- 1School of Clinical Medicine, Shandong Second Medical University, Weifang People’s Hospital, Weifang, China
- 2Department of Cardiology, Key Laboratory of Cardiopulmonary-Cerebral Resuscitation Research of Weifang, The First Affiliated Hospital of Shandong Second Medical University, Weifang People’s Hospital, Weifang, China
- 3School of Anesthesiology, Shandong Second Medical University, Weifang, China
- 4Department of Emergency Medicine, University of Michigan Medical School, Ann Arbor, MI, United States
Cardiac arrest is a leading cause of death globally. Only 25.8% of in-hospital and 33.5% of out-of-hospital individuals who achieve spontaneous circulation following cardiac arrest survive to leave the hospital. Respiratory failure and acute coronary syndrome are the two most common etiologies of cardiac arrest. Effort has been made to improve the outcomes of individuals resuscitated from cardiac arrest. Magnesium is an ion that is critical to the function of all cells and organs. It is often overlooked in everyday clinical practice. At present, there have only been a small number of reviews discussing the role of magnesium in cardiac arrest. In this review, for the first time, we provide a comprehensive overview of magnesium research in cardiac arrest focusing on the effects of magnesium on the occurrence and prognosis of cardiac arrest, as well as in the two main diseases causing cardiac arrest, respiratory failure and acute coronary syndrome. The current findings support the view that magnesium disorder is associated with increased risk of cardiac arrest as well as respiratory failure and acute coronary syndrome.
1 Introduction
Cardiac arrest is a leading cause of death globally. In the United States, according to the American Heart Association (AHA), 146,942 individuals experience out-of-hospital cardiac arrest (OHCA) and 292,000 individuals experience in-hospital cardiac arrest (IHCA) annually (1). A recent report by the baseline investigation of out-of-hospital cardiac arrest (BASIC-OHCA) registry estimated that emergency medical service-assessed OHCAs in China have reached more than 750,000 per year (2). The two most common etiologies of cardiac arrest are respiratory failure and acute coronary syndrome (ACS). Respiratory failure accounts for the largest proportion (22%) of IHCA and the second largest proportion (12%) of OHCA. ACS and other cardiac causes account for the largest proportion (16%) of OHCA and are the third leading cause (8%) of IHCA (1, 3). Only 25.8% of IHCA and 33.5% of OHCA patients who have a return of spontaneous circulation (ROSC) following cardiac arrest survive to leave the hospital (4–6). Post-cardiac arrest syndrome is responsible for the high mortality rate among those who achieve ROSC following cardiac arrest. Post-cardiac arrest syndrome includes the following four components: (1) brain injury following cardiac arrest, (2) myocardial dysfunction following cardiac arrest, (3) systemic ischemia/reperfusion response, and (4) ongoing precipitating pathology (7, 8). There is currently no effective therapeutic approach to protect against post-cardiac arrest syndrome.
Magnesium is the second most abundant intracellular cation, which is essential for every organ in the human body. Magnesium participates in practically every major cellular metabolic and biochemical process including polynucleotide binding, enzymatic reactions, cell signaling, and cell proliferation (9). Research continues to examine how magnesium affects cardiac arrest. An early study found that a solution containing high concentrations of potassium and magnesium along with adenosine triphosphate, creatine phosphate, and procaine, rapidly induced arrest of isolated rat hearts and increased the resistance to periods of transient ischemia (10). Related to this, severe magnesium deficiency induced by a high-protein diet was associated with cardiac arrest in rats (11). Since then, increasing evidence has highlighted the importance of magnesium in cardiac arrest. The objectives of this comprehensive review are discussing the impact of magnesium on incidence and prognosis following cardiac arrest. Additionally, we investigate the role of magnesium in the two main diseases causing cardiac arrest, respiratory failure and ACS (Figure 1). The mechanisms by which magnesium exerts its effects are also discussed (Figures 2, 3).
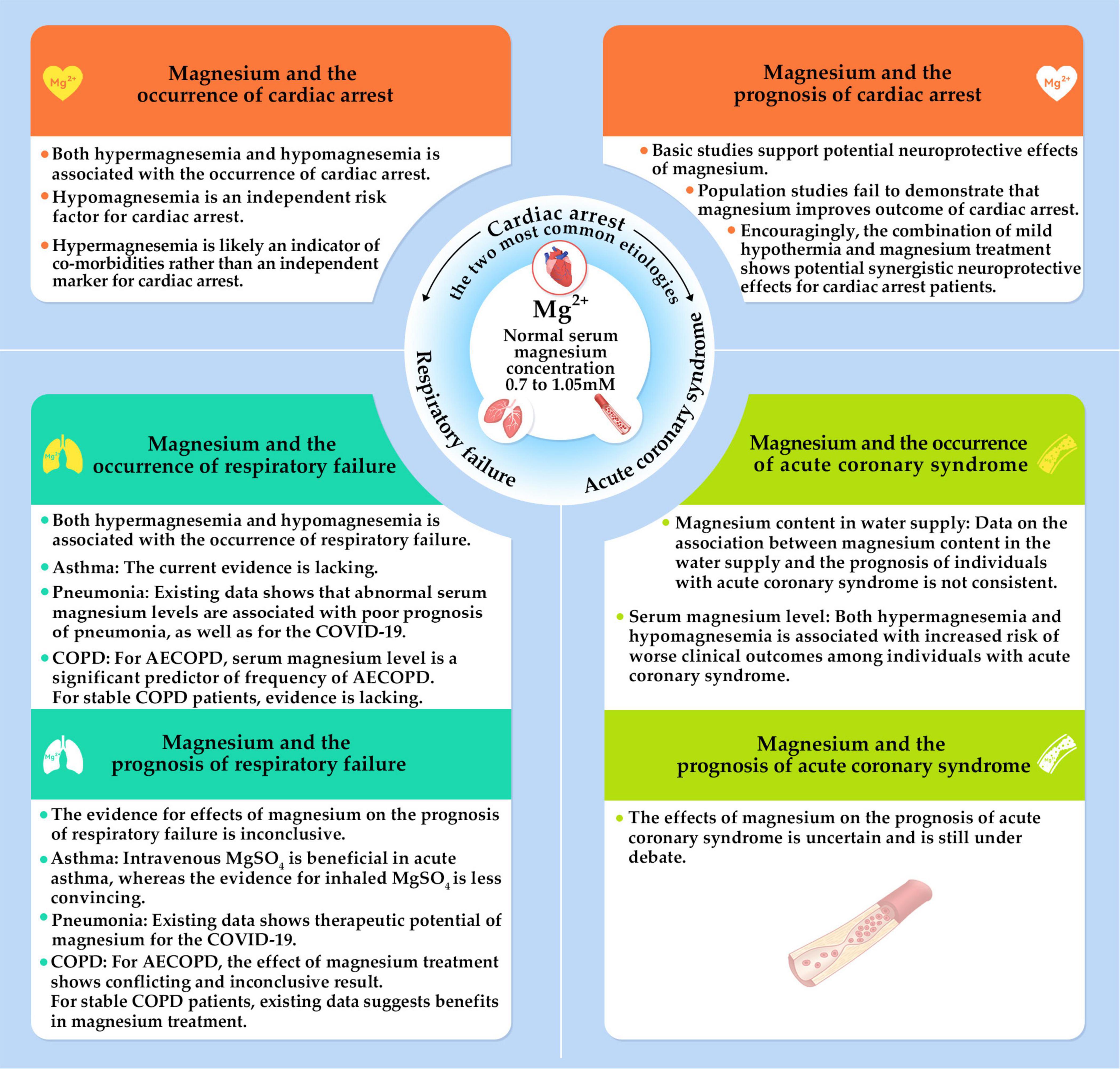
Figure 1. The impact of magnesium on the incidence of and prognosis after cardiac arrest. It also discusses the two most common etiologies of cardiac arrest, which are acute coronary syndrome and respiratory failure. AECOPD, acute exacerbation of chronic obstructive pulmonary disease.
2 Magnesium and cardiac arrest
2.1 Magnesium and the occurrence of cardiac arrest
Both hypermagnesemia and hypomagnesaemia are associated with cardiac arrest. Total serum magnesium concentration in healthy adults ranges from 0.7 to 1.05 mM (9). Hypermagnesemia usually occurs when creatinine clearance decreases in renal dysfunction and in instances of excessive magnesium intake from dietary supplements or magnesium containing medication. Common causes of hypomagnesaemia include a magnesium-insufficient diet, reduced intestinal absorption of magnesium, and the use of diuretics which increases the urinary excretion of magnesium (12).
