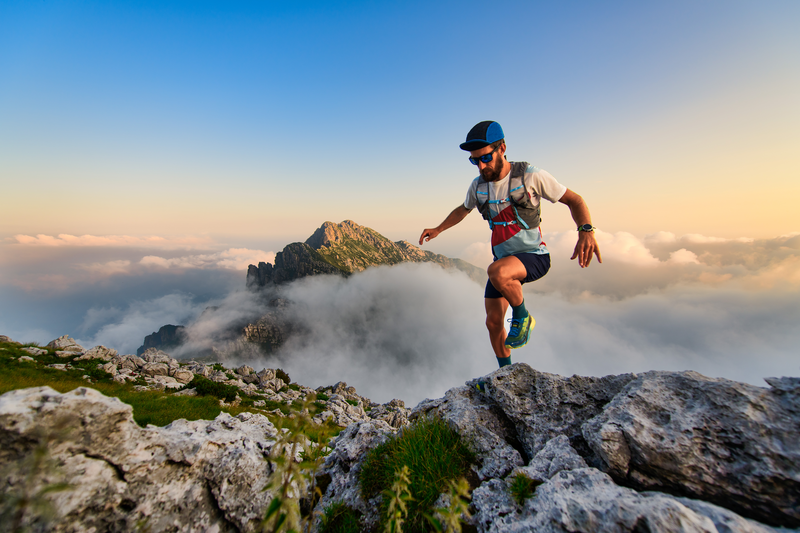
94% of researchers rate our articles as excellent or good
Learn more about the work of our research integrity team to safeguard the quality of each article we publish.
Find out more
MINI REVIEW article
Front. Nutr. , 03 April 2024
Sec. Clinical Nutrition
Volume 11 - 2024 | https://doi.org/10.3389/fnut.2024.1393182
This article is part of the Research Topic Understanding Obesity to Determine the Best Therapeutic Option: From Lifestyle Interventions to Therapies View all 16 articles
The review present data on the intricate relationship between bariatric surgery, gut microbiota, and metabolic health in obesity treatment. Bariatric surgery, is recognized as an effective intervention for managing morbid obesity, including various techniques with distinct mechanisms of action, efficacy, and safety profiles including Roux-en-Y Gastric Bypass (RYGB), Sleeve Gastrectomy (SG), Laparoscopic Adjustable Gastric Banding (LAGB), and Biliopancreatic Diversion (BPD). RYGB and SG are the most prevalent procedures globally, inducing gut microbiota changes that influence microbial diversity and abundance. Post-surgery, alterations in bacterial communities occur, such as the increased of Escherichia coli inversely correlated with fat mass and leptin levels. During digestion, microbiota produce physiologically active compounds like bile acids (Bas) and short-chain fatty acids (SCFAs). SCFAs, derived by microbial fermentation, influence appetite, energy metabolism, and obesity-related pathways. Bas, altered by surgery, modulate glucose metabolism and insulin sensitivity. Furthermore, SG and RYGB enhance incretin secretion, particularly glucagon-like peptide 1 (GLP-1). Therefore, understanding microbiota changes after bariatric surgery could be crucial for predicting metabolic outcomes and developing targeted interventions for obesity management.
The treatment of obesity presents numerous challenges due to its complex and multifactorial nature. In recent years, this condition has emerged as a global epidemic. In response to this growing health crisis, bariatric surgery has emerged as an effective intervention for managing morbid obesity and its associated comorbidities (1–3). Bariatric surgery modifies the gastrointestinal system to alter nutrient absorption (malabsorptive mechanisms) and/or restrict food intake (restrictive mechanisms) as an approach for weight management. Candidates for this surgical intervention typically have a body mass index (BMI) of 40 kg/m2 or higher, or a BMI of 35 kg/m2 or higher with significant comorbidities (3).
Various techniques are employed in bariatric surgery, each with its unique mechanism of action and associated considerations. Among the most commonly utilized techniques are biliopancreatic diversion (BPD), adjustable gastric banding (LAGB), sleeve gastrectomy (SG), and Roux-en-Y gastric bypass (RYGB). The selection of the appropriate bariatric procedure depends on several factors including the severity of obesity, presence of comorbidities, and patient preferences (4).
RYGB stands as one of the oldest and most popular bariatric procedures, ranking as the second most common worldwide (5). During this procedure, significant portions of the stomach and proximal small intestine are bypassed, resulting in the creation of a small gastric pouch directly connected to the small intestine. RYGB achieves weight loss by combining malabsorptive and restrictive mechanisms (6).
Studies consistently demonstrate that RYGB leads to substantial weight loss and improvement in associated comorbidities such as type 2 diabetes and hypertension (7, 8). However, RYGB poses inherent risks including intestinal blockage, internal hernia, and long-term metabolic issues stemming from nutrient malabsorption. Despite these risks, RYGB remains popular due to its proven effectiveness in promoting weight loss and metabolic health (5, 8, 9).
SG has gained prominence in recent years due to its less invasive nature compared to RYGB, while also preserving normal stomach function. Currently, it stands as the most common bariatric surgery (10, 11). This procedure involves the removal of a large portion of the stomach, leaving behind a narrow gastric tube that restricts food intake (10).
Both SG and RYGB yield similar outcomes in terms of weight loss and improvement in metabolic comorbidities. Additionally, SG, not requiring intestinal anastomosis, may be associated with a lower incidence of long-term complications such as intestinal blockage and internal hernia. Nevertheless, SG is not without risks, with potential postoperative issues including staple line leaking and stomach stricture (12).
