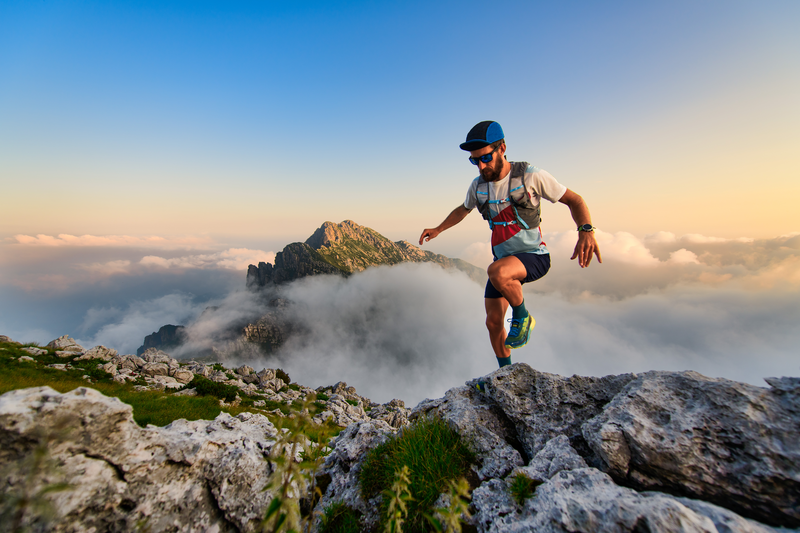
95% of researchers rate our articles as excellent or good
Learn more about the work of our research integrity team to safeguard the quality of each article we publish.
Find out more
ORIGINAL RESEARCH article
Front. Nutr. , 20 March 2023
Sec. Nutrition, Psychology and Brain Health
Volume 10 - 2023 | https://doi.org/10.3389/fnut.2023.996785
This article is part of the Research Topic Mechanisms and Pathways of Action for Nutritional Effects on Brain Health View all 13 articles
Background: Associations between body composition and execution function (EF) were currently studied in low altitude (LA) areas. However, the research on the correlation between waist circumference (WC) and EF among adolescents living at high altitude (HA) was limited.
Objective: We sought to explore the association between WC and EF in Chinese Tibetan adolescents aged 13–18 years in HA areas.
Methods: After excluding invalid data and extreme values, 1,228 participants (583 boys and 645 girls) were eventually included. The areas of Lhasa (average elevation of 3650 m), Nagqu (4500 m), Qamdo (3500 m), and Nyingchi (3100 m) in China were chosen as study sites. Participants completed tasks to measure inhibitory control, working memory, and cognitive flexibility. The predictive association between WC and EF was explored by One-way ANOVA, Pearson correlation, and linear regression analysis.
Results: After controlling for concomitant variables, the reaction time (RT) of responding to inhibitory control (difference incongruent and congruent), working memory (1-back, 2-back), and cognitive flexibility (heterogeneous, difference in heterogeneous and homogeneous) stimuli in subjects with WC ≥ 85th percentile was longer than that in those with WC of the 15th percentile or below [by 1.785 ms (95% CI: 0.078, 3.491), 208.734 ms (95% CI: 96.886, 320.582), 106.679 ms (95% CI: 16.485, 196.873), 82.307 ms (95% CI: 19.171, 145.442), and 58.397 ms (95% CI: 0.343,116.452), respectively], (P < 0.05).
Conclusion: After adjustment for concomitant variables, WC was significantly positively associated with the RT of inhibitory control, working memory, and cognitive flexibility among Chinese Tibetan adolescents in HA areas.
Executive function (EF) is an umbrella term for the related but distinct processes involved in the effortful control of goal-directed behavior (1), including three main factors, namely inhibitory control, working memory, and cognitive flexibility (2). Its purpose is to regulate and control the cognitive process (3). EF is known to be particularly sensitive to oxygen; studies have shown that the EF of people who live in high altitude (HA) areas with low temperatures and hypoxia may be impaired (4–6). Recently, the health question of humans in HA areas has become a topic under increasing discussion, and the impact of HA exposure on cognition has attracted the attention of scholars. Long-term exposure to HA areas may lead to cognitive deficits, such as in inhibitory control, working memory, and cognitive flexibility (7–9). Zhu et al. showed that the cognitive level of students in HA areas was relatively lower than that of students in low altitude (LA) areas, postulated to be mainly driven by chronic hypoxia in HA areas (10). Long-term exposure to HA areas has been shown to negatively affect individuals’ spatial working memory and significantly reduce their inhibitory control (11, 12).
As one of the factors influencing EF and underlying brain developmental processes in adolescents, body composition may be statistically correlated with EF (13–15). Soaring obesity rates and declining physical health have become among the most serious public health concerns worldwide (16, 17), notably in places as diverse as the United States (18, 19), Serbia (20), and China (21). Meanwhile, recent evidence suggests that obesity may have adverse health effects. Obesity has also been associated with cognitive performance across the life cycle, particularly with negative associations with EF (22, 23). As an effective indicator of abdominal obesity mass, waist circumference (WC) is used to define abdominal obesity (24), reflecting the accumulation of fat in the abdomen and valid predictor of future cardio-metabolic and chronic diseases (25). Even after controlling for body mass index (BMI), high WC appeared to be particularly detrimental to metabolic regulation, affecting type 2 diabetes risk (26). Most studies have focused on the relationship between BMI and EF (27, 28), but, there have been few studies on the correlations between WC and EF in HA areas.