Both case reports and clinical studies have discussed the relationship between levels of serum magnesium and the risk of cardiac arrest. Indeed, cardiac arrest occurred as a result of magnesium sulfate overdose for managing eclampsia (13, 14). Cardiac arrest occurred after laxative-induced hypermagnesemia in an individual with anorexia nervosa and chronic renal failure (15). Moreover, cardiac arrest was noted in an individual with emotional stress and torsade de pointes associated with hypomagnesemia (16). Clinical studies show that low serum magnesium level is a risk predictor of cardiac arrest. Results are conflicting concerning an association between hypermagnesemia and cardiac arrest. The Atherosclerosis Risk in Communities Study with subjects of 45–64 years old (n = 14,232) found that the risk of sudden cardiac death was higher among individuals who were in the lowest quartile (≤ 1.5 mEq/L) than those who were in the highest quartile (≥ 1.75 mEq/L) of the normal range of serum magnesium (17). Supporting these results was a prospective population-based study with a median follow-up of 8.7 years, with 9,820 participants, that showed low serum magnesium (≤ 0.80 mmol/L) was associated with an increased risk of sudden cardiac death (hazard ratio [HR]: 1.54; 95% confidence interval [CI]: 1.12–2.11; n = 217). The association between sudden cardiac death and high serum magnesium levels, however, was only marginally insignificant (HR: 1.35; 95% CI: 0.96–1.89) (18). The relationship between magnesium and the risk of sudden cardiac death, as measured by diet and plasma in 88,375 healthy women, was investigated in another prospective study. According to the results, higher dietary magnesium intake and plasma concentrations corresponded to reduced risks of sudden cardiac death (19). A systematic review that included eight studies encompassing 13,539 individuals with heart failure, examined the relationship between levels of serum magnesium with cardiovascular and all-cause mortality (12). An independent risk factor for sudden cardiac death is hypomagnesemia. Hypermagnesemia (serum magnesium > 2.4 mg/dl), however, was revealed an indicator for comorbidities but was not an independent marker of cardiovascular mortality (12).
The major mechanism of magnesium deficiency related with occurrence of cardiac arrest is considered to be the cardiac dysrhythmia, that magnesium deficiency alters the intracellular/extracellular K+ ratio resulting in a disturbance in membrane excitability due to an alteration of the resting membrane potential (20). In turn, this promotes cardiac arrest. Additionally, low levels of serum magnesium correspond to disturbances in endothelial function and vascular tone as well as to inflammation. This also may contribute to atherosclerosis development and progression, potentially causing increased heart disease mortality (Figure 2) (18). The discrepancies in the relationship between hypermagnesemia and cardiac arrest risk could be from underappreciated effects of kidney function (18). Hypermagnesemia may encourage hypotension and cardiac arrhythmias. According to recent evidence, however, increased mortality may not be attributed to hypermagnesemia as an underlying cause. It is likely to reveal comorbidities that have an impact on the prognosis of people with cardiovascular disease (12).
2.2 Magnesium and the prognosis of cardiac arrest
Considering the severe consequences of magnesium deficiency in cardiac arrest and the important electrophysiological functions of normal magnesium concentrations, magnesium treatment has been explored to improve the prognosis of cardiac arrest, such as successful resuscitation, short- or long-term survival, and neurological outcome. Here, we summarize studies on magnesium and the prognosis of cardiac arrest in basic studies, clinical studies, and the combined effect of magnesium and hypothermia. Current basic studies supported potential neuroprotective effects of magnesium. However, population studies failed to demonstrate that magnesium sulfate (MgSO4) improved cardiac arrest short- or long-term survival. Encouragingly, the combination of mild hypothermia and magnesium treatment showed potential synergistic neuroprotective effects for individuals with cardiac arrest.
In rats, MgSO4 reduced cerebral injury and preserved neurologic function when administered 2 days before transient global ischemia (21). Moreover, pre-treatment with MgSO4 was effective in resuscitation from hypoxia-induced cardiac arrest. This study showed that MgSO4 was beneficial because of its antiarrhythmic action during reperfusion by preventing asystole and ventricular fibrillation (22). Our group examined the single-dose intramuscular MgSO4 during cardiopulmonary resuscitation (CPR) in a rat model of asphyxia cardiac arrest. We explored the dose–response effect on levels of serum magnesium, neurologic function recovery, long-term survival, and neuronal loss (5). We assumed that the magnesium reduced neuronal calcium overload by directly modulating the N-methyl-daspartate receptor, preventing mitochondrial permeability transition induced by calcium, and decreasing the overload of mitochondrial calcium. A two- to fourfold increase in serum levels of magnesium was achieved within 15 min of ROSC by intramuscular MgSO4 during CPR. This result did not improve long-term survival, neurologic function recovery, or loss of neurons in the CA1 hippocampus. Intramuscular MgSO4, however, did increase the rate of 24-h survival. This result supports using intramuscular MgSO4 therapy during cardiac arrest and suggests that a single intramuscular MgSO4 dose may not be enough to block long-term injury pathway. In the future, whether repeated dosing strategies of MgSO4 or in combination therapy with targeted temperature management (TTM) may provide a more effective level of neuroprotection in cardiac arrest requires further investigations.
A prospective, placebo-controlled, randomized, double-blind trial of magnesium in OHCA, the MAGIC trial, found that as a first-line drug therapy, 5 g MgSO4 did not improve survival significantly (23). For IHCA, empirical magnesium supplementation (2 g bolus, followed by 8 g over 24 h) did not improve the resuscitation rate, 24-h survival, or survival to leaving the hospital in any subpopulation of patients with IHCA or overall (24). In particular, for prehospital individuals suffering cardiac arrest presenting with ventricular fibrillation, administration of 2 g of MgSO4 intravenous bolus during CPR failed to improve short- or long-term survival (25). A placebo-controlled, randomized, double-blind clinical trial featuring a factorial design evaluated whether diazepam, magnesium, or both could improve neurologic outcomes when administered immediately following resuscitation from OHCA. The results did not show a significant difference between the placebo and magnesium groups, although the people who received 2 g MgSO4 did experience a higher percentage of independence in daily living than the placebo controls (26). The inadequate number of individuals/group (75) was considered a factor limiting the power to detect a clinically important outcome difference. Additionally, systematic reviews did not show positive results (27–29). Therefore, there is currently no established recommendation that has be made based on these results for magnesium therapy in cardiac arrest, other than in cases in which hypomagnesemia is suspected or proven to have caused cardiac arrhythmias (30).
Targeted temperature management now has class I recommendations from the AHA for use in individuals post-ROSC. Still, significant controversies and questions remain in its implementation, including timing, target temperature, duration, method, rewarming, and side effects such as shivering, hypotension, hyperglycemia, hypokalemia, and infection (31). Based on the concept that postischemic hypothermia can have a synergistic effect to boost or unmask the neuroprotective effect of an agent (32–34), the combination effect of magnesium with TTM has been investigated in cardiac arrest. In rats, pre-treatment with magnesium before transient global ischemia was neuroprotective only in situations of mild hypothermia (35°C) (35). The use of a combination of pre- and postischemic magnesium combined with modest hypothermia (35°C) at different intervals has also been explored. Notably, postischemic treatment with magnesium and modest hypothermia (35°C) for 24 h decreased CA1 neuronal death more effectively than either treatment alone (36, 37). Clinical studies of combination therapy of magnesium with TTM in cardiac arrest are scant. Analysis of 438 survivors of cardiac arrest who completed a therapeutic hypothermia protocol, showed that lower magnesium levels at presentation and during therapeutic hypothermia were associated with favorable outcomes of cardiac arrest. Moreover, magnesium supplementation during the hospital stay was associated with improved neurological outcomes, suggesting that magnesium supplementation may potentiate the beneficial effects of therapeutic hypothermia (38). Several mechanisms explain why magnesium and hypothermia appear to act synergistically. First, both have multiple mechanisms of action following ischemia. Magnesium, for example, may maintain or increase levels of brain magnesium, thus restoring cellular parameters such as adenosine triphosphate (ATP) production, intracellular calcium, protein synthesis, and mitochondrial integrity. Meanwhile, hypothermia may restrain damage caused by free radical production, blood–brain barrier breakdown, and inflammation after ischemia (36). Furthermore, as reported in other studies which combined pharmacotherapy and hypothermia (32, 34, 39), magnesium and hypothermia therapy may prolong the therapeutic window, which supports beginning treatment several hours after cerebral ischemia. Additionally, magnesium may alleviate the side effects of hypothermia, such as reducing the shivering threshold, increasing the cooling rate, and reducing discomfort during surface cooling to 34–35°C (37, 40, 41). However, whether the combined treatment of magnesium and hypothermia could provide beneficial effects for individuals following cardiac arrest remains unclear and deserves further investigation.
3 Magnesium and diseases causing cardiac arrest
3.1 Magnesium and respiratory failure
Respiratory failure is the most common etiology of cardiac arrest (15%), which is the result of primary pulmonary pathology, including hypercarbia or hypoxia caused by exacerbation of asthma, chronic obstructive pulmonary disease (COPD), or multifocal pneumonia (3). The Global Burden of Diseases, Injuries, and Risk Factors Study (2019) found that chronic respiratory diseases, with a prevalence of 454.6 million cases globally, were responsible for 4 million deaths, making it the third leading cause of mortality worldwide (42). The five most significant lung diseases globally are asthma, COPD, lung cancer, pneumonia, and tuberculosis (43). Several treatment strategies have been studied to ameliorate symptoms, limit adverse outcomes, and increase quality of life.
