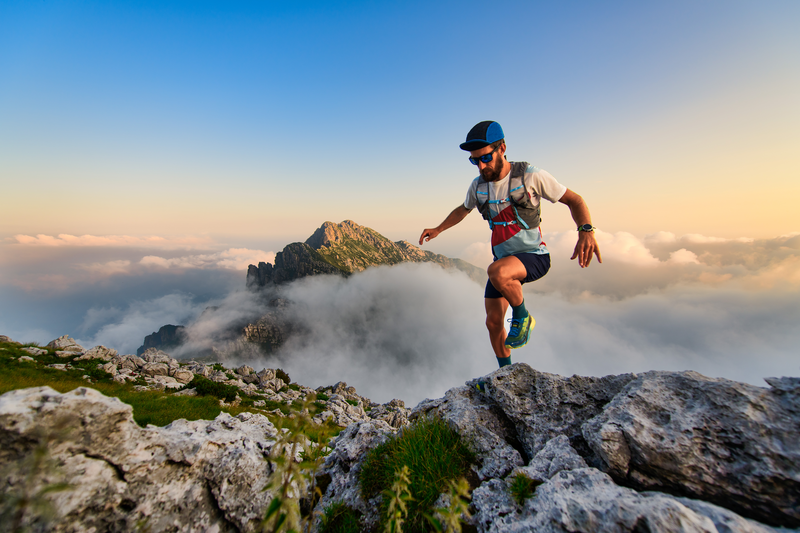
94% of researchers rate our articles as excellent or good
Learn more about the work of our research integrity team to safeguard the quality of each article we publish.
Find out more
ORIGINAL RESEARCH article
Front. Nutr. , 26 February 2024
Sec. Nutrition and Food Science Technology
Volume 11 - 2024 | https://doi.org/10.3389/fnut.2024.1330662
This article is part of the Research Topic Healthy Grains and Cereals: Sustainability, New Ingredients and Innovative Processing View all 7 articles
Introduction: The present research focuses on the chapatti making quality of high-yielding white maize hybrids compared to available low-yielding local yellow and white landraces in India.
Materials and methods: In this study, the top nine superior hybrids were selected for testing the physical properties of the maize kernels, proximate composition of flours and chapattis, physical parameters of chapatti, textural properties, sensory evaluation of chapattis and pasting properties of maize flour.
Results and discussion: The results revealed the superiority of white maize hybrids (WMH), viz., WHM 1, WHM 2, and WHM 8 over the local yellow and white landraces for most of the parameters studied. In sensory analysis, though, the yellow landrace was considered superior by the panellists in terms of colour but the white maize hybrids outperformed in overall sensory analysis and were more acceptable than the yellow and white maize landraces. These high yielding white maize hybrids with good consumer acceptance may cater for the needs of rural and tribal populations in India who prefer white maize as a staple food.
Maize (Zea mays L.) is one of the important cereal crops of the world being used as food, feed, fodder and raw material for a large number of industrial products (1, 2). Based on endosperm colour maize can be broadly categorized into two widely grown types, viz., yellow and white maize. White maize is the major staple food crop in parts of Africa, Central America, South America, Kenya, Malawi, Tanzania, and Zimbabwe. The majority of the maize grown in India is mostly the yellow type that is being diverted to poultry and animal feed. Maximum focus is given to yellow maize breeding programmes keeping in mind the feed industry resulting in little effort towards white maize improvement programmes which had led to less availability of high-yielding white maize hybrids. However, white maize landraces are extensively consumed by rural and tribal populations (3–5). In India, white maize is preferred by the farmers of Jammu and Kashmir, Gujarat, parts of Himachal Pradesh, Madhya Pradesh and Rajasthan. Thus, it is important to put concerted efforts into the genetic enhancement of white maize germplasm for developing high-yielding white maize hybrids and release these hybrids for commercial cultivation to cater to the needs of the people who prefer white maize as food.
White maize in India is used as a traditional cereal which requires cooking or heating before its consumption. So, it is important to focus on the quality characteristics, organoleptic traits and nutritional properties of developed products. Maize is a staple food in different parts of the country, particularly in South East-Asia, where it is consumed in the form of unleavened flatbread known as chapatti. These chapattis are consumed as a staple diet in several parts of India (6). Thus, it is important to study the chapatti-making qualities of maize flour prior to its release for commercial cultivation. Fewer efforts have been made for the evaluation of such characteristics when hybrids are generally developed. However, due to traditional preferences, white maize cannot be replaced by any other crop or even by yellow maize in areas where it is devoted as a staple food. Hence, it is important to develop productive white hybrids with comparable or better organoleptic properties than the low-yielding local landraces for the betterment of the tribal and rural populations of the country. These efforts will, in turn, contribute to the improvement of the nutritional status of rural communities which are often resource-limited. With this perspective, the current study was conducted to assess the nutritional properties of superior white maize hybrids and compare these hybrids with local yellow and white landraces to assess the acceptability of the white maize hybrids.
A total of 156 white maize hybrids were grown and tested at three locations, viz., Ludhiana, Hyderabad and Bihar in India. Mean grain yield data for three locations for these hybrids were pooled. Out of these 156 white maize hybrids, the top nine hybrids recorded >10% superiority for grain yield over the best check. The best check recorded yield of 6206.3 kg/ha whereas all the nine hybrids recorded grain yield >6,816 kg/ha grain yield. Two local landraces (white and yellow) and the check (Bio 605) were selected for evaluation of organoleptic and nutritional properties. One desi yellow landrace (Solan-L) and one local white landrace (Mali-1) were also tested for these characterstics. Solan-L and Mali-1 are landraces that are commonly used for preparing chapatti in tribal pockets. A total of twelve samples were subjected in the present study (Supplementary Table S1). Out of the 12, nine of the white hybrids were generated at ICAR-IIMR, Ludhiana, Solan-L was collected from Solan (Himachal Pradesh), Mali-1 was collected from Jammu and Kashmir and Bio 605 was a hybrid from Bio seed. All the samples were sun-dried, cleaned thoroughly for any foreign contamination, dust, diseased, infested or irregular seeds analyzed for physical properties and milled to prepare maize flour (<200 μ) using the laboratory mill (Perten Instruments, Hagersten, Sweden), sieved and stored properly for further analysis.
The maize grains were analyzed for physical quality, viz., thousand kernel weight, hectoliter weight and bulk density.
The TKW was recorded by weighing a hundred grains on a micro-weighing electronic analytical balance of sartorius© and multiplied by 10. The weight of 1,000 kernels was recorded in grams (gms) as per Kaur et al. (6).
The weight of grains filled in a measuring cylinder of 1.0-liter capacity was recorded. The final value was noted in kilograms/ hectoliters (kg/hL) (7).