At present, the correlation between WC and EF was not very uniform. Some studies have confirmed that high WC was negatively associated with EF (29, 30). In structural equation modeling in adolescents, WC through a higher metabolic risk factor cluster score (MetS-cluster score) and through lower high-density lipid cholesterol (HDLc) displayed a statistically significant negative relationship with reaction time (RT) of incongruent stimuli (29). Research has also found that the combined adverse effects of high WC on academic performance were observed in both boys and girls for grade point average (GPA) indicator (30). However, some other studies found that high WC was positively correlated EF (31). The results were inconsistent with other research regarding the relationship between WC and EF, which found that higher WC predicted higher cognitive ability among Indian children aged 9–10 years (31). The relationship between WC and EF remains unclear.
Most previous studies have focused on the relationship between BMI and EF. However, the research on the relationship between WC and EF remains limited. In addition, previous studies have mainly focused on adolescents in LA areas (32), while there have been few studies on the relationship between WC and EF among adolescents in HA areas. As one of the highest-altitude populations in the world, making it more likely to observe differences among Chinese Tibetan adolescents in HA areas. Hence, this paper sought to clarify the independent and joint correlations among WC and EF of Tibetan adolescents living in HA areas.
A stratified random cluster sampling method was adopted to identify test sites determined by surveys on the physical health of Tibetan students. Lhasa, Nagqu, Qamdo, and Nyingchi were chosen as test sites for this study (with average altitudes of 3650, 4500, 3500, and 3100 m, respectively). After performing the EF test, the valid data were the ones whose accuracy rate of each task reached over 80%. The specific inclusion criteria of the participants were people aged 13–18 years without color blindness or serious physical or mental illness. After excluding invalid data and extreme values, based on a gender ratio of approximately 1:1, data from 1,228 subjects (583 for boys and 645 for girls) were collected for this analysis in the Tibet Autonomous Region in China from August 2019 to December 2020 (Figure 1).
Waist circumference was measured according to the specific requirements of the physical health survey of students in China (33). In the test, the participants were asked to stand upright and breathe gently. The investigators faced the participants and put the nylon tape 1 centimeter (cm) above the navel on the horizontal plane of the waist. The line of sight was on the same level as the nylon tape, and the reading was in cm (accuracy: two decimal place). The test error could not exceed 0.01 cm.
Body mass was measured to the nearest 0.1 kg on an electronic scale (Tanita BWB-800S, Tanita Corporation, Tokyo, Japan). Stature was measured to the nearest 0.5 cm using a portable stadiometer (SECA 214, Seca Corporation, Hamburg, Germany). BMI was calculated according to the results of the recorded height and weight test using the standard formula. BMI = weight (kg)/height (m2) (33).
The EF task-cueing paradigm was that developed by Aiguo et al. (34), including inhibitory control test developed by Flanker (35), working memory test (36), and cognitive flexibility test (37). The RT for a correct test was recorded as the final result, and a shorter RT reflected a better performance. The RTs of the congruent and incongruent exercise were used to show inhibitory control. The 1-back and 2-back tests were used to indicate working memory. The difference in RTs between heterogeneous and homogeneous blocks was used to estimate cognitive flexibility. The EF tests were conducted on a computer using a program created by the E-prime 1.1 software system (Psychology Software Tools Inc., Pittsburgh, PA, USA).
The Flanker task involved two types of tests, congruent and incongruent. Participants gaze at the center of the computer screen for 500 ms, and then a series of five capital letters appear on the screen for 1000 ms. One of two different situations appear randomly: one situation is congruent (LLLLL or FFFFF) and the other incongruent (LLFLL or FFLFF). The center of the screen alternates with a + sign, and each stimulus appears at an interval of 2 s. Participants press either the “F” or “L” key with their left or right index finger. The test was divided into pre-test practice, stage 1, and stage 2. The pre-test practice was 12 rounds of the judgment response task, and stage 1 and stage 2 included 48 rounds each. The inhibitory control was calculated as the difference in response time between incongruent and congruent stimuli.
The 1-back task displayed five uppercase letters (A, S, P, G, T) in the center of the screen. The participants attempted to accurately remember the letters that appeared by pressing the “F” key if the letter appeared consistent with the previous letter, or the “J” key if it was inconsistent. The formal test was divided into two stages of 25 repetitions each. The stimulus interval for each capital letter was 3 s, and the time for the letter to appear on the screen was 2000 ms. In this study, the test age of 1-back was 10–18 years.
The task if the 2-back exercise was to indicate whether the letter in the center of the screen was consistent with the one letter in front, pressing the “F” key quickly if it was, or pressing the “J” key if not. Other test requirements and methods were the same as with the 1-back test.