Magnesium is proposed as an additive treatment for respiratory diseases because of a variety of mechanisms. Magnesium takes part in cellular homeostasis by serving as an enzymatic cofactor and involving in release of acetylcholine and histamine from mast cells and cholinergic nerve terminals. Magnesium inhibits contraction and relaxes smooth muscle by blocking calcium ion influx into the smooth muscle of respiratory system (44–46). Moreover, magnesium may regulate anti-inflammatory and antioxidant responses and assist in the proper functioning of micronutrients (e.g., vitamin D). Magnesium also plays a role in activating T cells (Figure 3). Thus, magnesium deficiency can trigger immunodeficiency, decrease antioxidant responses, exaggerate acute inflammatory response, and cause oxidative stress of respiratory system, which has been discussed widely in a recent review (47). Here, we summarize findings on the role of magnesium in respiratory failure, as well as in respiratory diseases responsible for respiratory failure, including asthma, pneumonia, and COPD in which magnesium is mostly explored.
3.1.1 Magnesium and the occurrence and prognosis of respiratory failure
Magnesium is involved in the development and prognosis of respiratory failure. A significant body of research has examined the relationship between levels of serum magnesium and respiratory failure. Because magnesium can desensitize the postsynaptic membrane and inhibit the presynaptic release of acetylcholine by acting at the neuromuscular junction, it may exacerbate or cause respiratory failure and muscle weakness (48, 49). Hypermagnesemia has been responsible for prolonged respiratory failure and total flaccid paralysis when supplementing oral magnesium in the case of acute kidney injury. This result has revealed that electrolyte abnormalities may go underrecognized and lead to respiratory failure (50). When treated by magnesium replacement, a patient with hypomagnesemia and myasthenia gravis developed acute respiratory failure (48). Similarly, in another situation of myasthenia gravis and atrial fibrillation, intravenous magnesium replacement induced respiratory failure (49). Although limited, the results of these studies indicate that magnesium should be avoided or administered carefully in patients who have borderline respiratory function or myasthenia gravis.
A retrospective, single-center study examined acute respiratory failure in the case of mechanical ventilation in patients who had been hospitalized with different levels of magnesium at admission. The lowest incidence of acute respiratory failure that needed mechanical ventilation was found when levels of serum magnesium were 1.7–1.9 mg/dL. Admission hypomagnesemia (< 1.5 mg/dL) and hypermagnesemia (> 2.3 mg/dL) were both related to an increase in the risk of acute respiratory failure (51). The mechanism that hypomagnesemia causes respiratory dysfunction is that the amount of acetylcholine released from nerve terminals is decreased. Hypermagnesemia diminishes the depolarizing action of acetylcholine in the neuromuscular junction, therefore causing the bronchial smooth muscles to weaken and relax (51). The effects of different levels of magnesium on patients in the intensive care unit (ICU) with acute respiratory failure have also been studied. Neither hypomagnesemia nor normomagnesemia had a significant impact on the duration of stay in the ICU or the duration of mechanical ventilation. Statistically, however, the mortality rate of patients who had hypermagnesemia was higher than that of patients with other levels of magnesium (P = 0.018) (52). Given data limitations, additional research is needed to confirm these results, and cut-off values for magnesium should be further evaluated for patients who have respiratory failure.
3.1.2 Magnesium and asthma
According to the latest report in 2019, asthma was the most common chronic respiratory disease with 262.4 million cases worldwide (42). To treat acute asthma, the primary therapeutic goals include reversing bronchospasm and correcting hypoxemia. Initial and conventional pharmacologic treatment of acute asthma calls for administering inhaled short-acting anticholinergic, β2-agonist and systemic corticosteroids (53). By blocking parasympathetic tone and inhibiting calcium channels, magnesium can act as a bronchodilator when administered intravenously and by inhalation. This treatment may serve as an adjunct therapy for acute asthma (53). Recent research results indicate that intravenous MgSO4 may improve acute asthma; however, inhaled MgSO4 may be less beneficial.
As an adjunct for acute asthma, intravenous magnesium has been studied with a long history (45, 54–60). To date, intravenous MgSO4 appears to be safe, and intravenous magnesium sulfate is considered for use in patients who have severe exacerbations (45, 54, 56, 58, 61, 62). The 2007 Cochrane review by Mohammed and Goodacre estimated the benefit of adding MgSO4 to β-agonists and systemic corticosteroids in children and adults who had acute asthma. They assessed 24 studies with 1,669 individuals, 15 of which included intravenous treatment and 9 included nebulized treatment (55). According to the results, intravenous MgSO4 treatment in adults provided only weak evidence for improving respiratory function and had no significant effect on hospital admission. Intravenous MgSO4 treatment in children showed reduced hospital admission and a significant improvement in respiratory function. In adults, only weak evidence was found of the effect of nebulized treatment on hospital admission or respiratory function, and in children, no significant effect was found on hospital admission or respiratory function (55). A 2008 review found that, when considering all studies, in the case of severe exacerbations, adding MgSO4 improved pulmonary function and reduced hospitalization (45). Another review focusing on children demonstrated that this age group was clearly benefited from intravenous MgSO4 (63).
For inhaled magnesium sulfate, a series of updated reviews by Rowe et al. investigated its effects for treating acute asthma from 2005 to 2017, with consistent results (44, 64, 65). The recent review including 25 trials and 2,907 randomized individuals examined whether inhaled MgSO4 had beneficial effects (1) when combined with ipratropium bromide and inhaled β2-agonist; (2) when added to inhaled β2-agonist; and (3) when compared with inhaled β2-agonist (65). However, the results did not demonstrate substantial benefits of nebulized MgSO4 for improved lung function or reduced hospital admission for acute asthma. Trial heterogeneity may limit the strength of the results (65). A meta-analysis of children who have acute asthma included eight randomized controlled trials (1,247 children). The findings did not suggest that inhaled magnesium offered a substantial benefit to improve lung function, limit hospital admissions, or reduce asthma symptoms or severity scores (66). A clinical trial recently published in JAMA also did not support any beneficial effects for children who had refractory acute asthma. When comparing nebulized magnesium with albuterol and a placebo with albuterol to treat asthma, the addition of magnesium did not decrease the hospitalization rate significantly within 24 h (67). As a result, these findings do not suggest that nebulized magnesium should be used alone or with albuterol in children who have refractory acute asthma.
3.1.3 Magnesium and pneumonia
Pneumonia affects hundreds of millions of people globally. Because of the aging population, the number of hospital admissions of pneumonia has been increasing over recent decades (68, 69). The changes in serum magnesium levels in pneumonia and the impact of magnesium levels on the prognosis of pneumonia have been studied, with only limited evidence. During pneumonia, the serum magnesium level deviated markedly from the normal, while a definite loss of magnesium to the body was observed during the febrile period of pneumonia (70). Hypomagnesemia was also common in community-acquired pneumonia in individuals with type 2 diabetes mellitus. In these individuals, hypomagnesemia at admission was associated with increased short-term mortality (71). In elderly patients with community-acquired pneumonia, abnormal serum magnesium levels corresponded significantly to in-hospital mortality and 30-day mortality (68, 72). However, interventional studies are lacking to confirm the effects of magnesium treatment on pneumonia outcomes.
The coronavirus (COVID-19) pandemic, which is caused by SARS-CoV-2 infection, has brought a heavy burden to our economic and healthcare systems (73). As a result, great efforts have been made to better understand its pathogenesis and to implement low-cost prophylactic interventions. Given the multiple effects of magnesium such as anti-inflammatory and antioxidant effects, it is assumed to play a significant role in COVID-19 development and mortality (47, 73–76). Results from clinical studies found that the need for mechanical ventilation and mortality rates were higher in hypermagnesemia (magnesium levels > 2.4 mg/dL) and hypomagnesemia (<1.8 mg/dL) patients than in other patients who were hospitalized with COVID-19 (77, 78). The COMEPA study found that the low level of serum magnesium was a significant indicator of the onset of long COVID symptoms, length of stay, and in-hospital mortality (79). Levels of serum magnesium and myocardial damage were significantly negatively correlated (80). These findings indicate that measuring levels of serum magnesium in patients with COVID-19 may help predict disease-related complications. A cross-sectional study revealed that taking more dietary magnesium was inversely correlated with the severity and symptoms of COVID-19 (81). An observational study of cohorts found that combining magnesium, vitamin D, and vitamin B12 decreased the number of older patients with COVID-19 who were experiencing clinical deterioration that needed oxygen, intensive care, or both (82). Overall, current research supports the relationship between COVID-19 and imbalanced magnesium homeostasis. Therefore, robust studies are needed to investigate the therapeutic or prophylactic potential of magnesium for COVID-19 (74).