The bulk density was calculated by taking 50 gms of grains in a 250 mL measuring cylinder (8). This was followed by tapping the cylinder gently ten times and the volume was noted and expressed as g/cm3.
The moisture content in maize flour and chapatti was determined using the standard air oven method. Weighed flour of finely ground grains and chapatti powder was subjected to the hot air oven for 3 to 4 hours at 1300 ± 10°C. The moisture content was calculated using the following formula (9):
The flour and chapatti powder samples were taken in pre-weighed crucibles followed by charring on a hot plate. The charred samples were placed in a muffle furnace at 550°C for 6 h and were then placed in the desiccators. The weight of the final crucible is noted as the total ash content (10).
The protein content of flour and chapatti samples was determined using the micro-Kjeldahl method (11). Nitrogen and protein contents were calculated as per the following formula:
A general composite conversion factor of 6.25 was used to calculate the percent crude protein content.
The fat content of the flour and chapatti samples was analyzed by FOSS instrument-Soxtec 2045 (Sweden). Crude fat (%) was calculated from the increase in the weight of the beakers (9):
The fiber estimation was performed using the fibertec (Foss instrument, Sweden) apparatus (12). The fiber content was calculated as per the following formula:
Where,
W1 = Weight of empty capsule.
W2 = Weight of sample.
W3 = Capsule weight + extracted and dried sample weight.
W4 = Weight of empty crucible.
W5 = Weight of crucible.
S = Capsule solubility (1.001849).
A = Capsule ash.
The TCC was calculated by using the following formula:
In the case of chapattis, moisture content was not taken into consideration while calculating TCC as per Sharma (13).
The maize kernels were subjected to the milling of grains and passed through a sieve to obtain fine flour. Chapatti making process involved the flour being kneaded with varying proportions of warm water till smooth, cohesive, non-sticky dough was obtained and percent water absorption was noted for each hybrid to prepare dough effectively. Dough after a while was rolled into chapattis and baked (6).
Lukewarm water was added to 100 gms of flour to form the smooth and non-sticky dough. The optimum amount of water added to prepare the dough was measured and noted (14).
The dough was divided into 40 gm of dough balls to prepare chapattis. The thickness and diameter of prepared chapattis were measured in three replicates. The diameter of the chapattis was measured using a scale and thickness was measured using a vernier caliper from various angles.
Chapattis were analyzed for sensory scores in terms of flavor, texture, colour, appearance and overall acceptability. Chapattis were presented on white papers labeled with random numbers to ten panelists. The panelists included five women and five men, of different states and ages. To test the flavor and textural feel of chapattis, all panelists were blindfolded to avoid any bias. Then the blindfolds were removed to rate for colour, appearance and overall acceptability. All the panelists were instructed to rinse their mouths with water after tasting every sample. A nine-point hedonic (1–9) scale (15) where 1 stands for “extremely disliking,” 9 stands for “extremely liking” and 5 stands for “neither like nor dislike,” was used.
Texture Profile Analysis (TPA) of each chapatti strip in triplicates was evaluated using the TA/XT2 Texture analyzer (Stable Micro Systems, Surrey, England). Texture Profile Analysis (TPA) involved measuring parameters such as adhesiveness, cohesiveness, springiness, hardness, chewiness, and gumminess. The samples were cut into consistent sizes and a cylindrical aluminum (P25) probe was used to apply pressure. The instrumental conditions were as follows: Pre-test speed: 10.0 mm/min, Post-test speed: 10.0 mm/min, Trigger: 15.0 g, and Load cell: 20.0 kg (16).
The pasting properties of maize flour samples were analyzed using a Rapid Visco Analyzer (RVA, Starch Master TM; Model: N17133; Newport Scientific Pvt. Ltd., Warriewood, Australia) following the standard AACC International Method 76-21.01. The samples were vigorously shaken up and down 10 times using the paddle inserted into the canister. The canister was placed into the instrument that had been pre-adjusted. The samples were weighed (3.00 gm) and added to the RVA canister along with 25 mL of distilled water. These samples were then heated from 50°C to 95°C at a rate of 12°C/min, held at 95°C for 5 min, and then cooled to 50°C at a rate of 12°C/min. After removing the canister from the instrument, the samples were disposed. The pasting properties including peak viscosity, trough viscosity, breakdown viscosity, final viscosity, setback viscosity, peak time, and pasting temperature were recorded (6).
The data was collected in triplicates and represented as the mean ± standard deviation. The data were subjected to analysis of variance (ANOVA) to determine statistically significant differences (p < 0.05) among the samples using the F-test. Duncan Multiple Range Test (DMRT) was done for the classification of the difference between any two treatment means as significant or non-significant. For comparison of proximate characters of flour and chapatti, a paired t-test was performed. Correlation analysis was performed using Kenall’s tau correlation matrix. Data analysis was done using R software (v4.1.2: R Core Team 2021) in a Completely Randomized Design (CRD).
The top nine hybrids out of the 156 hybrids recorded >10% superiority for grain yield/ha over the best check. The CD, CV, hybrids, with their mean grain yield values and % superiority of crosses, are presented in Supplementary Table S2.
The physical parameters such as TKW and bulk density are used in the assessment of the quality, yield and productivity of maize (Table 1).
The TKW varied between 223.39–293.57 gm with an average mean of 250.88 gm. WHM 2 (293.57 gm) showed significantly higher TKW than the local landraces. The maize hybrids with TKW of more than 290 gm are useful for industrial purposes as they are known to provide higher yields in the manufacturing of several products (6). TKW is positively correlated with kernel weight increment in achieving maximum grain filling rates (17). The bulk density varied between 0.722–0.782 gm/ml with an average of 0.752 gm/ml. Sandhu et al. (18) reported quite similar results with the bulk density range of 0.645–0.774 gm/ml. The hybrid WHM 5 (0.781 gm/ml) had a comparable bulk density to the yellow landrace, Solan-L (0.782 gm/ml). WHM 1 (0.764 gm/ml) and WHM 6 (0.758 gm/ml) had higher but non-significant bulk density than the white landrace, Mali-1 (0.756 gm/ml). The range of hectoliter weight in the study ranges between 73.1–79.25 kg/hL, the average being 75.70 kg/hL. For hectoliter weight, WHM 9 (79.25 kg/ hL) performed non-significantly better than yellow landrace (77.85 kg/hL), whereas, WHM 3 (76.75 kg/hL) and WHM 5 (76.90 kg/hL) had higher hectoliter weight than white landrace (76.25 kg/hL). High hectoliter weight is generally associated with a higher density of maize kernels and thus, a higher market has a value of the grains (19). Kara (20) reported that seed size also has a significant effect (p < 0.05) on hectoliter weight and the highest hectoliter weight (78.7 kg) were obtained from large seeds.
