such as palatable meat analogs, of value as replacers of animal-based products in the recent food market (127). These products are especially demanded by flexitarians, i.e., consumers who wants to reduce their meat consumption due to environmental or health concerns (128). These consumers often do not want to give up the special taste and texture of meat products. Thus, proteins that are fibrous and highly ordered in elongated structures, along with juiciness, tenderness and attractive flavor, are characters needed in these products (129, 130), as well as a desired nutrient profile. The importance of the fibrous structure formation of plant proteins in meat analogs and their contribution to functionality on different length scales has been highlighted in several studies (131–135) and is summarized in Figure 1. Also, amino acid composition with a high content of essential amino acids for human consumption and a high degree of protein digestibility as well as of other health-promoting components, are of great importance in these products (136).
Figure 1. Plant protein fibrous structures at different scales and their suitability for meat substitutes.
In addition to wheat, a range of different plant proteins, including soy, pea, peanut and less explored rapeseed (137), rice (138), oat (139), and a few legume crops (mung bean, lupin, faba bean, and chick pea) (126), have been evaluated and are used as a component in texturized meat analogs products. In such products, the matrix is primarily formed through denaturation, aggregation, cross-linking and phase-separation of proteins, and until now, soy, pea and wheat gluten are the most commonly used plant sources (140). The gluten proteins contribute specific and unique qualities, as they have the ability to form network and cross-links on a larger scale than any other plant protein (141). Thus, the gluten proteins of wheat have a special role in the meat analogs as a matrix and structural builder (142). Other sources, such as the legume proteins, or other less explored protein sources such as algal- and fungal-based ingredients (127), and edible insect powder (143) should then be added to contribute nutritional aspects (136).
Alien germplasm has the potential to contribute a range of quality attributes of interest for the production of meat analogs, including improved structural properties of the proteins, increased content of essential amino acids in wheat, increased content of minerals and nutritional elements in wheat, and improved taste performance of the products. Previous studies have indicated a high gluten strength in some alien wheat introgression lines with Leymus spp. and 3R (33, 38). Gluten strength is the most important quality criteria for baking and is measured by a range of instruments, among others, by the alveograph (144). Furthermore, multiple introgressions of alien segments into cultivated wheat from Thinopyrum ponticum, Aegilops longissima and Triticum aestivum were found to improve gluten quality characteristics and semolina yellow index in dual-purpose durum wheat (145). As described above, high levels of Fe and Zn and other nutritional components have also been reported in alien germplasm, which could be beneficial in relation to the production of meat analog, to replace the intake of such elements from meat products (34).
Alien genes have been transferred into wheat during the 20th century, with several positive outcomes, especially in the area of disease resistance genes (146). Traditionally, such a transfer has included a range of different techniques used, where the inclusion of crosses utilizing genetic material lacking the Ph1 gene has been an essential part (147). The Ph1 gene is a key regulator of chromosome pairing during meiosis in wheat, preventing recombination between homoeologous chromosomes, which results in that wheat behaves like a diploid during meiosis despite the crop being a hexaploid (148). Mutations induced in Ph1 (ph1b) allele by radiation allowed increased recombination between the homoeologous chromosomes of wheat and rye (149). Another way of inducing recombination has been the use of nullisomic lines, lacking the chromosome containing the Ph1 locus (150). However, independent of the methodology used, the procedure has been rather tedious and unprecise, and because of that, the number of commercial varieties containing alien chromosome fragments is rather limited (40). Additionally, the transfer of alien genes into adapted wheat often also results in linkage drag, i.e., the genetic transfer includes a larger piece of the alien chromosome than only the desired gene of interest (151). One of the most well-known examples of such a transfer is the 1BL/1RS translocation that resulted in the variety Petkus, which contributed higher yield and tolerance to abiotic stresses but simultaneously reduced the gluten strength and gave sticky doughs (152, 153). Another example is the transfer of the stem rust resistance gene Sr22 from T. boeoticum to wheat which caused a yield penalty and additional efforts were needed to reduce the linkage drag (152).
However, novel techniques such as whole genome sequencing and platforms with genetic data, as well as high-throughput phenotyping methodologies and large data set handling opportunities and speed breeding techniques have resulted in novel opportunities for a quicker and more specific transfer of alien genes into adapted wheat lines. Thus, recent advances show good opportunities for quick and precise transfer of alien genes using, e.g., genotyping-by-sequencing both for determination of the gene of interest (153), for the development of competitive allele-specific PCR (KASP) markers (154, 155) and for the selection of adapted wheat material containing the transferred gene (39, 156). Thus, the use of these novel technologies will most likely result in increased use of alien germplasm to transfer genes of interest both as related to quality traits (end-use, structure, nutrition) and resistance and yield, thereby targeting food security.
Additionally, precision genome editing, using the CRISPR-Cas9A technique is rapidly becoming popular. In wheat, it has been used to improve drought tolerance (157) and herbicide tolerance (158). As the acceptance of the use of CRISPR-Cas9 increases, it can become the method of choice for mirror alien genes of interest to have in wheat. CRISPR-mediated gene editing can facilitate the replacement of endogenous alleles with desired alien alleles carrying beneficial traits. By introducing precise mutations or sequence modifications using CRISPR, it can be possible to replace target gene variants in the crop genome with those from wild or related species, thereby introducing novel traits or improving agronomic performance. CRISPR systems can be multiplexed to simultaneously target multiple genomic loci, allowing for the introgression of multiple alien genes or alleles in a single transformation event. This enables the rapid introgression of complex trait combinations from wild or exotic germplasm into elite crop varieties, accelerating the breeding process. But to succeed with it, deeper knowledge of the functioning of causative alien genes is needed, followed by introducing similar mutations in the corresponding wheat alleles. CRISPR-Cas9 was used in a study that targeted α-gliadin genes to produce low gluten containing wheat lines with reduced immune reactivity. Upto 35 different genes were mutated in one of the lines and immunoreactivity was reduced by 85% (159). Thus, precision genome editing can become a promising alternative to homoeologous recombination between alien and wheat chromatin. With developments in next generation sequencing and comparative genomics key genes can be identified in the alien genomes to later on mirror those in the wheat genome.
The predicted climate change calls for more resilient, stable and robust crops, which is important not least as related to the staple crops such as wheat, feeding a large proportion of the human population. Plant breeding has resulted in a large increase in yield, resistance toward diseases and pests, and quality in crops currently under cultivation, although it has also narrowed the genetic base. Widening of the gene pool in the cultivated crops and the introduction of new genes that allows future cultivation of high yielding and stable cultivars is becoming more important than ever with the growing world population and changes in cultivation environments. Here, alien resources, holding genes contributing to novel resistances toward diseases and pests, tolerances to e.g., heat, drought and flooding, and qualities are becoming increasingly important. Alien genetic material have been shown to have potential to contribute genes targeting improved functional and nutritional qualities in wheat, as summarized in Figure 1. Furthermore, stability and resilience to various climatic conditions have been demonstrated in wheat material containing alien chromosome fragments. Novel eating habits and the transition toward a replacement of animal-based products with plant-based alternatives paves the way for unique quality desires of the plants for these products. The wheat proteins are unique, with the ability to form large polymers through cross-linking of the proteins. Thus, the wheat proteins are needed to form the matrices in many of these novel products. The incorporation of alien genes in wheat may contribute certain qualities of relevance for these new products, both in terms of functionality, e.g., matrices that resembles more meat, and nutrition, e.g., higher mineral levels or lower levels of phytases. Novel plant breeding methods, including whole genome sequencing, platforms with genetic data, high-throughput phenotyping, large data set handling and speed breeding techniques, but also emerging CRISPR techniques, contribute opportunities to use alien resources in wheat breeding in ways that are more precise and with a higher speed (Figure 2).
