- Department of Cardiology, Lanzhou University Second Hospital, Lanzhou, Gansu, China
Purpose: Observational studies have increasingly recognized the influence of gut microbes on blood pressure modulation. Despite these findings, a direct causal link between gut flora and hypertension remains unestablished due to inherent confounders and the challenges of reverse causality in observational research. In this study, we sought to elucidate the causal relationship between specific gut flora and hypertension and its intermediary mediators.
Methods: We employed a two-sample Mendelian randomization (MR) and mediation MR analysis, analyzing 211 species of gut bacteria, with a focus on the interleukin family as potential mediators and hypertension as the primary outcome. The central methodological technique was inverse variance-weighted estimation, supplemented by various other estimators.
Results: Our findings revealed that two bacterial species positively correlated with hypertension risk, while five exhibited a negative association. Further validation was conducted using sensitivity analyses. Notably, our mediation MR results suggest interleukin-1 receptor type 2 (IL-1R2) as a mediator for the effect of the genus Clostridium innocuum group on hypertension, accounting for a mediation proportion of 14.07% [mediation effect: (b = 0.0007, 95%CI: 0.0002–0.0011); proportion mediation = 14.07% (4.26–23.40%)].
Conclusion: Our research confirms a genetic causal relationship between specific gut microbes and hypertension, emphasizing the potential mediating role of interleukin-1 receptor type 2 (IL-1R2) and offering insights for clinical hypertension interventions.
1 Introduction
Globally, hypertension is an increasing health concern, affecting over 1.5 billion individuals (1). This condition is intrinsically linked with CVD (cardiovascular disease), cerebrovascular diseases, and kidney diseases (2–4). Notably, a 2 mmHg reduction in blood pressure can lead to a 7% decrease in cardiovascular disease mortality and a 10% decline in stroke mortality (5). While the interplay of genetic and environmental influences contributes to the onset of hypertension (6–8), its precise pathogenesis remains elusive.
The human gastrointestinal system houses a vast array of microorganisms, of which the intestinal bacteria play an indispensable role in maintaining ecological balance (9). Not only is it pivotal for digesting foods and aiding nutrient absorption, supplying energy for daily metabolic processes, but it also performs a vital endocrine function by secreting substances that partake in various physiological processes (10). Recent research has highlighted a potential connection between hypertension and the gut microbiota (11, 12). For instance, Li et al. showed a reduced abundance of gut microbiota in hypertensive individuals compared to healthy ones (12). Additionally, after transplanting fecal matter from hypertensive patients into germ-free mice, an elevation in blood pressure was observed after 10 weeks (12). While animal models provide valuable insights, they cannot entirely replace clinical studies. Exploring the causal relationship between hypertension and gut flora in clinical studies poses challenges, leading to the paucity of such studies. Currently, the number of clinical studies investigating the relationship between gut microbes and hypertension is limited, and those available often feature small sample sizes. Several factors contribute to this complexity. The primary pathophysiological mechanisms might be masked by compensatory pathways, and long-term dietary habits have a pronounced effect on gut flora. This is evident in studies examining the relationship between hypertension and Clostridium difficile, where divergent findings are presented (13, 14). It is also possible that the observed intestinal ecological disturbances are merely symptoms accompanying hypertension. The existing links between hypertension and gut flora largely stem from observational studies with limited sample sizes, tainted by numerous confounding variables. The nature of these studies makes it difficult to exclude the possibility of reverse causation, thereby compromising their reliability in establishing causality. In essence, while a relationship between hypertension and enterobacteria is evident, the causal links remain ambiguous, demanding further research to elucidate the intricate pathophysiological mechanism.
