- 1Biomedical Research Center, Qatar University, Doha, Qatar
- 2QU Health, Qatar University, Doha, Qatar
Obesity is a major health problem that affects millions of individuals, and it is associated with metabolic diseases including insulin resistance (IR), type 2 diabetes (T2D), and cardiovascular diseases (CVDs). However, Body fat distribution (BFD) rather than crude obesity is now considered as a more accurate factor associated with these diseases. The factors affecting BFD vary, from genetic background, epigenetic factors, ethnicity, aging, hormonal changes, to lifestyle and medication consumptions. The main goal of controlling BFD comes from the fact that fat accumulation in different depots has a different effect on the overall health and metabolic health of individuals. It is well established that fat storage in the abdominal visceral depot is associated with metabolic disorder occurrence, while gluteal-femoral subcutaneous fat depot seems to be protective against these diseases. In this paper, we will summarize the factors affecting fat distribution. Then, we will present evidence connecting gluteal-femoral fat depot with protection against metabolic disorders including IR, T2D, and CVDs. Finally, we will list the suggested mechanisms that lead to this protective effect. The abstract is visualized in Graphical Abstract.
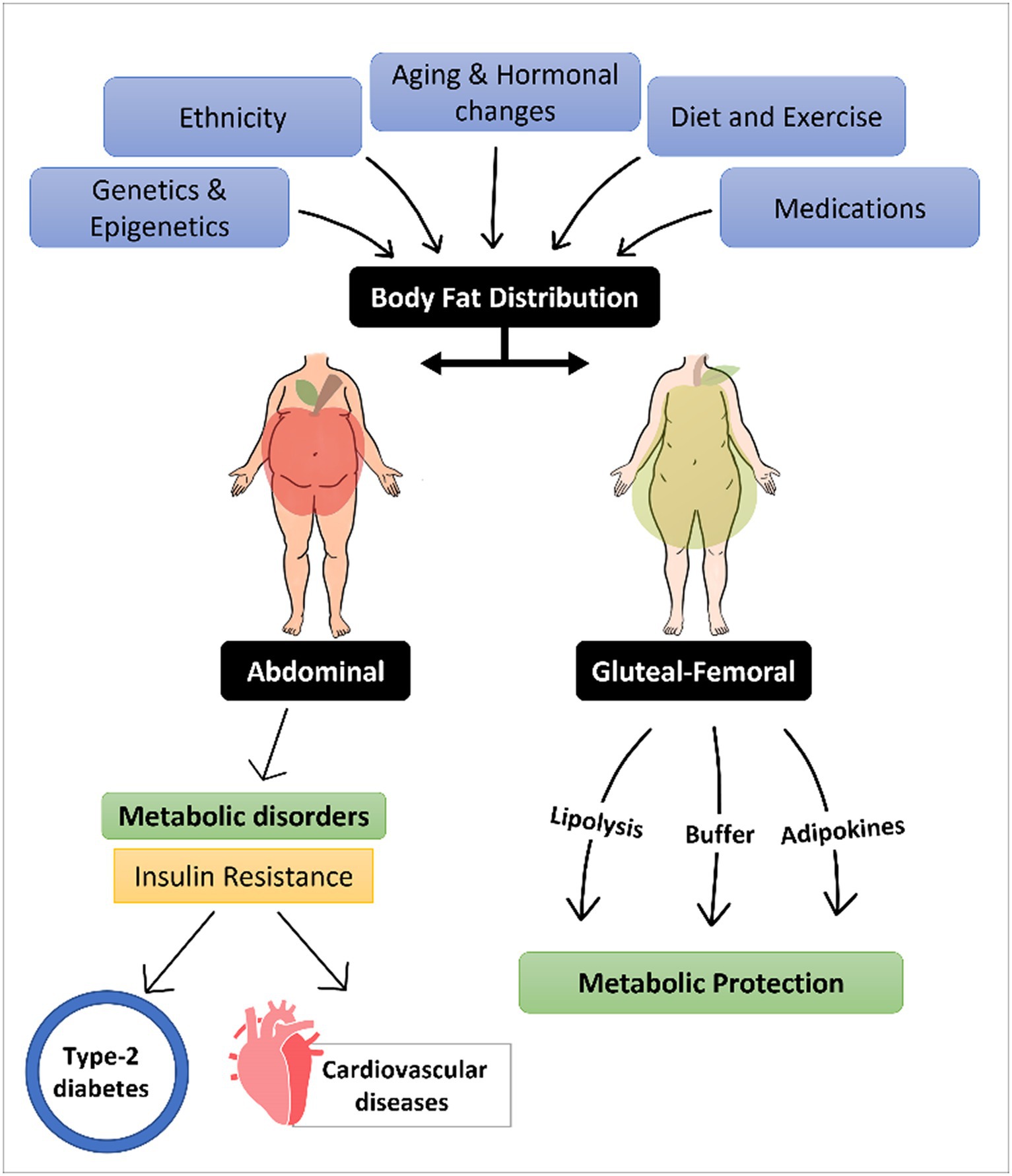
Graphical Abstract. Graphical abstract showing a summary of the factors affecting body fat distribution, the difference between abdominal and gluteal-femoral obesity on metabolic disorders progression, and the protective mechanisms of gluteal-femoral fat.
1 Introduction
Obesity is a major public health problem, affecting millions of individuals worldwide. According to World Obesity Atlas (1), the proportion of the obese population (BMI ≥ 30) will reach 17% in 2025, and 20% in 2030 affecting more than 1.5 billion people. It is noteworthy to mention that COVID-19 pandemic has worsened the obesity epidemic, and the restrictions from 2020 to 2022 have increased the risk of weight gain by increasing sedentary and unhealthy dietary behaviors, and significantly reduced access to health care (2).
Obesity is a complex multifactorial disease, meaning it is caused by multiple interacting factors, including genetics, environmental, and behavioral factors (3). The long-term serious effect of obesity lies in the increasing likelihood of many diseases, including insulin resistance (IR) and type 2 diabetes (T2D) (4), chronic kidney disease (CKD) (5), non-alcoholic fatty liver disease (NAFLD), and some cancers (6). Additionally, both obesity and T2D increase the prevalence of cardiovascular disorders (CVDs) (7). Although obesity and BMI represent a good indicator of the overall metabolic health profile, studies have shown that the distribution of body fat into fat depots plays a more important role in the co-morbidity of the metabolic diseases (8). This indicates that BFD measurements along BMI need to be considered to assess metabolic health. These measurements include simple waist to hip ratio, where the waist circumference (reflecting abdominal adiposity) is divided by the hip circumference [reflecting gluteal femoral (GF) fat adiposity]. Other more accurate measurements include body imaging to measure actual fat depot sizes.