The white maize hybrids varied significantly in terms of proximate compositional parameters (Table 2). The moisture content varied between 4.03–9.50% with an average of 6.97%, which is quite lesser than the ideal average value of moisture content (10.23%) in maize (21). In terms of moisture content, yellow landrace (4.03%) recorded the least moisture content. However, six white maize hybrids had lower moisture contents as compared to the white landrace (7.20%). The flour with lower moisture content has a longer shelf life because it is less likely to spoil due to the growth of microorganisms or other biochemical reactions (22). In the study, the moisture content of all the white maize hybrids was less than 10% indicating, they are fit for long-term storage. Ash content specifically relates to the mineral composition found within a food substance and serves as an indicator of the overall mineral constituents present within food products (23). The ash content varied between 1.30–2.27% with an average of 1.67% Enyisi et al. (24) also reported a similar range of ash content in maize flour, 1.10–2.95%. WHM 7 (2.27%) and WHM 8 (2%), had significantly the highest ash content as compared to both yellow landrace (1.63%) and white local landrace (1.70%). Hybrids with high ash contents are positively correlated to the inorganic nutrient contents in foods. Thus, consumption of these hybrids will provide higher nutritive values (25).
The fat content ranged between 4.03–6.00% and the average being 4.64% which is more than the average fat content in normal maize, 4.57% (21). WHM 8 (6.00%) had significantly higher fat content than yellow landrace (4.77%). However, all white maize hybrids had higher (non-significant) fat content than white landrace (4.03%). Maize flour that contains a higher fat content can enhance the energy content of the flour and thereby, serve as a valuable source of essential fatty acids (26).
Protein is a vital component of food and people who follow higher-protein diets tend to lose more weight, body fat and preserve more lean muscle mass than those who follow lower-protein diets (27). The protein content varied between 8.53–9.87% with an average of 9.14% protein content. WHM 5 (9.87%) had significantly higher protein content than both the yellow landrace (9.33%) and white (9.23%) landraces. Siyuan et al. (28) also studied the nutritional traits of white maize hybrids and found the average protein content as 9.42%.
Fiber also affects digestive health, aids in weight management, management of cardiovascular health and the prevention of chronic diseases (29). Fiber content of samples ranged between 1.03–2.27% with an average fiber content of 1.72%. WHM 7 (2.27%) had a higher (non-significant) value than the yellow (1.97%) and white landrace (1.90%). Similarly, Qamar et al. (30) reported a range of 0.95–2.01% fiber content in white maize flour.
Foods rich in carbohydrates are essential for a well-balanced diet as they supply glucose to the body (31). The TCC ranged between, 73.47–78.27% with an average of 75.87%. Qamar et al. (30) reported similar results with the TCC of white maize flour in the range of 65.38–78.74%. No white maize hybrid performed significantly higher than yellow landrace (78.27%) in terms of TCC. Although, WHM 6 (77.53%) and WHM 9 (77.67%) had significantly higher TCC than the white landrace (75.94%).
Physical parameters like water absorption capacity of flour, while preparing chapattis, their thickness, diameter and baking time were performed (Table 3). The baking time of the chapattis ranged between 1.14–2.50 min with an average of 1.64 min. The baking time of chapattis made from whole wheat (16) also ranged between 2.00–3.00 min, slightly higher than the present study. The highest baking time was required by the check, Bio 605 (2.50 min) followed by yellow landrace (2.32 min), white land race (2.17 min), and WHM 6 (2.05 min). Whereas, WHM 2 had the lowest baking time (1.14 min). The higher overall acceptability of white maize hybrids was correlated to a lesser baking time. The rationale behind this is that stronger dough often requires longer baking times, which may result in tougher chapattis with lower acceptability (32).
The amount of water that dough can absorb is a key factor that affects its handling and sheeting properties (33). The average water absorption capacity ranged from, 115.33–158.00 mL/100 gm with an average of 134.03 mL/100 gm. However, water absorption capacity was much lower in the case of chapattis prepared from composite flours with values between 70 and 90 mL/100 gm as reported by Tangariya et al. (34). The highest water absorption by flour during dough formation was reported by, WHM 6 (158 mL/100 gm). Thus, WMH 6 exhibits enhanced water retention capabilities during the baking process, resulting in a desirable soft texture in the final product. This further, suggests that this particular genotype has absorbed a significant amount of water, which is advantageous for the baking of chapattis (35). The white landrace (115.33 mL/100gm) had the lowest water absorption followed by WHM 2 (116.33 mL/100gm). However, WHM 1 having the highest acceptability rate (7.21) showed moderate water absorption capacity (139 mL/ 100 gm). An appropriate amount of water helps the dough to come together into a smooth, workable mass during kneading. This makes it easy to shape the dough into chapattis but too much water will make the dough sticky and difficult to handle (36, 37).
The thickness of chapattis ranged between, 1.58–3.76 mm with an average of 2.80 mm. Yadav et al. (16) obtained an average thickness of chapattis between 2.5–4.5 mm, which is quite higher than the chapattis prepared in the present study from white maize hybrids samples. WHM 4 (3.76 mm) rolled into the thickest chapatti. Its overall acceptability was lower than average. WHM 8 (1.58 mm) had the thinnest chapatti without breaking and the overall acceptability of the hybrid was more than the average value. Moreover, the white maize hybrids with the highest overall acceptability, WHM 1 (3.70 mm) and WHM 2 (3.17 mm) had thicker chapattis. The results suggest that panelists do prefer slightly thick chapattis prepared from maize flour. The chapattis with a thicker consistency were also softer and comfortably chewable, while those with a thinner consistency tended to turn crispier and delicate. The diameter of these chapattis ranged from 10.20 cm – 12.73 cm with an average of 11.33 cm. The hybrid with the highest diameter of chapatti was WHM 8 (11.67 cm), without showing cracks. Its overall acceptability was also more than the average value. However, the highest diameter of chapattis was recorded by check, Bio 605 (12.73 cm) followed by yellow landrace (12.30 cm).
The white maize hybrids varied significantly in terms of proximate compositional parameters for chapattis (Table 4). The moisture content increases during the preparation of chapattis and it is directly proportional to the softness of chapattis (6). The moisture content for chapattis ranged between 29.90–44.47% with an average of 41.04%. WHM 8 (44.47%) followed by WHM 9 (44.23%) had significantly higher moisture content than yellow landrace (39.40%). Whereas, only WHM 8 (44.47%), had significantly higher moisture content than white landrace (40.00%).
The ash content in chapattis varied between 1.17–1.93% with an average of 1.51%. WHM 5 (1.93%) followed by WHM 2 (1.90%) and WHM 6 (1.73%), had significantly higher ash content in chapattis than that of yellow landrace (1.20%) and white landrace (1.43%).
The fat content in chapattis ranged between 3.33–4.43% and the average being 3.71%. WHM 7 (4.43%) followed by WHM 5 (4.13%), had significantly higher fat content in their chapattis than the yellow landrace (3.37%) and white landrace (3.70%). Research also suggests that the perception of fat in the mouth is intricately connected to the processing of taste signals in the brain (38, 39).