Mendelian randomization (MR) is a research technique grounded in Mendel's second law that leverages the random assignment of alleles from parents to offspring during gamete formation (15, 16). This method uses genotypes, which determine intermediate phenotypes like exposure traits, to draw associations with disease outcomes (15, 16). Because of this natural randomness in allele assignment, MR inherently mitigates the effects of confounding environmental factors and precludes reverse causation (15, 16). As a result, MR offers a robust mechanism for deriving causal inferences from observational data. Therefore, in this study, we employed the MR approach to probe the causal relationship between hypertension and gut flora.
2 Methods and materials
2.1 Study design
In order to obtain reliable results, a two-sample MR should satisfy three essential assumptions: (1) Instrumental variables (IVs) must be significantly associated with gut microorganisms; (2) IVs are not associated with other factors that could confound the relationship between exposure and outcome variables; (3) IVs should influence outcomes solely via the gut microorganisms. As depicted in the flowchart (Figure 1), our study first employed a two-sample MR to establish the causal relationship between specific gut flora and interleukin (IL)-related factors in relation to hypertension. Following this, we utilized a mediated MR (two-step MR) to delve into the causal link between specific gut flora, as mediated by interleukin-related factors, and hypertension.
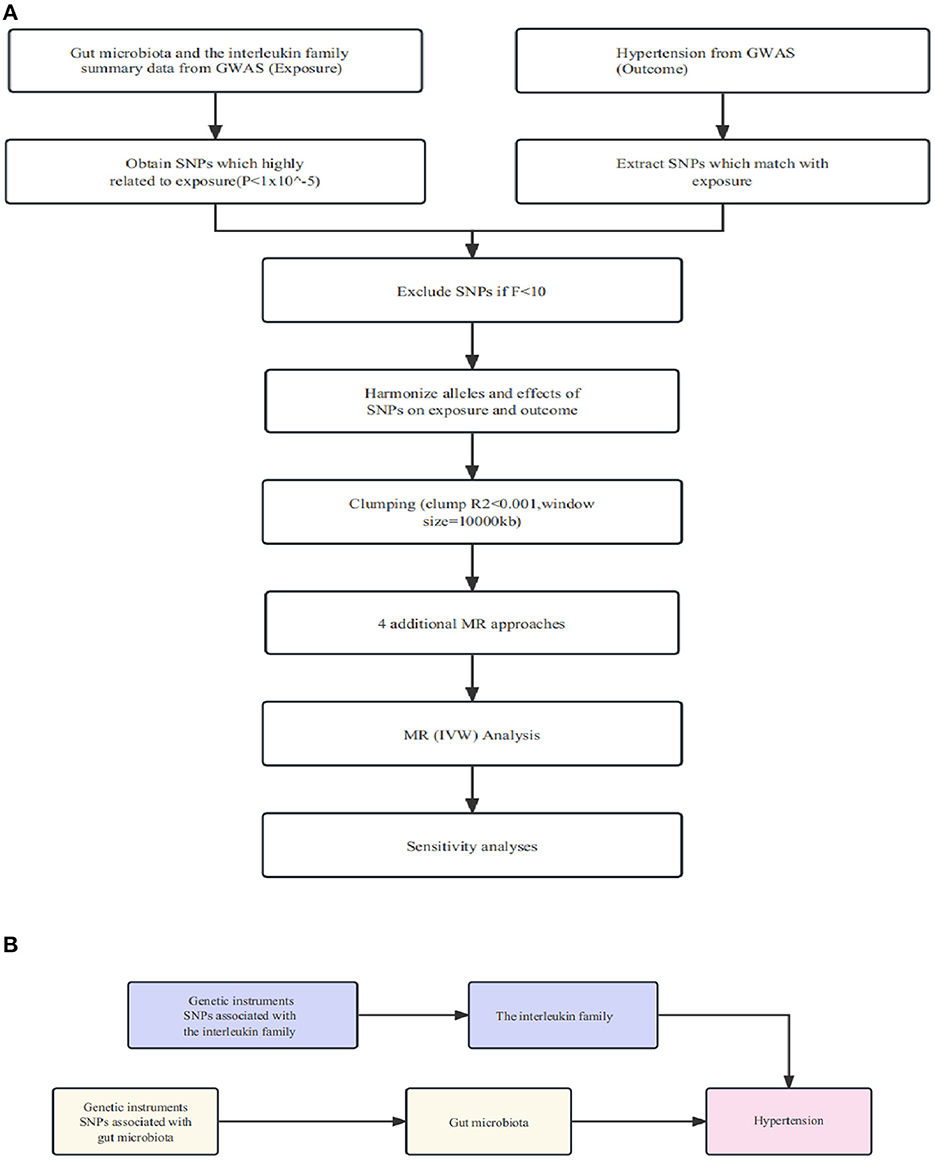
Figure 1. (A) Illustration of the study design and workflow. (B) Two-step Mendelian randomization assessment detailing the impact of gut microbiota on hypertension through IL-1R2.