EJ: Conceptualization, Data curation, Formal analysis, Funding acquisition, Investigation, Methodology, Project administration, Resources, Software, Supervision, Validation, Visualization, Writing – original draft, Writing – review & editing. YL: Conceptualization, Formal analysis, Investigation, Validation, Visualization, Writing – original draft, Writing – review & editing. OO: Writing – original draft, Formal analysis, Writing – review & editing, Visualization, Investigation, Conceptualization. RK: Conceptualization, Project administration, Supervision, Writing – original draft, Funding acquisition, Investigation, Writing – review & editing, Resources. AC: Writing – original draft, Investigation, Writing – review & editing, Supervision, Conceptualization. MR: Investigation, Conceptualization, Writing – review & editing, Writing – original draft, Supervision.
The author(s) declare that financial support was received for the research, authorship, and/or publication of this article. This research was funded by the Trees and Crops for the Future (TC4F) and SLU Grogrund.
The authors declare that the research was conducted in the absence of any commercial or financial relationships that could be construed as a potential conflict of interest.
All claims expressed in this article are solely those of the authors and do not necessarily represent those of their affiliated organizations, or those of the publisher, the editors and the reviewers. Any product that may be evaluated in this article, or claim that may be made by its manufacturer, is not guaranteed or endorsed by the publisher.
1. Erenstein, O, Jaleta, M, Mottaleb, KA, Sonder, K, Donovan, J, and Braun, HJ. Global trends in wheat production, consumption and trade In: Wheat improvement: food security in a changing climate. Cham: Springer International Publishing (2022). 47–66.
2. Shiferaw, B, Smale, M, Braun, HJ, Duveiller, E, Reynolds, M, and Muricho, G. Crops that feed the world 10. Past successes and future challenges to the role played by wheat in global food security. Food Security. (2013) 5:291–317. doi: 10.1007/s12571-013-0263-y
3. Trading Economics (2023). Wheat - 2023 data - 1977-2022 historical - 2024 forecast - price - quote – chart. Available at: tradingeconomics.com (Accessed September 23, 2023).
4. Alomari, DZ, Schierenbeck, M, Alqudah, AM, Alqahtani, MD, Wagner, S, Rolletschek, H, et al. Wheat grains as a sustainable source of protein for health. Nutrients. (2023) 15:4398. doi: 10.3390/nu15204398
5. Gadaleta, A, Marcotuli, I, Arriagada, O, Johansson, E, Rahmatov, M, Ceresino, E, et al. Chapter 1 - Use of genetic resources and prebreeding activities in order to improve nutritional and health-related properties of cereals and pseudocereals In: M Rakszegi, M Papageorgiou, and JM Rocha, editors. Developing sustainable and health promoting cereals and pseudocereals : Academic Press (2023). 5–24.
6. Govindan, V, Singh, RP, and Ibba, MI. “Wheat Biofortification and Mainstreaming Grain Zinc and Iron in CIMMYT Wheat Germplasm,” in Wheat Science. eds. OP Gupta, S Kumar, A Pandey, MK Khan, SK Singh, and GP Singh, et al. (United Kingdom: Taylor & Francis). CRC Press. (2023). 421–427.
7. Gupta, PK, Balyan, HS, Sharma, S, and Kumar, R. Biofortification and bioavailability of Zn, Fe and Se in wheat: present status and future prospects. Theor Appl Gene. (2021) 134:1–35. doi: 10.1007/s00122-020-03709-7
8. Johansson, E, Branlard, G, Cuniberti, M, Flagella, Z, Hüsken, A, Nurit, E, et al. Genotypic and environmental effects on wheat technological and nutritional quality In: Wheat quality for improving processing and human health (2020). 171–204.
9. Johansson, E, Hussain, A, Kuktaite, R, Andersson, SC, and Olsson, ME. Contribution of organically grown crops to human health. Int J Environ Res Public Health. (2014) 11:3870–93. doi: 10.3390/ijerph110403870
10. Kartseva, T, Alqudah, AM, Aleksandrov, V, Alomari, DZ, Doneva, D, Arif, MAR, et al. Nutritional genomic approach for improving grain protein content in wheat. Foods. (2023) 12:1399. doi: 10.3390/foods12071399
11. Arendt, EK, and Zannini, E. Cereal grains for the food and beverage industries. Sawston, Cambridge: Woodhead publishing series, Elsevier (2013).
12. Anwar, D, and Ghadir, EC. Nutritional quality, amino acid profiles, protein digestibility corrected amino acid scores and antioxidant properties of fried tofu and seitan. Food Environ Saf J. (2019) 18:176–190.
13. Filieri, R. Consumer co-creation and new product development: a case study in the food industry. Mark Intell Plann. (2013) 31:40–53. doi: 10.1108/02634501311292911
14. Fu, J, Sun, C, Chang, Y, Li, S, Zhang, Y, and Fang, Y. Structure analysis and quality evaluation of plant-based meat analogs. J Text Stud. (2023) 54:383–93. doi: 10.1111/jtxs.12705
15. Speck, M. Ancient grains for modern meals: mediterranean whole grain recipes for Barley, farro, kamut, polenta, wheat berries & more [a cookbook]. Berkeley, Canada: Ten Speed Press (2011).
16. Johansson, E, Muneer, F, and Prade, T. Plant breeding to mitigate climate change—present status and opportunities with an assessment of winter wheat cultivation in Northern Europe as an example. Sustainability. (2023) 15:12349. doi: 10.3390/su151612349
17. Thornton, PK, Ericksen, PJ, Herrero, M, and Challinor, AJ. Climate variability and vulnerability to climate change: a review. Glob Change Biol. (2014) 20:3313–28. doi: 10.1111/gcb.12581
18. Zhang, T, He, Y, DePauw, R, Jin, Z, Garvin, D, Yue, X, et al. Climate change may outpace current wheat breeding yield improvements in North America. Nat Commun. (2022) 13:5591. doi: 10.1038/s41467-022-33265-1
19. Kahiluoto, H, Kaseva, J, Balek, J, Olesen, JE, Ruiz-Ramos, M, Gobin, A, et al. Decline in climate resilience of European wheat. Proc Natl Acad Sci USA. (2019) 116:123–8. doi: 10.1073/pnas.1804387115
20. Rauf, S, da Silva, JT, Khan, AA, and Naveed, A. Consequences of plant breeding on genetic diversity. Int J Plant Breed. (2010) 4:1–21.