Adipose tissue functions not only as a fat storage organ, but also as a dynamic organ (9); therefore, fat storage in different depots have diverse impact on human health. In fact, each fat depot contributes differently as metabolic and endocrine organ, leading to different levels of metabolic disorders (10). It is well established that over-accumulating fat in the abdominal fat depot is associated with metabolic disorders; Increases of abdominal adiposity as opposed to overall adiposity, is associated with increases in the likelihood of getting T2D and CVDs 4 (11). Some studies showed hip circumference and thigh fat adiposity alone as an independent indicator of obesity-related metabolic risks (12). More recent evidence suggests that GF fat depot shows a protective role against these metabolic disorders; fat storage in the GF depot is preferred over storage in the abdominal depot to gain a metabolically healthy state (13–16). These opposing relationships reflect the unique intrinsic characteristics of different fat depots.
Explaining the factors that lead to distinct BFD and resolving the mechanisms behind the protective role of some depots, may help in finding novel therapeutic venues for preventing or treating obesity-related diseases. In this review, we summarized the important factors affecting BFD, whether uncontrollable (genetics, epigenetic factors, age, and hormonal profile) or controllable (diet, exercise, and consumption of certain medications). Then, we compiled detailed evidence supporting the protective role of the GF fat depot against metabolic disorders, including IR, T2D, and CVDs. Finally, we summarized the reported possible mechanisms explaining how this protection is accomplished.
2 Fat depots and gluteal-femoral fat biology
Adipose fat depots are the specific location where the fat tissue is built and located in the body. The human body stores fat in 2 anatomically and physiologically distinct fat depots; subcutaneous adipose tissue (SCAT) and visceral adipose tissue (VAT). SCAT is located between the skin and superficial musculature, and it can be further classified into superficial SCAT and deep SCAT (17). The main depots where SCAT is stored are the inguinal region (GF regions), and the anterior and the back of the abdominal wall as shown in Figure 1. On the other hand, VAT depot, also known as intra-abdominal fat, is the fat tissue stored within/between the visceral organs, such as liver and intestines (8). Physiologically, VAT is more vascular, innervated, and contains less preadipocyte differentiating capacity, greater percentage of large adipocytes and larger number of inflammatory and immune cells as compared to SCAT (18). Individuals with enhanced abdominal obesity, more specifically with high VAT depot content are referred to apple shaped, as compared to pear shaped individuals that express higher GF content (19), as illustrated in Graphical Abstract.
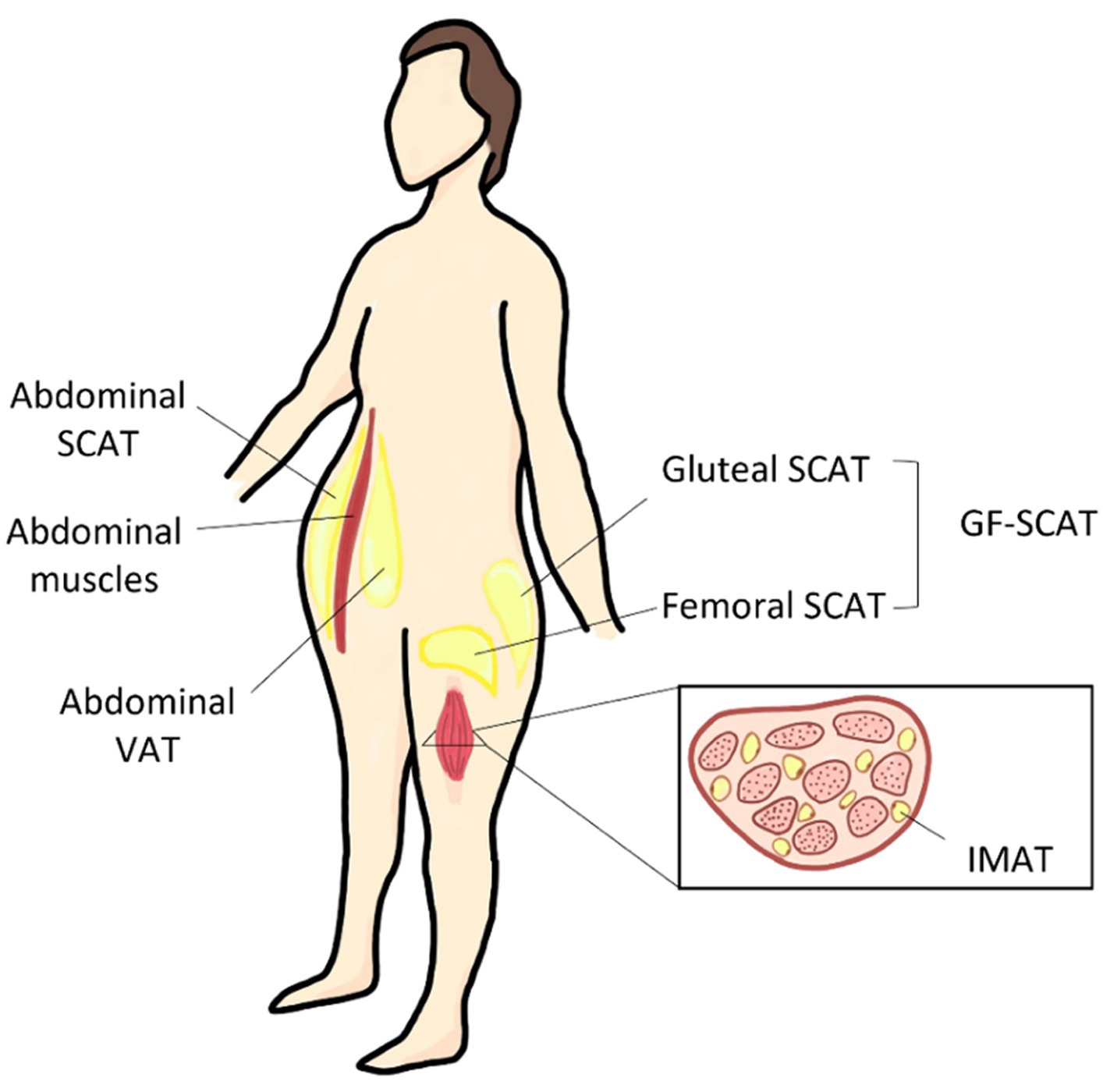
Figure 1. Different body fat depots and their anatomical location. SCAT, subcutaneous adipose tissue; VAT, visceral adipose tissue; GF, gluteal-femoral adipose tissue; and IMAT, intermuscular adipose tissue.
The GF fat as a combined depot is referred to as gluteal-femoral fat (GF fat/GF adipose tissue) as shown in Figure 1. In literature, GF fat is referred to as thigh, hip, or lower body fat, and could be further classified into different depots. These depots differ in their biology, histology, and physiological role. GF subcutaneous adipose tissue (GF-SCAT) is the fat tissue stored under the skin of the lower body part. Another thigh fat depot is the thigh intermuscular adipose tissue (thigh IMAT) illustrated in Figure 1, which is considered as an ectopic fat depot; fat stored in tissues other than specialized adipose tissue, skeletal muscle tissue in this case (8). In the following section, we discussed the factors affecting BFD, favoring specific depots over the others.