This experimental test was divided into three parts. Part 1: At the beginning of the task, a black Arabic number (1–4 and 6–9, in random order) continuously appeared in the center of the computer screen. Participants judged the size of a “5” by pressing the D key if the digit was smaller than the 5 and pressing the F key if the digit was larger than the 5. The final result for each participant was their average RT across all trials. Part 2: A green number (1–4 and 6–9, in random order) appeared in the center of the screen at the beginning of the task. Participants pressed the J key if the digit was odd and pressed the K key if it was even. The final result for each participant was his average RT across all tasks. The heterogeneous conditions comprised Parts 1 and 2. Part 3 was then conducted: This test randomly interspersed the tasks used in Part 1 and Part 2. That is, if the number was black, the participant judged the size of the 5 (by pressing the D key if it was smaller and the F key if it was larger, in both cases using the left hand). If the number was green, participants judged whether it was an odd or even number (by pressing the J key if it was odd and the K key if it was even, in both cases using the right hand). A participant’s final result was their average RT across all trials (i.e., homogeneous RT). The whole test consisted of six stages, which were conducted in the order of A, B, C, C, B, A. A was Part 1 (i.e., the size judgment), and was performed 16 times in total. B was the parity judgment, Part 2, and was performed 16 times in total. C was the size/parity judgment, Part 3, which was performed 32 times in total. A practice round was not included in the test score; it was performed eight times before Part 1 and Part 2, and 16 times before Part 3.
Waist circumference was categorized according to percentile by the Lambda Mu Sigma (LMS): very low (WC < 15th percentile), normal (15th percentile ≤ WC < 85th percentile), high (WC ≥ 85th percentile) (38). Many studies have demonstrated that EF could be affected by obesity rate (22, 23), dietary intake (39), physical activity (40), and maximum oxygen uptake (VO2max) (41). Therefore, sociodemographic and dietary intake information, BMI, WC, moderate-to-vigorous physical activity (MVPA), and VO2max were used as covariates in this study. Based on previous studies and data in this study on the relationship between WC and EF in adolescents, directed acyclic graph (DAG) was used to identify possible covariates on exposure, and outcomes (Figure 2) (42). Three models were developed for this study: Model 1 was conducted after adjusting for demographic indicators (gender, age, BMI, and site location) by questionnaires. Based on Model 1, Model 2 controlled for MVPA, VO2max. Physical activity status was obtained by the following two questions: “In the past 7 days, how many times did you have MVPA on school days and weekends, respectively?” If students answered more than 0 times, they were further asked about the duration each time, “On average, how long does each activity last?” The VO2max was estimated by 20 m SRT and the details measurement of 20 m SRT was provided in our previous study (38). Based upon Model 2, Model 3 included breakfast, sugar-sweetened beverage intake as additional variables. The associations between WC and EF were evaluated using one-way ANOVA, Pearson correlation, and linear regression analysis. Data were analyzed using IBM SPSS 25.0 software (IBM Corp., Armonk, NY, USA); the test significance level was α = 0.05. Results were considered statistically significant if P < 0.05.
Figure 2. Directed acyclic graph of the associations between waist circumference and executive function.
Among the 1,228 Tibetan adolescents studied, there were 583 boys (47.48%) and 645 girls (52.52%), with an average age of 15.77 ± 1.678 years. The average WC of children and adolescents aged 13–18 years was 68.21 ± 7.13 cm. Grouped by WC, the proportions of subjects in the <15th percentile WC group, the ≥ 15th and <85th percentile WC group, and the ≥ 85th percentile WC group were 16.69, 70.85, and 12.46, respectively. The average values of congruent, incongruent, 1-back, 2-back, heterogeneous, and homogeneous were 774.94, 794.51, 965.67, 1078.05, 744.15, and 1125.69 ms, respectively (Table 1).
All measures of EF index [including inhibitory control RT, working memory RT (1-back, 2-back), and cognitive flexibility RT] differed significantly by WC percentile groups (F = 5.479, 11.165, 8.63, and 9.315, respectively, P < 0.05). The RTs of EF (inhibitory control, working memory, and cognitive flexibility) increased with WC percentile in different groups (Table 2).
Table 3 showed the analysis of the double variable pearson correlations of the various variables of the subjects. There were no mean differences by gender in performance of executive function variables (P > 0.05). In general, the higher the WC, the longer the congruent RT, incongruent RT, difference in incongruent and congruent RT, 1-back RT, 2-back RT, homogeneous RT, heterogeneous RT, and difference in heterogeneous and homogeneous RT (r = 0.095, 0.102, 0.085, 0.092, 0.122, 0.076, 0.148, and 0.130, respectively, P < 0.05). In other words, there was a positive correlation between WC and inhibitory control, working memory, and cognitive flexibility RT, with shorter RT indicating better EF.