LAGB is a restrictive procedure wherein a silicone band is placed around the stomach to create a small upper gastric pouch. Unlike RYGB and SG, LAGB offers reversibility and adjustability, making it an attractive option for certain patients (13).
Although LAGB was previously popular due to its reversible and minimally invasive nature, its utilization has declined in recent years due to lower rates of sustained weight reduction and increased risk of long-term complications such as band slippage and stomach erosion (14, 15).
BPD combines gastric bypass with distal gastrectomy, resulting in a reduced intestinal absorption area and nutritional malabsorption (16). While BPD is highly effective for weight loss and improving metabolic comorbidities, it also raises the risk of long-term complications such as protein and vitamin deficiencies (17, 18).
The primary objective of the present mini review is to describe the most prevalent bariatric surgery techniques used in the treatment of obesity, including RYGB, SG, LAGB, and BPD. The manuscript explores how these surgical procedures alter the complex environmental community of microorganisms, part of our microbiota, and the effect that these changes have on human health and obesity. Furthermore, the article discusses the clinical aspects of bariatric surgery, including weight loss outcomes, post-operative complications, and contributions to metabolic health enhancement. It emphasizes the importance of the gut microbiota composition in weight management, influencing lipid metabolism, hormone signaling, and glucose homeostasis.
According to the World Health Organization, more than 650 million adults were obese in 2016, with a perspective of an increment (19). Besides, obesity is associated with elevated risk of various comorbidities, including type 2 diabetes, heart conditions, hypertension, among others (20). Consequently, bariatric surgery has emerged as an effective and long-lasting option for achieving significant health improvements.
Bariatric surgery is considered a potential treatment intervention for obesity, commonly for subjects with a BMI of 40 kg/m2 or higher, or a BMI of 35 kg/m2 or higher with significant comorbidities. The primary goal of bariatric surgery is to reduce the size of the stomach or alter the digestive tract to induce the decrease the volume of food taken and its absorption, with an impact from hormonal to molecular changes (21, 22). Consequently, bariatric surgery can result in a 50–70% in short term weight loss or 20–30% loss of the patient's initial weight (23).
The mechanisms of action, effectiveness, and safety profiles vary among the different types of bariatric surgery. The RYGB and SG are the most common procedures worldwide, accounting for 72,645 individuals (38.2%) and 87,467 individuals (46%) of all primary operations since 2014, respectively. On the other hand, One-anastomosis gastric bypass (OAGB) and gastric bypass (GB) surgeries are less frequently performed, representing 14,516 individuals (7.6%) and 9,534 individuals (5%) of all primary operations since 2014, respectively (24, 25). After one-year post-surgery, the mean weight loss was 28.9% with an improving in metabolic health. Remarkably, 66.1% of patients with type 2 diabetes did not need more medication. Consequently, the degree of diabetes remission correlated closely with weight loss achieved (25).
Despite the significant benefits of bariatric surgery, it is crucial to acknowledge potential complications associated with these procedures. Data from clinical studies and registry analyses into the rates of postoperative complications are limited. However, a systematic review reported that the most common complications within 30 days after bariatric procedures, includes anastomotic leak, myocardial infarction, pulmonary embolism (26). Another study reported that the most common complication after surgery is peritonitis with an incidence of 1–6% after GB and 3–7% after SG (27). The most frequent late postoperative complications are dumping syndrome and cholecystitis, each occurring in up to 30% of cases (27). Additionally, the perioperative mortality rate is <1% (27). Despite advancements in surgical techniques and perioperative care leading to improvements in safety outcomes, it is essential for healthcare providers and patients to be aware of the potential risks associated with bariatric surgery.
Minimally invasive approaches, such as laparoscopic and robotic-assisted procedures, have become increasingly common in bariatric surgery. These techniques result in shorter hospital stays, faster recovery times, and improved patient outcomes. Additionally, multidisciplinary care involving nutritionists, psychologists, and other healthcare professionals plays a crucial role in mitigating complications and supporting patients throughout their bariatric surgery (28). Furthermore, studies suggest that individuals with obesity who undergo bariatric surgery should engage in moderate physical activity and make dietary changes to sustain their weight loss (29, 30).
Consequently, bariatric surgery is a noteworthy treatment for obesity, offering significant weight loss outcomes and improvements in metabolic health. While RYGB and SG continue to be the predominant surgical modalities, ongoing research and advancements in surgical techniques aim to further enhance the safety and efficacy of these procedures.
Recent research has elucidated the intricate relationship between obesity and the gut microbiota, particularly focusing on the modulation of host metabolism by microbiota-derived metabolites. Short-chain fatty acids (SCFAs), such as acetate, propionate and butyrate, are produced through the fermentation of dietary fiber by gut bacteria. SCFAs act as a signaling molecules influencing metabolic processes crucial for energy homeostasis and lipid metabolism (31, 32). Furthermore, the gut microbiota impacts host metabolism by regulating the expression of genes involved in adipogenesis, lipid metabolism, and insulin sensitivity. Dysbiosis, observed in obese individual, contributes in disturbances involving host-microbiota interactions.
Obesity is associated with chronic low-level inflammation in several tissues, which has been correlated with metabolic diseases like type 2 diabetes, insulin resistance, and cardiovascular diseases. One of the effects of this chronic inflammation is gut barrier impairment. It has been proposed that hyperglycemia and a low gut bacteria diversity could lead to gut barrier permeabilization, allowing the entry of antigenic compounds like lipopolysaccharides to blood circulation. These antigenic compounds can induce endotoxemia, insulin resistance and chronic immune system activation (33). Therefore, modulating gut microbiota after bariatric surgery could potentially improve the intestinal barrier and restore metabolic homeostasis (33).