21. Reif, JC, Zhang, P, Dreisigacker, S, Warburton, ML, van Ginkel, M, Hoisington, D, et al. Wheat genetic diversity trends during domestication and breeding. Theor Appl Genet. (2005) 110:859–64. doi: 10.1007/s00122-004-1881-8
22. Swarup, S, Cargill, EJ, Crosby, K, Flagel, L, Kniskern, J, and Glenn, KC. Genetic diversity is indispensable for plant breeding to improve crops. Crop Sci. (2021) 61:839–52. doi: 10.1002/csc2.20377
23. Morris, CE, and Sands, DC. The breeder's dilemma—yield or nutrition? Nature Biotechnology. (2006) 24:1078–80. doi: 10.1038/nbt0906-1078
24. Brouns, F, Geisslitz, S, Guzman, C, Ikeda, TM, Arzani, A, Latella, G, et al. Do ancient wheats contain less gluten than modern bread wheat, in favour of better health? Nutr Bull. (2022) 47:157–67. doi: 10.1111/nbu.12551
25. Shewry, PR, Hassall, KL, Grausgruber, H, Andersson, AM, Lampi, AM, Piironen, V, et al. Do modern types of wheat have lower quality for human health? Nut Bull. (2020) 45:362–73. doi: 10.1111/nbu.12461
26. Cabanillas, B. Gluten-related disorders: Celiac disease, wheat allergy, and nonceliac gluten sensitivity. Crit Rev Food Sci Nutr. (2020) 60:2606–21. doi: 10.1080/10408398.2019.1651689
27. Shewry, PR, Piironen, V, Lampi, AM, Edelmann, M, Kariluoto, S, Nurmi, T, et al. The HEALTHGRAIN wheat diversity screen: effects of genotype and environment on phytochemicals and dietary fiber components. J Agric Food Chem. (2010) 58:9291–8. doi: 10.1021/jf100039b
28. Shewry, PR, and Hey, SJ. The contribution of wheat to human diet and health. Food Energy Secur. (2015) 4:178–202. doi: 10.1002/fes3.64
29. Hussain, A, Larsson, H, Kuktaite, R, and Johansson, E. Mineral composition of organically grown wheat genotypes: contribution to daily minerals intake. Int J Environ Res Publ Health. (2010) 7:3442–56. doi: 10.3390/ijerph7093442
30. Moreira-Ascarrunz, SD, Larsson, H, Prieto-Linde, ML, and Johansson, E. Mineral nutritional yield and nutrient density of locally adapted wheat genotypes under organic production. Foods. (2016) 5:89. doi: 10.3390/foods5040089
31. Booth, A, Barnes, A, Laar, A, Akparibo, R, Graham, F, Bash, K, et al. Policy action within urban African food systems to promote healthy food consumption: a realist synthesis in Ghana and Kenya. Int J Health Policy Manag. (2021) 10, 10:828–44. doi: 10.34172/IJHPM.2020.255
32. Lan, Y, Chawade, A, Kuktaite, R, and Johansson, E. Climate change impact on wheat performance-effects on vigour, plant traits and yield from early and late drought stress in diverse lines. Int J Mol Sci. (2022) 23:3333. doi: 10.3390/ijms23063333
33. Lan, Y, Kuktaite, R, Chawade, A, and Johansson, E. Diverse wheat lines-to mitigate the effect of drought on end-use quality. Front Food Sci Technol. (2023) 3:1163412. doi: 10.3389/frfst.2023.1163412
34. Lan, Y, Kuktaite, R, Chawade, A, and Johansson, E. Chasing high and stable wheat grain mineral content: mining diverse spring genotypes under induced drought stress. PLoS One. (2024) 19:e0298350. doi: 10.1371/journal.pone.0298350
35. Belderok, B, Mesdag, J, and Donner, DA. Bread-making quality of wheat: a century of breeding in Europe. Berlin, Germany: Springer Science & Business Media (2000).
36. Quintieri, L, Nitride, C, De Angelis, E, Lamonaca, A, Pilolli, R, Russo, F, et al. Alternative protein sources and novel foods: benefits, food applications and safety issues. Nutrients. (2023) 15:1509. doi: 10.3390/nu15061509
37. Chapman, SC, Chakraborty, S, Dreccer, MF, and Howden, SM. Plant adaptation to climate change—opportunities and priorities in breeding. Crop Pasture Sci. (2012) 63:251–68. doi: 10.1071/CP11303
38. Johansson, E, Henriksson, T, Prieto-Linde, ML, Andersson, S, Ashraf, R, and Rahmatov, M. Diverse wheat-alien introgression lines as a basis for durable resistance and quality characteristics in bread wheat. Front Plant Sci. (2020) 11:1067. doi: 10.3389/fpls.2020.01067
39. Yazdani, M, Rouse, MN, Steffenson, BJ, Bajgain, P, Patpour, M, Johansson, E, et al. Developing adapted wheat lines with broad-spectrum resistance to stem rust: Introgression of Sr59 through backcrossing and selections based on genotyping-by-sequencing data. PLoS One. (2023) 18:e0292724. doi: 10.1371/journal.pone.0292724
40. Molnár-Láng, M, Ceoloni, C, and Doležel, J. Alien introgression in wheat. Cham: Springer International Publishing (2015).