3.1.4 Magnesium and chronic obstructive pulmonary disease
The primary cause of death from chronic respiratory diseases was COPD, with 212.3 million prevalent cases and 3.3 million deaths in 2019 (42). The potential clinical benefits of intravenous or nebulized MgSO4 for individuals with acute exacerbations or stable COPD have been studied; however, existing studies have found conflicting and inconclusive results for its effectiveness.
In patients who have acute exacerbations of COPD, serum magnesium levels were a significant predictor of the frequency of acute exacerbations, emphasizing the importance of detecting magnesium levels in individuals with COPD (83, 84). The effect of magnesium treatment on outcomes of acute COPD exacerbations has been investigated by meta-analyses. One study found that intravenous MgSO4 corresponded to improving dyspnea scores, reducing hospital admissions, and limiting hospital length of stay. However, no significant difference in the necessity for non-invasive ventilation was indicated, nor was a change in oxygen saturation or pulmonary function testing found (85). A Cochrane systematic review showed that magnesium infusion improved dyspnea scores and decreased the duration of hospital stay. Yet it was not clear if there was any effect on improving lung function or oxygen saturation. In contrast, magnesium inhalation, compared to placebo, did not make a difference in most outcomes of COPD exacerbations. In addition, when MgSO4 was compared to ipratropium bromide no differences in outcomes were noted (86). Several other systematic reviews have found either no benefit or inconclusive effects of treating acute COPD exacerbation with magnesium sulfate (87, 88). The conflicting results may be the result of the limited number of participants in these studies, as well as clinical heterogeneities in the patient populations and medication dosages and routes.
For individuals with stable COPD, several studies suggested benefits in magnesium treatment. One study showed that serum magnesium levels were associated with quality of life but not with lung function in individuals with COPD (89). Oral magnesium supplementation showed potential anti-inflammatory effects, however, this did not involve influence on lung function, physical performance, or quality of life in individuals with stable COPD (90). In patients with stable COPD, intravenous magnesium loading was correlated with decreased lung hyperinflation and improved respiratory muscle strength (91, 92). Therefore, the potential benefits of clinical magnesium supplementation for COPD warrants further study.
3.2 Magnesium and ACS
Acute coronary syndrome, the acute manifestation of ischemic heart disease, is a major cause of cardiac arrest (12%). In 2019, there were an estimated 673,000 ACS principal diagnostic discharges in the United States (1). Substantial progress has been made to prevent, diagnose, and treat people with ACS. Still, the burden of ACS remains unacceptably high. This situation calls for a reappraisal of the ACS related mechanisms and exploration of novel treatment therapies (1, 93). Theoretically, magnesium offers several benefits for the cardiovascular system, such as platelet aggregation and coagulation, vascular tone, endothelial function, lipid metabolism, infarct size, and cardiac arrhythmias (Figure 3), which have been discussed detailly (94–96). Here, we summarize studies on the association of magnesium with ACS prognosis from three aspects, including magnesium content in the water supply, serum magnesium levels, and magnesium administration, on the prognosis of ACS.
The relationship between magnesium content in the water supply and the prognosis of individuals with ACS, especially mortality from acute myocardial infarction (AMI), has found inconsistent results. According to data of subjects residing in England, lacked evidence of a relationship between mortality from AMI and levels of magnesium in drinking water supplies was found (97). Data from individuals in China indicated that calcium intake provided a protective effect on AMI mortality. In this case, however, no significant difference was reported in AMI mortality among the groups that had different levels of magnesium (98). In Israel, the 30-day and one-year all-cause mortality of individuals with AMI was higher in the people who were exposed to desalinated seawater, which lacks magnesium, which may reveal the contribution of reduced magnesium intake on AMI mortality (99). These people also had significantly lower levels of serum magnesium than the people who were exposed to non-desalinated drinking water. These conflicting findings could be the result of geographic differences, variance in serum magnesium levels, and confounding factors such as intake situations of magnesium supplements or medicines. Therefore, better tracking serum magnesium levels may inform the role of magnesium in ACS prognosis.
Hypermagnesemia and hypomagnesemia are correlated with an increase in worsened clinical outcomes among individuals who experience ACS. A retrospective multicenter study included more than 10,000 people with AMI and found that levels of serum magnesium were correlated with in-hospital mortality and malignant arrhythmias in a U-shaped manner (< 1.8, 1.8–1.9, 1.9–2.0, and > 2.0 mg/dL vs. 7.4, 4.1, 4.7, and 9.7%, respectively) (100). These findings show that the optimal range of serum magnesium in people with AMI may be lower than current AMI recommendations (> 2.0 mg/dL) (101). Higher serum magnesium levels at admission in individuals with reperfused AMI complicated by malignant ventricular arrhythmias were independently related with in-hospital mortality (HR: 2.68, 95% CI: 1.24–5.80). Additionally, the number of in-hospital adverse events, such as extracorporeal membrane oxygenation, cardiogenic shock necessitating intra-aortic balloon pump, persistent vegetative state or tracheostomy, and tracheal intubation, was much higher in patients with higher levels of serum magnesium than in people who had lower levels of serum magnesium (102). Since only one individual with hypomagnesemia was included, a U-shaped relationship was not observed in this study. Similarly, admission levels of serum magnesium, regardless of other risk factors, were related inversely to major adverse cardiovascular events in people who had a drug-eluting stent implantation for AMI but stable angina. Individuals with the highest levels of serum magnesium (> 0.94 mmol/L) compared with people with the lowest levels (< 0.86 mmol/L) had an 8.11-fold higher risk of major adverse cardiovascular events following implantation of a drug-eluting stent (103). A retrospective study assessed the impact of different levels of serum magnesium at admission on in-hospital mortality, including sudden cardiac death and QTc interval, in people who were admitted to the cardiac care unit with acute decompensated heart failure or a primary AMI diagnosis. A level of serum magnesium ≥ 2.4 mg/dL independently indicated an increase in hospital mortality. However, no relationship was found between levels of serum magnesium and an interval of QTc or sudden cardiac death (104). The pathophysiologic effects of hypermagnesemia and hypomagnesemia on the cardiovascular system are similar to those on cardiac arrest. However, the pathophysiologic mechanisms of magnesium remain to be addressed in individuals with ACS with different complications, including ventricular arrhythmias, drug-eluting stent implantation, and heart failure. Furthermore, some limitations need to be considered when elucidating the results: (1) the time between blood tests and administration of magnesium should be clarified; (2) measurement of serum magnesium should be uniform to allow for better comparison of results; and (3) the ability to generalize results and the statistical power to detect data differences has been limited by small sample sizes from single-center studies (102, 103).
It is still under debate whether the administration of magnesium may affect the prognosis of ACS. The Second Leicester Intravenous Magnesium Intervention Trial (LIMIT-2) was a double-blind, randomized, placebo-controlled study that included 2,316 individuals with suspected AMI and verified the benefits of magnesium (105). Notably, the administration of intravenous magnesium sulfate significantly decreased the 28-day mortality of people with AMI versus the placebo group. The Fourth International Study of Infarct Survival (ISIS-4), which was a randomized factorial trial of more than 50,000 participants, however, did not find a decrease in five-week mortality or later survival advantage among the magnesium treatment group versus the placebo group (both overall or in subgroups) (106). The proposed explanations for discrepancies between the two studies included variation in the timing and duration of treatment, the dose of magnesium, concomitant thrombolysis, and methodological problems (107). In a meta-analysis of 26 clinical trials, no advantages were found as a result of early or late magnesium treatment and outcomes (107). Although magnesium appeared to decrease the incidence of ventricular tachycardia, ventricular fibrillation, and severe arrhythmia needing treatment, it may have increased the incidence of bradycardia, flushing, and profound hypotension (107). Concerning post-ACS cardiac arrhythmias, several meta-analyses showed significant fewer reperfusion arrhythmias in the magnesium treatment groups (108–114). A recent retrospective analysis, however, revealed that magnesium did not effectively lower the incidence of reperfusion arrhythmia in people with ST-elevation myocardial infarction, who underwent primary percutaneous coronary intervention (115). One explanation for inconsistent results of this work may be the variety of revascularization techniques employed, including cardiac surgery, thrombolysis, and percutaneous coronary intervention.
4 Study limitations
This study has potential limitations. First, while the review covers a broad range of studies, the generalizability of these findings to different populations (e.g., different age groups, ethnic backgrounds, or geographic locations) may be limited. This could be an important limitation to acknowledge. Second, the quality of the evidence from the studies reviewed might vary. Including a critical assessment of the quality of the studies (e.g., risk of bias, methodological strengths and weaknesses) could enhance the understanding of how robust the current evidence is. Third, while the review discusses associations between magnesium levels and cardiac arrest outcomes, it is important to acknowledge that these associations might be influenced by confounding factors, such as other underlying health conditions, lifestyle factors, or concurrent treatments. Additionally, the long-term effects of magnesium therapy in cardiac arrest patients are not thoroughly discussed. The review could benefit from addressing the need for long-term follow-up studies to understand the sustained impact of magnesium. Finally, while the review outlines potential mechanisms by which magnesium impacts cardiac arrest, deeper mechanistic insights or the need for more research in this area could be a valuable addition.