3 Factors affecting body fat distribution
Body fat distribution (BFD) is affected and mediated by multiple factors. Genetic background plays a significant role in determining BFD. Studies have shown that certain genes are associated with increased abdominal fat, while others are associated with increased fat in the hips and thighs (20–25). Epigenetic differences, which impact obesity, may also have an influence on body fat distribution (26–28). Ethnicity, aging, and hormonal changes also play a role in BFD (7, 29, 30). On the other hand, certain factors can be induced to manipulate BFD. Diet restriction and exercise can lead to fat reduction in the abdominal region (31). Certain medications, such as those used to treat diabetes or high blood pressure, can also lead to a redistribution in body fat (19). The factors that affect BFD are summarized in Table 1.
4 Gluteal-femoral fat protection against insulin resistance
Insulin resistance (IR) is a defined as a state where the body cells become irresponsive to the physiological insulin levels secreted in the blood (46). IR is a risk factor for many chronic metabolic disorders. The major consequence of IR is the progression of type 2 diabetes, where individuals diagnosed with IR are expected to develop T2D in 5 ~ 10 years (46). T2D is highly diverse disease. To personalize the treatment of T2D, a study attempted to classify the disease into clusters, depending on six variables: including glutamate decarboxylase antibodies, age, BMI, HbA1c, and homoeostatic model assessment 2 estimates of β-cell function and insulin resistance. The significance of this classification is to generate a way to estimate the risk of diabetic complications, which they successfully showed in their study. According to the researchers, one cluster (highest insulin resistance) showed more association to kidney disease, another cluster (insulin deficient) showed the highest risk of retinopathy, while a third cluster associated with higher fat liver, indicating a relevance of VAT to IR (47).
Previously, obesity was believed to be a major leading cause of IR. However, recent investigation showed that abdominal obesity is positively associated with IR, while considerable debate remains concerning the potential of a positive effect of thigh fat in metabolic protection against IR progression (19). This theory explains the metabolically healthy obese individuals and how their bodies escape the negative metabolic consequences of obesity (48). Thigh fat is protective against IR; a study demonstrated that lower thigh SCAT and higher VAT both contribute to insulin resistance profile. The study was conducted on a cohort from the general population, it demonstrated that high thigh SCAT alone or low VAT alone were positively associated with IS state while Individuals with combination of high SCAT and low IMAT had the highest IS state among all groups (49). A more recent study investigated both thigh fat depots (thigh SCAT and thigh IMAT) in a female cohort. They showed that higher thigh-SCAT and lower thigh-IMAT are associated with insulin sensitivity (14). The same findings were supported with studies done on children obese cohort (age of 7–17 years old), where a study suggested a protective effect of thigh fat, and its association to a healthy metabolic profile [more favorable HOMA-IR score, triglyceride serum level, systolic blood pressure (SBP), C-reactive protein (CRP), resistin, high-density lipoprotein (HDL), cholesterol, adiponectin, and blood lipid profile] (15). Whereas another study conducted on postmenopausal women suggested that thigh fat was associated with a favorable metabolic profile, including serum triglycerides, HDL-cholesterol, and various IR markers (50). Due to the reported association between thigh fat and protection against IR, a study suggested that regional adiposity, including thigh fat size could be used as a predictive way to predict the risk of developing IR. They reported that each increase in thigh fat led to a 59% decrease in the odds of becoming insulin resistant, independently from confounders (age, BMI, visceral adiposity, and gender) (51).
It has been reported that a larger gluteal femoral fat is associates with lower prevalence of IR, prediabetes, and dyslipidemia (52). However, as explained in the previous section, not all thigh fat seems to be protective. A study assessed critical biomarkers and how their levels are different among individuals with different BFDs. The findings suggested a significantly lower insulin resistance and triglycerides levels in individuals with higher GF fat (13), but according to Snijder et al. (52), only thigh-SCAT was found to be associated with favorable metabolic profiles. Higher thigh-SCAT was shown to be associated with lower transformed triglycerides (in both genders), higher HDL-cholesterol, and lower blood glucose level (in males only).
The revised studies showed how GF-SCAT is beneficial as a protective fat depot against metabolic disease. In contrast, IMAT is an ectopic depot for fat storage. In contrary with SCAT, fat deposition in IMAT is highly associated with increase IR and T2D (53). One suggested mechanism of how IMAT promotes IR progression is because fat accumulation within the thigh muscular tissue leads to reduced blood circulation, and decreased insulin uptake efficiency by the muscle cells (54). These studies confirm the association between GF SCAT levels, not GF IMAT, and developing IR leading to multiple metabolic disorders, including type 2 diabetes and cardiovascular disease as explained in the following section.
5 Gluteal-femoral fat cardio protection
Cardiovascular diseases (CVDs) are a general term that describes a range of pathologies affecting the health of the heart, the blood vessels, or the pericardium. It is critical to study the risk factors affecting and leading to CVDs as these diseases can be fatal. Previous studies have shown a clear association between BFD and the risk of developing CVDs (19, 50, 55). While increased fat storage in the abdominal depot; mainly ectopic visceral fat is associated with high CVDs progression risk, high GF fat storage was shown to be cardioprotective (56).
The cardioprotective role of GF fat has been confirmed in many studies involving diverse human subjects with different ages, BMIs, and diseases. As early as 1991, thigh fat has a negative association with cardiovascular diseases was first hypothesized. High thigh fat was shown to contribute to lipoprotein plasma profile; an indicator of lower risk of cardiovascular diseases (57). Following that finding, more research groups started showing the same correlation among different models. Accumulating evidence shows an association between increased lower body adiposity and GF fat and healthy lipid profile. Many studies showed evidence that elevated GF fat is associated with better serum lipid levels, including lower low-density lipoprotein-cholesterol profile, and higher high-density-cholesterol levels, which are indicators of improved cardiovascular health8. Van Pelt et al. (50) showed an enhanced triglycerides level in individuals with higher GT fat as compared to others with the same BMI.
In addition to improved serum lipid profile, increased GF mass has been shown to be directly associated with enhanced vascular health, including lower aortic calcification, decreased progression of present aortic calcification, as well as decreased arterial stiffness in a women cohort (58). Furthermore, studies showed a direct effect between GF fat and cardiac diseases. Yusuf et al. (59), conducted a study that involved 27,000 participants, they showed a negative association between hip circumference and the risk of myocardial infarction. Another study confirmed that a larger hip circumference is associated with lower risk of developing coronary heart disease in a population involving both genders (60). Shay et al. (16) reported that women characterized with higher GF fat content and suffer from type-1-diabetes have shown less risk of developing coronary artery disease, which was not shown in men.