The range of protein in chapattis varied between the ranges of 8.37–10.15% with an average of 9.27% protein content. Chapattis prepared from WHM 5 (10.15) had significantly highest protein content followed by WHM 4 (9.53%), WHM 6 (9.50%) and WHM 1 (9.47%), than yellow landrace (8.87%). The fiber content in chapattis ranged between 1.07–1.93% with an average fiber content of 1.58%. WHM 3 (1.93%) had similar fiber content to yellow landrace (1.93%). WHM 3 (1.93%) had significantly highest fiber content followed by WHM 7 (1.80%) than the white landrace (1.53%).
The TCC in the chapattis ranged between, 82.72–84.63% with an average of 83.93%. Yadav et al. (13) also reported TCC in chapattis as high as 83.37% similar to the average TCC (83.93%) in the present study. WHM 9 (85.03%) recorded a non-significantly higher TCC than the yellow landrace (84.63%) and significantly higher than the white landrace (84.03%). The pictures of grains, dough and chapattis are presented in Figure 1.
The comparison of flour and chapatti was performed using a t-test (Supplementary Table S3). During the process of preparing chapattis, there is a significant increase in the moisture content which is desirable for their softness. Whereas, fat and fiber content significantly decreased during the chapatti-making process mainly due to the biochemical degradations of these components during the preparation of chapattis (13). It is well-reported that this process of roasting decreases the fat content in chapattis prepared from maize flour (40). Moreover, the fat content in maize flour can be affected by the breakdown of the bonds in the fat molecule, within the maize matrix due to heat, leading to the effective release and mobilization of oil reserves in the maize grain following the process of roasting (41). However, there were no significant differences in protein and ash content between the flour and chapatti in the present study. However, there was a significant increase in the TCC in chapattis compared to the flours of respective samples. In a similar study by Kumar et al. (42), it was observed that an increase in carbohydrate content after roasting could potentially be attributed to the reduction in fat, protein, ash and moisture levels that occur during the roasting process.
A total of ten panelists evaluated the chapatti samples. These panelists were from different backgrounds. The testing of attributes such as flavor and mouth feel was performed by blindfolding the panelists to avoid any biases. The white maize hybrids performed significantly better than the landraces (yellow and white) and were considered superior in terms of the particular attributes. For the sensory attribute, the appearance ranged from 5.40–7.50 on a scale of 1 to 9 with an average of 6.59. Though WHM 1 (7.50) performed significantly better in terms of their appearance over the white landrace, none of the white hybrids showed significant superiority over the yellow landrace. Kaur et al. (43) also observed a similar range of appearance, 4.60–7.60. Whereas, colour ranged from 5.70–7.30 with an average of 6.44. In terms of colour of chapattis, no white maize hybrid performed better than yellow landrace (7.30). Though, WHM 7 (6.90) followed by, WHM 2 (6.70), WHM 1 (6.40) and WHM 4 (6.40), performed better than the white landrace (6.10) in terms of their colour but the difference was not significant. Tangariya et al. (34) obtained an average value for colour of 7.21 while performing sensory analysis of chapattis prepared from composite flours. Flavor values ranged between, 5.40–7.70 with an average of 6.10. Interestingly, WHM 1 (7.70), performed significantly better than the yellow (5.90) and white landrace (5.80) in terms of this attribute of flavor. The mouth feels attribute ranged between, 5.50–7.60 on a scale of 1 to 9 with an average of 6.15. WHM 1 (7.60) was significantly better as compared to the yellow (6.00) and white landrace (6.40) in terms of mouth feel. Though WHM 8 (6.90) was numerically superior over the yellow (6.0) and white landrace (6.4) the difference was non-significant.
The overall acceptability ranged between 5.00–7.21 with an average of 5.95. Considering the overall acceptability, WHM 1 (7.21) and WHM 8 (6.57) were significantly better than both the yellow (6.03) and white landrace (6.21). Kaur et al. (6) reported quite similar mean values of colour, taste/flavor and overall acceptability as 5.80, 5.90 and 5.70, respectively during the sensory analysis of maize flatbreads. Overall, WHM 1 performed significantly better as compared to the landraces. WHM 1 also had the highest acceptability in terms of chapatti as ranked by the panelists coupled with its highest grain yield. The yellow landrace was rated highest in terms of colour but did not outperform in terms of overall acceptability (Table 5). Thus, the blindfolding technique removed biases for other sensory parameters for hybrids and landraces.
The textural properties analyzed by the texture analyzer were correlated with the overall acceptability (Table 6). The hardness ranged between 5.15–13.41 N with a mean of 8.44 N. WHM 9 showed the lowest hardness of 5.15 N whereas, WHM 3 had the highest value of hardness, 13.91 N. WHM 3 with the highest hardness had lower acceptability and WHM 9 with the lowest hardness had a higher acceptability rate. WHM 1 with the highest acceptability rate had a moderate level of hardness and the local yellow and white landrace had lower hardness. This suggests that the consumers prefer chapattis of maize with a lower to moderate level of hardness. However, the hardness in the texture of chapattis is attributed to increased viscosity due to the absence of gluten in maize. However, maize chapattis are good for consumers having gluten intolerance or celiac disease (44). The springiness ranged between 0.48–0.74 cm/mm with a mean of 0.64 cm/mm. WHM 9 had the lowest springiness, 0.48 cm/mm. Yellow landrace had the highest springiness of 0.74 cm/mm followed by WHM 4 and WHM 7, both with similar values of 0.72 cm/mm. However, WHM 1 and WHM 2 with the highest overall acceptability have moderate values of springiness. The cohesiveness ranges between the ratio of 0.19–0.31 with the mean ratio of 0.26. The lowest cohesiveness was found in WHM 3 with a ratio of 0.19, whereas WHM 9 has the highest value of cohesiveness, 0.31. The overall acceptability of WHM 9 is more than the mean acceptability rate. However, WHM 1 and WHM 2 with the highest overall acceptability have lower value of cohesiveness.
WHM 4 had the lowest gumminess, 14.90 N, whereas, WHM 7 possessed the highest gumminess, 31.10 N. The gumminess values ranges between 14.90N–31.10N. The acceptability of both these white maize hybrids falls in the average category. The gumminess of white maize hybrids with the highest overall acceptability, WHM 1 and WHM 2 had a little lower value than the average gumminess. This indicates that consumers prefer a little lesser gumminess in chapattis of white maize hybrids. In the case of chapatti, gumminess is the extent of resistance offered by the chapatti during biting and chewing. It depends on the cohesiveness and hardness of the dough (45). WHM 1 had the lowest chewiness, 10.80 N and WHM 9 had the highest chewiness, 21.80 N. The chewiness varied between 10.80–21.80 N and the average being 14.78 N. Interestingly, WHM 1 with the lowest chewiness, possesses the highest acceptability by the panelists. This links the higher acceptability of white maize chapattis with a low chewiness for acceptance by consumers. El-Sohaimy et al. (46) while evaluating the sensory characteristics of flat bread supplemented with the flour of quinoa, reported that increasing the gumminess and chewiness in the flatbread causes a decrease in the organoleptic scores when compared to normal wheat bread.