Emerging evidence suggests the role of gut microbiota in obesity. Studies comparing the microbial communities in obese individuals with non-obese, have consistently revealed differences in the abundance Firmicutes and Bacteroidetes (34–36). Another study demonstrated an increase in Bacteroidetes thetaiotaomicron, a glutamate fermenting commensal, in obese individuals who follows a weight-loss intervention (like sleeve gastrectomy) (37). Consequently, B. thetaiotaomicron reduces plasma glutamate concentration and may protect against body weight gain induced by diet and adiposity (37). Moreover, research has shown that Bacteroidetes uniformis relieves high-fat-diet induced obesity, complementing the effect of B. thetaiotaomicron (38). Specifically, B. uniformis increases TNF-α production by dendritic cells (DCs) in response to purified lipopolysaccharide stimulation (reduced by high-fat-diet) (38).
Consequently, the increased abundance and diversity of SCFA-producing bacteria, lead to heightened production of host glucagon-like peptide 1 (GLP-1), which contributes to glucose-dependent stimulation of insulin secretion, inhibition of food intake, increase of natriuresis and diuresis, among other metabolic effects (39). Dysregulation of these pathways may contribute to overeating and weight gain, highlighting the molecular basis of the gut-brain axis in obesity (40). Furthermore, the gut microbiota produces metabolites that play critical roles in lipid metabolism, energy expenditure, and inflammation, thus shaping the metabolic phenotype of the host (31, 41, 42). Strategies aimed at modulating gut microbiota composition and activity, such as probiotics, prebiotics, and fecal microbiota transplantation, have shown potential in improving metabolic dysfunction associated with obesity.
Furthermore, the consumption of the probiotic Lactobacillus gasseri BNR17 has been approved by the Korean FDA as an ingredient to reduce visceral adipose tissue in adults with obesity (43). Moreover, some studies are based on Akkermansia muciniphila, Faecalibacterium prausnitzii and Clostridia strains considered as possible probiotics, most of them present in the human intestinal microbiota. These strains produce butyrate and other short-chain fatty acids (SCFAs), compounds that are decreased in people with obesity (44).
Recent studies have highlighted the significant role of the gut microbiota before and after bariatric surgery, associating the complex interplay between gut microbiota, surgical interventions, and metabolic health outcomes (Table 1). A ten-year review study described the alterations in gut microbiota composition in obese individuals before and after bariatric surgery, showing that changes in the composition and function of gut microbiota affect metabolic functions in obese patients, leading to significant physiological regulation (50).
The type of bariatric surgery performed influences the changes in gut microbiota. Several studies have investigated changes following RYGB, noting variation in microbial diversity across intestinal segments after surgery (51). Furthermore, other studies have reported the changes in the microbial communities. For example, one study found that the Bacteroides/Prevotella was lower in obese subjects and increased post-surgery. Additionally, lactic and acid bacteria (Lactobacillus, Leuconostoc, Bifidobacterium, and Pediococcus group) were reduced, while Escherichia coli increased after surgery and inversely correlated with fat mass and leptin levels independent of dietary changes (45). Another study reported that after RYGB, Enterobacter cancerogenus (Proteobacterium) increased, while Firmicutes and Bacteroidetes decreased, improving in host lipids and glucose levels (46). Similarly, other studies also reported a decrease in Firmicutes and Bacteroidetes (specifically Bacteroides thetaiotaomicron) after surgery while Proteobacteria species increased (47, 48).
Studies on SG, also found significant shifts in the gut microbiota. A next -generation sequencing analyses revealed a decrease in energy-reabsorbing potential after SG, indicated by the Bacteroidetes/Firmicutes ratio. Additionally, the capacity for butyrate fermentation decreased, attributed to Firmicutes changes (52).
The impact of microbial shifts extends beyond weight loss. For instance, the increase of Akkermansia muciniphila post-surgery is linked to improved glucose homeostasis and lipid metabolism critical in the remission of type 2 diabetes. Besides, the decrease in Firmicutes and increase in Bacteroidetes after surgery play a role in reducing low-grade inflammation associated with obesity and metabolic syndrome (49).
Understanding these bacterial changes (Figure 1) is essential for predicting the bariatric surgery outcomes. However, future research may focus on preoperative and postoperative modulation of the gut microbiota to enhance bariatric procedures.
Figure 1. Bacterial abundance changes in obese individuals before and after bariatric surgery. RYGB, Roux-en-Y gastric bypass; SG, sleeve gastrectomy. The figure was created with BioRender.com.
The human intestine harbors over 100 trillion microbial cells, which significantly influences metabolic regulation through symbiotic interactions with the host (53). During digestion, the gut microbiota generates various physiologically active compounds, including BAs, SCFAs, and long-chain fatty acids (LCFAs) (31). The activity and composition of the gut microbiota can be altered by various factors such as nutritional intake, gastric emptying, and gastric acid production, thereby being influenced by different bariatric surgical methods (54, 55).
The chronic inflammatory process observed in various tissues of individuals with obesity has been linked to gut mucosa impairment. This impairment is associated with reduced synthesis of the mucus protein layer, allowing the release of pro-inflammatory cytokines (IL-1b, IL-6, IL-12, IL-18). Bariatric surgery has been related to an improved intestinal barrier synthesis due to the expression of ZO-1, occludin, and claudin-1 tight junction proteins. Moreover, the restoration of bacteria involved in SCFA synthesis, which has been observed after bariatric surgery (33), has also been linked to intestinal barrier restoration (56). Therefore, the restoration of intestinal mucosa layer could potentially improve the inflammatory process and restore the metabolic homeostasis.