The protective effect of GF fat against CVD risk persists even with aging. A study was conducted on healthy elderly women, they confirmed a strong association between GF-fat and protection against vascular damage (55). These studies confirm the tight association between GF levels and the risk of CVDs. More studies were well revised by Manolopoulos et al. (61). In the following section, we will revise the reported mechanisms that explain this association, as well as the possible mechanisms that need more investigation to be proven.
6 Mechanisms of gluteal-femoral fat metabolic protection
As mentioned previously, thigh fat shows protective properties against IR and CVDs. While the mechanism behind this association remains not fully understood, research suggests three major mechanisms by which this protection is achieved. These mechanisms ultimately enhance metabolic health and lower the risk of developing IR and CVDs.
6.1 Catecholamine-mediated lipolysis
Lipids are mobilized by adipocyte lipolysis, a fundamental process of hydrolyzing triacylglycerol to fatty acids for internal or systemic energy use. The rate of lipolysis is low in the subcutaneous femoral-gluteal region, intermediate in the subcutaneous abdominal region and high in the visceral region (62). Indeed, abdominal adipocytes showed β-adrenergic lipolytic sensitivity 10–20-fold greater than gluteal adipocytes; this is due to an increase in the total number of β-adrenoceptors in this depot (63).
A study showed that the steady-state mRNA levels of beta-adrenergic receptors BAR 1 and BAR 2 were about twice as high in abdominal as in gluteal adipocytes of men and women (p < 0.01) explained by an increased expression of the genes that encode for BAR 1 and BAR 2 (64). In women, variation in the affinity properties of the α-2 adrenoceptor is an additional factor. Abdominal adipocytes showed a 40 times lower α-2 adrenergic antilipolytic sensitivity than did gluteal adipocytes (63).
The decreased action of β-adrenergic receptors and increased activity of α2-adrenergic adrenoceptors in combination with defects in hormone sensitive lipase function inhibits the lipolytic effect of catecholamines in subcutaneous fat cells, whereas increased activity of β-adrenergic receptors and decreased activity of α2-adrenoceptors increases the lipolytic response in visceral fat cells. These abnormalities in catecholamine function promote release of free fatty acids from the visceral fat cells to the liver through the portal system and might cause several of the metabolic complications to upper-body obesity (62).
6.2 Gluteal-femoral adipose depot acts as a buffer to control excess lipids
The protective properties of GF fat against insulin resistance and cardiovascular diseases relies mainly on the fact that it is physiologically different from other fat depots, especially abdominal fat depot. This mechanism suggests that the protection is due to the nature of thigh depot. Most of the thigh stored fat is stored in the subcutaneous fat depot (GF SCAT) rather than the ectopic thigh depot (GF IMAT). Fat storage in the GF SCAT depot keeps the excess fat away from the visceral region, which is linked to high risk of metabolic disorder progression. Additionally, accumulating evidence from in vivo and in vitro studies showed a differential regulation of GF SCAT fat mechanisms of fatty acid uptake and release at the adipocyte level. In case of increased dietary lipid intake, GF SCAT depot becomes a long-term store of free fatty acids, preventing them from accumulating in ectopic fat depots, especially in the viscera and within vital organs (65). This is likely since GF fat depot has a relatively high lipoprotein lipase activity as compared to other depots, making it a better reservoir of excess circulating fatty acids. This storage prevents lipid overflow within abdominal visceral depot, causing reduced lipotoxicity, as well as decreased risk of IR and CVDs on the long run (13).
This theory simply shows that the protective association is due to the absence of the risk, rather than an actual protection mechanism (66). However, more recent research has shown other mechanisms that contribute to this protection. According to Tran et al. (67), the protective effect of GF SCAT is not due to its depot, but rather to intrinsic differences between this depot and visceral fat depot. This research group assessed how transplanting GF SCAT from healthy mice to the visceral depot of metabolically unhealthy mice, which lead to enhanced metabolic profile in the recipient mice, including decreased total body weight, fat mass, blood glucose, and insulin levels. These findings recommend that the difference is not as simple as GF SCAT working as a buffer to control excess fat from getting stored in the visceral region. GF SCAT certainly plays a more crucial role at the physiological level to gain this protection and improve insulin sensitivity. Recent studies suggest that GF SCAT is intrinsically different from other depots as is it plays a critical role as an endocrine organ that is associated with a protective adipokine profile. The protective role of GF SCAT as an endocrine organ is discussed in detail in the next section.
6.3 Gluteal-femoral adipose depot contributes to lipoprotein profile and secretes protective adipokines
Lipoproteins are molecular structures that consist of proteins associated with fat with the main function to transport lipids (triglycerides and cholesterols) throughout the body (68) and serve an important role in metabolic health. The two major types of lipoproteins are the high-density lipoprotein (HDL) and low-density lipoprotein (LDL), which are often referred to as “good” and “bad” cholesterol in the metabolic disorder context. Terry et al. (57) showed a positive correlation between thigh fat and HDL levels, and where the first to suggest that thigh fat contributes to plasma lipoprotein profiles and might predict lower risk of metabolic disorders, mainly CVDs.
Conversely, Adipokines are special cytokines secreted by adipose tissue components, including adipocytes and other cells as macrophages. Adipokines (adiponectin and Leptin) are mainly produced by the adipocyte component of the adipose tissue, while interleukins (such as IL-6) are produced by residential macrophages (61). It is known that a healthy adipokine profile is associated with reduced risk developing CVDs. Recent studies suggested that GF depot has a different secretome profile than other depots. It was shown to be associated with elevated levels of beneficial adipokines, and less pro-inflammatory adipokines as opposed to abdominal fat depot.
6.3.1 Leptin
Leptin is a critical adipokine in terms of energy metabolism by controlling energy intake and storage as a fat mass (69). Leptin is predominantly expressed by isolated subcutaneous adipocytes as opposed to omental adipocytes, particularly in women (70). Leptin secretion levels are higher in SCAT as compared to VAT. In terms of mRNA expression of Leptin, no previous studies have compared leptin expression level among different SCATs. However, some studies indirectly suggested that the basal expression level of leptin does not differ among SCAT, and it is the same among abdominal SCAT and thigh SCAT (71). As a secreted adipokine, leptin levels negatively correlate with waist to hip ratio (72). A study conducted by Picó et al. (73) reported that the hormone leptin plays a key role in regulating the size and function of fat depots. Based on that, the authors suggest that targeting the leptin pathway may be a potential therapeutic strategy for managing metabolic disorders (73). All previous studies recommend a differential level of leptin and its association with fat distribution. However, the direct association and definition of leptin level as a mechanism of thigh fat protection is an area that needs more investigation.