WHM 6 and WHM 7 had the highest resilience, 0.11, whereas the lowest level of resilience was found in the white landrace (0.07) and the check, Bio 605 (0.04). WHM 2, WHM 3 and WHM 8 had the lowest resilience, 0.08. Although, there was no direct correlation between resilience and overall acceptability. However, the white maize hybrids with a high resilence level of resilience had moderate acceptability. The range of resilience varied between 0.04–0.11 with an average of 0.09. In the context of chapatti-making quality, resilience is an important textural attribute that influences the overall eating experience. This gives chapattis a pleasant and springy texture that is desirable to the consumers (47).
Correlation analysis was also performed between the characteristics of texture (hardness, springiness, cohesiveness, gumminess and resilience) and the overall acceptability. It was found that two characters, viz., hardness (−0.455) and chewiness (−0.443) had a significant but negative correlation with overall acceptability (Supplementary Table S4). Boukid (48) in a review on flatbreads stated that an increase in gumminess and chewiness in these flatbreads leads to detrimental effects on overall texture resulting in a lower acceptability rate by the consumers.
The viscosity of the paste was assessed to examine the alterations occurring in the starch throughout the extrusion process (49). The pasting properties of the flour samples are presented in Table 7 and the graph in Figure 2.
Figure 2. Pasting properties of maize flour [The lines of different colours in the graph represent different samples used in the study. Numbers 1 to 9 represent WHM 1 to WHM 9; 10, 11 and 12 represent yellow landrace, white landrace and check Bio 605, respectively; the X-axis represents the time taken in minutes; the Y-axis represents the range of viscosity (CP), temperature (°C) and speed (rpm), respectively].
Pasting property depends on the rigidity of starch granules which in turn affect the granule swelling potential (50). The peak viscosity ranged from 195–1,036 cP with an average of 629. 00 cP. WHM 2 (1036.00 cP) had significantly highest peak viscosity followed by WHM 9 (974.00 cP) than both yellow (297.00 cP) and white landrace (195.00 cP). Peak viscosity represents the water binding capacity of starch which often affects the quality of the final product. The high proportion of ungelatinized starch may lead to greater peak viscosity, whereas the lower peak viscosity might be due to more degradation during processing through depolymerization and molecular entanglement (51). Kaur et al. (6) also reported a range of peak viscosity ranging between 209 and 1,097 cP, which lies close to the estimated range of peak viscosity in the present study. The trough viscosity ranged from 180–731 cP with an average of 420.58 cP. WHM 2 (731.00 cP) showed significantly highest trough viscosity followed by WHM 9 (597.000 cP) than both yellow (268.00 cP) and white landrace (180.00 cP). The breakdown viscosity ranged from 15–389 cP with an average of 208.41 cP. WHM 1 (389.00 cP) had significantly highest breakdown viscosity followed by WHM 8 (397.00 cP) than both yellow (29.00 cP) and white landrace (15.00 cP). Breakdown value is related to response of starch to shear with constant heating, causing a rupture which results in a decrease in viscosity (52). The flours of hybrids with the lowest breakdown viscosities are expected to withstand high heat treatments and shear stress, and thus would be more suitable for incorporation into products required high-temperature treatment (53). In a study by Shafie et al. (54), whole grain rice varieties were studied for pasting properties and the range breakdown viscosity of 0.33–130.67 cP was obtained. Final viscosity is the viscosity of the paste after the complete cooking and cooling process or the viscosity in real use (55). The final viscosity ranged from 582–2057 cP with an average of 1210.08 cP. WHM 2 (2057.00 cP) had significantly highest final viscosity followed by WHM 8 (1,657 cP) than both yellow (715.00 cP) and white landrace (582.00 cP). The genotypes with highest final viscosity could make sure a consistent stability of products when used as a food ingredient for thickening and stabilizing roles (53). Sandhu et al. (18) studied the pasting properties of various types of maize starches and found an average final viscosity in their study of 1785 cP the setback visciosity ranged from 402-1,326 cP. WHM 8 (1149.00 cP) had significantly highest setback viscosity followed by WHM 2 (1326.00 cP), than both yellow (447.00 cP) and white landrace (402.00 cP). The lowest setback viscosity was observed in white maize check, Mali-1 (402.0 cP). However, WHM 5 showed lowest setback viscosity (564.00 cP) out of the total nine white maize hybrids. Setback viscosity is related to starch reordering and retrogradation. The low setback viscosity indicates the lower value of retrogradation. Therefore, the chapattis prepared from Mali-1 and WHM 5 remained fresh for a longer period of time (6). Aidoo et al. (53) reported that genotypes with highest setback value could be utilized as an ingredient in products stored under cold temperature and, also for making pasta, whereas flour samples with relatively lower setback values could also be utilized in making low viscous foods like complementary baby foods in cassava.
Similarly, Pinto et al. (56) studied several maize landraces in the regions of Brazil and found setback viscosity in the range of 689.00–1077.50 cP. The peak time ranged from 4.73–7.00 min with an average of 5.18 min. WHM 2 (5.33 min) and WHM 8 (5.27 min) had significantly higher peak times than yellow landrace (5.00 min). However, no white maize hybrid had a higher peak time than the white landrace (7.00 min). Sangeeta and Grewal (57) also reported an average peak time of 5.78 min while studying the pasting properties of maize varieties. The pasting temperatures ranged from 79°C – 88.8°C with an average of 83.76°C. No white maize hybrid had a significantly higher pasting temperature as compared to both yellow landrace (87.20°C) and white landrace (86.00°C). The high pasting temperatures of the flours is an indication of their resistance towards swelling. The values in the present study fall within the range of 68.70–99.9°C; the pasting temperature was estimated by Uarrota et al. (58) while studying the pasting properties of Brazilian maize. The pasting properties of a food pertain to the alterations that take place in the food when subjected to heat in the presence of water. These modifications impact the texture, digestibility, and ultimate application of the food product (59). The germplasm with high values of peak viscosity, breakdown and setback is efficient for the preparation of pasta (60). There is a wide variability of pasting properties in the white maize lines selected for food technology analysis. This suggests the possibility of choosing the appropriate genotype to develop several food products. Other than chapatti, pasting properties are also important in many other food products. Flour with high peak viscosity and low final viscosity has been reported to be more resistant to retrogradation which gives a more desirable texture to some pastas (61) and other specialty products such as tortillas (62). Liu et al. (63) reported that most of the starch pasting, and thermal parameters were positively associated with diameter, spread ratio, and sensory scores, whereas negatively associated with hardness and thickness of biscuit prepared from wheat flour.