41. Kishii, M. An update of recent use of aegilops species in wheat breeding. Front Plant Sci. (2019) 10:452618. doi: 10.3389/fpls.2019.00585
42. Kim, W, Johnson, JW, Baenziger, PS, Lukaszewski, AJ, and Gaines, CS. Agronomic effect of wheat-rye translocation carrying rye chromatin (1R) from different sources. Crop Sci. (2004) 44:1254–8. doi: 10.2135/cropsci2004.1254
43. Ren, T, Ren, Z, Yang, M, Yan, B, Tan, F, Fu, S, et al. Novel source of 1RS from Baili rye conferred high resistance to diseases and enhanced yield traits to common wheat. Mol Breed. (2018) 38:1–9. doi: 10.1007/s11032-018-0856-4
44. Schneider, A, Molnár, I, and Molnár-Láng, M. Utilisation of Aegilops (goatgrass) species to widen the genetic diversity of cultivated wheat. Euphytica. (2008) 163:1–19. doi: 10.1007/s10681-007-9624-y
45. Li, Z, Li, B, and Tong, Y. The contribution of distant hybridization with decaploid Agropyron elongatum to wheat improvement in China. J Genet Genom. (2008) 35:451–6. doi: 10.1016/S1673-8527(08)60062-4
46. Grewal, S, Othmeni, M, Walker, J, Hubbart-Edwards, S, Yang, CY, Scholefield, D, et al. Development of wheat-Aegilops caudata introgression lines and their characterization using genome-specific KASP markers. Front Plant Sci. (2020) 11:539861. doi: 10.3389/fpls.2020.00606
47. Grewal, S, Yang, C, Edwards, SH, Scholefield, D, Ashling, S, Burridge, AJ, et al. Characterisation of Thinopyrum bessarabicum chromosomes through genome-wide introgressions into wheat. Theor Appl Genet. (2018) 131:389–406. doi: 10.1007/s00122-017-3009-y
48. Grewal, S, Coombes, B, Joynson, R, Hall, A, Fellers, J, Yang, CY, et al. Chromosome-specific KASP markers for detecting Amblyopyrum muticum segments in wheat introgression lines. Plant Genome. (2022) 15:e20193. doi: 10.1002/tpg2.20193
49. King, J, Grewal, S, Othmeni, M, Coombes, B, Yang, CY, Walter, N, et al. Introgression of the Triticum timopheevii genome into wheat detected by chromosome-specific kompetitive allele specific PCR markers. Front Plant Sci. (2022) 13:919519. doi: 10.3389/fpls.2022.919519
50. Grewal, S, Guwela, V, Newell, C, Yang, CY, Ashling, S, Scholefield, D, et al. Generation of doubled haploid wheat-Triticum urartu introgression lines and their characterisation using chromosome-specific KASP markers. Front Plant Sci. (2021) 12:643636. doi: 10.3389/fpls.2021.643636
51. Gregory, PJ, Ingram, JS, and Brklacich, M. Climate change and food security. Philos Trans R Soc B Biol Sci. (2005) 360:2139–48. doi: 10.1098/rstb.2005.1745
52. Hatfield, JL, Boote, KJ, Kimball, BA, Ziska, LH, Izaurralde, RC, Ort, D, et al. Climate impacts on agriculture: implications for crop production. Agron J. (2011) 103:351–70. doi: 10.2134/agronj2010.0303
53. Olesen, JE, Trnka, M, Kersebaum, KC, Skjelvåg, AO, Seguin, B, Peltonen-Sainio, P, et al. Impacts and adaptation of European crop production systems to climate change. Eur J Agron. (2011) 34:96–112. doi: 10.1016/j.eja.2010.11.003
54. Planton, S, Déqué, M, Chauvin, F, and Terray, L. Expected impacts of climate change on extreme climate events. Comptes Rendus Geosci. (2008) 340:564–74. doi: 10.1016/j.crte.2008.07.009
55. Fischer, R, and Maurer, R. Drought resistance in spring wheat cultivars. I. Grain yield responses. Austr J Agric Res. (1978) 29:897–912. doi: 10.1071/AR9780897
56. Giunta, F, Motzo, R, and Deidda, M. Effect of drought on yield and yield components of durum wheat and triticale in a Mediterranean environment. Field Crops Res. (1993) 33:399–409. doi: 10.1016/0378-4290(93)90161-F
57. Senapati, N, Stratonovitch, P, Paul, MJ, and Semenov, MA. Drought tolerance during reproductive development is important for increasing wheat yield potential under climate change in Europe. J Exp Bot. (2019) 70:2549–60. doi: 10.1093/jxb/ery226
58. Purnhauser, L, Bóna, L, and Láng, L. Occurrence of 1BL. 1RS wheat-rye chromosome translocation and of Sr36/Pm6 resistance gene cluster in wheat cultivars registered in Hungary. Euphytica. (2011) 179:287–95. doi: 10.1007/s10681-010-0312-y
59. Rahmatov, M, Rouse, MN, Nirmala, J, Danilova, T, Friebe, B, Steffenson, BJ, et al. A new 2DS· 2RL Robertsonian translocation transfers stem rust resistance gene Sr59 into wheat. Theor Appl Genet. (2016) 129:1383–92. doi: 10.1007/s00122-016-2710-6
60. Ehdaie, B, Whitkus, R, and Waines, J. Root biomass, water‐use efficiency, and performance of wheat–rye translocations of chromosomes 1 and 2 in spring bread wheat ‘Pavon’. Crop Science. (2003) 43:710–7. doi: 10.2135/cropsci2003.7100
61. Salvador-Moreno, N, Ryan, PR, Holguín, I, Delhaize, E, Benito, C, and Gallego, FJ. Transcriptional profiling of wheat and wheat-rye addition lines to identify candidate genes for aluminum tolerance. Biol. Planta. (2018) 62:741–9. doi: 10.1007/s10535-018-0804-5
62. Cakmak, I, Derici, R, Torun, B, Tolay, I, Braun, HJ, and Schlegel, R. Role of rye chromosomes in improvement of zinc efficiency in wheat and triticale In: T Ando, K Fujita, T Mae, H Matsumoto, S Mori, and J Sekiya, editors. Plant Nutrition for Sustainable Food Production and Environment: Proceedings of the XIII International Plant Nutrition Colloquium, 13–19 September 1997. Japan. Springer Netherlands: Tokyo (1997). 237–41. doi: 10.1007/978-94-009-0047-9_65
63. Zheng, S, Byrne, PF, Haley, SD, Shan, X, and Reid, SD. Glutenin allelic variation and 1AL.1RS effects on dough mixing properties of wheat grown in irrigated and rainfed environments. Euphytica. (2010) 176:357–69. doi: 10.1007/s10681-010-0215-y
64. Piro, MC, Muylle, H, and Haesaert, G. Exploiting Rye in Wheat Quality Breeding: The Case of Arabinoxylan Content. Plants. (2023) 12. doi: 10.3390/plants12040737
65. Kaur, R, Dhillon, GS, Kaur, A, Kaur, S, Toor, PI, Kaur, D, et al. Marker-assisted introgression of genes into rye translocation leads to the improvement in bread making quality of wheat (Triticum aestivum L.). Heredity. (2022) 128:531–41. doi: 10.1038/s41437-022-00538-w
66. Sharma, A, Garg, S, Sheikh, I, Vyas, P, and Dhaliwal, HS. Effect of wheat grain protein composition on end-use quality. J. Food Sci. and Technol. (2020) 57:2771–85. doi: 10.1007/s13197-019-04222-6
67. Itam, M, Abdelrahman, M, Yamasaki, Y, Mega, R, Gorafi, Y, Akashi, K, et al. Aegilops tauschii introgressions improve physio-biochemical traits and metabolite plasticity in bread wheat under drought stress. Agronomy. (2020) 10:1588. doi: 10.3390/agronomy10101588
68. Ma, F, Li, R, Guo, G, Nie, F, Zhu, L, Liu, W, et al. Introgression of QTL from Aegilops tauschii enhances yield-related traits in common wheat. Crop J. (2023) 11:1521–32. doi: 10.1016/j.cj.2023.05.001
69. Wan, H, Yang, Y, Li, J, Zhang, Z, and Yang, W. Mapping a major QTL for hairy leaf sheath introgressed from Aegilops tauschii and its association with enhanced grain yield in bread wheat. Euphytica. (2015) 205:275–85. doi: 10.1007/s10681-015-1457-5
70. Pestsova, EG, Börner, A, and Röder, MS. Development and QTL assessment of Triticum aestivum–Aegilops tauschii introgression lines. Theor Appl Genet. (2006) 112:634–47. doi: 10.1007/s00122-005-0166-1
71. Zhou, Y, Bai, S, Li, H, Sun, G, Zhang, D, Ma, F, et al. Introgressing the Aegilops tauschii genome into wheat as a basis for cereal improvement. Nat Plants. (2021) 7:774–86. doi: 10.1038/s41477-021-00934-w
72. Merchuk-Ovnat, L, Fahima, T, Ephrath, JE, Krugman, T, and Saranga, Y. Ancestral QTL alleles from wild emmer wheat enhance root development under drought in modern wheat. Front Plant Sci. (2017) 8:260244. doi: 10.3389/fpls.2017.00703
73. Reynolds, MP, Calderini, DF, Condon, AG, and Rajaram, S. Physiological basis of yield gains in wheat associated with the Lr19 translocation from Agropyron elongatum In: Z Bedö and L Láng, editors. Wheat in a Global Environment: Proceedings of the 6th International Wheat Conference, 5–9 June 2000, Budapest, Hungary. Dordrecht: Springer Netherlands (2001). 345–51.