6.3.2 Adiponectin
Adiponectin is a hormone, an adipokine associated with insulin sensitivity and inflammation. Low levels of adiponectin are associated with increased risk of several metabolic diseases, including CVDs. Recently, adiponectin levels were shown to be significantly lower in individuals with high abdominal fat depot, while adiponectin levels were higher with high GF fat depot (74). This opposing effect of different depots on differential serum adiponectin levels lead a more recent study to assess that as a suggested mechanism of GF fat cardioprotection (75). Gradidge et al. (75) conducted a cross sectional study on a female cohort. They first confirmed the positive association of GF fat with adiponectin serum levels. Then, they proved a negative correlation between GF fat with triglyceride level and insulin resistance, a critical risk factors of developing CVDs (75). Although the study was not conclusive, it gives insight of a tight association between GF fat, adiponectin serum levels, and CVD risk factors (75). Another study showed that higher thigh fat results in higher adiponectin level, leading to enhanced insulin sensitivity (76). In conclusion, a higher GF fat is associated with higher adiponectin secretion and enhanced metabolic health.
6.3.3 IL-6
Interleukin-6 (IL-6) is an inflammatory cytokine produced by multiple tissues including adipose tissue, mainly by macrophages residing in the tissue (77). The secreted level of IL-6 correlates with obesity, the higher the BMI the higher the IL-6 levels in the plasma (78). In terms of different depots, IL-6 increases with visceral fat mass increase, and is negatively correlated with thigh fat mass (79). A study compared the IL-6 profile among obese individuals expressing different BFD profiles with and without metabolic syndrome. They first showed that BFD explains the difference between the obese participants in metabolic health, which they linked to higher levels of IL-6 blood levels, concluding that individuals with a more favorable BFD (lower VAT and higher thigh IMAT) express a more favorable inflammatory profile and metabolic health as compared to their unhealthy counterparts (same BMI, gender, and age group) (48). There are not enough studies to show a causality link between IL-6 profile and thigh fat protective role, and more investigation is needed in this area.
6.3.4 DPP4
DPP4 plays a major role in glucose metabolism. It is responsible for the degradation of incretins such as GLP-1. A comprehensive proteomic profiling of the human adipocyte secretome identified dipeptidyl peptidase 4 (DPP4) as a novel adipokine that may impair insulin sensitivity in an autocrine and paracrine fashion, the protein levels of DPP4 are fivefold higher in VAT compared with SAT in a cohort of obese patients (80).
7 Conclusion
The BFD is a highly accurate indicator of metabolic health due to its association with IR, T2D, and CVDs. Th factors that contribute to fat distribution include genetic background, age, hormonal changes, lifestyle (diet and exercise), and medication consumption. It is necessary to understand BFD, as fat storage in certain depots shows a protective effect against metabolic disorders. The gluteal femoral fat depot is one of these depots. Many reports showed its protection against IR, and its metabolic complications (T2D and CVDs). The mechanisms of this protection include three major mechanisms: first, GF depot demonstrates a lower rate of lipolysis. Second, GF depot acts as a metabolic reservoir of excess lipids, preventing them from being stored in the visceral fat depot. And finally, GF depot has a unique physiological nature of GF in terms of lipoproteins, adipokines, and cytokines secretion, it produces protective cytokines (leptin and adiponectin) in higher levels as compared to other depots, while secreting lower levels of proinflammatory adipokines and cytokines (DPP4 ND IL-6). The protective effect of GF fat is interesting, and the mechanisms behind it is still an active area of research that merits further studying to be fully understood.
Author contributions
MA: Investigation, Visualization, Writing – original draft. KN: Supervision, Writing – review & editing. ME: Conceptualization, Funding acquisition, Project administration, Supervision, Writing – review & editing.
Funding
The author(s) declare that financial support was received for the research, authorship, and/or publication of this article. This research was funded by the Qatar National Research Fund (QNRF) (grant no. NPRP13S-1230-190008).
Acknowledgments
The authors would like to thank Qatar Foundation for funding this project.
Conflict of interest
The authors declare that the research was conducted in the absence of any commercial or financial relationships that could be construed as a potential conflict of interest.
Publisher’s note
All claims expressed in this article are solely those of the authors and do not necessarily represent those of their affiliated organizations, or those of the publisher, the editors and the reviewers. Any product that may be evaluated in this article, or claim that may be made by its manufacturer, is not guaranteed or endorsed by the publisher.
References
1. World Obesity Federation Global Obesity Observatory. (2023). World obesity day atlases | obesity atlas. Available at: https://data.worldobesity.org/publications/?cat=19
2. Micheletti Cremasco, M, Mulasso, A, Moroni, A, Testa, A, Degan, R, Rainoldi, A, et al. Relation among perceived weight change, sedentary activities and sleep quality during COVID-19 lockdown: a study in an academic Community in Northern Italy. Int J Environ Res Public Health. (2021) 18:2943. doi: 10.3390/ijerph18062943
3. Lin, X, and Li, H. Obesity: epidemiology, pathophysiology, and therapeutics. Front Endocrinol. (2021) 12:706978. doi: 10.3389/fendo.2021.706978
4. Goran, MI, Ball, GDC, and Cruz, ML. Obesity and risk of type 2 diabetes and cardiovascular disease in children and adolescents. J Clin Endocrinol Metab. (2003) 88:1417–27. doi: 10.1210/jc.2002-021442
5. Kovesdy, CP, Furth, SL, and Zoccali, C. Obesity and kidney disease. Can J Kidney Health Dis. (2017) 4:205435811769866. doi: 10.1177/2054358117698669
6. Divella, R, Mazzocca, A, Daniele, A, Sabbà, C, and Paradiso, A. Obesity, non-alcoholic fatty liver disease and adipocytokines network in promotion of Cancer. Int J Biol Sci. (2019) 15:610–6. doi: 10.7150/ijbs.29599
7. Frank, AP, de Souza, SR, Palmer, BF, and Clegg, DJ. Determinants of body fat distribution in humans may provide insight about obesity-related health risks. J Lipid Res. (2019) 60:1710–9. doi: 10.1194/jlr.R086975
8. Chait, A, and den Hartigh, LJ. Adipose tissue distribution, inflammation and its metabolic consequences, including diabetes and cardiovascular disease. Front Cardiovasc Med. (2020) 7:22. doi: 10.3389/fcvm.2020.00022
9. Palavicini, JP, Chavez-Velazquez, A, Fourcaudot, M, Tripathy, D, Pan, M, Norton, L, et al. The insulin-sensitizer pioglitazone remodels adipose tissue phospholipids in humans. Front Physiol. (2021) 12:784391. doi: 10.3389/fphys.2021.784391
10. Hermsdorff, HHM, and Monteiro, JBR. Visceral, subcutaneous or intramuscular fat: where is the problem? Arq Bras Endocrinol Metabol. (2004) 48:803–11. doi: 10.1590/S0004-27302004000600005
11. Kobayashi, M, Ogawa, S, Tayama, J, Sagara, I, Takeoka, A, Bernick, P, et al. Intra-abdominal fat accumulation is an important predictor of metabolic syndrome in young adults. Medicine. (2020) 99:e22202. doi: 10.1097/MD.0000000000022202
12. Lissner, L, Björkelund, C, Heitmann, BL, Seidell, JC, and Bengtsson, C. Larger hip circumference independently predicts health and longevity in a Swedish female cohort. Obes Res. (2001) 9:644–6. doi: 10.1038/oby.2001.85
13. Yim, JE, Heshka, S, Albu, JB, Heymsfield, S, and Gallagher, D. Femoral-gluteal subcutaneous and intermuscular adipose tissues have independent and opposing relationships with CVD risk. J Appl Physiol Bethesda Md. (1985) 104:700–7. doi: 10.1152/japplphysiol.01035.2007
14. Goss, AM, and Gower, BA. Insulin sensitivity is associated with thigh adipose tissue distribution in healthy postmenopausal women. Metabolism. (2012) 61:1817–23. doi: 10.1016/j.metabol.2012.05.016
15. Samouda, H, De Beaufort, C, Stranges, S, Hirsch, M, Van Nieuwenhuyse, JP, Dooms, G, et al. Cardiometabolic risk: leg fat is protective during childhood. Pediatr Diabetes. (2016) 17:300–8. doi: 10.1111/pedi.12292
16. Shay, CM, Secrest, AM, Goodpaster, BH, Kelsey, SF, Strotmeyer, ES, and Orchard, TJ. Regional adiposity and risk for coronary artery disease in type 1 diabetes: does having greater amounts of gluteal-femoral adiposity lower the risk? Diabetes Res Clin Pract. (2010) 89:288–95. doi: 10.1016/j.diabres.2010.03.028
17. Wronska, A, and Kmiec, Z. Structural and biochemical characteristics of various white adipose tissue depots. Acta Physiol. (2012) 205:194–208. doi: 10.1111/j.1748-1716.2012.02409.x
18. Pond, CM. The evolution of mammalian adipose tissues In: ME Symonds, editor. Adipose tissue biology. Cham: Springer International Publishing (2017). 1–59.