The evaluation of white maize hybrids for several food characteristics revealed promising results. The study highlights the importance of developing high-yielding white maize hybrids to cater to the preferences of rural and tribal populations who rely on white maize as their staple food. The superior white maize hybrids exhibited higher TKW, which is associated with higher yields. The bulk density and hectoliter weight of the hybrids showed variations, suggesting variability in grain density and porosity. The nutritional analysis of the white maize hybrids revealed their potential as a nutritious food source. The chapatti-making qualities of the white maize hybrids were evaluated and some hybrids showed superior characteristics. WHM 1 performed best in terms of proximate compositional parameters. These findings suggest the potential of the white maize hybrids for use in chapatti production, which is a staple food in several parts of India. Sensory analysis concluded that three white maize hybrids, viz., WHM 1, WHM 2, and WHM 8 performed significantly better than yellow landrace, local white landraces and the check hybrid. The highest-yielding white maize hybrid, WMH 1 with better nutrition status and higher acceptability can potentially replace existing low-yielding white maize landraces. Future research and breeding efforts should focus on developing white maize hybrids with enhanced organoleptic traits to meet the diverse needs and preferences of consumers.
The original contributions presented in the study are included in the article/Supplementary material, further inquiries can be directed to the corresponding authors.
AA: Formal analysis, Investigation, Methodology, Writing – original draft. AD: Conceptualization, Writing – review & editing, Software, Supervision. RK: Resources, Writing – review & editing. SS: Resources, Writing – review & editing. NK: Methodology, Writing – review & editing. SD: Methodology, Writing – review & editing. YK: Writing – review & editing. DS: Resources, Writing – review & editing. SR: Conceptualization, Resources, Supervision, Writing – review & editing.
The author(s) declare financial support was received for the research, authorship, and/or publication of this article. This work was supported by ICAR-Indian Institute of Maize Research (ICAR-IIMR), PAU Campus, Ludhiana, Punjab, India.
I would like to express my profound gratitude to Ms. Kavita Thakur and Ms. Manisha Bhandari, Department of Food Science and Technology, Punjab Agricultural University, Ludhiana, Punjab, India for their kind help in my research.
The authors declare that the research was conducted in the absence of any commercial or financial relationships that could be construed as a potential conflict of interest.
All claims expressed in this article are solely those of the authors and do not necessarily represent those of their affiliated organizations, or those of the publisher, the editors and the reviewers. Any product that may be evaluated in this article, or claim that may be made by its manufacturer, is not guaranteed or endorsed by the publisher.
The Supplementary material for this article can be found online at: https://www.frontiersin.org/articles/10.3389/fnut.2024.1330662/full#supplementary-material
1. Das, AK, Choudhary, M, Kumar, P, Karjagi, CG, Kr, Y, Kumar, R, et al. Heterosis in genomic era: advances in the molecular understanding and techniques for rapid exploitation. Crit Rev Plant Sci. (2021) 40:218–42. doi: 10.1080/07352689.2021.1923185
2. Lone, AA, Dar, ZA, Gull, A, Gazal, A, Naseer, S, Khan, MH, et al. Breeding maize for food and nutritional security In: AK Goyal, editor. Cereal Grains. London: IntechOpen (2021). 39–54.
3. Kumar, S, Das, AK, Naliath, R, Kumar, R, Karjagi, CG, Sekhar, JC, et al. Potential use of random and linked SSR markers in establishing the true heterotic pattern in maize (Zea mays). Crop Pasture Sci. (2022) 73:1345–53. doi: 10.1071/CP21376
4. Malvar, RA, Revilla, P, Moreno-González, J, Butrón, A, Sotelo, J, and Ordás, A. White maize: genetics of quality and agronomic performance. Crop Sci. (2008) 48:1373–81. doi: 10.2135/cropsci2007.10.0583
5. Mukri, G, Kumar, R, Rajendran, A, Kumar, B, Hooda, KS, Karjagi, CG, et al. Strategic selection of white maize inbred lines for tropical adaptation and their utilization in developing stable, medium to long duration maize hybrids. Maydica. (2018) 63:8. Available at: https://journals-crea.4science.it/index.php/maydica/article/view/1707
6. Kaur, N, Kumar, R, Singh, A, Shobha, D, Das, AK, Chaudhary, D, et al. Improvement in nutritional quality of traditional unleavened flat bread using quality protein maize. Front Nutr. (2022) 9:963368. doi: 10.3389/fnut.2022.963368
7. Barary, M, Kordi, S, Rafie, M, and Mehrabi, AA. Effect of harvesting time on grain yield, yield components, and some qualitative properties of four maize Hybirds. Int J Agric Food Res. (2015) 3:1–7. doi: 10.24102/ijafr.v3i4.354
8. Barnwal, P, Kadam, DM, and Singh, KK. Influence of moisture content on physical properties of maize. Int Agrophys. (2012) 26:331–4. doi: 10.2478/v10247-012-0046-2
9. AOAC (2005). Official methods of analysis. 18th ed. Arlington, V.A Association of Official Analytical Chemist PP. 806–842.
10. Van Wychen, S., and Laurens, L. M. (2023). Determination of total solids and ash in algal biomass: Laboratory analytical procedure (LAP) (No. NREL/TP-2700-87520). National Renewable Energy Laboratory (NREL), Golden, CO (United States).
11. Lynch, JM, and Barbano, DM. Kjeldahl nitrogen analysis as a reference method for protein determination in dairy products. J AOAC Int. (1999) 82:1389–98. doi: 10.1093/jaoac/82.6.1389
12. Fahey, GC, Novotny, L, Layton, B, and Mertens, DR. Critical factors in determining Fiber content of feeds and foods and their ingredients. J AOAC Int. (2019) 102:52–62. doi: 10.5740/jaoacint.18-0067
13. Sharma, P (2006) Nutritive quality evaluation of different products prepared from composite flours of maize and linseed. [Dissertation]. [Himachal Pradesh, India] CSKHPKV, Palmpur.