74. Kumar, A, Kapoor, P, Chunduri, V, Sharma, S, and Garg, M. Potential of Aegilops sp. For improvement of grain processing and nutritional quality in wheat (Triticum aestivum). Front. Plant Sci. (2019) 10. doi: 10.3389/fpls.2019.00308
75. Bingham, SA, Day, NE, Luben, R, Ferrari, P, Slimani, N, Norat, T, et al. Dietary fibre in food and protection against colorectal cancer in the European Prospective Investigation into Cancer and Nutrition (EPIC): an observational study. Lancet. (2003) 361:1496–501. doi: 10.1016/S0140-6736(03)13174-1
76. Chhuneja, P, Dhaliwal, HS, Bains, NS, and Singh, K. Aegilops kotschyi and Aegilops tauschii as sources for higher levels of grain iron and zinc. Plant Breed. (2006) 125:529–31. doi: 10.1111/j.1439-0523.2006.01223.x
77. Tiwari, VK, Rawat, N, Neelam, K, Kumar, S, Randhawa, GS, and Dhaliwal, HS. Substitutions of 2S and 7U chromosomes of Aegilops kotschyi in wheat enhance grain iron and zinc concentration. Theor Appl Genet. (2010) 121:259–69. doi: 10.1007/s00122-010-1307-8
78. Neelam, K, Rawat, N, Tiwari, V, Prasad, R, Tripathi, S, Randhawa, G, et al. Evaluation and identification of wheat-Aegilops addition lines controlling high grain iron and zinc concentration and mugineic acid production. Cereal Res Commun. (2012) 40:53–61. doi: 10.1556/crc.40.2012.1.7
79. Alvarez, JB, and Guzmán, C. Interspecific and intergeneric hybridization as a source of variation for wheat grain quality improvement. Theor Appl Genet. (2018) 131:225–51. doi: 10.1007/s00122-017-3042-x
80. Ivanizs, L, Marcotuli, I, Rakszegi, M, Kalapos, B, Szőke-Pázsi, K, Farkas, A, et al. Identification of new QTLs for dietary fiber content in Aegilops biuncialis. Int J Mol Sci. (2022) 23. doi: 10.3390/ijms23073821
81. Mohamed, IES, Oe, H, Kamal, NM, Mustafa, HM, Gorafi, YSA, Tahir, ISA, et al. Enhancing wheat flour quality through introgression of high-molecular-weight glutenin subunits from Aegilops tauschii accessions. Front Sustain Food Syst. (2022) 6:887795. doi: 10.3389/fsufs.2022.887795
82. Li, Y, Li, Q, Hu, Q, Guzman, C, Lin, N, Xu, Q, et al. Aegilops sharonensis HMW-GSs with unusually large molecular weight improves bread-making quality in wheat-Ae. sharonensis introgression lines. J Sci Food Agric. (2023) 103:1668–75. doi: 10.1002/jsfa.12389
83. Li, Y, Zhou, R, Wang, J, Liao, X, Branlard, G, and Jia, J. Novel and favorable QTL allele clusters for end-use quality revealed by introgression lines derived from synthetic wheat. Molecular Breeding. (2012) 29:627–43. doi: 10.1007/s11032-011-9578-6
84. Shchukina, LV, Simonov, AV, Demenkova, MA, Klykov, AG, Shamanin, VP, Pozherukova, VE, et al. Increased grain protein and gluten contents of bread wheat caused by introgression of a T. timopheevii segment into chromosome 2A. Euphytica. (2022) 218:170. doi: 10.1007/s10681-022-03121-w
85. Liu, H, Tang, H, Ding, P, Mu, Y, Habib, A, Liu, Y, et al. Effects of the 1BL/1RS translocation on 24 traits in a recombinant inbred line population. Cereal Res Commun. (2020) 48:225–32. doi: 10.1007/s42976-020-00027-y
86. Jin, P, Chao, K, Li, J, Wang, Z, Cheng, P, Li, Q, et al. Functional Verification of Two Genes Related to Stripe Rust Resistance in the Wheat-Leymus mollis Introgression Line M8664-3. Front. Plant Sci. (2021):12. doi: 10.3389/fpls.2021.754823
87. Qi, LL, Pumphrey, MO, Friebe, B, Chen, PD, and Gill, BS. Molecular cytogenetic characterization of alien introgressions with gene Fhb3 for resistance to Fusarium head blight disease of wheat. Theoretical and Applied Genetics. (2008) 117:1155–66. doi: 10.1007/s00122-008-0853-9
88. Villareal, RL, Bañuelos, O, Mujeeb-Kazi, A, and Rajaram, S. Agronomic performance of chromosomes 1B and T1BL. 1RS near-isolines in the spring bread wheat Seri M82. Euphytica. (1998) 103:195–202. doi: 10.1023/A:1018392002909
89. Hoffmann, B. Alteration of drought tolerance of winter wheat caused by translocation of rye chromosome segment 1RS. Cereal Res Commun. (2008) 36:269–78. doi: 10.1556/CRC.36.2008.2.7
90. Howell, T, Moriconi, JI, Zhao, X, Hegarty, J, Fahima, T, Santa-Maria, GE, et al. A wheat/rye polymorphism affects seminal root length and yield across different irrigation regimes. J Exp Bot. (2019) 70:4027–37. doi: 10.1093/jxb/erz169
91. Ehdaie, B, Layne, AP, and Waines, JG. Root system plasticity to drought influences grain yield in bread wheat. Euphytica. (2012) 186:219–32. doi: 10.1007/s10681-011-0585-9
92. Palta, JA, Chen, X, Milroy, SP, Rebetzke, GJ, Dreccer, MF, and Watt, M. Large root systems: are they useful in adapting wheat to dry environments? Funct Plant Biol. (2011) 38:347–54. doi: 10.1071/FP11031
93. Djanaguiraman, M, Prasad, PVV, Kumari, J, Sehgal, SK, Friebe, B, Djalovic, I, et al. Alien chromosome segment from Aegilops speltoides and Dasypyrum villosum increases drought tolerance in wheat via profuse and deep root system. BMC Plant Biol. (2019) 19:242. doi: 10.1186/s12870-019-1833-8
94. Awlachew, ZT, Singh, R, Kaur, S, Bains, NS, and Chhuneja, P. Transfer and mapping of the heat tolerance component traits of Aegilops speltoides in tetraploid wheat Triticum durum. Mol Breed. (2016) 36:78. doi: 10.1007/s11032-016-0499-2
95. Liu, C, Guo, T, Du, Y, and Hu, YG. Nitrogen and phosphorus use efficiency of 43 wheat alien chromosome addition lines evaluated by hydroponic culture. J Plant Nutr. (2018) 41:2470–81. doi: 10.1080/01904167.2018.1476539
96. Johansson, E, Kuktaite, R, Labuschagne, M, Lama, S, Lan, Y, Nakimbugwe, D, et al. “Adaptation to abiotic stress factors and their effects on cereal and pseudocereal grain quality,” in Developing Sustainable and Health Promoting Cereals and Pseudocereals. eds. M Rakszegi, M Papageorgiou, and JM Rocha (Cambridge, Massachusetts: Academic Press). (2023). 339–358.