The correlation between WC and executive function is presented in Table 4. After controlling for concomitant variables, the RTs of inhibitory control (difference incongruent and congruent), working memory (1-back, 2-back), and cognitive flexibility (heterogeneous, difference in heterogeneous and homogeneous) stimuli in subjects with WC ≥ 85th percentile was longer than that in those with WC of the 15th percentile or below [by 1.785 ms (95% CI: 0.078, 3.491), 208.734 ms (95% CI: 96.886, 320.582), 106.679 ms (95% CI: 16.485, 196.873), 82.307 ms (95% CI: 19.171, 145.442), and 58.397 ms (95% CI: 0.343,116.452), respectively], (P < 0.05). In the RTs of inhibitory control evalution, the subjects in the WC ≥ 85th percentile rate significantly different in the inhibitory control compared to those in the WC ≥ 15th percentile and <85th percentile (P < 0.05).
This study showed that WC was negatively associated with the inhibitory control, working memory, and cognitive flexibility among Chinese Tibetan adolescents in HA aeras. The RT of inhibitory control (difference incongruent and congruent), working memory (1-back, 2-back), and cognitive flexibility (heterogeneous, difference in heterogeneous and homogeneous) stimuli in subjects with WC ≥ 85th percentile was longer than that in those with WC of the 15th percentile or below, respectively. There were no mean differences by sex in performance EF variables, consistent with findings in prior research (43, 44).
In terms of inhibitory control, after controlling for concomitant variables, WC was negatively correlated with inhibitory control among Tibetan adolescents in HA areas in China. The associations between WC and inhibitory control in the study were generally consistent with those of previous studies. A study found that WC was negatively correlated with inhibitory control (P = 0.008) in Danish adolescents (23). At the same time, in the structural equation model, WC was indirectly positively correlated with incongruent RT through higher metabolic risk factor cluster (MetS-cluster) score and lower HDLc. The only statistically significant direct relationship between WC and inhibitory control was for the incongruent RT in the model including HDLc as a mediator (29). Longitudinal associations between inhibitory control and body composition throughout childhood found that better inhibitory control predicted lower subsequent obesity at each measured time point (45). Recent prospective studies (46) reported evidence suggesting that low inhibitory control in adolescents increases the risk of gaining more weight and WC. Interestingly, this paper found that high WC values the factors that predisposes the development of low inhibitory control.
In this study, after adjustment in concomitant variables, the RT of working memory (1-back, 2-back) in the highest percentiles of WC was slower than among those in the lowest percentiles of WC. WC was negatively correlated with working memory of children and adolescents. There have been numerous studies on the associations between WC and working memory that were consistent with the results of this study. Khan et al. explored the associations between abdominal adipose and hippocampal memory forms among pre-pubertal children (7–9-year-olds, n = 126) and found that total abdominal adipose tissue had a significant negative association with hippocampal-dependent relational memory behavioral accuracy (47). Hassevoort et al. found that central adiposity was negatively correlated with hippocampal-dependent relational memory among 7–10-year-old children (n = 40) who completed a task designed to assess relevant memory performance (P < 0.05) (48). After adjusting for multiple factors, we observed lower visuospatial function, executive performance, and language scores in the abdominal obesity group (WC ≥ 90 cm for men and ≥85 cm for women) compared with those in the non-abdominal obesity group, which was supported by the negative correlation between WC and visuospatial function (49). Some studies have linked obesity to an increased risk of memory problems in children (50). Gonzales found that larger WC was associated with decreased associated with diminished working-memory-related blood oxygen level-dependent (BOLD) response in the right superior frontal gyrus (β = −0.008, P = 0.001, 95% CI: −0.012 to −0.004) (51).
However, the results were not in accord with a previous southern Indian study of the relationship between WC and working memory performance (31). This cross-sectional study of 540 children reported that after adjusting for age, sex, and socioeconomic factors, higher WC predicted greater cognitive ability (i.e., long-term retrieval/storage, memory, reasoning, verbal abilities, attention, and concentration) in South India (31). The reasons for the discrepancy between this study and previous results may be that the subjects lived in Tibetan HA areas and were therefore exposed to chronic hypoxia (4–6). A study found that acute short-term exposure to HA areas could cause significant WM deficits in healthy children; this effect was particularly significant and more severe in children who lived at HA for a long time (7). The response accuracy of the HA group was significantly reduced in the linguistic and spatial working memory tasks compared with that of the LA group (4). Furthermore, obese children did not demonstrate significant retrieval-induced forgetting (RIF), whereas RIF was present in children (8–12-year-olds) without obesity and in adolescents (13–18-year-olds) with and without obesity (44). One longitudinal study showed that better working memory in grade three predicted lower subsequent body composition in grade four (45).
After adjustment for concomitant variables, WC was negatively associated with cognitive flexibility (heterogeneous, difference in heterogeneous and homogeneous) among subjects living at HA in this study. To support this theory, cognitive flexibility was impaired in obese adolescents (52, 53). Cross-sectional and longitudinal studies involving performance-based cognitive tasks have shown that overweight children and adolescents exhibit poorer cognitive flexibility than do their healthy-weight peers. These findings demonstrated that selective alterations in specific components of cognitive flexibility of overweight adolescents (52, 53). The relationship between WC and cognitive flexibility remains to be confirmed by longitudinal studies. Currently, there is a lack of relevant longitudinal studies on this topic, but Tomaso et al. conducted a longitudinal study on the relationship between cognitive flexibility and body composition (45). A longitudinal study showed that better SF in third grade predicted lower subsequent BMI in fourth grade, but, BMI did not predict subsequent cognitive flexibility performance at any point in time (45).