14. Gujral, HS, and Gaur, S. Effects of barley flour, wet gluten and liquid shortening on the texture and storage characteristics of chapatti. J Texture Stud. (2002) 33:461–9. doi: 10.1111/j.1745-4603.2002.tb01360.x
15. Larmond, E. Methods for sensory evaluation of food. Ottawa: Canada Department of Agriculture (1970). 1284 p.
16. Yadav, DN, Patki, PE, Mahesh, C, Sharma, GK, and Bawa, AS. Optimisation of baking parameters of chapati with respect to vitamin B1 and B2 retention and quality. Int J Food Sci Technol. (2008) 43:1474–83. doi: 10.1111/j.1365-2621.2008.01712.x
17. Wei, S, Wang, X, Li, G, Qin, Y, Jiang, D, and Dong, S. Plant density and nitrogen supply affect the grain-filling parameters of maize kernels located in different ear positions. Front Plant Sci. (2019) 10:180. doi: 10.3389/fpls.2019.00180
18. Sandhu, KS, Singh, N, and Malhi, NS. Some properties of corn grains and their flours I: physicochemical, functional and chapati-making properties of flours. Food Chem. (2007) 101:938–46. doi: 10.1016/j.foodchem.2006.02.040
19. Guelpa, A, du Plessis, A, and Manley, M. A high-throughput X-ray micro-computed tomography (μCT) approach for measuring single kernel maize (Zea mays L.) volumes and densities. J Cereal Sci. (2016) 69:321–8. doi: 10.1016/j.jcs.2016.04.009
20. Kara, B. Effect of seed size and shape on grain yield and some ear characteristics of maize. Res Crops. (2011) 12:680–5.
21. Rouf Shah, T, Prasad, K, and Kumar, P. Maize- A potential source of human nutrition and health: a review. Cogent Food Agric. (2016) 2:1166995. doi: 10.1080/23311932.2016.1166995
22. Kumari, A, Sharma, S, Sharma, N, Chunduri, V, Kapoor, P, Kaur, SG, et al. Influence of biofortified colored wheats (purple, blue, black) on physicochemical, antioxidant and sensory characteristics of chapatti (Indian flatbread). Molecules. (2020) 25:5071. doi: 10.3390/molecules25215071
23. Adigwe, NE, Kiin-Kabari, DB, and Emelike, NJT. Nutritional quality and in vitro protein digestibility of complementary foods formulated from maize, cowpea and Orange-fleshed sweet potato flours: a preliminary study. Asian Food Sci J. (2023) 22:25–37. doi: 10.9734/afsj/2023/v22i2619
24. Enyisi, IS, Umoh, VJ, Whong, CMZ, Abdullahi, IO, and Alabi, O. Chemical and nutritional value of maize and maize products obtained from selected markets in Kaduna state, Nigeria. Afr J Food Sci Technol. (2014) 5:100–4. doi: 10.14303/ajfst.2014.029
25. Bourn, D, and Prescott, J. A comparison of the nutritional value, sensory qualities, and food safety of organically and conventionally produced foods. Crit Rev Food Sci Nutr. (2002) 42:1–34. doi: 10.1080/10408690290825439
26. Siyame, P, Kassim, N, and Makule, E. Effectiveness and suitability of oyster mushroom in improving the nutritional value of maize flour used in complementary foods. Int J Food Sci. (2021) 2021:1–8. doi: 10.1155/2021/8863776
27. Leidy, HJ, Clifton, PM, Astrup, A, Wycherley, TP, Westerterp-Plantenga, MS, Luscombe-Marsh, ND, et al. The role of protein in weight loss and maintenance. Am J Clin Nutr. (2015) 101:1320S–9S. doi: 10.3945/ajcn.114.084038
28. Siyuan, S, Tong, L, and Liu, R. Corn phytochemicals and their health benefits. Food Sci Human Wellness. (2018) 7:185–95. doi: 10.1016/j.fshw.2018.09.003
29. Anderson, JW, Baird, P, Davis, RH Jr, Ferreri, S, Knudtson, M, Koraym, A, et al. Health benefits of dietary fiber. Nutr Rev. (2009) 67:188–205. doi: 10.1111/j.1753-4887.2009.00189.x
30. Qamar, S, Aslam, M, Huyop, F, and Javed, MA. Comparative study for the determination of nutritional composition in commercial and noncommercial maize flours. Pak J Bot. (2017) 49:519–23.
31. Clemente-Suárez, VJ, Mielgo-Ayuso, J, Martín-Rodríguez, A, Ramos-Campo, DJ, Redondo-Flórez, L, and Tornero-Aguilera, JF. The burden of carbohydrates in health and disease. Nutrients. (2022) 14:3809. doi: 10.3390/nu14183809
32. Amir, RM, Hussain, MN, Ameer, K, Ahmed, A, Ahmad, A, Nadeem, M, et al. Comprehensive assessment and evaluation of selected wheat varieties for their relationship to chapatti quality attributes. Food Sci Technol. (2020) 40:436–43. doi: 10.1590/fst.31619
33. Banerji, A, Ananthanarayan, L, and Lele, S. Rheological and nutritional studies of amaranth enriched wheat chapatti (Indian flat bread). J Food Process Preserv. (2018) 42:e13361. doi: 10.1111/jfpp.13361
34. Tangariya, P, Sahoo, A, Awasthi, P, and Pandey, A. Quality analysis of composite flour and its effectiveness for chapatti formulation. J Pharmacogn Phytochem. (2018) 7:1013–9.
35. Kundu, M, Khatkar, BS, and Gulia, N. Assessment of chapatti quality of wheat varieties based on physicochemical, rheological and sensory traits. Food Chem. (2017) 226:95–101. doi: 10.1016/j.foodchem.2016.12.046
36. Serin, S, and Sayar, S. The effect of the replacement of fat with carbohydrate-based fat replacers on the dough properties and quality of the baked pogaca: a traditional high-fat bakery product. Food Sci Technol. (2016) 37:25–32. doi: 10.1590/1678-457X.05516
37. Thakur, S, Singh, N, and Kaur, A. Characteristics of normal and waxy corn: physicochemical, protein secondary structure, dough rheology and chapatti making properties. J Food Sci Technol. (2017) 54:3285–96. doi: 10.1007/s13197-017-2775-5
38. Jump, DB, and Clarke, SD. Regulation of gene expression by dietary fat. Annu Rev Nutr. (1999) 19:63–90. doi: 10.1146/annurev.nutr.19.1.63
39. Rolls, ET. Neural representation of fat texture in the mouth. In: JP Montmayeur and J le Coutre, editors. Fat detection: Taste, texture, and post Ingestive effects. Boca Raton (FL), USA: CRC Press/Taylor & Francis; (2010).
40. Hamilton, RM, and Thompson, BK. Chemical and nutrient content of corn (Zea mays) before and after being flame roasted. J Sci Food Agric. (1992) 58:425–30. doi: 10.1002/JSFA.2740580318
41. Oboh, G, Ademiluyi, AO, and Akindahunsi, AA. The effect of roasting on the nutritional and antioxidant properties of yellow and white maize varieties. Int J Food Sci Technol. (2010) 45:1236–42. doi: 10.1111/j.1365-2621.2010.02263.x
42. Kumar, Y, Sharanagat, VS, Singh, L, and Mani, S. Effect of germination and roasting on the proximate composition, total phenolics, and functional properties of black chickpea (Cicer arietinum). Legum Sci. (2020) 2:e20. doi: 10.1002/leg3.20
43. Kaur, RJ, Sharma, S, and Kaur, G. Processing and quality evaluation of tortilla and corn chips. Asian Reson. (2014) 3:197–202.