97. Johansson, E, Malik, AH, Hussain, A, Rasheed, F, Newson, WR, Plivelic, T, et al. Wheat gluten polymer structures: the impact of genotype, environment, and processing on their functionality in various applications. Cereal Chem. (2013) 90:367–76. doi: 10.1094/CCHEM-08-12-0105-FI
98. Gao, Y, and Liu, Q. A new target for improving wheat end-use quality. New Phytol. (2023) 239:5–6. doi: 10.1111/nph.18889
99. Wieser, H. Chemistry of gluten proteins. 3rd. International Symposium on Sourdough. (2007) 24:115–9. doi: 10.1016/j.fm.2006.07.004
100. Wieser, H, Koehler, P, and Scherf, KA. Chemistry of wheat gluten proteins: Quantitative composition. Cereal Chemistry. (2023) 100:36–55. doi: 10.1002/cche.10553
101. Payne, PI, Holt, LM, Jackson, EA, and Law, CN. Wheat storage proteins: their genetics and their potential for manipulation by plant breeding. Philos Trans R Soc Lond. B Biol Sci. (1984) 304:359–71. doi: 10.1098/rstb.1984.0031
102. Malik, AH, Kuktaite, R, and Johansson, E. Combined effect of genetic and environmental factors on the accumulation of proteins in the wheat grain and their relationship to bread-making quality. J Cereal Sci. (2013) 57:170–4. doi: 10.1016/j.jcs.2012.09.017
103. Fenn, D, Lukow, O, Bushuk, W, and Depauw, R. Milling and baking quality of 1BL/1RS translocation wheats. I: effects of genotype and environment. Cereal Chem. (1994) 71:189–95.
104. Sharma, Y, Sheikh, I, Sharma, A, Yadav, AN, Kumar, K, Chhuneja, P, et al. Transfer of grain softness from 5U-5A wheat-Aegilops triuncialis substitution line to bread wheat through induced homeologous pairing. Journal of Plant Biochemistry and Biotechnology. (2020) 29:407–17. doi: 10.1007/s13562-020-00554-z
105. Li, Y, Zhou, R, Wang, J, Liao, X, Branlard, G, and Jia, J. Novel and favorable QTL allele clusters for end-use quality revealed by introgression lines derived from synthetic wheat. Mol Breed. (2012) 29:627–43.
106. Orlovskaya, OA, Vakula, SI, Khotyleva, LV, and Kilchevsky, AV. Mineral composition of bread wheat lines with introgressions of alien genetic material. Proc Appl Bot Genet Breed. (2023) 184:42–52. doi: 10.30901/2227-8834-2023-1-42-52
107. Saini, P, Kaur, H, Tyagi, V, Yadav, AN, Saini, P, Sharma, V, et al. Genetic enhancement of nutritional and end-use quality in bread wheat through alien introgressions from wild relatives. Cereal Res Commun. (2023) 51:295–314. doi: 10.1007/s42976-022-00309-7
108. Singh, S, Jighly, A, Sehgal, D, Burgueño, J, Joukhadar, R, Singh, SK, et al. Direct introgression of untapped diversity into elite wheat lines. Nat Food. (2021) 2:819–27. doi: 10.1038/s43016-021-00380-z
109. Welch, RM, and Graham, RD. Breeding for micronutrients in staple food crops from a human nutrition perspective. J. Experim. Botany. (2004) 55:353–64. doi: 10.1093/jxb/erh064
110. Cakmak, I. Enrichment of cereal grains with zinc: agronomic or genetic biofortification? Plant and Soil. (2008) 302:1–17. doi: 10.1007/s11104-007-9466-3
111. Ibba, MI, Gupta, OP, Govindan, V, Johnson, AAT, Brinch-Pedersen, H, Nikolic, M, et al. Wheat biofortification to alleviate global malnutrition. Front Nutr. (2022) 9:1001443. doi: 10.3389/fnut.2022.1001443
112. Bouis, HE. Enrichment of food staples through plant breeding: a new strategy for fighting micronutrient malnutrition. Proc Nutr. (2000) 16:701–4. doi: 10.1016/S0899-9007(00)00266-5
113. Puntis, JWL. Malnutrition in developed countries. Ann Nest. (2009) 67:65–72. doi: 10.1159/000226614
114. Bruins, MJ, Bird, JK, Aebischer, CP, and Eggersdorfer, M. Considerations for secondary prevention of nutritional deficiencies in high-risk groups in high-income countries. Nutr. (2018) 10:47. doi: 10.3390/nu10010047
115. Alomari, DZ, Alqudah, AM, Pillen, K, Von Wirén, N, and Röder, MS. Toward identification of a putative candidate gene for nutrient mineral accumulation in wheat grains for human nutrition purposes. J Exp Bot. (2021) 72:6305–18. doi: 10.1093/jxb/erab297
116. Alomari, DZ, Eggert, K, Von Wiren, N, Alqudah, AM, Polley, A, Plieske, J, et al. Identifying candidate genes for enhancing grain Zn concentration in wheat. Front Plant Sci. (2018) 9:1313. doi: 10.3389/fpls.2018.01313
117. Bouis, HE, Hotz, C, McClafferty, B, Meenakshi, JV, and Pfeiffer, WH. Biofortification: a new tool to reduce micronutrient malnutrition. Food Nutr Bull. (2011) 32:S31–40. doi: 10.1177/15648265110321S105
118. Graham, RD, Welch, RM, and Bouis, HE. Addressing micronutrient malnutrition through enhancing the nutritional quality of staple foods: principles, perspectives and knowledge gaps. Adv Agron. (2001) 70:77–142. doi: 10.1016/s0065-2113(01)70004-1
119. Wakeel, A, Farooq, M, Bashir, K, and Ozturk, L. Chapter 13 - Micronutrient Malnutrition and Biofortification: Recent Advances and Future Perspectives In: MA Hossain, T Kamiya, DJ Burritt, L-S Phan Tran, and T Fujiwara, editors. Plant Micronutrient Use Efficiency : Academic Press (2018). 225–43. doi: 10.1016/B978-0-12-812104-7.00017-4
120. Brevis, JC, Morris, CF, Manthey, F, and Dubcovsky, J. Effect of the grain protein content locus Gpc-B1 on bread and pasta quality. J Cereal Sci. (2010) 51:357–65. doi: 10.1016/j.jcs.2010.02.004
121. Distelfeld, A, Uauy, C, Fahima, T, and Dubcovsky, J. Physical map of the wheat high-grain protein content gene Gpc-B1 and development of a high-throughput molecular marker. New Phytol. (2006) 169:753–63. doi: 10.1111/j.1469-8137.2005.01627.x