5 Conclusion
Magnesium is an important intracellular cation that is critical for the physiological function of multiple organs including the brain, heart, and skeletal muscles. Magnesium is being tested as a treatment for cardiac arrest. This review summarized the role that magnesium can play in cardiac arrest. We assessed early research and clinical data published to date and found that a magnesium disorder indicates an increased risk of cardiac arrest as well as ACS and respiratory failure. This finding demonstrates the need for daily clinical practice to verify levels of serum magnesium. Magnesium appears to be mostly safe as an adjuvant therapy in the setting of cardiac arrest. Early results of a combination of mild hypothermia and magnesium indicate that this promising treatment may benefit individuals who are resuscitated from cardiac arrest. It continues to be controversial, however, whether or not magnesium should be used in people who are experiencing cardiac arrest as well as ACS or respiratory failure. Rigorous large cohort multi-center trials may help to clarify these issues.
Author contributions
BL: Conceptualization, Funding acquisition, Supervision, Validation, Writing – original draft, Writing – review & editing. ML: Data curation, Validation, Writing – original draft. JW: Conceptualization, Validation, Writing – original draft. FZ: Data curation, Validation, Writing – original draft. FW: Data curation, Validation, Writing – original draft. CJ: Data curation, Validation, Writing – review & editing. JL: Data curation, Validation, Writing – review & editing. YW: Data curation, Validation, Writing – review & editing. TS: Conceptualization, Supervision, Validation, Writing – review & editing. RZ: Conceptualization, Funding acquisition, Supervision, Validation, Writing – original draft, Writing – review & editing.
Funding
The authors declare that financial support was received for the research, authorship, and/or publication of this article. This research was funded by the Natural Science Foundation of Shandong Province [ZR2020QH005, ZR2021QH262, and ZR2022QH142]; the National Natural Science Foundation of China [82202427]; the Science and Technology Development Program of Weifang Medical University [2023FYQ006]; and the Science and Technology Development Program of Weifang [2022YX082].
Acknowledgments
We thank LetPub (www.letpub.com) for linguistic assistance and pre-submission expert review.
Conflict of interest
The authors declare that the research was conducted in the absence of any commercial or financial relationships that could be construed as a potential conflict of interest.
Publisher’s note
All claims expressed in this article are solely those of the authors and do not necessarily represent those of their affiliated organizations, or those of the publisher, the editors and the reviewers. Any product that may be evaluated in this article, or claim that may be made by its manufacturer, is not guaranteed or endorsed by the publisher.
References
1. Tsao CW, Aday A, Almarzooq Z, Anderson C, Arora P, Avery C, et al. Heart disease and stroke statistics-2023 update: A report from the American Heart Association. Circulation. (2023) 147:e93–621. doi: 10.1161/CIR.0000000000001137
2. Zheng J, Lv C, Zheng W, Zhang G, Tan H, Ma Y, et al. Incidence, process of care, and outcomes of out-of-hospital cardiac arrest in China: A prospective study of the BASIC-OHCA registry. Lancet Public Health. (2023) 8:e923–32. doi: 10.1016/S2468-2667(23)00173-1
3. Chen N, Callaway CW, Guyette FX, Rittenberger JC, Doshi AA, Dezfulian C, et al. Arrest etiology among patients resuscitated from cardiac arrest. Resuscitation. (2018) 130:33–40. doi: 10.1016/j.resuscitation.2018.06.024
4. Benjamin E, Virani S, Callaway C, Chamberlain A, Chang A, Cheng S, et al. Heart disease and stroke statistics-2018 update: A report from the American Heart Association. Circulation. (2018) 137:e67–492. doi: 10.1161/CIR.0000000000000573
5. Zhang R, Bryson T, Fogo G, Liao J, Raghunayakula S, Mathieu J, et al. Rapid treatment with intramuscular magnesium sulfate during cardiopulmonary resuscitation does not provide neuroprotection following cardiac arrest. Mol Neurobiol. (2022) 59:1872–81. doi: 10.1007/s12035-021-02645-x
6. Shi X, Wu Y, Li H, Ma S, Li D, Gao D, et al. Outcome of bystander cardiopulmonary resuscitation after out-of-hospital cardiac arrest in Beijing. Emerg Crit Care Med. (2021) 1:64–9. doi: 10.1097/EC9.0000000000000002
7. Neumar R, Nolan J, Adrie C, Aibiki M, Berg R, Böttiger B, et al. Post-cardiac arrest syndrome: Epidemiology, pathophysiology, treatment, and prognostication. A consensus statement from the International Liaison Committee on Resuscitation (American Heart Association, Australian and New Zealand Council on Resuscitation, European Resuscitation Council, Heart and Stroke Foundation of Canada, InterAmerican Heart Foundation, Resuscitation Council of Asia, and the Resuscitation Council of Southern Africa); the American Heart Association Emergency Cardiovascular Care Committee; the Council on Cardiovascular Surgery and Anesthesia; the Council on Cardiopulmonary, Perioperative, and Critical Care; the Council on Clinical Cardiology; and the Stroke Council. Circulation. (2008) 118:2452–83.
8. An L, Qi Z, Shao H, Li C. Functional changes in the hypothalamic-pituitary-adrenal axis after successful cardiopulmonary resuscitation. Emerg Crit Care Med. (2023) 3:46–50. doi: 10.1097/EC9.0000000000000074
9. de Baaij JH, Hoenderop JG, Bindels RJ. Magnesium in man: Implications for health and disease. Physiol Rev. (2015) 95:1–46. doi: 10.1152/physrev.00012.2014
10. Hearse DJ, Stewart DA, Braimbridge MV. Cellular protection during myocardial ischemia: The development and characterization of a procedure for the induction of reversible ischemic arrest. Circulation. (1976) 54:193–202. doi: 10.1161/01.CIR.54.2.193
11. Fouty RA. Liquid protein diet magnesium dificiency and cardiac arrest. JAMA. (1978) 240:2632–3. doi: 10.1001/jama.1978.03290240032015
12. Voultsos P, Bazmpani M, Papanastasiou CA, Papadopoulos CE, Efthimiadis G, Karvounis H, et al. Magnesium disorders and prognosis in heart failure: A systematic review. Cardiol Rev. (2022) 30:281–5. doi: 10.1097/CRD.0000000000000397
13. Rabinerson D, Gruber A, Kaplan B, Royburt M, Ovadia J. Accidental cardiopulmonary arrest following magnesium sulphate overdose. Eur J Obstet Gynecol Reprod Biol. (1994) 55:149–50. doi: 10.1016/0028-2243(94)90071-X
14. Swartjes JM, Schutte MF, Bleker OP. Management of eclampsia: Cardiopulmonary arrest resulting from magnesium sulfate overdose. Eur J Obstet Gynecol Reprod Biol. (1992) 47:73–5. doi: 10.1016/0028-2243(92)90217-M