The results in this study were basically consistent with those of previous studies that also reported a negative correlation between high WC and EF of adolescents. A relatively consistent finding was that obesity was associated with poor EF across the life cycle, particularly in children and adolescents (54). One investigation found that obese (high visceral adipose tissue, H-VAT) children performed significantly lower on cognitive function tests than did normal-weight children (13). Another found that obesity (in terms of waist-hip ratio) in children and adolescents was negatively correlated with all areas of cognitive control (27). However, these results were reversed in the southern Indian study of the relationship between WC and cognitive performance noted above (31). Other studies have differed as well. One study found that Raven colored progressive matrices (CPM) scores were poorer among students with either the lowest or highest body fat percentile (BF%) than among other BF% groups (P < 0.05) (55).
Therefore, there is no consensus about the correlation between WC and EF. Improvement in EF levels has been associated with weight loss among overweight and obese adolescents (56), suggesting that EF could be improved and have a positive impact on weight loss (57). EF may have a protective effect on weight problems, especially in young adolescents when these abilities have had more time to develop and children begin to gain more independence (45). The findings suggested that the adverse association between obesity and EF may be attributed to visceral fat (VF), rather than fat stored elsewhere in the body (28). Both obesity and cognitive function could have strong associations with environmental influencing factors (socioeconomic status), though those associations can vary considerably in different directions depending on the setting (58). The results of multiple studies highlight the necessity of developing comprehensive adolescent health plans that promote both healthy body composition and brain function in the future.
Many previous studies have compared WC and executive function in adolescents; nevertheless, their datasets have generally been small and unrepresentative. The strengths of this study include its large sample size (N = 1228) for WC and use of multiple-aspect executive function tasks among adolescents living at HA in China. Most previous studies have focused on the associations between BMI and EF, and without regard to elevation; few systematic studies have examined the relationship between WC and EF in HA areas.
This study has some limitations to note. First, this study did not include participants at LA, and we did not compare the differences between the LA and HA. Second, this was a cross-sectional investigation, and therefore could not discern a causal relationship between WC and EF. Third, the investigation of influencing factors in this study was largely based on the participants’ recall ability and attitude, which inevitably led to certain deviations in the survey results. In the future, objective instruments could be used for accurate testing of physical activity parameters.
In general, after adjustment for concomitant variables, WC was significantly positively associated with the RT of inhibitory control, working memory, and cognitive flexibility tasks measuring executive function among Chinese Tibetan adolescents in HA areas. The reasons for these results should be further investigated by longitudinal studies. Furthermore, effective measures should be taken to reduce obesity and improve EF among adolescents in the HA areas of Chinese Tibet. Further investigation is needed to provide an evidence base for efforts to improve the physical health and EF of adolescents in these areas.
The original contributions presented in this study are included in the article/supplementary material, further inquiries can be directed to the corresponding author.
Written informed consent was obtained from the minor(s)’ legal guardian/next of kin for the publication of any potentially identifiable images or data included in this article.
YL and XY: conceptualization. YL and LG: methodology. XY: validation, supervision, and funding acquisition. YL: formal analysis, visualization, resources, writing—original draft preparation, and project administration. YL and LS: investigation. YG and PS: data curation. YL and FZ: writing—review and editing. All authors read and agreed to the published version of the manuscript.
This work was supported by the National Office for Philosophy and Social Science Fund of China (Award No. 21BTY121).
We thank all the persons who have helped us with this study.
The authors declare that the research was conducted in the absence of any commercial or financial relationships that could be construed as a potential conflict of interest.
All claims expressed in this article are solely those of the authors and do not necessarily represent those of their affiliated organizations, or those of the publisher, the editors and the reviewers. Any product that may be evaluated in this article, or claim that may be made by its manufacturer, is not guaranteed or endorsed by the publisher.
1. Allan J, McMinn D, Daly MA. Bidirectional relationship between executive function and health behavior: evidence, implications, and future directions. Front Neurosci. (2016) 23:386. doi: 10.3389/fnins.2016.00386
2. Funahashi S. Neuronal mechanisms of executive control by the prefrontal cortex. Neurosci Res. (2001) 39:147–65. doi: 10.1016/s0168-0102(00)00224-8
3. Chen A, Yin H, Jun Y, Yu Y. Effects of acute aerobic exercise of different intensity on executive function. Acta Psy Sin. (2011) 43:1055–62.