44. Bala, M, Kumar, A, Nanda, SK, and Gupta, RK. Effect of casein and hydrocolloid on maize dough and chapati properties. Int J Curr Microbiol App Sci. (2018) 7:2058–70. doi: 10.20546/ijcmas.2018.704.236
45. Rozylo, R, and Laskowski, J. Predicting bread quality (bread loaf volume and crumb texture). Polish J Food Nutr Sci. (2011) 61:61–7. doi: 10.2478/v10222-011-0006-8
46. El-Sohaimy, SA, Shehata, MG, Mehany, T, and Zeitoun, MA. Nutritional, physicochemical, and sensorial evaluation of flat bread supplemented with quinoa flour. Int J Food Sci. (2019) 2019:e4686727. doi: 10.1155/2019/4686727
47. Correia, P, Guiné, RP, Fonseca, M, and Batista, L. Analysis of textural properties of gluten free breads. J Hyg Eng Des. (2021) 34:102–8.
48. Boukid, F. Flatbread-A canvas for innovation: A review. Appl Food Res. (2022) 2:100071. doi: 10.1016/j.afres.2022.100071
49. de Souza, VF, Ortiz, JAR, do Nascimento, EDG, and Ascheri, JLR. Pasting properties of expanded extrudate and pellets from corn flour and rice flour. Braz J Food Technol. (2011) 14:106–14. doi: 10.4260/BJFT2011140200014
50. Sandhya Rani, MR, and Bhattacharya, KR. Rheology of rice-flour pastes: effect of variety, concentration, and temperature and time of cooking. J Texture Stud. (1989) 20:127–37. doi: 10.1111/j.1745-4603.1989.tb00427.x
51. Hagenimana, A, Ding, X, and Fang, T. Evaluation of rice flour modified by extrusion cooking. J Cereal Sci. (2006) 43:38–46. doi: 10.1016/j.jcs.2005.09.003
52. Mishra, S, and Rai, T. Morphology and functional properties of corn, potato and tapioca starches. Food Hydrocoll. (2006) 20:557–66. doi: 10.1016/j.foodhyd.2005.01.001
53. Aidoo, R, Oduro, IN, Agbenorhevi, JK, Ellis, WO, and Pepra-Ameyaw, NB. Physicochemical and pasting properties of flour and starch from two new cassava accessions. Int J Food Prop. (2022) 25:561–9. doi: 10.1080/10942912.2022.2052087
54. Shafie, B, Cheng, SC, Lee, HH, and Yiu, PH. Characterization and classification of whole-grain rice based on rapid visco analyzer (RVA) pasting profile. Int Food Res J. (2016) 23: 2138–43.
55. Oyeyinka, SA, Adeloye, AA, Olaomo, OO, and Kayitesi, E. Effect of fermentation time on physicochemical properties of starch extracted from cassava root. Food Biosci. (2020) 33:1–8. doi: 10.1016/j.fbio.2019.100485
56. Pinto, ATB, Pereira, J, Oliveira, TRD, Prestes, RA, Mattielo, RR, and Demiate, IM. Characterization of corn landraces planted grown in the Campos Gerais region (Paraná, Brazil) for industrial utilization. Braz Arch Biol Technol. (2009) 52:17–28. doi: 10.1590/S1516-89132009000700003
57. Sangeeta,, and Grewal, RB. Pasting properties of maize flour from variety HQPM-1 and HQPM-7. J Pharmacogn Phytochem. (2018) 7:223–5.
58. Uarrota, VG, Amante, ER, Demiate, IM, Vieira, F, Delgadillo, I, and Maraschin, M. Physicochemical, thermal, and pasting properties of flours and starches of eight Brazilian maize landraces (Zea mays L.). Food Hydrocoll. (2013) 30:614–24. doi: 10.1016/j.foodhyd.2012.08.005
59. Ocheme, OB, Adedeji, OE, Chinma, CE, Yakubu, CM, and Ajibo, UH. Proximate composition, functional, and pasting properties of wheat and groundnut protein concentrate flour blends. Food Sci Nutr. (2018) 6:1173–8. doi: 10.1002/fsn3.670
60. Alfieri, M, Bresciani, A, Zanoletti, M, Pagani, MA, Marti, A, and Redaelli, R. Physical, chemical and pasting features of maize Italian inbred lines. Eur Food Res Technol. (2020) 246:2205–14. doi: 10.1007/s00217-020-03565-1
61. Dennett, AL, Schofield, PR, Roake, JE, Howes, NK, and Chin, J. Starch swelling power and amylose content of triticale and Triticum timopheevii germplasm. J Cereal Sci. (2009) 49:393–7.
62. Guo, G, Jackson, DS, Graybosch, RA, and Parkhurst, AM. Wheat tortilla quality: Impact of amylose content adjustments using waxy wheat flour. Cereal Chemistry. (2003) 80:427–36.
Keywords: white maize, chapatti , proximate composition, pasting properties, textural properties, sensory evaluation
Citation: Arora A, Das AK, Kumar R, Sharma S, Kaur N, Dixit S, Kaur Y, Saxena DC and Rakshit S (2024) Development of high-yielding white maize hybrids with better chapatti-making quality compared to traditionally used local landraces. Front. Nutr. 11:1330662. doi: 10.3389/fnut.2024.1330662
Received: 31 October 2023; Accepted: 31 January 2024;
Published: 26 February 2024.
Edited by:
Elessandra R. Zavareze, Federal University of Pelotas, BrazilReviewed by:
Vanita Pandey, Indian Institute of Wheat and Barley Research (ICAR), IndiaCopyright © 2024 Arora, Das, Kumar, Sharma, Kaur, Dixit, Kaur, Saxena and Rakshit. This is an open-access article distributed under the terms of the Creative Commons Attribution License (CC BY). The use, distribution or reproduction in other forums is permitted, provided the original author(s) and the copyright owner(s) are credited and that the original publication in this journal is cited, in accordance with accepted academic practice. No use, distribution or reproduction is permitted which does not comply with these terms.
*Correspondence: Sujay Rakshit, cy5yYWtzaGl0QGljYXIuZ292Lmlu; Abhijit Kumar Das, ZGFzLm15c2VsZkBnbWFpbC5jb20=
Disclaimer: All claims expressed in this article are solely those of the authors and do not necessarily represent those of their affiliated organizations, or those of the publisher, the editors and the reviewers. Any product that may be evaluated in this article or claim that may be made by its manufacturer is not guaranteed or endorsed by the publisher.
Research integrity at Frontiers
Learn more about the work of our research integrity team to safeguard the quality of each article we publish.