122. Uauy, C, Distelfeld, A, Fahima, T, Blechl, A, and Dubcovsky, J. A NAC Gene Regulating Senescence Improves Grain Protein, Zinc, and Iron Content in Wheat. Science. (2006) 314:1298–301. doi: 10.1126/science.1133649
123. Cabas-Lühmann, P, Schwember, AR, Arriagada, O, Marcotuli, I, Matus, I, Alfaro, C, et al. Meta-QTL analysis and candidate genes for quality traits, mineral content, and abiotic-related traits in wild emmer. Fron Plant Sci. (2024) 15:1305196. doi: 10.3389/fpls.2024.1305196
124. Peleg, Z, Cakmak, I, Ozturk, L, Yazici, A, Jun, Y, Budak, H, et al. Quantitative trait loci conferring grain mineral nutrient concentrations in durum wheat× wild emmer wheat RIL population. Theoretical and applied genetics. (2009) 119:353–69. doi: 10.1007/s00122-009-1044-z
125. Balyan, HS, Gupta, PK, Kumar, S, Dhariwal, R, Jaiswal, V, Tyagi, S, et al. Genetic improvement of grain protein content and other health-related constituents of wheat grain. Plant Breed. (2013) 132:446–57. doi: 10.1111/pbr.12047
126. Zhang, J, Zhen, M, Chen, Q, Li, Q, Zhang, Y, Liu, L, et al. Plant-based meat substitutes by high-moisture extrusion: Visualizing the whole process in data systematically from raw material to the products. J Integr Agric. (2022) 21:2435–44. doi: 10.1016/S2095-3119(21)63892-3
127. Lappi, J, Silventoinen-Veijalainen, VS, Rosa-Sibakov, N, and Sozer, N. The nutritional quality of animal-alternative processed foods based on plant or microbial proteins and the role of food matrix. Trends Food Sci Technol. (2022) 129:144–54. doi: 10.1016/j.tifs.2022.09.020
128. Maningat, CC, Jeradechachai, T, and Buttshaw, MR. Textured wheat and pea proteins for meat alternative applications. Cereal Chem. (2022) 99:37–66. doi: 10.1002/cche.10503
129. Grossman, L, and Weiss, J. Alternative protein sources as technofunctional food ingredients. Annu Rev Food Sci Technol. (2021) 12:93–117. doi: 10.1146/annurev-food-062520-093642
130. Riaz, M. “Texturized vegetable proteins,” in Handbook of food proteins. eds. G Philips and P Williams (Sawston, Cambridge: Woodhead publishing series, Elsevier), (2011). 395–418.
131. Ceresino, E, Johansson, E, Sato, HH, Plivelic, T, Hall, SA, and Kuktaite, R. Morphological and structural heterogeneity of solid gliadin foams modified with transgutaminase and food grade dispersants. Food Hydrocol. (2020) 108:105995. doi: 10.1016/j.foodhyd.2020.105995
132. Chen, Q, Zhang, J, Zhang, Y, Kaplan, DL, and Wang, Q. Protein-amylose/amylopectin moleclar interactions during high-moisture extruded texturization toward plant-based meat substitutes applications. Food Hydrocol. (2022) 127:107559. doi: 10.1016/j.foodhyd.2022.107559
133. Muneer, F, Hedenqvist, MS, Hall, S, and Kuktaite, R. Are ultrafine submicron sized gliadin fibrous materials suitable as bio-absorbents? Processing and post-treatment derived structures and functional properties. React Funct Polym. (2022) 181:105444. doi: 10.1016/j.reactfunctpolym.2022.105444
134. Muneer, F, Hedenqvist, MS, Hall, S, and Kuktaite, R. Innovative green way to design biobased electrospun fibers from wheat gluten and these fibers’ potential as absorbents of biofluids. ACS Environ Au. (2022) 2:232–41. doi: 10.1021/acsenvironau.1c00049
135. Zhang, J, Chen, Q, Kaplan, DL, and Wang, Q. High-moisture protein fiber formation toward plant-based meat substitutes applications: science, technology, and prospect. Trends Food Sci Technol. (2022) 128:202–16. doi: 10.1016/j.tifs.2022.08.008
136. Bohrer, BM. An investigation of the formulation and nutritional composition of modern meat analogue products. Food Sci Hum Wellness. (2019) 8:320–9. doi: 10.1016/j.fshw.2019.11.006
137. Zahari, I, Ferawati, F, Helstad, A, Ahlstrom, C, Ostbring, K, and Rayner, M. Development of high- moisture meat analogues with hemp and soy protein using extrusion cooking. Foods. (2020) 9:772. doi: 10.3390/foods9060772
138. Lee, JS, Oh, H, Choi, I, Yoon, CS, and Han, J. Physichochemical characteristics of rice protein-based novel textured vegetable protein as meat analogues produced by low-moisture extrusion cooking technology. LWT Food Sci Technol. (2022) 157:113056. doi: 10.1016/j.lwt.2021.113056
139. Pöri, P, Nisov, A, and Nordlund, E. Enzymatic modified of oat proteins concentrate with trans- and protein-glutaminase for increased fibrous structure formation during high-moisture extrusion processing. LWT Food Sci Technol. (2022) 156:113035. doi: 10.1016/j.lwt.2021.113035
140. Wittek, P, Ellwanger, F, Karbstein, HP, and Emin, MA. Morphology development and flow characteristics during high moisture extrusion of a plant-based meat analogue. Foods. (2021) 10:1753. doi: 10.3390/foods10081753
141. Lucas, I, Becker, T, and Jekle, M. Gluten polymer networks—a microstructural classification in complex systems. Polymers. (2018) 10:617. doi: 10.3390/polym10060617
142. Samard, S, Gu, B-Y, and Ryu, G-H. Effects of extrusion types, screw speed and addition of wheat gluten on physicochemical characteristics and cooking stability of meat analogues. J Sci Food Agric. (2019) 99:4922–31. doi: 10.1002/jsfa.9722
143. Azzollini, D, Derossi, A, Fogliano, V, Lakemond, CMM, and Severini, C. Effects of formulation and process conditions on microstructure, texture and digestibility of extruded insect-riched snacks. Innovative Food Sci Emer Technol. (2018) 45:344–53. doi: 10.1016/j.ifset.2017.11.017
144. Selga, L, Johansson, E, and Andersson, R. Prediction models to evaluate baking quality instruments for commercial wheat flour. Cereal Chem. (2024) 101:681–91. doi: 10.1002/cche.10772