4. Ma H, Zhang D, Li X, Ma H, Wang N, Wang Y. Long-term exposure to high altitude attenuates verbal and spatial working memory: evidence from an event-related potential study. Brain Behav. (2019) 9:1256. doi: 10.1002/brb3.1256
5. Yan X, Zhang J, Gong Q, Weng X. Adaptive influence of long term high altitude residence on spatial working memory: an fMRI study. Brain Cogn. (2011) 77:53–9. doi: 10.1016/j.bandc.2011.06.002
6. Virues-ortega J, Bucks R, Kirkham FJ, Baldeweg T, Baya-Botti A, Hogan A. Bolivian children living at altitude project (BoCLA 06). Changing patterns of neuropsychological functioning in children living at high altitude above and below 4000 m: a report from the Bolivian children living at altitude (BoCLA) study. Dev Sci. (2011) 14:1185–93. doi: 10.1111/j.1467-7687.2011.01064.x
7. Rimoldi S, Rexhaj E, Duplain H, Urben S, Billieux J, Allemann Y, et al. Acute and chronic altitude-induced cognitive dysfunction in children and adolescents. J Pediatr. (2016) 169:238–43. doi: 10.1016/j.jpeds.2015.10.009
8. Virués-Ortega J, Garrido E, Javierre C, Kloezeman K. Human behaviour and development under high-altitude conditions. Dev Sci. (2006) 9:400–10. doi: 10.1111/j.1467-7687.2006.00505.x
9. Yan X. Cognitive impairments at high altitudes and adaptation. High Alt Med Biol. (2014) 15:141–5. doi: 10.1089/ham.2014.1009
10. Zhu X, Chen Z, Qi H. Relationship between cognitive level and oxygenated hemoglobin content of high school students in high altitude area of China. Chn High Altitude Med Biol. (2020) 41:165–71.
11. Ma H-L, Mo T, Zeng T-A, Wang Y. Long-term exposure to high altitude affects spatial working memory of migrants: evidence from temporal and frequency-domain analyses. Acta Phy Sin. (2020) 72:181–9. doi: 10.13294/j.aps.2020.0012
12. Ma H, Ting M, Yan W. Effects of long-term high altitude exposure on spatial working memory of Tibetan residents: evidence from time-frequency analysis. Chn High Altitude Med Biol. (2020) 41:88–93. doi: 10.13452/j.cnki.jqmc.2020.02.003
13. Raine L, Drollette E, Kao S, Westfall D, Chaddock-Heyman L, Kramer A, et al. The associations between adiposity, cognitive function, and achievement in children. Med Sci Sports Exerc. (2018) 50:1868–74. doi: 10.1249/MSS.0000000000001650
14. Laurent J, Watts R, Adise S, Allgaier N, Chaarani B, Garavan H, et al. Associations among body mass index. Cortical thickness, and executive function in children. JAMA Pediatr. (2020) 174:170–7. doi: 10.1001/jamapediatrics.2019.47
15. Li H, Chen A, Li W, Zhao Q, Wang J. Effect of body mass index on executive function in elementary school students. Chin J Sch Health. (2016) 37:1834–6. doi: 10.16835/j.cnki.1000-9817.2016.12.023
16. Spinelli A, Buoncristiano M, Kovacs V, Yngve A, Spiroski I, Obreja G, et al. Prevalence of severe obesity among primary school children in 21 european countries. Obes Facts. (2019) 12:244–58. doi: 10.1159/000500436
17. GBD 2015 Obesity Collaborators, Afshin A, Forouzanfar M, Reitsma M, Sur P, Estep K. Health effects of overweight and obesity in 195 countries over 25 years. N Engl J Med. (2017) 377:13–27. doi: 10.1056/NEJMoa1614362
18. Wang Y, Beydoun M, Min J, Xue H, Kaminsky L, Cheskin L. Has the prevalence of overweight, obesity and central obesity levelled off in the United States? Trends, patterns, disparities, and future projections for the obesity epidemic. Int J Epidemiol. (2020) 49:810–23. doi: 10.1093/ije/dyz273
19. Hales C, Carroll M, Fryar C, Ogden C. Prevalence of obesity among adults and youth: United States, 2015-2016. NCHS Data Brief. (2017) 288:1–8.