15. Tatsumi H, Masuda Y, Imaizumi H, Kuroda H, Yoshida S, Kyan R, et al. A case of cardiopulmonary arrest caused by laxatives-induced hypermagnesemia in a patient with anorexia nervosa and chronic renal failure. J Anesth. (2011) 25:935–8. doi: 10.1007/s00540-011-1220-6
16. Grant HI, Yeston NS. Cardiac arrest secondary to emotional stress and torsade de pointes in a patient with associated magnesium and potassium deficiency. Crit Care Med. (1991) 19:292–4. doi: 10.1097/00003246-199102000-00031
17. Peacock JM, Ohira T, Post W, Sotoodehnia N, Rosamond W, Folsom AR. Serum magnesium and risk of sudden cardiac death in the Atherosclerosis Risk in Communities (ARIC) Study. Am Heart J. (2010) 160:464–70. doi: 10.1016/j.ahj.2010.06.012
18. Kieboom B, Niemeijer M, Leening M, van den BM, Franco O, Deckers J, et al. Serum magnesium and the risk of death from coronary heart disease and sudden cardiac death. J Am Heart Assoc. (2016) 5:e002707. doi: 10.1161/JAHA.115.002707
19. Chiuve SE, Korngold EC, Januzzi JL Jr., Gantzer ML, Albert CM. Plasma and dietary magnesium and risk of sudden cardiac death in women. Am J Clin Nutr. (2011) 93:253–60. doi: 10.3945/ajcn.110.002253
20. Hollmann MW, Strumper D, Salmons VA, Washington JM, Durieux ME. Effects of calcium and magnesium pretreatment on hyperkalaemic cardiac arrest in rats. Eur J Anaesthesiol. (2003) 20:606–11. doi: 10.1097/00003643-200308000-00003
21. Sirin BH, Coşkun E, Yilik L, Orta R, Sirin H, Tetik C. Neuroprotective effects of preischemia subcutaneous magnesium sulfate in transient cerebral ischemia. Eur J Cardiothorac Surg. (1998) 14:82–8. doi: 10.1016/S1010-7940(98)00140-7
22. Siemkowicz E. Magnesium sulfate solution dramatically improves immediate recovery of rats from hypoxia. Resuscitation. (1997) 35:53–9. doi: 10.1016/S0300-9572(97)00028-2
23. Fatovich DM, Prentice DA, Dobb GJ. Magnesium in cardiac arrest (the magic trial). Resuscitation. (1997) 35:237–41. doi: 10.1016/S0300-9572(97)00062-2
24. Thel MC, Armstrong AL, McNulty SE, Califf RM, O’Connor CM. Randomised trial of magnesium in in-hospital cardiac arrest. Duke Int Med Housestaff Lancet. (1997) 350:1272–6. doi: 10.1016/S0140-6736(97)05048-4
25. Allegra J, Lavery R, Cody R, Birnbaum G, Brennan J, Hartman A, et al. Magnesium sulfate in the treatment of refractory ventricular fibrillation in the prehospital setting. Resuscitation. (2001) 49:245–9. doi: 10.1016/S0300-9572(00)00375-0
26. Longstreth WT Jr., Fahrenbruch CE, Olsufka M, Walsh TR, Copass MK, Cobb LA, et al. Randomized clinical trial of magnesium, diazepam, or both after out-of-hospital cardiac arrest. Neurology. (2002) 59:506–14. doi: 10.1212/WNL.59.4.506
27. Reis AG, Ferreira de Paiva E, Schvartsman C, Zaritsky AL. Magnesium in cardiopulmonary resuscitation: Critical review. Resuscitation. (2008) 77:21–5. doi: 10.1016/j.resuscitation.2007.10.001
28. Huang Y, He Q, Yang M, Zhan L. Antiarrhythmia drugs for cardiac arrest: A systemic review and meta-analysis. Crit Care. (2013) 17:R173. doi: 10.1186/cc12852
29. Pearce A, Lockwood C, Van Den Heuvel C. The use of therapeutic magnesium for neuroprotection during global cerebral ischemia associated with cardiac arrest and cardiac bypass surgery in adults: A systematic review protocol. JBI Database Syst Rev Implement Rep. (2015) 13:3–13. doi: 10.11124/jbisrir-2015-1675
30. Tangvoraphonkchai K, Davenport A. Magnesium and cardiovascular disease. Adv Chronic Kidney Dis. (2018) 25:251–60. doi: 10.1053/j.ackd.2018.02.010
31. Granfeldt A, Holmberg MJ, Nolan JP, Soar J, Andersen LW, Force International Liaison Committee on Resuscitation (Ilcor) Advanced Life Support Task. Targeted temperature management in adult cardiac arrest: Systematic review and meta-analysis. Resuscitation. (2021) 167:160–72. doi: 10.1016/j.resuscitation.2021.08.040
32. Liu Y, Barks JD, Xu G, Silverstein FS. Topiramate extends the therapeutic window for hypothermia-mediated neuroprotection after stroke in neonatal rats. Stroke. (2004) 35:1460–5. doi: 10.1161/01.STR.0000128029.50221.fa
33. Zausinger S, Scholler K, Plesnila N, Schmid-Elsaesser R. Combination drug therapy and mild hypothermia after transient focal cerebral ischemia in rats. Stroke. (2003) 34:2246–51. doi: 10.1161/01.STR.0000083622.65684.21
34. Zausinger S, Westermaier T, Plesnila N, Steiger HJ, Schmid-Elsaesser R. Neuroprotection in transient focal cerebral ischemia by combination drug therapy and mild hypothermia: Comparison with customary therapeutic regimen. Stroke. (2003) 34:1526–32. doi: 10.1161/01.STR.0000070841.31224.29
35. Zhu H, Meloni BP, Moore SR, Majda BT, Knuckey NW. Intravenous administration of magnesium is only neuroprotective following transient global ischemia when present with post-ischemic mild hypothermia. Brain Res. (2004) 1014:53–60. doi: 10.1016/j.brainres.2004.03.073
36. Zhu H, Meloni BP, Bojarski C, Knuckey MW, Knuckey NW. Post-ischemic modest hypothermia (35 degrees C) combined with intravenous magnesium is more effective at reducing CA1 neuronal death than either treatment used alone following global cerebral ischemia in rats. Exp Neurol. (2005) 193:361–8. doi: 10.1016/j.expneurol.2005.01.022
37. Meloni BP, Campbell K, Zhu H, Knuckey NW. In search of clinical neuroprotection after brain ischemia: The case for mild hypothermia (35 degrees C) and magnesium. Stroke. (2009) 40:2236–40. doi: 10.1161/STROKEAHA.108.542381
38. Perucki WH, Hiendlmayr B, O’Sullivan DM, Gunaseelan AC, Fayas F, Fernandez AB. Magnesium levels and neurologic outcomes in patients undergoing therapeutic hypothermia after cardiac arrest. Ther Hypothermia Temp Manag. (2018) 8:14–7. doi: 10.1089/ther.2017.0016
39. Nito C, Kamiya T, Ueda M, Arii T, Katayama Y. Mild hypothermia enhances the neuroprotective effects of FK506 and expands its therapeutic window following transient focal ischemia in rats. Brain Res. (2004) 1008:179–85. doi: 10.1016/j.brainres.2004.02.031
40. Zweifler RM, Voorhees ME, Mahmood MA, Parnell M. Magnesium sulfate increases the rate of hypothermia via surface cooling and improves comfort. Stroke. (2004) 35:2331–4. doi: 10.1161/01.STR.0000141161.63181.f1
41. Wadhwa A, Sengupta P, Durrani J, Akça O, Lenhardt R, Sessler DI, et al. Magnesium sulphate only slightly reduces the shivering threshold in humans. Br J Anaesth. (2005) 94:756–62. doi: 10.1093/bja/aei105
42. GBD 2019 Chronic Respiratory Diseases Collaborators. Global burden of chronic respiratory diseases and risk factors, 1990-2019: An update from the Global Burden of Disease Study 2019. EClinicalMed. (2023) 59:101936.
43. GBD Chronic Respiratory Disease Collaborators. Prevalence and attributable health burden of chronic respiratory diseases, 1990-2017: A systematic analysis for the Global Burden of Disease Study 2017. Lancet Respir Med. (2020) 8:585–96.