2.2 Data sources
For exposure variables, we sourced summary statistics on gut microorganisms from a comprehensive, multi-ethnic GWAS meta-analysis involving 340,024 participants from 18 cohorts. This analysis comprised 211 taxa, including 131 genera, 35 families, 20 orders, 16 classes, and 9 phyla (17).
For mediator and outcome phenotypes, we relied on the data of the MRC-IEU consortium [IEU OpenGWAS project (bXJjaWV1LmFjLnVr)]. The study populations for these phenotypes were primarily of European descent (18). Specific data IDs can be referenced in Supplementary material S1.
2.3 Selection for genetic variation
Utilizing Plink Software, we first screened for single nucleotide polymorphisms (SNPs) from GWAS of gut microbiota with P < 1*10−5, a genetic distance of 10,000 kb, and a linkage disequilibrium parameter (r2) of <0.001. When the identified SNPs were absent in the outcomes GWAS summary data, we substituted them with proxies boasting an r2 ≥ 0.8. Subsequently, we computed the F statistic for each SNP to detect any weak IV bias (19). SNPs with an F statistic of <10 indicated potential weak IV bias, and to ensure accurate results, these were excluded from the study (20).
2.4 Statistical analysis
While the core focus of this study was on the results derived from the inverse variance weighting (IVW) method (19), it was essential to ensure the directional consistency of results across all utilized methods with the IVW. For a more rigorous secondary analysis, we employed MR-Egger regression, weighted median, simple mode, weighted mode, and MR-Pleiotropy Residual Sum and Outlier (MR-PRESSO) (15, 21–23) to provide a sensitivity examination for our IVW findings.
2.5 Pleiotropy and heterogeneity analysis
We initiated our analysis using the MR-PRESSO (23) approach to identify outliers and proceeded with a re-analysis after their exclusion. Subsequently, to assess any individual SNP's potential to drive the association between exposure and outcome, a leave-one-out sensitivity analysis was performed by iteratively removing one SNP at a time. Furthermore, to discern horizontal pleiotropy in MR analysis, the MR-Egger regression test was employed, emphasizing the statistical significance of the intercept term (24). Finally, we computed the Cochran Q statistic to detect heterogeneity (25), setting the significance of the threshold at P = 0.05. All statistical analyses were conducted in R, version 4.2.3, with the MR and MR-PRESSO packages.
3 Results
3.1 Instrument variables included in the analysis
Detailed information on the SNPs available for our study can be found in Supplementary Figure S2. All SNPs included in our analysis possessed an F-value exceeding 10.
3.2 Effects of microbial taxa on hypertension
In our investigation of the effects of microbial taxa on hypertension, we identified a positive association between hypertension and two gut microbiota taxa—the genus Clostridium innocuum group (OR = 1.0047, 95% CI: 1.0004–1.0090, p = 0.0336) and the genus Olsenella (OR = 1.0044, 95%CI: 1.0001–1.0088, p = 0.0430). The results suggest their potential to heighten hypertension risk (Figure 2; Supplementary Figures S3–S5). Conversely, five gut microbiota taxa, including the genus Senegalimassilia (OR = 0.9901, 95%CI: 0.9830–0.9973, p = 0.0072), the genus Parabacteroides (OR = 0.9874, 95%CI: 0.9776–0.9972, p = 0.0121), the Clostridiales vadin BB60 group family (OR = 0.9936, 95%CI: 0.9886–0.9986, p = 0.0125), the genus Desulfovibrio (OR = 0.9929, 95%CI: 0.9864–0.9995, p = 0.0342), and the genus Flavonifractor (OR = 0.9909, 95%CI: 0.9823–0.9997, p = 0.0416), were linked to a diminished hypertension risk, suggesting their possible antihypertensive properties (Figure 2; Supplementary Figures S3–S5). To validate the aforementioned results, we conducted a sensitivity analysis using the MR-Egger, weighted median, simple mode, and weighted mode methods (Supplementary Figure S6).
3.3 Effects of interleukin on hypertension
Through our MR analysis, we identified correlations between interleukin-related factors and hypertension risk. Specifically, three factors, namely, IL-1R2 (OR = 1.0038, 95%CI: 1.0007–1.0070, p = 0.0180), interleukin-23 (OR = 1.0021, 95%CI: 1.0001–1.0042, p = 0.0381), and interleukin-1 receptor-like 1 (OR = 1.0022, 95%CI: 1.0004–1.0041, p = 0.0176), displayed a positive association, suggesting they may heighten the risk of hypertension (Figure 3; Supplementary Figures S7–S9). Four factors, namely, interleukin-2 receptor subunit alpha levels (OR = 0.9974, 95%CI: 0.9958–0.9989, p = 0.0010), interleukin-11 receptor subunit alpha (OR = 0.9970, 95%CI: 0.9944–0.9996, p = 0.0250), interleukin-27 (OR = 0.9977, 95%CI: 0.9956–0.9999, p = 0.0385), and interleukin-31 (OR = 0.9978, 95%CI: 0.9959–0.9996, p = 0.0189), were inversely associated, indicating a potential protective effect against hypertension (Figure 3; Supplementary Figures S7–S9). We additionally performed a sensitivity analysis to corroborate this result (Supplementary Figure S10).
3.4 Effects of microbial taxa on IL-1R2
The results revealed a causal effect of the genetically predicted Clostridium innocuum group (OR = 1.1879, 95%CI: 1.0079–1.4001, p = 0.0400) on IL-1R2 as indicated by the IVW method (Figure 4; Supplementary Figures S11–S13). Given our earlier findings that established connections between “microbial taxa → hypertension” and “microbial taxa → IL-1R2”, we postulated that IL-1R2 may potentially mediate the relationship between microbial taxa and hypertension. We also conducted a sensitivity analysis to reinforce this conclusion (Supplementary Figure S14).
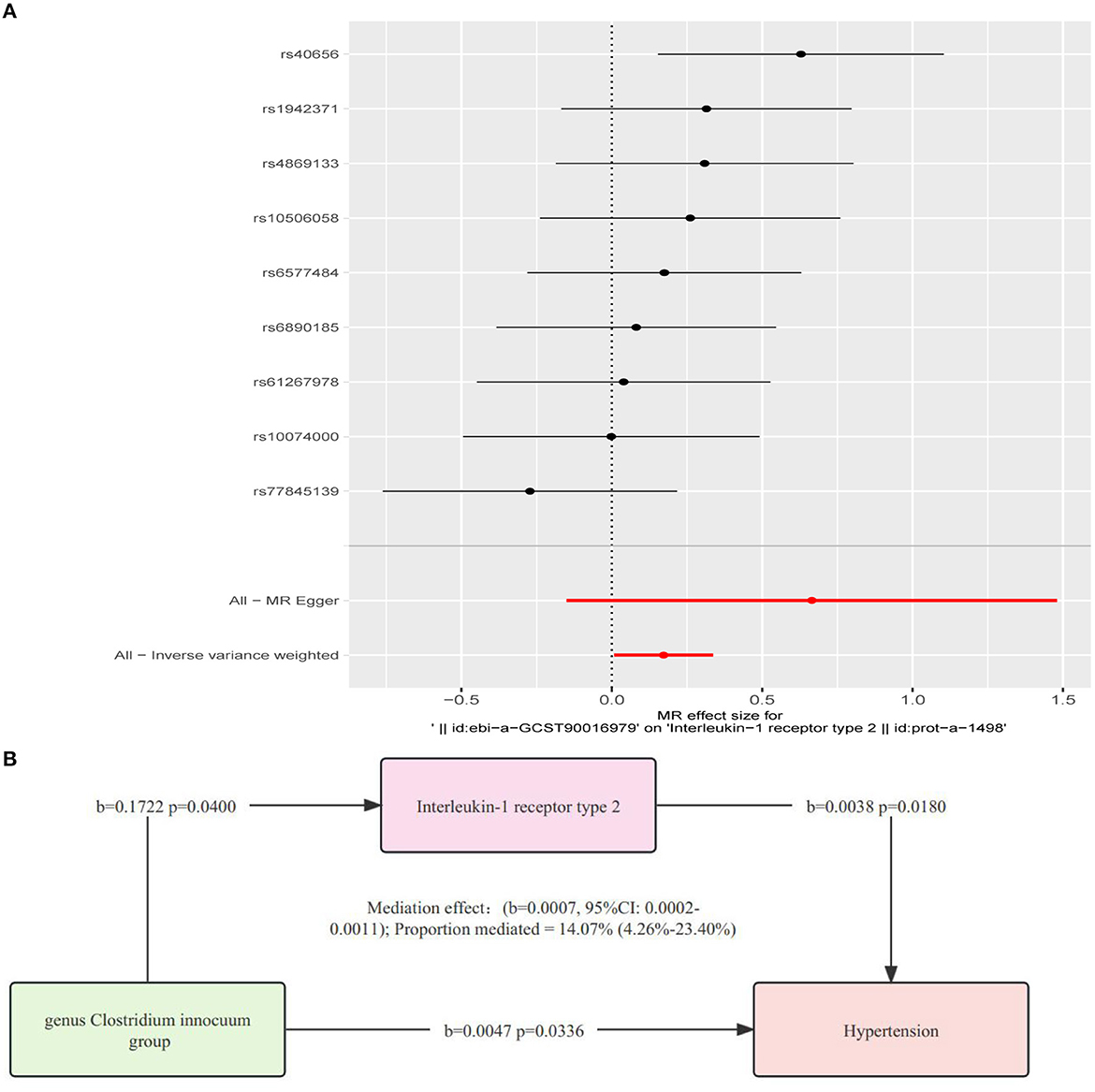
Figure 4. (A) Analysis of the causative relationship from Clostridium innocuum group to IL-1R2 (P < 1.00E-05). (B) Depiction of the role of interleukin-1 receptor type 2 in mediating the causal effect of gut microbiota on hypertension, with MR estimates for the connection among interleukin-1 receptor type 2, gut microbiome, and hypertension provided via the inverse variance-weighted approach.
3.5 The mediation effect of IL-1R2 in the causal association between the Clostridium innocuum group and hypertension
Using the two-step IL-1R2 method, we determined that IL-1R2 acts as a mediator in the causal relationship from the Clostridium innocuum group to hypertension (b = 0.0007, 95%CI: 0.0002–0.0011). The mediation effect was quantified at 14.07% (4.26–23.40%) (Figure 4).
4 Discussion
In this comprehensive mediation MR study, we identified a causal relationship between seven microbial taxa (including the Clostridium innocuum group) and hypertension risk. Mediation MR results suggest that IL-1R2 may account for 14.07% of the effect that the Clostridium innocuum group has on hypertension. This analysis underscores the link between gut microbiota and hypertension, highlighting the mediating role of IL-1R2.
Gut flora modulates the immune response in two primary ways: direct immune cell recognition, triggering an immune response, and regulation through metabolites like short-chain fatty acids (SCFAs) (26, 27). These SCFAs, such as butyric acid, influence the immune response not only in the intestines but also in the heart, brain, and other mucosal sites (28, 29). Notably, the intestinal bacteria Faecalibacterium, Ruminococcaceae, Coprococcus, and Roseburia are known producers of butyric acid (30, 31). A broader array, including the Parabacteroides and Clostridiales vadin BB60 group highlighted in our findings, produces other SCFAs such as acetate, propionate, and hexanoate (32, 33). The protective attributes of the Parabacteroides and the Clostridiales vadin BB60 group observed in our results may be attributed to their SCFA production. In addition, Shiyun Luo et al. found the protective role of Flavonifractor against atherosclerosis, suggesting a potential link between its observed hypertension protection in our study and its anti-atherosclerosis effects.
There is a prevailing consensus from animal model studies that the intestinal flora has a robust association with hypertension (34–38). While research has identified a positive relationship between certain bacterial strains such as Klebsiella, Anaerotruncus, and Eggerthella and hypertension and a negative relationship between bacterial strains such as Roseburia and Bifidobacterium and hypertension, the exact causality remains elusive (12, 14, 39–42). Emerging insights point to neuroinflammation as a potential intermediary in the influence of gut flora on hypertension. For instance, hypertensive mice introduced to standard mouse gut flora exhibited reduced neuroinflammation in the hypothalamic paraventricular nucleus and notably decreased blood pressure (43). Furthermore, in angiotensin II-induced hypertensive mice, anti-inflammatory treatments managed to restore their gut microbial balance (44). Wang et al. proposed a bi-directional regulation of gut flora and hypertension via both humoral and neural mechanisms (45), albeit with sparse evidence. Additionally, Santisteban et al. observed that an imbalanced gut flora and its metabolites could stimulate macrophages to release copious inflammatory factors, subsequently elevating blood pressure (46). Despite the limited direct evidence linking inflammation-mediated gut flora to hypertension, these findings illuminate promising avenues for future hypertension research.
Members of the IL-1 family or their receptors serve as therapeutic targets for several conditions, including atherosclerosis, type 2 diabetes, arthritis, and cancer (47, 48). IL-1R2, a primary IL-1 binding protein found in human neutrophils, monocytes, and B cells, plays a crucial role in regulating IL-1-mediated inflammatory responses (49–52). It also acts as a decoy receptor for the IL-1 system (49). Elevated IL-1R2 expression has been observed in various cancers, such as pancreatic ductal adenocarcinoma (53), prostate cancer, and benign prostatic hyperplasia (54). Additionally, Pou et al. established a connection between IL-1R2 and atherosclerotic vasculopathy (55). While the link between hypertension and inflammation is well acknowledged, to the best of our knowledge, no current studies delve into the relationship between IL-1R2 and hypertension. Our findings indicate that IL-1R2 could bridge the causal link between intestinal microorganisms and hypertension, laying the groundwork for exploring the intersection of hypertension and inflammation and potentially inspiring future therapeutic strategies for hypertension.
It is important to note that studies on differences in gut flora among hypertensive patients yield inconsistent results. For instance, both Dan et al. and Huart et al. observed a higher prevalence of Clostridium sensu stricto in hypertensive groups (56, 57). Verhaar et al. reported contrary findings (58). Similar inconsistencies are evident in studies exploring Bacteroides, Clostridiales, Lactobacillaceae, and Oscillibacter (12, 39, 40, 56, 59). Such discrepancies might arise from several factors: the studies might not have accounted for potential confounders such as gender, age, race, diet, and medication use. Furthermore, bacterial classifications might differ between studies. Another consideration is the possible influence of contrasting causal factors in hypertension, though their precise roles remain unclear.
We pioneered the use of mediation MR to investigate the causal relationship among the gut microbiome, inflammation, and hypertension. Not only did we utilize various common sensitivity analyses, but we also ruled out the influence of confounding factors and reverse causality. Our preliminary findings suggest a causal relationship between the gut microbiome and hypertension and its intermediaries, offering further theoretical support for hypertension treatment and prevention and presenting new approaches for its management. For instance, one could initially regulate specific gut microbes through diet, medication, or other means to manage hypertension. Additionally, researching targeted drugs against interleukin-related receptors might lead to significant breakthroughs in hypertension prevention and treatment.
Despite the many strengths of our study, such as a large sample size, the use of multiple sensitivity analysis methods to ensure research reliability, ruling out confounding factors, and clarifying causality, there are unavoidable limitations. Despite the significant sample size of the study, it is worth noting that participants were exclusively of European descent. Hence, our findings may not be entirely applicable to other regions or ethnicities. Furthermore, our results remain theoretical and have not been validated through clinical or animal experiments, leaving the specific mechanisms unclear. Further cellular, animal, and clinical experiments are needed to elucidate these mechanisms. Finally, our exposure data selected over 200 gut microbial species from the research of Kurilshikov et al. (17). In reality, there often exists more than one dataset for a single microbial species. We did not investigate all the data for each microbial species, which might lead to false positives or negatives or limit the applicability of our findings.
5 Conclusion
Our mediation MR research suggests potential causal relationships among the gut microbiome, inflammation, and hypertension. Specifically, the IL-1R2 pathway mediates a regulatory effect of the gut microbiome on blood pressure. Our findings provide genetic evidence of the connection between the gut microbiome, IL-1R2, and hypertension. This sheds light on new possibilities for hypertension prevention and treatment in the future, possibly by modulating specific gut microbial communities or interleukin-related targets.
Data availability statement
The original contributions presented in the study are included in the article/Supplementary material, further inquiries can be directed to the corresponding author.
Author contributions
SZ: Data curation, Formal analysis, Software, Supervision, Writing – original draft, Writing – review & editing. JZ: Conceptualization, Methodology, Writing – review & editing. FD: Supervision, Validation, Writing – review & editing. SS: Supervision, Writing – review & editing.
Funding
The author(s) declare that no financial support was received for the research, authorship, and/or publication of this article.
Conflict of interest
The authors declare that the research was conducted in the absence of any commercial or financial relationships that could be construed as a potential conflict of interest.
Publisher's note
All claims expressed in this article are solely those of the authors and do not necessarily represent those of their affiliated organizations, or those of the publisher, the editors and the reviewers. Any product that may be evaluated in this article, or claim that may be made by its manufacturer, is not guaranteed or endorsed by the publisher.
Supplementary material
The Supplementary Material for this article can be found online at: https://www.frontiersin.org/articles/10.3389/fnut.2023.1293170/full#supplementary-material
References
1. Skoog I. Hypertension and cognition. Int Psychogeriatr. (2003) 15(Suppl. 1):139–46. doi: 10.1017/S1041610203009104
2. Rapsomaniki E, Timmis A, George J, Pujades-Rodriguez M, Shah AD, Denaxas S, et al. Blood pressure and incidence of twelve cardiovascular diseases: lifetime risks, healthy life-years lost, and age-specific associations in 1·25 million people. Lancet. (2014) 383:1899–911. doi: 10.1016/S0140-6736(14)60685-1
3. Li L, Scott CA, Rothwell PM. Association of younger vs. older ages with changes in incidence of stroke and other vascular events, 2002-2018. JAMA. (2022) 328:563–74. doi: 10.1001/jama.2022.12759
4. Szczech LA, Granger CB, Dasta JF, Amin A, Peacock WF, McCullough PA, et al. Acute kidney injury and cardiovascular outcomes in acute severe hypertension. