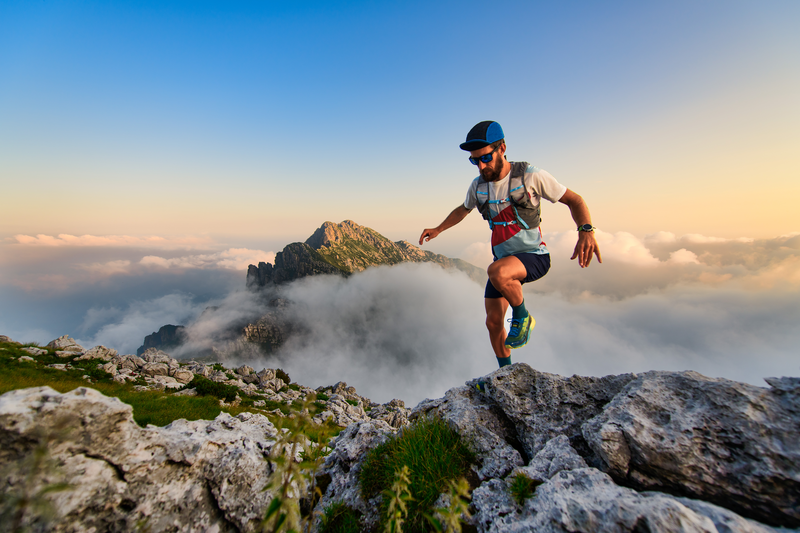
94% of researchers rate our articles as excellent or good
Learn more about the work of our research integrity team to safeguard the quality of each article we publish.
Find out more
ORIGINAL RESEARCH article
Front. Nutr. , 05 June 2024
Sec. Nutrition and Food Science Technology
Volume 11 - 2024 | https://doi.org/10.3389/fnut.2024.1386988
With the growing global population and climate change, achieving food security is a pressing challenge. Vertical farming has the potential to support local food production and security. As a Total Controlled Environment Agriculture (TCEA) system, vertical farming employs LED lighting which offers opportunities to modulate light spectrum and intensity, and thus can be used to influence plant growth and phytochemical composition, including antioxidants beneficial for human health. In this study, we investigated the effect of four red-to-blue light ratios of LEDs (R:B 1, 2.5, 5 and 9) on the growth and antioxidant components in red amaranth microgreens and red lettuce. Plant growth, total phenols, betalains, anthocyanins, vitamin C and antioxidant capacity (ferric reducing antioxidant power assay) were evaluated. A higher proportion of red light resulted in biometric responses, i.e., stem elongation in red amaranth and longer leaves in red lettuce, while the increase in the blue light fraction led to the upregulation of antioxidative components, especially total phenols, betalains (in red amaranth) and anthocyanins (in red lettuce). The antioxidant capacity of both crops was strongly positively correlated with the levels of these phytochemicals. Optimizing the red-to-blue ratio in LED lighting could be effective in promoting antioxidant-rich crops with potential health benefits for consumers.
Global population projections estimate 9.7 billion people by 2050, possibly 10.4 billion in 2100 (1), and with that a major challenge in achieving food security. Food production, consumption and waste are the key factors in shaping the health of people and the planet (2). Unhealthy diets pose a threat for people and the planet on a global scale. Even though the global food system contributes 21–37% (3) of global greenhouse gas emissions, there is still a high prevalence of malnutrition and food insecurity. Poor diets are a significant burden on the global health system and society (4). The combination of population growth, limited, arguably diminishing, farmland available for food production and the impacts of climate change, have raised interest in Controlled Environment Agriculture (CEA), and particularly vertical farming, as a robust route to the production of some foodstuffs (5). These systems offer the potential for continuous production (24/7/365), albeit with a requirement for energy inputs for temperature, humidity, and lighting control facilitating the delivery of fresh and nutritious food available at national and local levels. The extension of CEA to vertical farming takes the concept further as it reduces the growing system footprint by growing in three dimensions and as a result is opening up the opportunity of urban food production using derelict and/or poorly used land (6, 7).
Light is arguably the most essential factor for plant growth and development, and the controlled lighting systems in vertical farming are being utilized to influence plant growth rates, yield and composition including important nutrients and health-beneficial phytochemicals (8–10). Light-emitting diodes (LED) lights have been used as an efficient light source for commercial plant cultivation in vertical farming. They provide the opportunity to modulate light quality (wavelengths), intensity and duration (photoperiod), which play a key role in plant physiological processes (11). Plants use wavelength-specific photoreceptors known as phytochromes (red/far red light), cryptochromes (blue light/UV-A), and phototropins (blue light) to perceive and interpret incoming light signals to regulate their physiology and development (12, 13). Currently, red light (approx. 600–700 nm) and blue light (approx. 400–500 nm) have been recognized as the most suitable treatments for plant growth and development of tailored food (11), although other wavelengths, e.g., UV, are well reported to enhance secondary metabolites such as (poly)phenolics (13, 14). Indeed, these secondary metabolites and some primary metabolites comprise a major component of the plant antioxidant system. These compounds are produced in plants as part of their defense mechanisms in response to various environmental stresses and/or to play a crucial role in neutralizing reactive oxygen species (ROS) and maintaining a steady oxidative state to allow normal plant biochemical function (15). Many of these components have also been reported to exert health benefits when eaten by humans (16, 17).
The antioxidant effect of plant foods is mainly attributed to compounds such as flavonoids, including anthocyanins, phenolic acids, tannins, betalains, carotenoids, glucosinolates and certain vitamins (18–20). It has been shown that the consumption of plant foods high in antioxidants (rather than isolated supplements) is associated with a lower risk of chronic oxidative stress and the related symptoms (21–23). The biosynthesis of antioxidants in plants can be influenced by light intensity and light spectrum; for instance, anthocyanin levels increased in red holy basil but decreased in mustard microgreens when blue light only or higher blue light fractions were used (24, 25).
Among the most common crops grown in vertical farms are salad crops and microgreens. Microgreens are tender immature greens grown from the seeds of vegetables and herbs, harvested upon the appearance of the first pair of true leaves when the cotyledons are fully expanded (26). Recent studies have revealed that microgreens are richer than mature greens in some vitamins, sugars, and antioxidants, including carotenoids (27). The access to this new form of nutrient dense produce provides a great opportunity to deliver micronutrients and phytochemicals in a safe way. In addition, red/darker varieties of vegetables have higher nutrient composition, anthocyanin content, vitamin C, and mineral content compared to green vegetables (19, 28, 29). Thus, two crops of red/darker variety were chosen for our study: red amaranth microgreens and red lettuce. For their high content of nutrients, including the antioxidants betalains and anthocyanins, respectively (19, 28, 30), these crops are gaining great popularity as a healthy and sustainable food option. In this study, red amaranth and red lettuce were grown in a vertical farm under different red-to-blue ratios (R:B) of the LED light spectrum, with the goal to examine the effect of the light treatments on growth and on the content of phytochemicals with antioxidant capacity.
The experiment was conducted in a 10-week period in the state-of-the-art vertical farm at the Intelligent Growth Solutions Ltd. Crop Research Center in Invergowrie, Scotland. The seeds of Micro Amaranth Red Aztec (Amaranthus tricolor L.) and Red Batavia Lettuce (Lactuca sativa L.) were purchased from CN seeds (Ely, United Kingdom) and were sown using a Mosa Drum Seeder sowing machine (Mechanical Botanical, Chiddingfold, United Kingdom) into propagation trays filled with a substrate medium produced from a 75:25 (v/v) mix of coconut coir and vermiculite. The propagation trays were then transferred into a dark germination chamber for 2 days and then placed on the growth trays with lights (GTLs) in the vertical farm growth towers.
LED lights (Osram, Austria) were arranged horizontally 300 mm above the tray base in the GTLs and emitted blue light (B, λ = 400 nm-499 nm, peak at 451 nm), green light (G, λ = 500 nm-599 nm, peak at 521 nm), red light (R, λ = 600 nm-699 nm, peak at 660 nm) and far-red light (FR, λ = 700 nm-799 nm, peak at 730 nm). The crops were grown under four different light regimes: the red-to-blue light ratio (R:B) was adjusted to 1, 2.5, 5 and 9 (Table 1). The photosynthetic photon flux density (PPFD) was kept as close to the standard light recipe (2.55) as possible at ~255 μmol m−2 s−1 (Table 1). The photoperiod was 18 h d−1, temperature 25°C, relative humidity 70–75%, pH and EC of the nutrient solution were 5.7–6.3 and 1.7–1.8 dS m−1, respectively; the nutrient solution and watering schedule were kept identical for all the light treatments.
Table 1. Four light recipes used for the treatment of red amaranth and red lettuce in the vertical farm.
Plants were harvested nine and 22 days after sowing for amaranth and lettuce, respectively. The leaf length in lettuce was recorded as well as the stem height in red amaranth. After the fresh weight was recorded, the plants were snap frozen in liquid nitrogen and freeze-dried using Christ Gamma 1–16 LSC freeze dryer (Osterode am Harz, Germany) for a minimum of 3 days. The dry matter was weighed to determine the percentage of dry weight and the plant material was homogenized using Retch ZM 200 ultracentrifugal mill with sieve size 0.5 mm (Haan, Germany). Samples were kept in 50 mL-falcon tubes at −20°C until required for analysis. Every biological replicate represented a pool of two propagation trays.
For colorimetric analyses, 10 mL of 80% methanol was added to 200 mg of homogenized plant material in a 15 mL falcon tube. The suspensions were vortexed for 30 s, sonicated in an ultrasonic bath with ice for 15 min then agitated on an orbital shaker for 30 min. The samples were then centrifuged for 10 min at 3,900 rpm, and the supernatants stored at −80°C.
For vitamin C analysis, 50 mg of homogenized plant material in a 2 mL microtube was resuspended in 1.5 mL of 5% (w/v) metaphosphoric acid containing 5 mM tris(2-carboxyethyl)phosphine (TCEP). The suspension was vortexed for 10 s and agitated on IKA Vibrax VXR basic shaker at 1,750 rpm for 60 min at 5°C. The suspension was then centrifuged at 5°C for 10 min at 13,000 rpm and the supernatant filtered in Thomson Standard Filter Vials prior analysis.
Total phenolic content (TPC) was measured using the method described by Slinkard and Singleton (31). Amaranth and lettuce methanolic extracts were diluted with distilled water to 20 and 10% solutions, respectively. To 250 μL of diluted samples, 250 μL of Folin–Ciocalteu reagent was added and the mixture incubated for 3 min at room temperature. Further, 500 μL of saturated sodium carbonate were added and the samples incubated for 1 h at room temperature. The absorbance was measured using Ultrospec 2100 spectrophotometer (Amersham Biosciences, Buckinghamshire, United Kingdom) at 750 nm. Quantification was made using the gallic acid standard curve (0.02–0.8 mg mL−1, r2 = 0.9918). Results were expressed in g of gallic acid equivalent (GAE) per 100 g of sample on dry weight (g GAE 100 g−1 DW) and fresh weight basis (g GAE 100 g−1 FW).
Betalains were measured in red amaranth only as they are absent from red lettuce (32). The quantification of betalains (as sum of betacyanins and betaxanthins) was carried out according to Sokolova et al., with minor modifications (33). Methanolic extracts were diluted with water to 10% solutions and the content of betacyanins and betaxanthins was determined at 536 and 485 nm, respectively, using the following equation:
where A is optical density in nm (for betacyanins: A = A536nm − A650nm, and for betaxanthins: A = A485nm − A650nm); DF = dilution factor; MW = molecular weight (550 g mol−1 for betacyanins, and 339 g mol−1 for betaxanthins); ɛ = molar extinction coefficient in L mol−1 cm−1 (60,000 for betacyanins, and 48,000 for betaxanthins); l = path length in cm; 103 = factor for conversion from g to mg; the measurement at the wavelength of 650 nm was used to correct for impurities. Total betalains were calculated by adding betacyanins and betaxanthins and the results were expressed as mg betalains per 100 g of sample in dry weight (mg 100 g−1 DW) and fresh weight (mg 100 g−1 FW).
Anthocyanins were measured in red lettuce only as they are absent from red amaranth (34). The quantification of anthocyanins was carried out using a pH differential absorbance method according to Lee et al. (35) with minor modifications. Solutions of 0.2 M potassium chloride (pH 1.0) or 0.1 M sodium acetate (pH 4.5) were added to the methanolic extracts in a 9:1 ratio, mixed and incubated for 10 min at room temperature. The absorbance was measured using a spectrophotometer at 510 nm and 700 nm in buffers at pH 1.0 and pH 4.5, respectively. The concentration of anthocyanins was estimated using the following equation:
where A (optical density in nm) = (A510nm ̶ A700nm) pH 1.0 ̶ (A510nm ̶ A700nm) pH 4.5; MW (molecular weight) = 449.2 g mol−1 for cyanidin-3-glucoside (C3G); DF = dilution factor; ε = molar extinction coefficient, in L mol−1 cm−1 (26,900 for C3G); l = path length in cm; 103 = factor for conversion from g to mg.
The quantity of anthocyanins was expressed as mg of C3G equivalents per 100 g of sample on dry weight (mg C3GE 100 g−1 DW) as well as fresh weight basis (mg C3GE 100 g−1 FW).
Vitamin C (ascorbic acid) content was determined as described previously by Freitag et al. (36). Briefly, the samples were analyzed using HPLC (ASI-100 autosampler, and Ultimate 3000 pump) coupled to a DAD detector (UVD340U, Dionex, ThermoFisher Scientific, United Kingdom). Sample (20 μL) was injected onto an ICSep COREGAL-64H column (7.8 × 300 mm × 10 μm, ChromTech, United States) and an isocratic run of 30 min with a mobile phase containing 4 mM sulfuric acid in ultrapure water was applied. Ascorbic acid was quantified at 245 nm against external calibration curve of ascorbic acid (75–250 μg mL−1, r2 = 0.999) and the results expressed as both mg 100 g−1 DW and mg 100 g−1 FW.
Ferric reducing antioxidant power assay (FRAP) was used to measure antioxidant capacity (37). In short, 900 μL of FRAP reagent consisting of 300 mM sodium acetate trihydrate (pH 3.6), 10 mM ferric 2,4,6-tripyridyl-s-triazine (TPTZ) in 40 mM hydrochloric acid and 20 mM ferric (Fe3+) chloride hexahydrate in a 10:1:1 ratio, was added to 100 μL of diluted methanolic extracts (10% solution of amaranth extract; 5% solution of lettuce extract). The absorbance was measured at 593 nm using a spectrophotometer after exactly 4 min following the addition the FRAP reagent. The reaction was estimated based on the ferrous (Fe2+) sulfate standard curve (100–1,000 μM, r2 = 0.998). Results were expressed as mmol Fe2+ 100 g−1 sample on dry weight (mmol Fe2+ 100 g−1 DW) and fresh weight basis (mmol Fe2+ 100 g−1 FW).
Statistical analysis was conducted with IBM SPSS Version 29.0.1.0 (171; SPSS Inc., United States). The results shown are the means of five biological replicates ± standard deviation (SD). One-way analysis of variance (ANOVA) at significance level of p < 0.05 with Tukey’s HSD post-hoc test was used to determine statistical differences between light treatments. Pearson correlation analysis was performed using the MetaboAnalyst 5.0 web-based platform (38).
The effect of different light treatments on the dry biomass content and growth of red amaranth and red lettuce was evaluated. The light treatments significantly affected stem height and leaf length in red amaranth and red lettuce, respectively (Figures 1A,B; Supplementary Table S1). As the proportion of red light increased, the stem height in amaranth significantly increased, with the RB9-treated plants being the tallest (Figure 1A). A similar trend was observed for the leaf length in lettuce. Higher R:B ratio resulted in longer leaves, with the longest under RB9 treatment (Figure 1B; Supplementary Table S1). RB1-treated plants of both species were significantly shorter in height or length when compared to all other treatments. In red amaranth, the percentage of dry weight (DW%) was significantly higher in RB2.5- and RB5-treated plants (8.79 and 9.17%, respectively) compared to RB1 and RB9 treatments (7.98 and 8.12%, respectively; Figure 1C; Supplementary Table S1). In red lettuce, there were no significant differences between treatments in DW%, with the average value of 6.29% DW (Figure 1D; Supplementary Table S1).
Figure 1. Stem height of red amaranth (A) and leaf length of red lettuce (B), dry weight percentage of red amaranth (C) and red lettuce (D) affected by four light ratios. Values represent mean ± SD (n = 5). Different letters indicate significant difference among treatments for each crop (ANOVA, Tukey’s test, p < 0.05).
To evaluate the antioxidant content in red amaranth and red lettuce, total phenol content (TPC), total betalains (in red amaranth), total anthocyanins (in red lettuce), vitamin C and antioxidant capacity (FRAP) were quantified per dry weight (DW; Figure 2; Supplementary Table S2) and fresh weight (FW; Supplementary Table S3).
Figure 2. Total phenol content (TPC; A and B), betalains/anthocyanins (ACNs; C and D), vitamin C (E and F) and FRAP antioxidant capacity (G and H) in red amaranth and red lettuce under four different light treatments. Values represent mean ± SD (n = 5). Different letters indicate significant difference (ANOVA, Tukey’s test, p < 0.05).
The different light recipes had a significant effect on total phenol content in red amaranth and red lettuce (Figures 2A,B). The TPC ranged from 1.98 to 2.2 g GAE 100 g−1 DW in red amaranth and from 5.6 to 6.54 g GAE 100 g−1 DW in red lettuce. In red amaranth, RB1-treated plants had significantly higher content of total phenols than RB5- and RB9-treated plants. In red lettuce, RB1-treated plants were significantly higher in total phenols than plants treated with RB9. In both plant species the trend was generally similar toward reduced TPC with increasing red/blue light ratio.
In red amaranth, the light recipes tested had a significant effect on betalain content (Figure 2C). It ranged from 667 to 937 mg 100 g−1 DW. RB5- and RB9-treated plants were lower in betalains than plants under other light treatments and the recipe with the highest blue light fraction (RB1) resulted in the highest betalain content. In red lettuce, total anthocyanins ranged from 158 to 231 mg C3GE 100 g−1 DW. Anthocyanins were significantly lower in RB5- and RB9-treated plants compared to the RB1 treatment (Figure 2D). The highest blue light fraction (RB1) resulted in the highest anthocyanin content. As for the TPC, the trend was for a reduced betalain and anthocyanin content with increasing red/blue light ratio.
The vitamin C content ranged from 331 to 577 mg 100 g−1 DW in red amaranth and from 283 to 423 mg 100 g−1 DW in red lettuce (Figures 2E,F). The different R:B ratios had a significant effect on vitamin C content in both crops. In red amaranth, RB2.5 light recipe resulted in a significantly higher vitamin C content than RB1 and RB9 recipes. RB9-treated plants had significantly lower vitamin C content compared to the other treatments (Figure 2E). In red lettuce, RB2.5 led to significantly higher levels of vitamin C compared to all other treatments, while RB5-treated plants had significantly lower vitamin C content compared to the other treatments (Figure 2F). Therefore, no clear trend of vitamin C accumulation with red/blue light ratio was evident.
The light recipes had a significant effect on antioxidant capacity in both red amaranth and red lettuce (Figures 2G,H). The antioxidant capacity ranged from 9.4 to 13.5 mmol Fe2+ 100 g−1 DW in red amaranth and from 33.6 to 52.5 mmol Fe2+ 100 g−1 DW in red lettuce. In both crops, RB9-treated plants had significantly lower antioxidant capacity than plants grown under the other light treatments and the treatment with the highest blue light fraction resulted in the highest antioxidant capacity. This trend was also observed for TPC and betalain content with a decreasing value as the red/blue light ratio increased, which suggests that (poly)phenols and betalains make dominant contributions to the antioxidant capacity.
In red amaranth, correlation analysis (Figure 3A) revealed a strong positive correlation between FRAP and vitamin C (r = 0.78, p < 0.001), FRAP and betalains (r = 0.69, p < 0.001), FRAP and TPC (r = 0.77, p < 0.001) and between TPC and betalains (r = 0.72, p < 0.001). Moderate positive correlations were observed between vitamin C and betalains (r = 0.56, p < 0.01), vitamin C and TPC (r = 0.52, p < 0.05) and vitamin C and dry weight (r = 0.57, p < 0.01). Stem height was strongly negatively correlated with FRAP (r = −0.81, p < 0.001), betalains (r = −0.81, p < 0.001) and TPC (r = −0.82, p < 0.001). In red lettuce (Figure 3B), a strong positive correlation was found between TPC and anthocyanins (r = 0.89, p < 0.001). FRAP was strongly positively correlated with TPC (r = 0.83, p < 0.001) and anthocyanins (r = 0.78, p < 0.001). Dry weight was strongly or moderately correlated with TPC (r = 0.69, p < 0.001) and anthocyanins (r = 0.61, p < 0.01). Leaf length was strongly negatively correlated with FRAP (r = −0.75, p < 0.001), TPC (r = −0.78, p < 0.001) and anthocyanins (r = −0.82, p < 0.001). Vitamin C was not significantly correlated to any other parameter. The correlation heatmaps for the same parameters quantified in fresh weight are shown in Supplementary Figure S1.
Figure 3. Correlation heatmaps showing Pearson correlation coefficients between stem height/leaf length, dry weight percentage, total phenol content (TPC), betalains/anthocyanins, vitamin C and FRAP in dry weight of red amaranth (A) and red lettuce (B), as generated by MetaboAnalyst 5.0. Data were log10-transformed and auto-scaled; *p < 0.05, **p < 0.01, ***p < 0.001.
The present study indicates that the R:B ratio of LED lighting has a significant effect on the growth and antioxidant content in red leafy vegetables grown in a vertical farm. Physical characteristics such as stem height and leaf length are commercially important for growing produce to make it more appealing to consumers. In previous studies, both significant and non-significant responses in yield to different R:B ratios have been shown in various crops including red and green lettuce, sprouting broccoli and basil (39–43). In several lettuce cultivars red illumination increased growth and yield (39, 43). An increase in leaf area has also been achieved in lettuce cultivars by using increased red light proportion (44–46), which is consistent with our study in terms of higher red light fraction resulting in plants with longer leaves. Higher blue light percentages (lower R:B ratio) decreased plant height in Brassica microgreens and green holy basil (25, 47) whereas Rihan et al. reported an increased growth and yield in sweet basil with lower R:B ratios (41). Green and far-red light also play a role in the response of the plants to the light. The addition of green light had a positive and significant impact on leaf growth and yield in lettuce (39). In our study, the total quantity of green and far-red light fractions was kept constant in all treatments, but we recognize that their ratio to red and blue fractions varied in the four tested light recipes, which could have an additional effect on the measured parameters. Our results suggest that the increase in R:B ratio is favorable for red amaranth and red lettuce plant growth, regarding stem height and leaf length, respectively. However, this effect might not be always desirable as very long stalks or leaves might reduce marketability in a commercial setting, although the ability to vary this has been shown to be useful as a global/regional preference in the same crop criteria can vary considerably (48). Moreover, our data indicate that red amaranth with higher stems and red lettuce with longer leaves under higher R:B ratio have significantly lower levels of antioxidants and thus also reduced potential health beneficial effects for consumers (which, in the case of red lettuce, could possibly be caused by a dilution effect, as positive correlations were observed between dry weight and TPC/anthocyanins).
Phenolic compounds such as flavonoids and phenolic acids are plant metabolites that help protect plants against biotic (e.g., pathogens) and abiotic stressors (e.g., drought, high amount of light). There is also an extensive literature on the health benefits of phenolic compounds, including their cytotoxic, anti-inflammatory, antihypertensive, and anti-diabetic actions (49, 50). Blue light has been shown to enhance the accumulation of phenolic compounds in certain species (13, 25). In the present study, the increase of the proportion of blue light (lower R:B ratio) led to a higher content of total phenols (TPC) in both red amaranth and red lettuce. This is consistent with the findings by Kwack et al., showing that higher red-light intensity resulted in reduced total phenolic content in alfalfa sprouts and red radish (51). In Chinese kale sprouts, monochromatic blue light has been shown to significantly increase total phenol content compared to darkness, while monochromatic red light had no effect on TPC (52). A lower R:B ratio has been shown to increase total phenolics in lettuce and basil (25, 46). Thus, blue light can be used to increase total phenols in a variety of crops, but consideration is needed as the effects are species dependent and species respond differently in terms of phytochemical accumulation (53). Moreover, the addition of UV-A and UV-B light to the spectrum might result in enhanced accumulation of phenolic compounds with antioxidant properties as was demonstrated, e.g., in lettuce (54).
Secondary metabolites also play a role in taste, color and aroma of plants (13). Betalains (indole-derived chromoalkaloids typical for Caryophyllales) and anthocyanins (class of flavonoids) commonly give plants their characteristic red, purple, or yellow color. Anthocyanins and betalains are mutually exclusive, with the latter largely replacing the former in plants of the order Caryophyllales that also includes the genus Amaranthus (55, 56). In the present study, the highest concentration of betalains in red amaranth was detected under the highest blue fraction (RB1) and, as in the case of the phenolics, decreased with the increase in red light fraction. It was shown previously that red amaranth treated with blue light compared to dark conditions, had increased levels of betalains due to the upregulation of key genes involved in the synthesis of betalains by blue light (56). Similarly, the increase in the proportion of blue light led to significantly higher levels of anthocyanins in red lettuce, which is consistent with the previous light studies on red lettuce (46, 57). This is likely to be related to blue light activating the expression of genes that induce anthocyanin biosynthesis (57). In Chinese kale sprouts, monochromatic blue light irradiation has been shown to be the most effective in increasing anthocyanin content compared to other light treatments (52). The strong correlation of antioxidant capacity with betalains and anthocyanins indicates that they play an important role in the antioxidant capacity of red amaranth and red lettuce, respectively. Future work could examine if these changes in total betalain and anthocyanin contents are accompanied by changes in the profiles of the individual betalains/anthocyanins and other phytochemicals and elucidate the precise mechanisms underlying the complex signaling networks that regulate plant biochemical processes in response to different red and blue light ratios (including the regulation of the tyrosine-based betalain biosynthetic pathway and the phenylpropanoid pathway). Enhanced blue light was previously shown to increase flavonoids and phenolic acids in red leaf lettuce, including quercetin-glycosides, protocatechuic acid and chicoric acid (53). On contrary, Lee et al. found that supplemental red but not blue irradiation in red lettuce led to significantly enhanced accumulation of individual phenolic compounds, including chlorogenic acid, caffeic acid, chicoric acid, rutin, kaempferol, and luteolin, while the total phenol content was higher under the supplemental blue light (58). Other pigments than betalains/anthocyanins that could have been affected by the R:B treatments, but were not measured in this study, are chlorophylls and carotenoids. A one-to-one ratio of red and blue light seems to be the most effective treatment to achieve higher levels of chlorophyll contents in red amaranth and red lettuce (39, 45, 59–61), as blue light addition is key to chlorophyll biosynthesis in plant leaves (62, 63). Carotenoids have been shown to be increased under blue light in red amaranth and lettuce (60, 61, 64), which correlates with our study findings as they are accessory pigments related to antioxidant mechanisms (65).
Vitamin C (ascorbic acid) is an essential dietary vitamin required as a co-factor for many enzymes and can act as an important antioxidant in many body tissues (66). It plays a key role in bodily processes, e.g., iron absorption, collagen synthesis and immune system stimulation (67, 68). Light influences vitamin C accumulation in plants and is dependent on exposure time as well as intensity and quality of light (11, 67). Here, the RB2.5-treated plants had the highest levels of vitamin C in both crops. There was no clear trend observed in the vitamin C levels in relation to the R:B ratio. In previous studies on lettuce, the highest content of vitamin C was detected under 100% red light, indicating that pure red light can promote the synthesis or accumulation of vitamin C in lettuce most probably as a result of light stress (69, 70). In our study, the levels of vitamin C in both crops reacted to an increase in blue light as well as red light. The literature has shown both increases and decreases in vitamin C content in plants treated with higher levels of blue or red light (71–73). In kale sprouts the highest levels of vitamin C were found in plants under white LED treatment, followed by red LED treatment (52). In our study, vitamin C correlated strongly with antioxidant capacity in red amaranth. However, there was no significant relationship found for vitamin C in red lettuce, indicating that the high amounts of phenolic compounds present in red lettuce were the main contributors to the antioxidant capacity (45).
In both crops, the treatment with the highest proportion of blue light (RB1) resulted in the highest antioxidant capacity. Blue light has been previously shown to increase antioxidant capacity in lettuce (45, 74). The effects of light spectrum on total phenol content and betalains/anthocyanins are reflected in the total antioxidant capacity in both crops, with significant positive correlations in both red amaranth and red lettuce. Phenolic compounds are considered important antioxidants as they act as free radical scavengers (17, 75). Previously, a positive correlation between antioxidant capacity and TPC was demonstrated, for example, for basil cultivars and sage (25, 76). Similarly, betalains are known as very strong antioxidants, even superior to many flavonoids and vitamins C and E in their antiradical action (77). Phenolic compounds and betalains play an important health-promoting role in human diet, and here we demonstrate that their content in crops can be enhanced by optimizing the light spectrum.
In this study, we investigated the effects of different red-to-blue (R:B) light ratios on biometrics as well as on antioxidative components in red amaranth and red lettuce. Light ratios influenced the growth of red amaranth and red lettuce, and the accumulation of plant secondary metabolites. Higher proportions of red light resulted in increased stem height in red amaranth and leaf length in red lettuce, with R:B of 9:1 having the strongest effect. The increase of the blue light fraction caused the upregulation of antioxidative components and antioxidant capacity, which reached the highest levels under R:B of 1:1 in most instances. Our findings demonstrate that it is possible to use LED lights in a vertical farm setting to modulate, possibly enhance, the phenotypic properties and/or nutritional quality of crops, using different ratios of red and blue light. Overall, light recipes can be individually tailored according to the type of crop as well as the desired outcomes.
The original contributions presented in the study are included in the article/Supplementary material, further inquiries can be directed to the corresponding author.
AB: Conceptualization, Data curation, Formal analysis, Investigation, Methodology, Project administration, Writing – original draft, Writing – review & editing. MP: Conceptualization, Data curation, Formal analysis, Methodology, Supervision, Writing – original draft, Writing – review & editing. VP: Conceptualization, Methodology, Writing – original draft, Writing – review & editing. SP: Data curation, Formal analysis, Writing – original draft, Writing – review & editing. CA: Data curation, Formal analysis, Writing – original draft, Writing – review & editing. TK: Methodology, Resources, Writing – original draft, Writing – review & editing. GM: Data curation, Supervision, Writing – original draft, Writing – review & editing. AJ: Funding acquisition, Project administration, Supervision, Writing – original draft, Writing – review & editing. DS: Conceptualization, Funding acquisition, Supervision, Writing – original draft, Writing – review & editing.
The author(s) declare that financial support was received for the research, authorship, and/or publication of this article. The authors acknowledge support from the Scottish Government Rural and Environment Science and Analytical Services Division (RESAS), specifically projects JHI-B1-6 and RI-B7-1. AB’s studentship was funded as part of the Underpinning National Capacity component of the RESAS Strategic Research Program. DS acknowledges support from Innovate UK for vertical farming projects 10071841, 10076759, 10008565, 93802 and 51297 whose progress aided the writing here.
We are grateful to Sarah Auld for technical support.
VP and TK were employed by company Intelligent Growth Solutions Ltd.
The remaining authors declare that the research was conducted in the absence of any commercial or financial relationships that could be construed as a potential conflict of interest.
All claims expressed in this article are solely those of the authors and do not necessarily represent those of their affiliated organizations, or those of the publisher, the editors and the reviewers. Any product that may be evaluated in this article, or claim that may be made by its manufacturer, is not guaranteed or endorsed by the publisher.
The Supplementary material for this article can be found online at: https://www.frontiersin.org/articles/10.3389/fnut.2024.1386988/full#supplementary-material
1. United Nations. World population prospects 2022: Summary of results. (2022). Available at: https://www.un.org/en/desa/world-population-projected-reach-98-billion-2050-and-112-billion-2100.
2. Willett, W, Rockström, J, Loken, B, Springmann, M, Lang, T, Vermeulen, S, et al. Food in the Anthropocene: the EAT–lancet commission on healthy diets from sustainable food systems. Lancet. (2019) 393:447–92. doi: 10.1016/s0140-6736(18)31788-4
3. Mbow, C, Rosenzweig, C, Barioni, LG, Benton, TG, Herrero, M, Krishnapillai, M, et al. Food security. Climate change and land: An IPCC special report on climate change, desertification, land degradation, sustainable land management, food security, and greenhouse gas fluxes in terrestrial ecosystems. Cambridge university press. (2019) 437–550.
4. Institute of global health metrics and evaluation. The global burden of disease (GBD). (2020). Available at: https://www.healthdata.org/research-analysis/gbd
5. Dsouza, A, Newman, L, Graham, T, and Fraser, EDG. Exploring the landscape of controlled environment agriculture research: a systematic scoping review of trends and topics. Agric Syst. (2023) 209:103673. doi: 10.1016/j.agsy.2023.103673
6. Oh, S, and Lu, C. Vertical farming - smart urban agriculture for enhancing resilience and sustainability in food security. J Hortic Sci Biotechnol. (2023) 98:133–40. doi: 10.1080/14620316.2022.2141666
7. Yuan, X, Yu, L, Wu, H, She, H, Luo, J, and Li, X. Sustainable development goals (SDGs) priorities of senior high school students and global public: recommendations for implementing education for sustainable development (ESD). Educ Res Int. (2021) 11:458. doi: 10.3390/educsci11090458
8. Folta, KM, and Maruhnich, SA. Green light: a signal to slow down or stop. J Exp Bot. (2007) 58:3099–111. doi: 10.1093/jxb/erm130
9. Nguyen, TKL, Cho, KM, Lee, HY, Cho, DY, Lee, GO, Jang, SN, et al. Effects of white LED lighting with specific shorter blue and/or green wavelength on the growth and quality of two lettuce cultivars in a vertical farming system. Agronomy. (2021) 11:2111. doi: 10.3390/agronomy11112111
10. Craver, JK, Gerovac, JR, Lopez, RG, and Kopsell, DA. Light intensity and light quality from sole-source light-emitting diodes impact phytochemical concentrations within Brassica microgreens. J Am Soc Hortic Sci. (2017) 142:3–12. doi: 10.21273/JASHS03830-16
11. Loi, M, Villani, A, Paciolla, F, Mule, G, and Paciolla, C. Challenges and opportunities of light-emitting diode (LED) as key to modulate antioxidant compounds in plants. A review. Antioxidants. (2020) 10:42. doi: 10.3390/antiox10010042
12. Paik, I, and Huq, E. Plant photoreceptors: multi-functional sensory proteins and their signaling networks. Semin Cell Dev Biol. (2019) 92:114–21. doi: 10.1016/j.semcdb.2019.03.007
13. Wong, CE, Teo, ZWN, Shen, L, and Yu, H. Seeing the lights for leafy greens in indoor vertical farming. Trends Food Sci Technol. (2020) 106:48–63. doi: 10.1016/j.tifs.2020.09.031
14. Wang, M, Leng, C, Zhu, Y, Wang, P, Gu, Z, and Yang, R. UV-B treatment enhances phenolic acids accumulation and antioxidant capacity of barley seedlings. LWT. (2022) 153:112445. doi: 10.1016/j.lwt.2021.112445
15. Hasanuzzaman, M, Bhuyan, MHMB, Zulfiqar, F, Raza, A, Mohsin, SM, Mahmud, JA, et al. Reactive oxygen species and antioxidant defense in plants under abiotic stress: revisiting the crucial role of a universal defense regulator. Antioxid Basel Switz. (2020) 9:681. doi: 10.3390/antiox9080681
16. Pandey, KB, and Rizvi, SI. Plant polyphenols as dietary antioxidants in human health and disease. Oxidative Med Cell Longev. (2009) 2:270–8. doi: 10.4161/oxim.2.5.9498
17. Ruskovska, T, Maksimova, V, and Milenkovic, D. Polyphenols in human nutrition: from the in vitro antioxidant capacity to the beneficial effects on cardiometabolic health and related inter-individual variability – an overview and perspective. Br J Nutr. (2020) 123:241–54. doi: 10.1017/S0007114519002733
18. Bastos, EL, and Schliemann, W. Betalains as Antioxidants In: HM Ekiert, KG Ramawat, and J Arora, editors. Plant antioxidants and health. Reference series in phytochemistry. Cham: Springer (2021).
19. Hameed, MK, Umar, W, Razzaq, A, Wei, S, Niu, Q, Huang, D, et al. Quantification of total polyphenols, antioxidants, anthocyanins and secondary metabolites by UPLC VION IMS QTOF MS/MS analysis in green and red lettuce cultivars. Sci Hortic. (2023) 315:111994. doi: 10.1016/j.scienta.2023.111994
20. Kaur, S, and Mondal, P. Study of total phenolic and flavonoid content, antioxidant activity and antimicrobial properties of medicinal plants. Microbiol Exp. (2014) 1:23–8. doi: 10.15406/jmen.2014.01.00005
21. Aune, D, Giovannucci, E, Boffetta, P, Fadnes, LT, Keum, N, Norat, T, et al. Fruit and vegetable intake and the risk of cardiovascular disease, total cancer and all-cause mortality—a systematic review and dose-response meta-analysis of prospective studies. Int J Epidemiol. (2017) 46:1029–56. doi: 10.1093/ije/dyw319
22. Bhupathiraju, SN, Wedick, NM, Pan, A, Manson, JE, Rexrode, KM, Willett, WC, et al. Quantity and variety in fruit and vegetable intake and risk of coronary heart disease. Am J Clin Nutr. (2013) 98:1514–23. doi: 10.3945/ajcn.113.066381
23. Joshipura, KJ, Hu, FB, Manson, JE, Stampfer, MJ, Rimm, EB, Speizer, FE, et al. The effect of fruit and vegetable intake on risk for coronary heart disease. Ann Intern Med. (2001) 134:1106–14. doi: 10.7326/0003-4819-134-12-200106190-00010
24. Brazaitytė, A, Miliauskienė, J, Vaštakaitė-Kairienė, V, Sutulienė, R, Laužikė, K, Duchovskis, P, et al. Effect of different ratios of blue and red LED light on Brassicaceae microgreens under a controlled environment. Plants Basel Switz. (2021) 10:801. doi: 10.3390/plants10040801
25. Chutimanukul, P, Wanichananan, P, Janta, S, Toojinda, T, Darwell, CT, and Mosaleeyanon, K. The influence of different light spectra on physiological responses, antioxidant capacity and chemical compositions in two holy basil cultivars. Sci Rep. (2022) 12:588. doi: 10.1038/s41598-021-04577-x
26. Kyriacou, MC, Rouphael, Y, Di Gioia, F, Kyratzis, A, Serio, F, Renna, M, et al. Micro-scale vegetable production and the rise of microgreens. Trends Food Sci Technol. (2016) 57:103–15. doi: 10.1016/j.tifs.2016.09.005
27. Mishra, GP, Priti, DHK, Aski, M, Sangwan, S, and Stobdan, T. Microgreens: a novel food for nutritional security In: S Praveen, editor. Conceptualizing plant-based nutrition. Cham: Springer (2022).
28. Llorach, R, Martínez-Sánchez, A, Tomás-Barberán, FA, Gil, MI, and Ferreres, F. Characterisation of polyphenols and antioxidant properties of five lettuce varieties and escarole. Food Chem. (2008) 108:1028–38. doi: 10.1016/j.foodchem.2007.11.032
29. Park, S, Valan Arasu, M, Lee, MK, Chun, JH, Seo, JM, Lee, SW, et al. Quantification of glucosinolates, anthocyanins, free amino acids, and vitamin C in inbred lines of cabbage (Brassica oleracea L.). Food Chem. (2014) 145:77–85. doi: 10.1016/j.foodchem.2013.08.010
30. Ferriz-Martínez, RA, Espinosa-Villarreal, N, Chávez-Servín, JL, Mercado-Luna, A, de la Torre-Carbot, K, Serrano-Arellano, J, et al. Effect of foliar application of hydrogen peroxide macroconcentrations on growth parameters, phenolic compounds and antioxidant capacity in the leaves and seeds of Amaranthus hypochondriacus L. Plan Theory. (2023) 12:1499. doi: 10.3390/plants12071499
31. Slinkard, K, and Singleton, VL. Total phenol analysis: automation and comparison with manual methods. Am J Enol Vitic. (1977) 28:49–55. doi: 10.5344/ajev.1977.28.1.49
32. Kim, MJ, Moon, Y, Tou, JC, Mou, B, and Waterland, NL. Nutritional value, bioactive compounds and health benefits of lettuce (Lactuca sativa L.). J Food Compos Anal. (2016) 49:19–34. doi: 10.1016/j.jfca.2016.03.004
33. Sokolova, D, Shvachko, N, Mikhailova, A, and Popov, V. Betalain content and morphological characteristics of table beet accessions: their interplay with abiotic factors. Agronomy. (2022) 12:1033. doi: 10.3390/agronomy12051033
34. Calva-Estrada, SJ, Jiménez-Fernández, M, and Lugo-Cervantes, E. Betalains and their applications in food: the current state of processing, stability and future opportunities in the industry. Food Chem Mol Sci. (2022) 4:100089. doi: 10.1016/j.fochms.2022.100089
35. Lee, J, Durst, RW, and Wrolstad, RE. Determination of total monomeric anthocyanin pigment content of fruit juices, beverages, natural colorants, and wines by the pH differential method: collaborative study. J AOAC Int. (2005) 88:1269–78. doi: 10.1093/jaoac/88.5.1269
36. Freitag, S, Verrall, SR, Pont, SDA, McRae, D, Sungurtas, JA, Palau, R, et al. Impact of conventional and integrated management systems on the water-soluble vitamin content in potatoes, field beans, and cereals. J Agric Food Chem. (2018) 66:831–41. doi: 10.1021/acs.jafc.7b03509
37. Benzie, IFF, and Strain, JJ. The ferric reducing ability of plasma (FRAP) as a measure of “antioxidant power”: the FRAP assay. Anal Biochem. (1996) 239:70–6. doi: 10.1006/abio.1996.0292
38. Pang, Z, Chong, J, Zhou, G, de Lima Morais, DA, Chang, L, Barrette, M, et al. MetaboAnalyst 5.0: narrowing the gap between raw spectra and functional insights. Nucleic Acids Res. (2021) 49:W388–96. doi: 10.1093/nar/gkab382
39. Alrajhi, AA, Alsahli, AS, Alhelal, IM, Rihan, HZ, Fuller, MP, Alsadon, AA, et al. The effect of LED light spectra on the growth, yield and nutritional value of red and green lettuce (Lactuca sativa). Plan Theory. (2023) 12:463. doi: 10.3390/plants12030463
40. Kopsell, DA, Sams, CE, Barickman, TC, and Morrow, RC. Sprouting broccoli accumulate higher concentrations of nutritionally important metabolites under narrow-band light-emitting diode lighting. J Am Soc Hortic Sci. (2014) 139:469–77. doi: 10.21273/JASHS.139.4.469
41. Rihan, HZ, Aldarkazali, M, Mohamed, SJ, McMulkin, NB, Jbara, MH, and Fuller, MP. A novel new light recipe significantly increases the growth and yield of sweet basil (Ocimum basilicum) grown in a plant factory system. Agronomy. (2020) 10:934. doi: 10.3390/agronomy10070934
42. Wang, J, Lu, W, Tong, Y, and Yang, Q. Leaf morphology, photosynthetic performance, chlorophyll fluorescence, stomatal development of lettuce (Lactuca sativa L.) exposed to different ratios of red light to blue light. Front. Plant Sci. (2016) 7:250. doi: 10.3389/fpls.2016.00250
43. Yudina, L, Sukhova, E, Mudrilov, M, Nerush, V, Pecherina, A, Smirnov, AA, et al. Ratio of intensities of blue and red light at cultivation influences photosynthetic light reactions, respiration, growth, and reflectance indices in lettuce. Biology. (2022) 11:60. doi: 10.3390/biology11010060
44. Izzo, LG, Mickens, MA, Aronne, G, and Gómez, C. Spectral effects of blue and red light on growth, anatomy, and physiology of lettuce. Physiol Plant. (2021) 172:2191–202. doi: 10.1111/ppl.13395
45. Son, KH, and Oh, MM. Leaf shape, growth, and antioxidant phenolic compounds of two lettuce cultivars grown under various combinations of blue and red light-emitting diodes. HortScience. (2013) 48:988–95. doi: 10.21273/HORTSCI.48.8.988
46. Spalholz, H, Perkins-Veazie, P, and Hernández, R. Impact of sun-simulated white light and varied blue:red spectrums on the growth, morphology, development, and phytochemical content of green- and red-leaf lettuce at different growth stages. Sci Hortic. (2020) 264:109195. doi: 10.1016/j.scienta.2020.109195
47. Ying, Q, Kong, Y, Jones-Baumgardt, C, and Zheng, Y. Responses of yield and appearance quality of four Brassicaceae microgreens to varied blue light proportion in red and blue light-emitting diodes lighting. Sci Hortic. (2020) 259:108857. doi: 10.1016/j.scienta.2019.108857
48. Swegarden, H, Sams, CE, and Griffiths, PD. Genetic components of consumer liking based on visual appearance of leaf types in Brassica vegetables. Euphytica. (2021) 217:88. doi: 10.1007/s10681-021-02805-z
49. Cosme, P, Rodríguez, AB, Espino, J, and Garrido, M. Plant phenolics: bioavailability as a key determinant of their potential health-promoting applications. Antioxidants. (2020) 9:1263. doi: 10.3390/antiox9121263
50. Lorenzo, CD, Colombo, F, Biella, S, Stockley, C, and Restani, P. Polyphenols and human health: the role of bioavailability. Nutrients. (2021) 13:273. doi: 10.3390/nu13010273
51. Kwack, Y, Kim, K, Hwang, H, and Chun, C. Growth and quality of sprouts of six vegetables cultivated under different light intensity and quality. Hortic Environ Biotechnol. (2015) 56:437–43. doi: 10.1007/s13580-015-1044-7
52. Qian, H, Liu, T, Deng, M, Miao, H, Cai, C, Shen, W, et al. Effects of light quality on main health-promoting compounds and antioxidant capacity of Chinese kale sprouts. Food Chem. (2016) 196:1232–8. doi: 10.1016/j.foodchem.2015.10.055
53. Taulavuori, K, Hyöky, V, Oksanen, J, Taulavuori, E, and Julkunen-Tiitto, R. Species-specific differences in synthesis of flavonoids and phenolic acids under increasing periods of enhanced blue light. Environ Exp Bot. (2016) 121:145–50. doi: 10.1016/j.envexpbot.2015.04.002
54. Lee, MJ, Son, JE, and Oh, MM. Growth and phenolic compounds of Lactuca sativa L. grown in a closed-type plant production system with UV-A, -B, or -C lamp. J Sci Food Agric. (2013) 94:197–204. doi: 10.1002/jsfa.6227
55. Sakuta, M, Tanaka, A, Iwase, K, Miyasaka, M, Ichiki, S, Hatai, M, et al. Anthocyanin synthesis potential in betalain-producing Caryophyllales plants. J Plant Res. (2021) 134:1335–49. doi: 10.1007/s10265-021-01341-0
56. Liu, S, Wang, X, and Peng, L. Comparative transcriptomic analysis of the metabolism of betalains and flavonoids in red amaranth hypocotyl under blue light and dark conditions. Molecules. (2023) 28:5627. doi: 10.3390/molecules28155627
57. Lee, JH, Kwon, YB, Roh, YH, Choi, IL, Kim, J, Kim, Y, et al. Effect of various LED light qualities, including wide red Spectrum-LED, on the growth and quality of Mini red Romaine lettuce (cv. Breen). Plan Theory. (2023) 12:2056. doi: 10.3390/plants12102056
58. Lee, M, Xu, J, Wang, W, and Rajashekar, CB. The effect of supplemental blue, red and far-red light on the growth and the nutritional quality of red and green leaf lettuce. Am J Plant Sci. (2019) 10:2219–35. doi: 10.4236/ajps.2019.1012157
59. Meas, S, Luengwilai, K, and Thongket, T. Enhancing growth and phytochemicals of two amaranth microgreens by LEDs light irradiation. Sci Hortic. (2020) 265:109204. doi: 10.1016/j.scienta.2020.109204
60. Toscano, S, Cavallaro, V, Ferrante, A, Romano, D, and Patané, C. Effects of different light spectra on final biomass production and nutritional quality of two microgreens. Plan Theory. (2021) 10:1584. doi: 10.3390/plants10081584
61. Bantis, F. Light Spectrum differentially affects the yield and phytochemical content of microgreen vegetables in a plant factory. Plan Theory. (2021) 10:2182. doi: 10.3390/plants10102182
62. Banaś, AK, Aggarwal, C, Łabuz, J, Sztatelman, O, and Gabryś, H. Blue light signalling in chloroplast movements. J Exp Bot. (2012) 63:1559–74. doi: 10.1093/jxb/err429
63. Li, Q, and Kubota, C. Effects of supplemental light quality on growth and phytochemicals of baby leaf lettuce. Environ Exp Bot. (2009) 67:59–64. doi: 10.1016/j.envexpbot.2009.06.011
64. Kong, Y, and Nemali, K. Blue and far-red light affect area and number of individual leaves to influence vegetative growth and pigment synthesis in lettuce. Front Plant Sci. (2021) 12:667407. doi: 10.3389/fpls.2021.667407
65. Meléndez-Martínez, M, Mandić, AI, Bantis, F, Böhm, V, Borge, GIA, Brnčićf, M, et al. A comprehensive review on carotenoids in foods and feeds: status quo, applications, patents, and research needs. Crit Rev Food Sci Nutr. (2021) 62:1999–2049. doi: 10.1080/10408398.2020.1867959
66. Jacob, RA, and Sotoudeh, G. Vitamin C function and status in chronic disease. Nutr Clin Care. (2002) 5:66–74. doi: 10.1046/j.1523-5408.2002.00005.x
67. Paciolla, C, Fortunato, S, Dipierro, N, Paradiso, A, De Leonardis, S, Mastropasqua, L, et al. Vitamin C in plants: from functions to biofortification. Antioxidants. (2019) 8:519. doi: 10.3390/antiox8110519
68. Travica, N, Ried, K, Sali, A, Scholey, A, Hudson, I, and Pipingas, A. Vitamin C status and cognitive function: a systematic review. Nutrients. (2017) 9:960. doi: 10.3390/nu9090960
69. Chen, X, Li, Y, Wang, L, and Guo, W. Red and blue wavelengths affect the morphology, energy use efficiency and nutritional content of lettuce (Lactuca sativa L.). Sci Rep. (2021) 11:8374. doi: 10.1038/s41598-021-87911-7
70. Locato, V, Cimini, S, and De Gara, L. Strategies to increase vitamin C in plants: from plant defense perspective to food biofortification. Front. Plant Sci. (2013) 4:152. doi: 10.3389/fpls.2013.00152
71. Song, Y, Qiu, K, Gao, J, and Kuai, B. Molecular and physiological analyses of the effects of red and blue LED light irradiation on postharvest senescence of pak choi. Postharvest Biol Technol. (2020) 164:111155. doi: 10.1016/j.postharvbio.2020.111155
72. Yan, Z, He, D, Niu, G, Zhou, Q, and Qu, Y. Growth, nutritional quality, and energy use efficiency in two lettuce cultivars as influenced by white plus red versus red plus blue LEDs. Int J Agric Biol Eng. (2020) 13:33–40. doi: 10.25165/j.ijabe.20201302.5135
73. Zhang, L, Ma, G, Yamawaki, K, Ikoma, Y, Matsumoto, H, Yoshioka, T, et al. Regulation of ascorbic acid metabolism by blue LED light irradiation in citrus juice sacs. Plant Sci. (2015) 233:134–42. doi: 10.1016/j.plantsci.2015.01.010
74. Johkan, M, Shoji, K, Goto, F, Hashida, S, and Yoshihara, T. Blue light-emitting diode light irradiation of seedlings improves seedling quality and growth after transplanting in red leaf lettuce. HortScience. (2010) 45:1809–14. doi: 10.21273/HORTSCI.45.12.1809
75. Kumar, S, Sandhir, R, and Ojha, S. Evaluation of antioxidant activity and total phenol in different varieties of Lantana camara leaves. BMC Res Notes. (2014) 7:560. doi: 10.1186/1756-0500-7-560
76. Khiya, Z, Oualcadi, Y, Gamar, A, Berrekhis, F, Zair, T, and Hilali, FE. Correlation of total polyphenolic content with antioxidant activity of hydromethanolic extract and their fractions of the Salvia officinalis leaves from different regions of Morocco. J Chemom. (2021) 2021:1–11. doi: 10.1155/2021/8585313
Keywords: vertical farming, light ratio, antioxidants, controlled environment agriculture, nutritive value enhancement
Citation: Bucky A, Pičmanová M, Porley V, Pont S, Austin C, Khan T, McDougall G, Johnstone A and Stewart D (2024) Light manipulation as a route to enhancement of antioxidant properties in red amaranth and red lettuce. Front. Nutr. 11:1386988. doi: 10.3389/fnut.2024.1386988
Received: 16 February 2024; Accepted: 20 May 2024;
Published: 05 June 2024.
Edited by:
Yifen Lin, Fujian Agriculture and Forestry University, ChinaReviewed by:
Aušra Brazaitytė, Lithuanian Research Centre for Agriculture and Forestry, LithuaniaCopyright © 2024 Bucky, Pičmanová, Porley, Pont, Austin, Khan, McDougall, Johnstone and Stewart. This is an open-access article distributed under the terms of the Creative Commons Attribution License (CC BY). The use, distribution or reproduction in other forums is permitted, provided the original author(s) and the copyright owner(s) are credited and that the original publication in this journal is cited, in accordance with accepted academic practice. No use, distribution or reproduction is permitted which does not comply with these terms.
*Correspondence: Annika Bucky, YS5idWNreS4yMkBhYmRuLmFjLnVr
Disclaimer: All claims expressed in this article are solely those of the authors and do not necessarily represent those of their affiliated organizations, or those of the publisher, the editors and the reviewers. Any product that may be evaluated in this article or claim that may be made by its manufacturer is not guaranteed or endorsed by the publisher.
Research integrity at Frontiers
Learn more about the work of our research integrity team to safeguard the quality of each article we publish.