Among the bariatric procedures, SG and RYGB stand out as the most widely practiced, both contributing to an increase in the secretion of incretins by augmenting the number of secreting cells, particularly GLP-1 (57).
Short-chain fatty acids, including butyrate, propionate, and acetate, are key metabolites derived from the metabolism of complex carbohydrates by gut microbiota (58). While studies indicate higher fecal concentrations of SCFAs in obese individuals, their role in energy metabolism and obesity remains controversial due to their dual effects on hunger reduction, lipogenesis inhibition, and induction of browning in white adipose cells (40, 59, 60). SCFAs exert their appetite-suppressing effects by interacting with isolated neurons in the nodal ganglia, triggering intracellular Ca2+ signaling, and elevating serum levels of leptin, GLP-1, and peptide YY (PYY) (61, 62).
Following bariatric surgery, fecal SCFA levels decrease, primarily attributed to low-carbohydrate diets, possibly indicating inefficient utilization of dietary SCFAs for energy during weight loss (63). RYGB surgery reduces stomach acid secretion, leading to higher levels of partially digested proteins in the intestine and resulting in putrescine generation. Additionally, an increase in Klebsiella bacteria post-RYGB further contributes to putrescine production. The metabolism of putrescine yields gamma-aminobutyric acid (GABA), exacerbating insulin resistance and elevating GLP-1 levels (64).
After RYGB, there is an increase in the abundance of Streptococcus, Veillonella, and Akkermansia species. Streptococcus and Veillonella metabolize lactate, impacting butyrate metabolism and epithelial barrier integrity, which may potentially ameliorate metabolic disorders, and reduce systemic inflammation. Akkermansia muciniphila, has been associated with protection against diabetes and obesity in animal studies, may improve insulin sensitivity and reduce inflammation, further enhancing intestinal epithelial integrity in humans (65, 66).
Bariatric surgery induces alterations in BA metabolism, enhancing energy homeostasis. BAs play an important role in gut microbiota composition and post-surgery weight loss by modulating glucose metabolism, increasing insulin sensitivity, and reducing gluconeogenesis through elevated GLP-1 secretion and activation of G protein-coupled receptor (TGR5) and nuclear receptor (FXRα) pathways (67–70).
Ilhan et al. (71) reported decreased fecal BA concentration in obese patients post-RYGB. This decrease was associated with microbiota composition changes (71). RYGB-induced architectural modifications enhance the BA influx into the lower intestine. This facilitates reabsorption of conjugated BAs in the terminal ileum and conversion of primary to secondary BAs by gut microbes in the colon. These metabolic improvements, including gut microbiota repopulation and altered primary/secondary BAs ratio, had a positive impact on metabolic syndrome (45, 72).
In contrast, Evers et al. (73) observed decreased levels of lithocholic acid (LCA) in the colon and increased levels in the portal vein post-SG. LCA promotes CA7S production in the livers of mice and humans, impacting host metabolism. The researchers also determined that LCA activates the vitamin D receptor and induces cholic acid sulfonation both in vitro in human hepatocytes and in vivo in mice. The CA7S synthesized by LCA in human hepatocytes can trigger GLP-1 secretion in enteroendocrine cells, establishing a link between BA level alterations post-SG and the favorable effects on energy and glucose homeostasis (73).
In conclusion, the comprehensive review of microbiota dynamics preceding bariatric surgery emphasizes the role of gut microbiota in the management of obesity and associated metabolic disorders. The observed alterations in gut microbial composition following bariatric procedures, such as Roux-en-Y gastric bypass and sleeve gastrectomy, highlight the potential for microbiota modulation as a therapeutic strategy to enhance surgical outcomes. These alterations, including changes in microbial diversity and abundance, have been linked to improvements in glucose homeostasis, lipid metabolism, and inflammation, crucial factors in achieving remission of type 2 diabetes and metabolic syndrome. Understanding the complex interplay between gut microbiota, surgical interventions, and metabolic health outcomes is essential for optimizing patient care and developing targeted interventions to enhance the efficacy of bariatric surgery. Further research into preoperative and postoperative microbiota modulation holds promise for improving the safety and long-term success of bariatric procedures, ultimately offering hope for individuals grappling with obesity and its related complications.
AZ: Conceptualization, Investigation, Supervision, Writing – original draft, Writing – review & editing. EP-C: Conceptualization, Investigation, Writing – original draft, Writing – review & editing. VR-P: Investigation, Writing – review & editing. SC-U: Investigation, Writing – review & editing. RT-T: Investigation, Writing – review & editing. PG-R: Investigation, Writing – review & editing. RZ-V: Investigation, Writing – review & editing. DS-R: Investigation, Writing – review & editing.
The author(s) declare that financial support was received for the research, authorship, and/or publication of this article. The publication fee of this article were funded by Universidad UTE.
The authors are grateful to Universidad UTE for their support.
The authors declare that the research was conducted in the absence of any commercial or financial relationships that could be construed as a potential conflict of interest.
All claims expressed in this article are solely those of the authors and do not necessarily represent those of their affiliated organizations, or those of the publisher, the editors and the reviewers. Any product that may be evaluated in this article, or claim that may be made by its manufacturer, is not guaranteed or endorsed by the publisher.
1. Cerreto M, Santopaolo F, Gasbarrini A, Pompili M, Ponziani FR. Bariatric surgery and liver disease: general considerations and role of the gut-liver axis. Nutrients. (2021) 13:2649. doi: 10.3390/nu13082649
2. Istfan NW, Lipartia M, Anderson WA, Hess DT, Apovian CM. Approach to the patient: management of the post-bariatric surgery patient with weight regain. J Clin Endocrinol Metab. (2021) 106:251–63. doi: 10.1210/clinem/dgaa702
3. Gasoyan H, Tajeu G, Halpern MT, Sarwer DB. Reasons for underutilization of bariatric surgery: the role of insurance benefit design. Surg Obes Relat Dis. (2019) 15:146–51. doi: 10.1016/j.soard.2018.10.005
4. Pourdeh EF, Ulker I, Pourdeh EF, Ulker I. Do all bariatric surgery methods have the same effects on the gut microbiota? In: Bariatric Surgery - Past and Present. London: IntechOpen (2022). Available online at: https://www.intechopen.com/chapters/83487
5. Amor I Ben, Kassir R, Petrucciani N, Almunifi A, Debs T, Gugenheim J. An alternative technique of reversal of Roux-en-Y gastric bypass: the small bowel limb transposition. Obes Surg. (2019) 29:4142–3. doi: 10.1007/s11695-019-04158-y
6. Peterli R, Wolnerhanssen BK, Peters T, Vetter D, Kroll D, Borbely Y, et al. Effect of Laparoscopic Sleeve Gastrectomy vs Laparoscopic Roux-en-Y Gastric Bypass on Weight Loss in Patients With Morbid Obesity: The SM-BOSS Randomized Clinical Trial. JAMA. (2018) 319:255–65. doi: 10.1001/jama.2017.20897
7. Chahal-Kummen M, Blom-Høgestøl IK, Eribe I, Klungsøyr O, Kristinsson J, Mala T. Abdominal pain and symptoms before and after Roux-en-Y gastric bypass. BJS Open. (2019) 3:317–26. doi: 10.1002/bjs5.50148
8. Wijngaarden LH, van Veldhuisen SL, Klaassen RA, van der Harst E, van Rossem CC, Demirkiran A, et al. Predicting symptom relief after reoperation for suspected internal herniation after laparoscopic roux-en-Y gastric bypass. Obes Surg. (2018) 28:3801–8. doi: 10.1007/s11695-018-3404-8
9. Nuytens F, D'Hondt M, Van Rooy F, Vansteenkiste F, Pottel H, Abasbassi M, et al. Closure of mesenteric defects is associated with a higher incidence of small bowel obstruction due to adhesions after laparoscopic antecolic Roux-en-y gastric bypass: a retrospective cohort study. Int J Surg. (2019) 71:149–55. doi: 10.1016/j.ijsu.2019.09.017
10. Garofalo F, Pescarus R, Denis R, Atlas H, Garneau P, Philie M, et al. Laparoscopic sleeve gastrectomy: a radiological guide to common postsurgical failure. Can Assoc Radiol J. (2018) 69:184–96. doi: 10.1016/j.carj.2017.10.004
11. Batman B, Altun H. Benefits of suture reinforcement in laparoscopic sleeve gastrectomy. Surg Laparosc Endosc Percutan Tech. (2019) 29:539–42. doi: 10.1097/SLE.0000000000000722
12. Wang H, Lu J, Feng J, Wang Z. Staple line oversewing during laparoscopic sleeve gastrectomy. Ann R Coll Surg Engl. (2017) 99:509–14. doi: 10.1308/rcsann.2017.0074
13. Leca BM, Khan U, Abraham J, Halder L, Shuttlewood E, Shah N, et al. Laparoscopic adjustable gastric banding-should a second chance be given? Obes Surg. (2020) 30:2913–9. doi: 10.1007/s11695-020-04613-1
14. Özden S, Saylam B, Avsar FM. Long-term results of the patients who were applied laparoscopic adjustable gastric banding. Turk J Surg. (2018) 35:79–85. doi: 10.5578/turkjsurg.4038
15. Mansour S, Borzellino G, Kluger Y, Khuri S. Unexpected gastrointestinal tract injury years following laparoscopic adjustable gastric banding. Int J Surg Case Rep. (2020) 77:412–7. doi: 10.1016/j.ijscr.2020.11.023
16. Bianchi A, Pagan-Pomar A, Jimenez-Segovia M, Martinez-Corcoles JA, Gonzalez-Argenté FX. Biliopancreatic diversion in the surgical treatment of morbid obesity: long-term results and metabolic consequences. Obes Surg. (2020) 30:4234–42. doi: 10.1007/s11695-020-04777-w
17. Pérez-Pevida B, Trifu DS, Kamocka A, Álvarez Hernández J. Malnutrition secondary to gastrojejunal stricture after biliopancreatic diversion. Int J Surg Case Rep. (2018) 44:230–2. doi: 10.1016/j.ijscr.2018.02.040
18. Steenackers N, Brouwers E, Mertens A, Van Cleynenbreugel S, Lannoo M, Flamaing J, et al. Late complications of biliopancreatic diversion in an older patient: a case report. BMC Geriatr. (2021) 21:1. doi: 10.1186/s12877-021-02578-z
20. Kloock S, Ziegler CG, Dischinger U. Obesity and its comorbidities, current treatment options and future perspectives: Challenging bariatric surgery? Pharmacol Ther. (2023) 251:108549. doi: 10.1016/j.pharmthera.2023.108549
21. Courcoulas AP, Yanovski SZ, Bonds D, Eggerman TL, Horlick M, Staten MA, et al. Long-term outcomes of bariatric surgery: a National Institutes of Health symposium. JAMA Surg. (2014) 149:1323–9. doi: 10.1001/jamasurg.2014.2440
22. Gulinac M, Miteva DG, Peshevska-Sekulovska M, Novakov IP, Antovic S, Peruhova M, et al. Long-term effectiveness, outcomes and complications of bariatric surgery. World J Clin Cases. (2023) 11:4504. doi: 10.12998/wjcc.v11.i19.4504
23. Alfadda AA, Al-Naami MY, Masood A, Elawad R, Isnani A, Ahamed SS, et al. Long-term weight outcomes after bariatric surgery: a single center Saudi Arabian cohort experience. J Clin Med. (2021) 10:21. doi: 10.3390/jcm10214922
24. Alsuhibani A, Thompson JR, Wigle PR, Guo JJ, Lin AC, Rao MB, et al. Metabolic and bariatric surgery utilization trends in the united states: evidence from 2012 to 2021 National Electronic Medical Records Network. Ann Surg Open. (2023) 4:e317. doi: 10.1097/AS9.0000000000000317
25. Welbourn R, Hollyman M, Kinsman R, Dixon J, Liem R, Ottosson J, et al. Bariatric surgery worldwide: baseline demographic description and one-year outcomes from the fourth IFSO global registry report 2018. Obes Surg. (2019) 29:782–95. doi: 10.1007/s11695-018-3593-1
26. Chang SH, Freeman NLB, Lee JA, Stoll CRT, Calhoun AJ, Eagon JC, et al. Early major complications after bariatric surgery in the USA, 2003-2014: a systematic review and meta-analysis. Obes Rev. (2018) 19:529–37. doi: 10.1111/obr.12647
27. Kassir R, Debs T, Blanc P, Gugenheim J, Ben Amor I, Boutet C, et al. Complications of bariatric surgery: Presentation and emergency management. Int J Surg. (2016) 27:77–81. doi: 10.1016/j.ijsu.2016.01.067
28. Shiau J, Biertho L. Bariatric surgery: postoperative management. In: Canadian Adult Obesity Clinical Practice Guidelines. Mountain View: Creative Commons (2020). Available online at: https://obesitycanada.ca/guidelines/postop
29. Santos C, Carvalho M, Oliveira L, Palmeira A, Monteiro Rodrigues L, Gregório J. The long-term association between physical activity and weight regain, metabolic risk factors, quality of life and sleep after bariatric surgery. Int J Environ Res Public Health. (2022) 19:8328. doi: 10.3390/ijerph19148328
30. Barrea L, Salzano C, Pugliese G, Laudisio D, Frias-Toral E, Savastano S, et al. The challenge of weight loss maintenance in obesity: a review of the evidence on the best strategies available. Int J Food Sci Nutr. (2022) 73:1030–46. doi: 10.1080/09637486.2022.2130186
31. Lin K, Zhu L, Yang L. Gut and obesity/metabolic disease: focus on microbiota metabolites. MedComm (Beijing). (2022) 3:3. doi: 10.1002/mco2.171
32. Den Besten G, Van Eunen K, Groen AK, Venema K, Reijngoud DJ, Bakker BM. The role of short-chain fatty acids in the interplay between diet, gut microbiota, and host energy metabolism. J Lipid Res. (2013) 54:2325. doi: 10.1194/jlr.R036012
33. Cardenas D, Verde L, Pablo Chapela S. Gut microbiota and obesity: new insights. Front Nutr. (2022) 9:1–12. doi: 10.3389/fnut.2022.1018212
34. Zambrano AK, Cadena-Ullauri S, Guevara-Ramírez P, Frias-Toral E, Ruiz-Pozo VA, Paz-Cruz E, et al. The impact of a very-low-calorie ketogenic diet in the gut microbiota composition in obesity. Nutrients. (2023) 15:2728. doi: 10.3390/nu15122728
35. Huttenhower C, Gevers D, Knight R, Abubucker S, Badger JH, Chinwalla AT, et al. Structure, function and diversity of the healthy human microbiome. Nature. (2012) 486:207–14. doi: 10.1038/nature11234
36. Ley RE, Turnbaugh PJ, Klein S, Gordon J. Human gut microbes associated with obesity. Yearbook Endocrinol. (2006) 444:163–5. doi: 10.1016/S0084-3741(08)70094-5
37. Liu R, Hong J, Xu X, Feng Q, Zhang D, Gu Y, et al. Gut microbiome and serum metabolome alterations in obesity and after weight-loss intervention. Nat Med. (2017) 23:859–68. doi: 10.1038/nm.4358
38. Gauffin Cano P, Santacruz A, Moya Á, Sanz Y. Bacteroides uniformis CECT 7771 ameliorates metabolic and immunological dysfunction in mice with high-fat-diet induced obesity. PLoS ONE. (2012) 7:7. doi: 10.1371/journal.pone.0041079
39. Müller TD, Finan B, Bloom SR, D'Alessio D, Drucker DJ, Flatt PR, et al. Glucagon-like peptide 1 (GLP-1). Mol Metab. (2019) 30:72. doi: 10.1016/j.molmet.2019.09.010
40. Schwiertz A, Taras D, Schäfer K, Beijer S, Bos NA, Donus C, et al. Microbiota and SCFA in lean and overweight healthy subjects. Obesity. (2010) 18:190–5. doi: 10.1038/oby.2009.167
41. Zhao L, Zhang F, Ding X, Wu G, Lam YY, Wang X, et al. Gut bacteria selectively promoted by dietary fibers alleviate type 2 diabetes. Science. (2018) 359:1151–6. doi: 10.1126/science.aao5774
42. Tremaroli V, Bäckhed F. Functional interactions between the gut microbiota and host metabolism. Nature. (2012) 489:242–9. doi: 10.1038/nature11552