44. Blitz M, Blitz S, Beasely R, Diner B, Hughes R, Knopp J, et al. Inhaled magnesium sulfate in the treatment of acute asthma. Cochrane Database Syst Rev. (2005) 19:CD003898. doi: 10.1002/14651858.CD003898.pub3
45. Rowe BH, Camargo CA Jr. The role of magnesium sulfate in the acute and chronic management of asthma. Curr Opin Pulm Med. (2008) 14:70–6. doi: 10.1097/MCP.0b013e3282f19867
46. Gourgoulianis KI, Chatziparasidis G, Chatziefthimiou A, Molyvdas PA. Magnesium as a relaxing factor of airway smooth muscles. J Aerosol Med. (2001) 14:301–7. doi: 10.1089/089426801316970259
47. Arancibia-Hernandez YL, Aranda-Rivera AK, Cruz-Gregorio A, Pedraza-Chaverri J. Antioxidant/anti-inflammatory effect of Mg(2+) in coronavirus disease 2019 (COVID-19). Rev Med Virol. (2022) 32:e2348. doi: 10.1002/rmv.2348
48. Singh P, Idowu O, Malik I, Nates JL. Acute respiratory failure induced by magnesium replacement in a 62-year-old woman with myasthenia gravis. Tex Heart Inst J. (2015) 42:495–7. doi: 10.14503/THIJ-14-4584
49. Jessop K. Intravenous magnesium sulfate inducing acute respiratory failure in a patient with myasthenia gravis. BMJ Case Rep. (2022) 15:e250455. doi: 10.1136/bcr-2022-250455
50. Sawalha K, Kakkera K. Acute respiratory failure from hypermagnesemia requiring prolonged mechanical ventilation. J Investig Med High Impact Case Rep. (2020) 8:2324709620984898. doi: 10.1177/2324709620984898
51. Thongprayoon C, Cheungpasitporn W, Srivali N, Erickson SB. Admission serum magnesium levels and the risk of acute respiratory failure. Int J Clin Pract. (2015) 69:1303–8. doi: 10.1111/ijcp.12696
52. Cirik MÖ, Kilin M, Doğanay GE, Ünver M, Yildiz M, Avci S. The relationship between magnesium levels and mortality in the respiratory intensive care unit. Medicine. (2020) 99:e23290. doi: 10.1097/MD.0000000000023290
53. Garner O, Ramey JS, Hanania NA. Management of life-threatening asthma: Severe asthma series. Chest. (2022) 162:747–56. doi: 10.1016/j.chest.2022.02.029
54. Rowe BH, Bretzlaff JA, Bourdon C, Bota GW, Camargo CA Jr. Intravenous magnesium sulfate treatment for acute asthma in the emergency department: A systematic review of the literature. Ann Emerg Med. (2000) 36:181–90. doi: 10.1067/mem.2000.105659
55. Mohammed S, Goodacre S. Intravenous and nebulised magnesium sulphate for acute asthma: Systematic review and meta-analysis. Emerg Med J. (2007) 24:823–30. doi: 10.1136/emj.2007.052050
56. Lazarus SC. Clinical practice. Emergency treatment of asthma. N Engl J Med. (2010) 363:755–64. doi: 10.1056/NEJMcp1003469
57. Adams JY, Sutter ME, Albertson TE. The patient with asthma in the emergency department. Clin Rev Allergy Immunol. (2012) 43:14–29. doi: 10.1007/s12016-011-8273-z
58. Long B, Lentz S, Koyfman A, Gottlieb M. Evaluation and management of the critically ill adult asthmatic in the emergency department setting. Am J Emerg Med. (2021) 44:441–51. doi: 10.1016/j.ajem.2020.03.029
59. Goodacre S, Cohen J, Bradburn M, Gray A, Benger J, Coats T, et al. Intravenous or nebulised magnesium sulphate versus standard therapy for severe acute asthma (3Mg trial): A double-blind, randomised controlled trial. Lancet Respir Med. (2013) 1:293–300. doi: 10.1016/S2213-2600(13)70070-5
60. Kew KM, Kirtchuk L, Michell CI. Intravenous magnesium sulfate for treating adults with acute asthma in the emergency department. Cochrane Database Syst Rev. (2014) 2014:CD010909. doi: 10.1002/14651858.CD010909.pub2
61. Alter HJ, Koepsell TD, Hilty WM. Intravenous magnesium as an adjuvant in acute bronchospasm: A meta-analysis. Ann Emerg Med. (2000) 36:191–7. doi: 10.1067/mem.2000.109170
62. Rowe BH, Bretzlaff JA, Bourdon C, Bota GW, Camargo CA Jr. Magnesium sulfate for treating exacerbations of acute asthma in the emergency department. Cochrane Database Syst Rev. (2000) 2000:CD001490. doi: 10.1002/14651858.CD001490
63. Cheuk DK, Chau TC, Lee SL. A meta-analysis on intravenous magnesium sulphate for treating acute asthma. Arch Dis Child. (2005) 90:74–7. doi: 10.1136/adc.2004.050005
64. Powell C, Dwan K, Milan SJ, Beasley R, Hughes R, Knopp-Sihota JA, et al. Inhaled magnesium sulfate in the treatment of acute asthma. Cochrane Database Syst Rev. (2012) 12:CD003898. doi: 10.1002/14651858.CD003898.pub5
65. Knightly R, Milan SJ, Hughes R, Knopp-Sihota JA, Rowe BH, Normansell R, et al. Inhaled magnesium sulfate in the treatment of acute asthma. Cochrane Database Syst Rev. (2017) 11:CD003898. doi: 10.1002/14651858.CD003898.pub6
66. Normansell R, Knightly R, Milan S, Knopp-Sihota J, Rowe B, Powell C, et al. Inhaled magnesium sulfate in the treatment of acute asthma in children. Paediatr Respir Rev. (2018) 26:31–3. doi: 10.1016/j.prrv.2018.01.001
67. Schuh S, Sweeney J, Rumantir M, Coates AL, Willan AR, Stephens D, et al. Effect of nebulized magnesium vs placebo added to albuterol on hospitalization among children with refractory acute asthma treated in the emergency department: A randomized clinical trial. JAMA. (2020) 324:2038–47. doi: 10.1001/jama.2020.19839
68. Nasser R, Naffaa ME, Mashiach T, Azzam ZS, Braun E. The association between serum magnesium levels and community-acquired pneumonia 30-day mortality. BMC Infect Dis. (2018) 18:698. doi: 10.1186/s12879-018-3627-2
69. Prina E, Ranzani OT, Torres A. Community-acquired pneumonia. Lancet. (2015) 386:1097–108. doi: 10.1016/S0140-6736(15)60733-4
70. Peabody FW. Studies of the inorganic metabolism in pneumonia with especial reference to calcium and magnesium. J Exp Med. (1913) 17:71–82. doi: 10.1084/jem.17.1.71
71. Liu X, Wang S, Ji M, Wang X, Sun J, Zhang M, et al. Hypomagnesemia is associated with increased mortality in the short-term but not the long-term in community-acquired pneumonia patients with type 2 diabetes. Magnes Res. (2022) 35:33–8. doi: 10.1684/mrh.2022.0499
72. Wang S, Lu D, Zhang J, Wang Z, Li X, Ma C. The association between abnormal serum magnesium levels and prognosis of elderly patients with community-acquired pneumonia. Magnes Res. (2021) 34:159–65. doi: 10.1684/mrh.2022.0493
73. Guerrero-Romero F, Micke O, Simental-Mendía L, Rodríguez-Morán M, Vormann J, Iotti S, et al. Importance of Magnesium Status in COVID-19. Biology. (2023) 12:735. doi: 10.3390/biology12050735
74. Trapani V, Rosanoff A, Baniasadi S, Barbagallo M, Castiglioni S, Guerrero-Romero F, et al. The relevance of magnesium homeostasis in COVID-19. Eur J Nutr. (2022) 61:625–36. doi: 10.1007/s00394-021-02704-y
75. Tang CF, Ding H, Jiao RQ, Wu XX, Kong LD. Possibility of magnesium supplementation for supportive treatment in patients with COVID-19. Eur J Pharmacol. (2020) 886:173546. doi: 10.1016/j.ejphar.2020.173546
76. Wallace TC. Combating COVID-19 and building immune resilience: A potential role for magnesium nutrition? J Am Coll Nutr. (2020) 39:685–93. doi: 10.1080/07315724.2020.1785971
77. Tasdemir Mecit BB, Orhan S. Magnesemia in COVID-19 ICU patients: The relationship between serum magnesium level and mortality. Magnes Res. (2022) 35:80–7. doi: 10.1684/mrh.2022.0504
78. Segev A, Sagir A, Matetzky S, Segev A, Atar S, Shechter M, et al. Admission serum magnesium levels is associated with short and long-term clinical outcomes in COVID-19 Patients. Nutrients. (2023) 15:2016. doi: 10.3390/nu15092016
79. La Carrubba A, Veronese N, Di Bella G, Cusumano C, Di PA, Ciriminna S, et al. Prognostic value of magnesium in COVID-19: Findings from the COMEPA Study. Nutrients. (2023) 15:830. doi: 10.3390/nu15040830
80. Gunay S, Caliskan S, Sigirli D. Relationship of magnesemia with myocardial damage and mortality in patients with COVID-19. Magnes Res. (2021) 34:93–102.
81. Nouri-Majd S, Ebrahimzadeh A, Mousavi SM, Zargarzadeh N, Eslami M, Santos HO, et al. Higher intake of dietary magnesium is inversely associated with COVID-19 severity and symptoms in hospitalized patients: A cross-sectional study. Front Nutr. (2022) 9:873162. doi: 10.3389/fnut.2022.873162
82. Tan CW, Ho LP, Kalimuddin S, Cherng BP, Teh YE, Thien SY, et al. Cohort study to evaluate the effect of vitamin D, magnesium, and vitamin B(12) in combination on progression to severe outcomes in older patients with coronavirus (COVID-19). Nutrition. (2020) 7:111017. doi: 10.1016/j.nut.2020.111017
83. Bhatt SP, Khandelwal P, Nanda S, Stoltzfus JC, Fioravanti GT. Serum magnesium is an independent predictor of frequent readmissions due to acute exacerbation of chronic obstructive pulmonary disease. Respir Med. (2008) 102:999–1003. doi: 10.1016/j.rmed.2008.02.010
84. Gumus A, Haziroglu M, Gunes Y. Association of serum magnesium levels with frequency of acute exacerbations in chronic obstructive pulmonary disease: A prospective study. Pulm Med. (2014) 2014:329476. doi: 10.1155/2014/329476
85. Gottlieb M, Moyer E, Meissner H. What is the role of magnesium sulfate for acute exacerbations of chronic obstructive pulmonary disease? Ann Emerg Med. (2023) 81:577–9. doi: 10.1016/j.annemergmed.2022.08.004
86. Ni H, Aye SZ, Naing C. Magnesium sulfate for acute exacerbations of chronic obstructive pulmonary disease. Cochrane Database Syst Rev. (2022) 5:CD013506. doi: 10.1002/14651858.CD013506.pub2
87. Shivanthan MC, Rajapakse S. Magnesium for acute exacerbation of chronic obstructive pulmonary disease: A systematic review of randomised trials. Ann Thorac Med. (2014) 9:77–80. doi: 10.4103/1817-1737.128844
88. Dobler CC, Morrow AS, Beuschel B, Farah MH, Majzoub AM, Wilson ME, et al. Pharmacologic therapies in patients with exacerbation of chronic obstructive pulmonary disease: A systematic review with meta-analysis. Ann Intern Med. (2020) 172:413–22. doi: 10.7326/M19-3007
89. Hashim Ali Hussein S, Nielsen LP, Konow Bogebjerg Dolberg M, Dahl R. Serum magnesium and not vitamin D is associated with better QoL in COPD: A cross-sectional study. Respir Med. (2015) 109:727–33. doi: 10.1016/j.rmed.2015.03.005
90. Zanforlini BM, Ceolin C, Trevisan C, Alessi A, Seccia DM, Noale M, et al. Clinical trial on the effects of oral magnesium supplementation in stable-phase COPD patients. Aging Clin Exp Res. (2022) 34:167–74. doi: 10.1007/s40520-021-01921-z
91. do Amaral AF, Rodrigues-Junior AL, Terra Filho J, Vannucchi H, Martinez JA. Effects of acute magnesium loading on pulmonary function of stable COPD patients. Med Sci Monit. (2008) 14:CR524–9.