145. Kuzmanovic, L, Rossini, F, Ruggeri, R, Pagnotta, MA, and Ceoloni, C. Engineered durum wheat germplasm with multiple alien introgressions: agronomic and quality performance. Agronomy. (2020) 10:486. doi: 10.3390/agronomy10040486
146. Heun, M, and Friebe, B. Introgression of powdery mildew resistance from rye into wheat. Phytopathology. (1990) 80:242–5. doi: 10.1094/Phyto-80-242
147. Zhang, W, Cao, Y, Zhang, M, Zhu, X, Ren, S, Long, Y, et al. Meiotic homoeologous recombination-based alien gene introgression in the genomics era of wheat. Crop Sci. (2017) 57:1189–98. doi: 10.2135/cropsci2016.09.0819
148. Martínez-Pérez, E, Shaw, P, Reader, S, Aragón-Alcaide, L, Miller, T, and Moore, G. Homologous chromosome pairing in wheat. J Cell Sci. (1999) 112:1761–9. doi: 10.1242/jcs.112.11.1761
149. Lukaszewski, AJ, Rybka, K, Korzun, V, Malyshev, SV, Lapinski, B, and Whitkus, R. Genetic and physical mapping of homoeologous recombination points involving wheat chromosome 2B and rye chromosome 2R. Genome. (2004) 47:36–45. doi: 10.1139/g03-089
150. Gupta, PK, and Vasistha, NK. Wheat cytogenetics and cytogenomics: the present status. Nucleus. (2018) 61:195–212. doi: 10.1007/s13237-018-0243-x
151. Klindworth, DL, Hareland, GA, Elias, EM, and Xu, SS. Attempted compensation for linkage drag affecting agronomic characteristics of durum wheat 1AS/1DL translocation lines. Crop Sci. (2013) 53:422–9. doi: 10.2135/cropsci2012.05.0310
152. Graybosch, RA, Peterson, C, Hansen, L, Worrall, D, Shelton, D, and Lukaszewski, A. Comparative flour quality and protein characteristics of 1BL/1RS and 1AL/1RS wheat-rye translocation lines. J Cereal Sci. (1993) 17:95–106. doi: 10.1006/jcrs.1993.1010
153. Mago, R, Spielmeyer, W, Lawrence, G, Lagudah, E, Ellis, J, and Pryor, A. Identification and mapping of molecular markers linked to rust resistance genes located on chromosome 1RS of rye using wheat-rye translocation lines. Theor Appl Genet. (2002) 104:1317–24. doi: 10.1007/s00122-002-0879-3
154. Mohler, V, Paczos-Grzęda, E, and Sowa, S. Loving the Alien: the contribution of the wild in securing the breeding of cultivated hexaploid wheat and oats. Agriculture. (2023) 13:2060. doi: 10.3390/agriculture13112060
155. Edae, EA, Olivera, PD, Jin, Y, Poland, JA, and Rouse, MN. Genotype-by-sequencing facilitates genetic mapping of a stem rust resistance locus in Aegilops umbellulata, a wild relative of cultivated wheat. BMC Genom. (2016) 17:1039. doi: 10.1186/s12864-016-3370-2
156. Ashraf, R, Johansson, E, Vallenback, P, Steffenson, BJ, Bajgain, P, and Rahmatov, M. Identification of a small translocation from 6R possessing stripe rust resistance to wheat. Plant Dis. (2023) 107:720–9. doi: 10.1094/PDIS-07-22-1666-RE
157. Bansal, M, Adamski, NM, Toor, PI, Kaur, S, Sharma, A, Srivastava, P, et al. A robust KASP marker for selection of four pairs of linked leaf rust and stripe rust resistance genes introgressed on chromosome arm 5DS from different wheat genomes. Mol Biol Rep. (2021) 48:5209–16. doi: 10.1007/s11033-021-06525-4
158. Poland, J, Endelman, J, Dawson, J, Rutkoski, J, Wu, S, Manes, Y, et al. Genomic selection in wheat breeding using genotyping-by-sequencing. Plant Genome. (2012) 5. doi: 10.3835/plantgenome2012.06.0006
159. Kim, D, Alptekin, B, and Budak, H. CRISPR/Cas9 genome editing in wheat. Funct Integr Genom. (2018) 18:31–41. doi: 10.1007/s10142-017-0572-x
160. Arndell, T, Sharma, N, Langridge, P, Baumann, U, Watson-Haigh, NS, and Whitford, R. gRNA validation for wheat genome editing with the CRISPR-Cas9 system. BMC Biotechnol. (2019) 19:71. doi: 10.1186/s12896-019-0565-z
161. Sánchez-León, S, Gil-Humanes, J, Ozuna, CV, Giménez, MJ, Sousa, C, Voytas, DF, et al. Low-gluten, nontransgenic wheat engineered with CRISPR/Cas9. Plant Biotechnol J. (2018) 16:902–10. doi: 10.1111/pbi.12837
Keywords: wheat production, nutritional and functional qualities, food security, plant breeding, dietary habit
Citation: Johansson E, Lan Y, Olalekan O, Kuktaite R, Chawade A and Rahmatov M (2024) Alien introgression to wheat for food security: functional and nutritional quality for novel products under climate change. Front. Nutr. 11:1393357. doi: 10.3389/fnut.2024.1393357
Received: 28 February 2024; Accepted: 20 May 2024;
Published: 12 June 2024.
Edited by:
João Miguel Rocha, University of Porto, PortugalReviewed by:
Michael Tilley, Agricultural Research Service (USDA), United StatesCopyright © 2024 Johansson, Lan, Olalekan, Kuktaite, Chawade and Rahmatov. This is an open-access article distributed under the terms of the Creative Commons Attribution License (CC BY). The use, distribution or reproduction in other forums is permitted, provided the original author(s) and the copyright owner(s) are credited and that the original publication in this journal is cited, in accordance with accepted academic practice. No use, distribution or reproduction is permitted which does not comply with these terms.
*Correspondence: Eva Johansson, ZXZhLmpvaGFuc3NvbkBzbHUuc2U=
Disclaimer: All claims expressed in this article are solely those of the authors and do not necessarily represent those of their affiliated organizations, or those of the publisher, the editors and the reviewers. Any product that may be evaluated in this article or claim that may be made by its manufacturer is not guaranteed or endorsed by the publisher.
Research integrity at Frontiers
Learn more about the work of our research integrity team to safeguard the quality of each article we publish.