43. Kim J, Yun JM, Kim MK, Kwon O, Cho B. Lactobacillus gasseri BNR17 supplementation reduces the visceral fat accumulation and waist circumference in obese adults: a randomized, double-blind, placebo-controlled trial. J Med Food. (2018) 21:454–61. doi: 10.1089/jmf.2017.3937
44. Vallianou N, Stratigou T, Christodoulatos GS, Tsigalou C, Dalamaga M. Probiotics, prebiotics, synbiotics, postbiotics, and obesity: current evidence, controversies, and perspectives. Curr Obes Rep. (2020) 9:179–92. doi: 10.1007/s13679-020-00379-w
45. Koulas SG, Stefanou CK, Stefanou SK, Tepelenis K, Zikos N, Tepetes K, et al. Gut microbiota in patients with morbid obesity before and after bariatric surgery: a ten-year review study (2009–2019). Obes Surg. (2021) 31:317–26. doi: 10.1007/s11695-020-05074-2
46. Osto M, Abegg K, Bueter M, le Roux CW, Cani PD, Lutz TA. Roux-en-Y gastric bypass surgery in rats alters gut microbiota profile along the intestine. Physiol Behav. (2013) 119:92–6. doi: 10.1016/j.physbeh.2013.06.008
47. Furet JP, Kong LC, Tap J, Poitou C, Basdevant A, Bouillot JL, et al. Differential adaptation of human gut microbiota to bariatric surgery–induced weight loss: links with metabolic and low-grade inflammation markers. Diabetes. (2010) 59:3049. doi: 10.2337/db10-0253
48. Graessler J, Qin Y, Zhong H, Zhang J, Licinio J, Wong ML, et al. Metagenomic sequencing of the human gut microbiome before and after bariatric surgery in obese patients with type 2 diabetes: correlation with inflammatory and metabolic parameters. Pharmacogenom J. (2013) 13:514–22. doi: 10.1038/tpj.2012.43
49. Li JV, Reshat R, Wu Q, Ashrafian H, Bueter M, le Roux CW, et al. Experimental bariatric surgery in rats generates a cytotoxic chemical environment in the gut contents. Front Microbiol. (2011) 2:183. doi: 10.3389/fmicb.2011.00183
50. Li JV, Ashrafian H, Bueter M, Kinross J, Sands C, Le Roux CW, et al. Metabolic surgery profoundly influences gut microbial–host metabolic cross-talk. Gut. (2011) 60:1214–23. doi: 10.1136/gut.2010.234708
51. Damms-Machado A, Mitra S, Schollenberger AE, Kramer KM, Meile T, Königsrainer A, et al. Effects of surgical and dietary weight loss therapy for obesity on gut microbiota composition and nutrient absorption. Biomed Res Int. (2015) 2015:806248. doi: 10.1155/2015/806248
52. Davies N, O'Sullivan JM, Plank LD, Murphy R. Gut microbial predictors of type 2 diabetes remission following bariatric surgery. Obes Surg. (2020) 30:3536–48. doi: 10.1007/s11695-020-04684-0
53. Boulangé CL, Neves AL, Chilloux J, Nicholson JK, Dumas ME. Impact of the gut microbiota on inflammation, obesity, and metabolic disease. Genome Med. (2016) 8:2. doi: 10.1186/s13073-016-0303-2
54. Sánchez-Alcoholado L, Gutiérrez-Repiso C, Gómez-Pérez AM, García-Fuentes E, Tinahones FJ, Moreno-Indias I. Gut microbiota adaptation after weight loss by Roux-en-Y gastric bypass or sleeve gastrectomy bariatric surgeries. Surg Obes Relat Dis. (2019) 15:1888–95. doi: 10.1016/j.soard.2019.08.551
55. Aron-Wisnewsky J, Clement K. The effects of gastrointestinal surgery on gut microbiota: potential contribution to improved insulin sensitivity. Curr Atheroscler Rep. (2014) 16:1–11. doi: 10.1007/s11883-014-0454-9
56. Zhang Y, Zhu X, Yu X, Novák P, Gui Q, Yin K. Enhancing intestinal barrier efficiency: A novel metabolic diseases therapy. Front Nutr. (2023) 10:1–20. doi: 10.3389/fnut.2023.1120168
57. Cavin JB, Couvelard A, Lebtahi R, Ducroc R, Arapis K, Voitellier E, et al. Differences in alimentary glucose absorption and intestinal disposal of blood glucose after roux-en-Y gastric bypass vs sleeve gastrectomy. Gastroenterology. (2016) 150:454–464.e9. doi: 10.1053/j.gastro.2015.10.009
58. Martin-Gallausiaux C, Marinelli L, Blottière HM, Larraufie P, Lapaque N. SCFA: mechanisms and functional importance in the gut. Proc Nutr Soc. (2021) 80:37–49. doi: 10.1017/S0029665120006916
59. Turnbaugh PJ, Ley RE, Mahowald MA, Magrini V, Mardis ER, Gordon JI. An obesity-associated gut microbiome with increased capacity for energy harvest. Nature. (2006) 444:1027–31. doi: 10.1038/nature05414
60. Xiong RG, Zhou DD, Wu SX, Huang SY, Saimaiti A, Yang ZJ, et al. Health benefits and side effects of short-chain fatty acids. Foods. (2022) 11:2863. doi: 10.3390/foods11182863
61. Jiao A, Yu B, He J, Yu J, Zheng P, Luo Y, et al. Short chain fatty acids could prevent fat deposition in pigs via regulating related hormones and genes. Food Funct. (2020) 11:1845–55. doi: 10.1039/C9FO02585E
62. Goswami C, Iwasaki Y, Yada T. Short-chain fatty acids suppress food intake by activating vagal afferent neurons. J Nutr Biochem. (2018) 57:130–5. doi: 10.1016/j.jnutbio.2018.03.009