20. Djordjic V, Radisavljevic S, Milanovic I, Bozic P, Grbic M, Jorga J, et al. WHO European Childhood obesity surveillance initiative in serbia: a prevalence of overweight and obesity among 6-9-year-old school children. J Pediatr Endocrinol Metab. (2016) 29:1025–30. doi: 10.1515/jpem-2016-0138
21. Yang S, Guo B, Ao L, Yang C, Zhang L, Zhou J, et al. Obesity and activity patterns before and during COVID-19 lockdown among youths in China. Clin Obes. (2020) 10:12416. doi: 10.1111/cob.12416
22. Pearce A, Leonhardt C, Vaidya C. Executive and reward-related function in pediatric obesity: a meta-analysis. Child Obes. (2018) 14:265–79. doi: 10.1089/chi.2017.0351
23. Huang T, Tarp J, Domazet S, Thorsen A, Froberg K, Andersen L, et al. Associations of adiposity and aerobic fitness with executive function and math performance in Danish adolescents. J Pediatr. (2015) 167:810–5. doi: 10.1016/j.jpeds.2015.07.009
24. Ross R, Neeland IJ, Yamashita S, Shai I, Seidell J, Magni P, et al. Waist circumference as a vital sign in clinical practice: a consensus statement from the IAS and ICCR working group on visceral obesity. Nat Rev Endocrinol. (2020) 16:177–89. doi: 10.1038/s41574-019-0310-7
25. Setiono F, Guerra L, Leung C, Leak T. Sociodemographic characteristics are associated with prevalence of high-risk waist circumference and high-risk waist-to-height ratio in U.S. adolescents. BMC Pediatr. (2021) 21:215. doi: 10.1186/s12887-021-02685-1
26. Wang Y, Rimm E, Stampfer M, Willett W, Hu F. Comparison of abdominal adiposity and overall obesity in predicting risk of type 2 diabetes among men. Am J Clin Nutr. (2005) 81:555–63. doi: 10.1093/ajcn/81.3.555
27. Liang J, Matheson B, Kaye W, Boutelle K. Neurocognitive correlates of obesity and obesity-related behaviors in children and adolescents. Int J Obes. (2014) 38:494–506. doi: 10.1038/ijo.2013.142
28. Schwartz D, Leonard G, Perron M, Richer L, Syme C, Veillette S, et al. Visceral fat is associated with lower executive functioning in adolescents. Int J Obes. (2013) 37:1336–43. doi: 10.1038/ijo.2013.104
29. Bugge A, Möller S, Westfall D, Tarp J, Gejl A, Wedderkopp N, et al. Associations between waist circumference, metabolic risk and executive function in adolescents: a cross-sectional mediation analysis. PLoS One. (2018) 13:e0199281. doi: 10.1371/journal.pone.0199281
30. Esteban-Cornejo I, Tejero-González C, Castro-Piñero J, Conde-Caveda J, Cabanas-Sanchez V, Sallis J, et al. Independent and combined influence of neonatal and current body composition on academic performance in youth: the UP & DOWN Study. Pediatr Obes. (2015) 10:157–64. doi: 10.1111/ijpo.239
31. Veena S, Hegde B, Ramachandraiah S, Krishnaveni G, Fall C, Srinivasan K. Relationship between adiposity and cognitive performance in 9-10-year-old children in South India. Arch Dis Child. (2014) 99:126–34. doi: 10.1136/archdischild-2013-304478
32. Bi C. Relationship between cardiorespiratory endurance and executive function and exercise intervention of tibetan children and adolescents in high altitude areas. Shanghai: East China Normal University (2021).
33. Department of Physical Education, Health and Art Education, Ministry of Education, National Student Physique and Health Research Group,. National student physique and health survey manual. Beijing Higher Edu Pre. (2014) 2014:17–24.
34. Chen A, Jiang R, Ji X, Tao B, Zhu F, Yan J. Effects of 8-week moderate fancy rope skipping training on executive function in preadolescent deaf children: a school-based experimental study. J Sports Sci. (2015) 36:105–9. doi: 10.13598/j.issn1004-4590.2015.04.017
35. Wylie S, Ridderinkhof K, Eckerle M, Manning C. Inefficient response inhibition in individuals with mild cognitive impairment. Neuropsychologia. (2007) 45:1408–19. doi: 10.1016/j.neuropsychologia.2006.11.003
36. Smith E, Jonides J. Working memory: a view from neuroimaging. Cogn Psychol. (1997) 3:5–42. doi: 10.1006/cogp.1997.0658
37. Salthouse T, Atkinson T, Berish D. Executive functioning as a potential mediator of age-related cognitive decline in normal adults. J Exp Psychol Gen. (2003) 132:566–94. doi: 10.1037/0096-3445.132.4.566
38. Liu Y, Yin X, Zhang F, Li Y, Bi C, Sun Y, et al. Relationship between waist circumference and cardiorespiratory fitness in Chinese children and adolescents: results from a cross-sectional survey. J Exerc Sci Fit. (2022) 20:1–8. doi: 10.1016/j.jesf
39. Egbert A, Creber C, Loren D, Bohnert A. Executive function and dietary intake in youth: a systematic review of the literature. Appetite. (2019) 139:197–212. doi: 10.1016/j.appet.2019.04.013
40. Li L, Zhang J, Cao M, Hu W, Zhou T, Huang T. The effects of chronic physical activity interventions on executive functions in children aged 3-7 years: a meta-analysis. J Sci Med Sport. (2020) 23:949–54. doi: 10.1016/j.jsams.2020.03.007
41. Zhan Z, Ai J, Ren F, Li L, Chu C, Chang Y. Cardiorespiratory fitness, age, and multiple aspects of executive function among preadolescent children. Front Psychol. (2020) 11:1198. doi: 10.3389/fpsyg.2020.01198
42. Textor J, van der Zander B, Gilthorpe M, Liskiewicz M, Ellison G. Robust causal inference using directed acyclic graphs: the R package ‘dagitty’. Int J Epidemiol. (2016) 45:1887–94. doi: 10.1093/ije/dyw341
43. Li Y, Dai Q, Jackson J, Zhang J. Overweight is associated with decreased cognitive functioning among school-age children and adolescents. Obesity. (2008) 16:1809–15. doi: 10.1038/oby.2008.296
44. Davidson T, Ramirez E, Kwarteng E, Djan K, Faulkner L, Parker M, et al. Retrieval-induced forgetting in children and adolescents with and without obesity. Int J Obes. (2022) 46:851–8. doi: 10.1038/s41366-021-01036-5
45. Tomaso C, James T, Nelson J, Espy K, Nelson T. Longitudinal associations between executive control and body mass index across childhood. Pediatr Obes. (2022) 17:e12866. doi: 10.1111/ijpo.12866
46. Mayer M, Catalani F, Fraire J, Deltetto N, Martín L, Beneitez A, et al. Inhibitory control and obesity in adolescents: a prospective cohort study. Appetite. (2022) 171:105910. doi: 10.1016/j.appet.2022.105910
47. Khan N, Baym C, Monti J, Raine L, Drollette E, Scudder M, et al. Central adiposity is negatively associated with hippocampal-dependent relational memory among overweight and obese children. J Pediatr. (2015) 166: 302–8.e1. doi: 10.1016/j.jpeds.2014.10.008
48. Hassevoort K, Khazoum S, Walker J, Barnett S, Raine L, Hammond B, et al. Macular carotenoids, aerobic fitness, and central adiposity are associated differentially with hippocampal-dependent relational memory in preadolescent children. J Pediatr. (2017) 183:108–14.e1. doi: 10.1016/j.jpeds.2017.01.016
49. Fan X, Zhong Y, Zhang L, Li J, Xie F, Zhang Z. Abdominal obesity: an independent influencing factor of visuospatial and executive/language ability and the Serum Levels of Aβ40/Aβ42/Tau protein. Dis Mark. (2022) 2022:3622149. doi: 10.1155/2022/3622149
50. Cheke L, Bonnici H, Clayton N, Simons J. Obesity and insulin resistance are associated with reduced activity in core memory regions of the brain. Neuropsychologia. (2017) 96:137–49. doi: 10.1016/j.neuropsychologia
51. Gonzales M, Kaur S, Eagan D, Goudarzi K, Pasha E, Doan D, et al. Central adiposity and the functional magnetic resonance imaging response to cognitive challenge. Int J Obes. (2014) 38:1193–9. doi: 10.1038/ijo.2014.5
52. Verdejo-García A, Pérez-Expósito M, Schmidt-Río-Valle J, Fernández-Serrano M, Cruz F, Pérez-García M, et al. Selective alterations within executive functions in adolescents with excess weight. Obesity. (2010) 18:1572–8. doi: 10.1038/oby.2009.475
53. Delgado-Rico E, Río-Valle J, González-Jiménez E, Campoy C, Verdejo-García A. BMI predicts emotion-driven impulsivity and cognitive inflexibility in adolescents with excess weight. Obesity. (2012) 20:1604–10. doi: 10.1038/oby.2012.47
54. Smith E, Hay P, Campbell L, Trollor JN. A review of the association between obesity and cognitive function across the lifespan: implications for novel approaches to prevention and treatment. Obes Rev. (2011) 12:740–55. doi: 10.1111/j.1467-789X.2011
55. Haapala E, Lintu N, Väistö J, Robinson L, Viitasalo A, Lindi V, et al. Associations of physical performance and adiposity with cognition in children. Med Sci Sports Exerc. (2015) 47:2166–74. doi: 10.1249/MSS.0000000000000652
56. Holcke M, Marcus C, Gillberg C, Fernell E. Paediatric obesity: a neurodevelopmental perspective. Acta Paediatr. (2008) 97:819–21. doi: 10.1111/j.1651-2227.2008.00816.x
57. Staiano A, Abraham A, Calvert S. Competitive versus cooperative exergame play for African American adolescents’ executive function skills: short-term effects in a long-term training intervention. Dev Psychol. (2012) 48:337–42. doi: 10.1037/a0026938
Keywords: waist circumference, executive function, Tibetan, high altitude, adolescents
Citation: Liu Y, Zhang F, Gan L, Shi L, Yin X, Guo Y and Sun P (2023) Associations between waist circumference and executive function among Chinese Tibetan adolescents living at high altitude. Front. Nutr. 10:996785. doi: 10.3389/fnut.2023.996785
Received: 18 July 2022; Accepted: 06 March 2023;
Published: 20 March 2023.
Edited by:
Andrew Scholey, Monash University, AustraliaReviewed by:
Marcos Mayer, CESIM Foundation, ArgentinaCopyright © 2023 Liu, Zhang, Gan, Shi, Yin, Guo and Sun. This is an open-access article distributed under the terms of the Creative Commons Attribution License (CC BY). The use, distribution or reproduction in other forums is permitted, provided the original author(s) and the copyright owner(s) are credited and that the original publication in this journal is cited, in accordance with accepted academic practice. No use, distribution or reproduction is permitted which does not comply with these terms.
*Correspondence: Xiaojian Yin, eGp5aW4xOTY1QDE2My5jb20=
Disclaimer: All claims expressed in this article are solely those of the authors and do not necessarily represent those of their affiliated organizations, or those of the publisher, the editors and the reviewers. Any product that may be evaluated in this article or claim that may be made by its manufacturer is not guaranteed or endorsed by the publisher.
Research integrity at Frontiers
Learn more about the work of our research integrity team to safeguard the quality of each article we publish.