19. Alser, M, and Elrayess, M. From an apple to a pear: moving fat around for reversing insulin resistance. Int J Environ Res Public Health. (2022) 19:14251. doi: 10.3390/ijerph192114251
20. Neville, MJ, Wittemans, LBL, Pinnick, KE, Todorčević, M, Kaksonen, R, Pietiläinen, KH, et al. Regional fat depot masses are influenced by protein-coding gene variants. PLoS One. (2019) 14:e0217644. doi: 10.1371/journal.pone.0217644
21. Justice, AE, Karaderi, T, Highland, HM, Young, KL, Graff, M, Lu, Y, et al. Protein-coding variants implicate novel genes related to lipid homeostasis contributing to body-fat distribution. Nat Genet. (2019) 51:452–69. doi: 10.1038/s41588-018-0334-2
22. Loh, NY, Minchin, JEN, Pinnick, KE, Verma, M, Todorčević, M, Denton, N, et al. RSPO3 impacts body fat distribution and regulates adipose cell biology in vitro. Nat Commun. (2020) 11:2797. doi: 10.1038/s41467-020-16592-z
23. Shungin, D, Winkler, TW, Croteau-Chonka, DC, Ferreira, T, Locke, AE, Mägi, R, et al. New genetic loci link adipose and insulin biology to body fat distribution. Nature. (2015) 518:187–96. doi: 10.1038/nature14132
24. Heid, IM, Jackson, AU, Randall, JC, Winkler, TW, Qi, L, Steinthorsdottir, V, et al. Meta-analysis identifies 13 new loci associated with waist-hip ratio and reveals sexual dimorphism in the genetic basis of fat distribution. Nat Genet. (2010) 42:949–60. doi: 10.1038/ng.685
25. Fox, CS, Liu, Y, White, CC, Feitosa, M, Smith, AV, Heard-Costa, N, et al. Genome-wide association for abdominal subcutaneous and visceral adipose reveals a novel locus for visceral fat in women. PLoS Genet. (2012) 8:e1002695. doi: 10.1371/journal.pgen.1002695
26. Karastergiou, K, Fried, SK, Xie, H, Lee, MJ, Divoux, A, Rosencrantz, MA, et al. Distinct developmental signatures of human abdominal and gluteal subcutaneous adipose tissue depots. J Clin Endocrinol Metab. (2013) 98:362–71. doi: 10.1210/jc.2012-2953
27. Hilton, C, Neville, MJ, Wittemans, LBL, Todorcevic, M, Pinnick, KE, Pulit, SL, et al. MicroRNA-196a links human body fat distribution to adipose tissue extracellular matrix composition. EBioMedicine. (2019) 44:467–75. doi: 10.1016/j.ebiom.2019.05.047
28. Divoux, A, Karastergiou, K, Xie, H, Guo, W, Perera, RJ, Fried, SK, et al. Identification of a novel lncRNA in gluteal adipose tissue and evidence for its positive effect on preadipocyte differentiation. Obes Silver Spring Md. (2014) 22:1781–5. doi: 10.1002/oby.20793
29. Sun, C, Kovacs, P, and Guiu-Jurado, E. Genetics of body fat distribution: comparative analyses in populations with European, Asian and African ancestries. Genes. (2021) 12:841. doi: 10.3390/genes12060841
30. St-Onge, MP, and Gallagher, D. Body composition changes with aging: the cause or the result of alterations in metabolic rate and macronutrient oxidation? Nutr Burbank Los Angel Cty Calif. (2010) 26:152–5. doi: 10.1016/j.nut.2009.07.004
31. Gallagher, D, Heshka, S, Kelley, DE, Thornton, J, Boxt, L, Pi-Sunyer, FX, et al. Changes in adipose tissue depots and metabolic markers following a 1-year diet and exercise intervention in overweight and obese patients with type 2 diabetes. Diabetes Care. (2014) 37:3325–32. doi: 10.2337/dc14-1585
32. Rohde, K, Klös, M, Hopp, L, Liu, X, Keller, M, Stumvoll, M, et al. IRS1 DNA promoter methylation and expression in human adipose tissue are related to fat distribution and metabolic traits. Sci Rep. (2017) 7:12369. doi: 10.1038/s41598-017-12393-5
33. Haffner, SM, Stern, MP, Hazuda, HP, Pugh, J, Patterson, JK, and Malina, R. Upper body and centralized adiposity in Mexican Americans and non-Hispanic whites: relationship to body mass index and other behavioral and demographic variables. Int J Obes. (1986) 10:493–502.