63. Sowah SA, Riedl L, Damms-Machado A, Johnson TS, Schübel R, Graf M, et al. Effects of weight-loss interventions on short-chain fatty acid concentrations in blood and feces of adults: a systematic review. Adv Nutr. (2019) 10:673–84. doi: 10.1093/advances/nmy125
64. Faria SL, Santos A, Magro DO, Cazzo E, Assalin HB, Guadagnini D, et al. Gut microbiota modifications and weight regain in morbidly obese women after roux-en-Y gastric bypass. Obes Surg. (2020) 30:4958–66. doi: 10.1007/s11695-020-04956-9
65. Liu Z, Coales I, Penney N, McDonald JAK, Phetcharaburanin J, Seyfried F, et al. A subset of roux-en-y gastric bypass bacterial consortium colonizes the gut of nonsurgical rats without inducing host-microbe metabolic changes. mSystems. (2020) 5:20. doi: 10.1128/mSystems.01047-20
66. Fouladi F, Carroll IM, Sharpton TJ, Bulik-Sullivan E, Heinberg L, Steffen KJ, et al. A microbial signature following bariatric surgery is robustly consistent across multiple cohorts. Gut Microbes. (2021) 13:1. doi: 10.1080/19490976.2021.1930872
67. Seyfried F, Phetcharaburanin J, Glymenaki M, Nordbeck A, Hankir M, Nicholson JK, et al. Roux-en-Y gastric bypass surgery in Zucker rats induces bacterial and systemic metabolic changes independent of caloric restriction-induced weight loss. Gut Microbes. (2021) 13:1–20. doi: 10.1080/19490976.2021.1875108
68. Pournaras DJ, Glicksman C, Vincent RP, Kuganolipava S, Alaghband-Zadeh J, Mahon D, et al. The role of bile after Roux-en-Y gastric bypass in promoting weight loss and improving glycaemic control. Endocrinology. (2012) 153:3613–9. doi: 10.1210/en.2011-2145
69. Aron-Wisnewsky J, Doré J, Clement K. The importance of the gut microbiota after bariatric surgery. Nat Rev Gastroenterol Hepatol. (2012) 9:590–8. doi: 10.1038/nrgastro.2012.161
70. Martinot E, Sèdes L, Baptissart M, Lobaccaro JM, Caira F, Beaudoin C, et al. Bile acids and their receptors. Mol Aspects Med. (2017) 56:2–9. doi: 10.1016/j.mam.2017.01.006
71. Ilhan ZE, DiBaise JK, Dautel SE, Isern NG, Kim YM, Hoyt DW, et al. Temporospatial shifts in the human gut microbiome and metabolome after gastric bypass surgery. NPJ Biofilms Microbiomes. (2020) 6:1. doi: 10.1038/s41522-020-0122-5
72. Talavera-Urquijo E, Beisani M, Balibrea JM, Alverdy JC. Is bariatric surgery resolving NAFLD via microbiota-mediated bile acid ratio reversal? A comprehensive review. Surg Obes Relat Dis. (2020) 16:1361–9. doi: 10.1016/j.soard.2020.03.013
73. Evers SS, Sandoval DA, Seeley RJ. The physiology and molecular underpinnings of the effects of bariatric surgery on obesity and diabetes. Annu Rev Physiol. (2017) 79:313–34. Available online at: https://pubmed.ncbi.nlm.nih.gov/27912678/
Keywords: bariatric surgery, gut microbiota, obesity, microbiota dynamics, obesity treatment
Citation: Zambrano AK, Paz-Cruz E, Ruiz-Pozo VA, Cadena-Ullauri S, Tamayo-Trujillo R, Guevara-Ramírez P, Zambrano-Villacres R and Simancas-Racines D (2024) Microbiota dynamics preceding bariatric surgery as obesity treatment: a comprehensive review. Front. Nutr. 11:1393182. doi: 10.3389/fnut.2024.1393182
Received: 28 February 2024; Accepted: 18 March 2024;
Published: 03 April 2024.
Edited by:
Jorge Carriel Mancilla, Catholic University of Santiago de Guayaquil, EcuadorReviewed by:
Claudia Maza, Centro Médico Militar, GuatemalaCopyright © 2024 Zambrano, Paz-Cruz, Ruiz-Pozo, Cadena-Ullauri, Tamayo-Trujillo, Guevara-Ramírez, Zambrano-Villacres and Simancas-Racines. This is an open-access article distributed under the terms of the Creative Commons Attribution License (CC BY). The use, distribution or reproduction in other forums is permitted, provided the original author(s) and the copyright owner(s) are credited and that the original publication in this journal is cited, in accordance with accepted academic practice. No use, distribution or reproduction is permitted which does not comply with these terms.
*Correspondence: Ana Karina Zambrano, YW5hemFtYnJhbm8xN0Bob3RtYWlsLmNvbQ==
†These authors have contributed equally to this work
Disclaimer: All claims expressed in this article are solely those of the authors and do not necessarily represent those of their affiliated organizations, or those of the publisher, the editors and the reviewers. Any product that may be evaluated in this article or claim that may be made by its manufacturer is not guaranteed or endorsed by the publisher.
Research integrity at Frontiers
Learn more about the work of our research integrity team to safeguard the quality of each article we publish.