92. Amaral AF, Jr LG, Vannucchi H, Crescêncio JC, Vianna EO, Martinez JA. The effect of acute magnesium loading on the maximal exercise performance of stable chronic obstructive pulmonary disease patients. Clinics. (2012) 67:615–22. doi: 10.6061/clinics/2012(06)12
93. Eisen A, Giugliano RP, Braunwald E. Updates on acute coronary syndrome: A review. JAMA Cardiol. (2016) 1:718–30. doi: 10.1001/jamacardio.2016.2049
95. Liu M, Dudley SC Jr. Magnesium, oxidative stress, inflammation, and cardiovascular disease. Antioxidants. (2020) 9:907. doi: 10.3390/antiox9100907
96. Volpe SL. Magnesium in disease prevention and overall health. Adv Nutr. (2013) 4:378S–83S. doi: 10.3945/an.112.003483
97. Maheswaran R, Morris S, Falconer S, Grossinho A, Perry I, Wakefield J, et al. Magnesium in drinking water supplies and mortality from acute myocardial infarction in north west England. Heart. (1999) 82:455–60. doi: 10.1136/hrt.82.4.455
98. Yang CY, Chang CC, Tsai SS, Chiu HF. Calcium and magnesium in drinking water and risk of death from acute myocardial infarction in Taiwan. Environ Res. (2006) 101:407–11. doi: 10.1016/j.envres.2005.12.019
99. Shlezinger M, Amitai Y, Goldenberg I, Shechter M. Desalinated seawater supply and all-cause mortality in hospitalized acute myocardial infarction patients from the Acute Coronary Syndrome Israeli Survey 2002-2013. Int J Cardiol. (2016) 220:544–50. doi: 10.1016/j.ijcard.2016.06.241
100. Shafiq A, Goyal A, Jones PG, Sahil S, Hoffman M, Qinter M, et al. Serum magnesium levels and in-hospital mortality in acute myocardial infarction. J Am Coll Cardiol. (2017) 69:2771–2. doi: 10.1016/j.jacc.2017.03.579
101. Antman E, Anbe D, Armstrong P, Bates E, Green L, Hand M, et al. ACC/AHA guidelines for the management of patients with ST-elevation myocardial infarction–executive summary. A report of the American College of Cardiology/American Heart Association Task Force on Practice Guidelines (Writing Committee to revise the 1999 guidelines for the management of patients with acute myocardial infarction). J Am Coll Cardiol. (2004) 44:671–719.
102. Mizuguchi Y, Konishi T, Nagai T, Sato T, Takenaka S, Tada A, et al. Prognostic value of admission serum magnesium in acute myocardial infarction complicated by malignant ventricular arrhythmias. Am J Emerg Med. (2021) 44:100–5. doi: 10.1016/j.ajem.2021.02.005
103. An G, Du Z, Meng X, Guo T, Shang R, Li J, et al. Association between low serum magnesium level and major adverse cardiac events in patients treated with drug-eluting stents for acute myocardial infarction. PLoS One. (2014) 9:e98971. doi: 10.1371/journal.pone.0098971
104. Naksuk N, Hu T, Krittanawong C, Thongprayoon C, Sharma S, Park J, et al. Association of serum magnesium on mortality in patients admitted to the intensive cardiac care unit. Am J Med. (2017) 130:229.e5–229.e13. doi: 10.1016/j.amjmed.2016.08.033
105. Woods KL, Fletcher S, Roffe C, Haider Y. Intravenous magnesium sulphate in suspected acute myocardial infarction: Results of the second Leicester Intravenous Magnesium Intervention Trial (LIMIT-2). Lancet. (1992) 339:1553–8. doi: 10.1016/0140-6736(92)91828-V
106. Collins R, Peto R, Flather M, Parish S. ISIS-4: A randomised factorial trial assessing early oral captopril, oral mononitrate, and intravenous magnesium sulphate in 58,050 patients with suspected acute myocardial infarction. ISIS-4 (Fourth International Study of Infarct Survival) Collaborative Group. Lancet. (1995) 345:669–85. doi: 10.1016/S0140-6736(95)90865-X
107. Li J, Zhang Q, Zhang M, Egger M. Intravenous magnesium for acute myocardial infarction. Cochrane Database Syst Rev. (2007) 2007:CD002755.
108. Salaminia S, Sayehmiri F, Angha P, Sayehmiri K, Motedayen M. Evaluating the effect of magnesium supplementation and cardiac arrhythmias after acute coronary syndrome: A systematic review and meta-analysis. BMC Cardiovasc Disord. (2018) 18:129. doi: 10.1186/s12872-018-0857-6
109. Alghamdi AA, Al-Radi OO, Latter DA. Intravenous magnesium for prevention of atrial fibrillation after coronary artery bypass surgery: A systematic review and meta-analysis. J Card Surg. (2005) 20:293–9. doi: 10.1111/j.1540-8191.2005.200447.x
110. Burgess DC, Kilborn MJ, Keech AC. Interventions for prevention of post-operative atrial fibrillation and its complications after cardiac surgery: A meta-analysis. Eur Heart J. (2006) 27:2846–57. doi: 10.1093/eurheartj/ehl272
111. De Oliveira GS Jr., Knautz JS, Sherwani S, McCarthy RJ. Systemic magnesium to reduce postoperative arrhythmias after coronary artery bypass graft surgery: A meta-analysis of randomized controlled trials. J Cardiothorac Vasc Anesth. (2012) 26:643–50. doi: 10.1053/j.jvca.2012.03.012
112. Shiga T, Wajima Z, Inoue T, Ogawa R. Magnesium prophylaxis for arrhythmias after cardiac surgery: A meta-analysis of randomized controlled trials. Am J Med. (2004) 117:325–33. doi: 10.1016/j.amjmed.2004.03.030
113. Gu WJ, Wu ZJ, Wang PF, Aung LH, Yin RX. Intravenous magnesium prevents atrial fibrillation after coronary artery bypass grafting: A meta-analysis of 7 double-blind, placebo-controlled, randomized clinical trials. Trials. (2012) 13:41. doi: 10.1186/1745-6215-13-41
114. Miller S, Crystal E, Garfinkle M, Lau C, Lashevsky I, Connolly SJ. Effects of magnesium on atrial fibrillation after cardiac surgery: A meta-analysis. Heart. (2005) 91:618–23. doi: 10.1136/hrt.2004.033811
115. Szapary LB, Szakacs Z, Farkas N, Schonfeld K, Babocsay D, Gajer M, et al. The effect of magnesium on reperfusion arrhythmias in STEMI patients, treated with PPCI. A systematic review with a meta-analysis and trial sequential analysis. Front Cardiovasc Med. (2020) 7:608193. doi: 10.3389/fcvm.2020.608193
Keywords: magnesium, cardiac arrest, resuscitation, respiratory failure, acute coronary syndrome
Citation: Liu B, Li M, Wang J, Zhang F, Wang F, Jin C, Li J, Wang Y, Sanderson TH and Zhang R (2024) The role of magnesium in cardiac arrest. Front. Nutr. 11:1387268. doi: 10.3389/fnut.2024.1387268
Received: 17 February 2024; Accepted: 22 April 2024;
Published: 15 May 2024.
Edited by:
Andrea M. P. Romani, Case Western Reserve University, United StatesReviewed by:
Michał Czapla, Wroclaw Medical University, PolandJiefeng Xu, Zhejiang University, China
Copyright © 2024 Liu, Li, Wang, Zhang, Wang, Jin, Li, Wang, Sanderson and Zhang. This is an open-access article distributed under the terms of the Creative Commons Attribution License (CC BY). The use, distribution or reproduction in other forums is permitted, provided the original author(s) and the copyright owner(s) are credited and that the original publication in this journal is cited, in accordance with accepted academic practice. No use, distribution or reproduction is permitted which does not comply with these terms.
*Correspondence: Rui Zhang, zhang6969rui@163.com; Thomas Hudson Sanderson, thsand@med.umich.edu