34. Juppi, HK, Sipilä, S, Fachada, V, Hyvärinen, M, Cronin, N, Aukee, P, et al. Total and regional body adiposity increases during menopause-evidence from a follow-up study. Aging Cell. (2022) 21:e13621. doi: 10.1111/acel.13621
35. Sabag, A, Way, KL, Keating, SE, Sultana, RN, O’Connor, HT, Baker, MK, et al. Exercise and ectopic fat in type 2 diabetes: a systematic review and meta-analysis. Diabetes Metab. (2017) 43:195–210. doi: 10.1016/j.diabet.2016.12.006
36. Verheggen, RJHM, Maessen, MFH, Green, DJ, Hermus, ARMM, Hopman, MTE, and Thijssen, DHT. A systematic review and meta-analysis on the effects of exercise training versus hypocaloric diet: distinct effects on body weight and visceral adipose tissue. Obes Rev Off J Int Assoc Study Obes. (2016) 17:664–90. doi: 10.1111/obr.12406
37. Gepner, Y, Shelef, I, Schwarzfuchs, D, Zelicha, H, Tene, L, Yaskolka Meir, A, et al. Effect of distinct lifestyle interventions on mobilization of fat storage pools: CENTRAL magnetic resonance imaging randomized controlled trial. Circulation. (2018) 137:1143–57. doi: 10.1161/CIRCULATIONAHA.117.030501
38. Knerr, PJ, Mowery, SA, Douros, JD, Premdjee, B, Hjøllund, KR, He, Y, et al. Next generation GLP-1/GIP/glucagon triple agonists normalize body weight in obese mice. Mol Metab. (2022) 63:101533. doi: 10.1016/j.molmet.2022.101533
39. Ametov, AS, and Gusenbekova, DG. DPP-4 inhibitors and fat metabolism in patients with type 2 diabetes In: RG Ahmed, editor. Diabetes and its complications (2018). InTech.
40. Curtis, JR, Westfall, AO, Allison, J, Bijlsma, JW, Freeman, A, George, V, et al. Population-based assessment of adverse events associated with long-term glucocorticoid use. Arthritis Rheum. (2006) 55:420–6. doi: 10.1002/art.21984
41. Fardet, L, and Fève, B. Systemic glucocorticoid therapy: a review of its metabolic and cardiovascular adverse events. Drugs. (2014) 74:1731–45. doi: 10.1007/s40265-014-0282-9
42. Pernicova, I, and Korbonits, M. Metformin—mode of action and clinical implications for diabetes and cancer. Nat Rev Endocrinol. (2014) 10:143–56. doi: 10.1038/nrendo.2013.256
43. Wilding, J. Thiazolidinediones, insulin resistance and obesity: finding a balance. Int J Clin Pract. (2006) 60:1272–80. doi: 10.1111/j.1742-1241.2006.01128.x
44. Ghosh, A, Dutta, K, Bhatt, SP, Gupta, R, Tyagi, K, Ansari, IA, et al. Dapagliflozin improves body fat patterning, and hepatic and pancreatic fat in patients with type 2 diabetes in North India. J Clin Endocrinol Metab. (2022) 107:e2267–75. doi: 10.1210/clinem/dgac138
45. Neeland, IJ, Ross, R, Després, JP, Matsuzawa, Y, Yamashita, S, Shai, I, et al. Visceral and ectopic fat, atherosclerosis, and cardiometabolic disease: a position statement. Lancet Diabetes Endocrinol. (2019) 7:715–25. doi: 10.1016/S2213-8587(19)30084-1
46. Lee, SH, Park, SY, and Choi, CS. Insulin resistance: from mechanisms to therapeutic strategies. Diabetes Metab J. (2022) 46:15–37. doi: 10.4093/dmj.2021.0280
47. Ahlqvist, E, Storm, P, Käräjämäki, A, Martinell, M, Dorkhan, M, Carlsson, A, et al. Novel subgroups of adult-onset diabetes and their association with outcomes: a data-driven cluster analysis of six variables. Lancet Diabetes Endocrinol. (2018) 6:361–9. doi: 10.1016/S2213-8587(18)30051-2
48. Koster, A, Stenholm, S, Alley, DE, Kim, LJ, Simonsick, EM, Kanaya, AM, et al. Body fat distribution and inflammation among obese older adults with and without metabolic syndrome. Obesity. (2010) 18:2354–61. doi: 10.1038/oby.2010.86
49. Amati, F, Pennant, M, Azuma, K, Dubé, JJ, Toledo, FGS, Rossi, AP, et al. Lower thigh subcutaneous and higher visceral abdominal adipose tissue content both contribute to insulin resistance. Obesity. (2012) 20:1115–7. doi: 10.1038/oby.2011.401
50. Van Pelt, RE, Jankowski, CM, Gozansky, WS, Schwartz, RS, and Kohrt, WM. Lower-body adiposity and metabolic protection in postmenopausal women. J Clin Endocrinol Metab. (2005) 90:4573–8. doi: 10.1210/jc.2004-1764
51. McLaughlin, T, Lamendola, C, Liu, A, and Abbasi, F. Preferential fat deposition in subcutaneous versus visceral depots is associated with insulin sensitivity. J Clin Endocrinol Metab. (2011) 96:E1756–60. doi: 10.1210/jc.2011-0615
52. Snijder, MB, Dekker, JM, Visser, M, Bouter, LM, Stehouwer, CDA, Yudkin, JS, et al. Trunk fat and leg fat have independent and opposite associations with fasting and postload glucose levels: the Hoorn study. Diabetes Care. (2004) 27:372–7. doi: 10.2337/diacare.27.2.372
53. Yu, F, He, B, Chen, L, Wang, F, Zhu, H, Dong, Y, et al. Intermuscular fat content in Young Chinese men with newly diagnosed type 2 diabetes: based on MR mDIXON-quant quantitative technique. Front Endocrinol. (2021) 12:536018. doi: 10.3389/fendo.2021.536018
54. Goodpaster, BH, Thaete, FL, and Kelley, DE. Thigh adipose tissue distribution is associated with insulin resistance in obesity and in type 2 diabetes mellitus. Am J Clin Nutr. (2000) 71:885–92. doi: 10.1093/ajcn/71.4.885
55. Fantin, F, Rossi, AP, Cazzadori, M, Comellato, G, Mazzali, G, Gozzoli, MP, et al. Central and peripheral fat and subclinical vascular damage in older women. Age Ageing. (2013) 42:359–65. doi: 10.1093/ageing/aft005
56. Sam, S. Differential effect of subcutaneous abdominal and visceral adipose tissue on cardiometabolic risk. Horm Mol Biol Clin Investig. (2018) 33. doi: 10.1515/hmbci-2018-0014
57. Terry, RB, Stefanick, ML, Haskell, WL, and Wood, PD. Contributions of regional adipose tissue depots to plasma lipoprotein concentrations in overweight men and women: possible protective effects of thigh fat. Metabolism. (1991) 40:733–40. doi: 10.1016/0026-0495(91)90093-C
58. Ferreira, I, Snijder, MB, Twisk, JWR, van Mechelen, W, Kemper, HCG, Seidell, JC, et al. Central fat mass versus peripheral fat and lean mass: opposite (adverse versus favorable) associations with arterial stiffness? The Amsterdam growth and health longitudinal study. J Clin Endocrinol Metab. (2004) 89:2632–9. doi: 10.1210/jc.2003-031619
59. Yusuf, S, Hawken, S, Ounpuu, S, Bautista, L, Franzosi, MG, Commerford, P, et al. Obesity and the risk of myocardial infarction in 27,000 participants from 52 countries: a case-control study. Lancet Lond Engl. (2005) 366:1640–9. doi: 10.1016/S0140-6736(05)67663-5
60. Canoy, D, Boekholdt, SM, Wareham, N, Luben, R, Welch, A, Bingham, S, et al. Body fat distribution and risk of coronary heart disease in men and women in the European prospective investigation into Cancer and nutrition in Norfolk cohort: a population-based prospective study. Circulation. (2007) 116:2933–43. doi: 10.1161/CIRCULATIONAHA.106.673756
61. Manolopoulos, KN, Karpe, F, and Frayn, KN. Gluteofemoral body fat as a determinant of metabolic health. Int J Obes. (2005) 34:949–59. doi: 10.1038/ijo.2009.286
62. Arner, P. Differences in lipolysis between human subcutaneous and omental adipose tissues. Ann Med. (1995) 27:435–8. doi: 10.3109/07853899709002451
63. Wahrenberg, H, Lönnqvist, F, and Arner, P. Mechanisms underlying regional differences in lipolysis in human adipose tissue. J Clin Invest. (1989) 84:458–67. doi: 10.1172/JCI114187
64. Arner, P, Hellström, L, Wahrenberg, H, and Brönnegård, M. Beta-adrenoceptor expression in human fat cells from different regions. J Clin Invest. (1990) 86:1595–600. doi: 10.1172/JCI114880
65. Frayn, KN. Adipose tissue as a buffer for daily lipid flux. Diabetologia. (2002) 45:1201–10. doi: 10.1007/s00125-002-0873-y
66. Van Pelt, RE, Evans, EM, Schechtman, KB, Ehsani, AA, and Kohrt, WM. Contributions of total and regional fat mass to risk for cardiovascular disease in older women. Am J Physiol Endocrinol Metab. (2002) 282:E1023–8. doi: 10.1152/ajpendo.00467.2001
67. Tran, TT, Yamamoto, Y, Gesta, S, and Kahn, CR. Beneficial effects of subcutaneous fat transplantation on metabolism. Cell Metab. (2008) 7:410–20. doi: 10.1016/j.cmet.2008.04.004
68. Feingold, KR. Introduction to lipids and lipoproteins In: KR Feingold, B Anawalt, MR Blackman, A Boyce, G Chrousos, and E Corpas, et al., editors. Endotext. South Dartmouth (MA): MDText.com, Inc. (2000)
69. Blüher, S, and Mantzoros, CS. Leptin in humans: lessons from translational research. Am J Clin Nutr. (2009) 89:991S–7S. doi: 10.3945/ajcn.2008.26788E
70. White, UA, and Tchoukalova, YD. Sex dimorphism and depot differences in adipose tissue function. Biochim Biophys Acta. (2014) 1842:377–92. doi: 10.1016/j.bbadis.2013.05.006
71. Chaparro, J, Reeds, DN, Wen, W, Xueping, E, Klein, S, Semenkovich, CF, et al. Alterations in thigh subcutaneous adipose tissue gene expression in protease inhibitor-based highly active antiretroviral therapy. Metabolism. (2005) 54:561–7. doi: 10.1016/j.metabol.2004.08.022
72. Minocci, A, Savia, G, Lucantoni, R, Berselli, ME, Tagliaferri, M, Calò, G, et al. Leptin plasma concentrations are dependent on body fat distribution in obese patients. Int J Obes Relat Metab Disord. (2000) 24:1139–44. doi: 10.1038/sj.ijo.0801385
73. Picó, C, Palou, M, Pomar, CA, Rodríguez, AM, Palou, A, Gigante, DP, et al. Leptin as a key regulator of the adipose organ. Rev Endocr Metab Disord. (2022) 23:13–30.
74. Borges, MC, Oliveira, IO, Freitas, DF, Horta, BL, Ong, KK, Gigante, DP, et al. Obesity-induced hypoadiponectinaemia: the opposite influences of central and peripheral fat compartments. Int J Epidemiol. (2017) 46:2044–55. doi: 10.1093/ije/dyx022
75. Gradidge, PJL, Jaff, NG, Norris, SA, Toman, M, and Crowther, NJ. The negative association of lower body fat mass with cardiometabolic disease risk factors is partially mediated by adiponectin. Endocr Connect. (2022) 11:e220156. doi: 10.1530/EC-22-0156
76. Buemann, B, Astrup, A, Pedersen, O, Black, E, Holst, C, Toubro, S, et al. Possible role of adiponectin and insulin sensitivity in mediating the favorable effects of lower body fat mass on blood lipids. J Clin Endocrinol Metab. (2006) 91:1698–704. doi: 10.1210/jc.2005-1062
77. Kamimura, D, Ishihara, K, and Hirano, T. IL-6 signal transduction and its physiological roles: the signal orchestration model. Rev Physiol Biochem Pharmacol. (2003) 149:1–38. doi: 10.1007/s10254-003-0012-2
78. Fain, JN, Madan, AK, Hiler, ML, Cheema, P, and Bahouth, SW. Comparison of the release of adipokines by adipose tissue, adipose tissue matrix, and adipocytes from visceral and subcutaneous abdominal adipose tissues of obese humans. Endocrinology. (2004) 145:2273–82. doi: 10.1210/en.2003-1336
79. Willett, WC, Dietz, WH, and Colditz, GA. Guidelines for healthy weight. N Engl J Med. (1999) 341:427–34. doi: 10.1056/NEJM199908053410607
Keywords: metabolic protection, prediabetes, type 2 diabetes, cardiovascular diseases, body fat distribution, thigh fat
Citation: Alser M, Naja K and Elrayess MA (2024) Mechanisms of body fat distribution and gluteal-femoral fat protection against metabolic disorders. Front. Nutr. 11:1368966. doi: 10.3389/fnut.2024.1368966
Edited by:
Rahele Ziaei, Isfahan University of Medical Sciences, IranReviewed by:
Lucas Massier, Karolinska Institutet (KI), SwedenPascal Gottmann, German Institute of Human Nutrition Potsdam-Rehbruecke (DIfE), Germany
Copyright © 2024 Alser, Naja and Elrayess. This is an open-access article distributed under the terms of the Creative Commons Attribution License (CC BY). The use, distribution or reproduction in other forums is permitted, provided the original author(s) and the copyright owner(s) are credited and that the original publication in this journal is cited, in accordance with accepted academic practice. No use, distribution or reproduction is permitted which does not comply with these terms.
*Correspondence: Mohamed A. Elrayess, bS5lbHJheWVzc0BxdS5lZHUucWE=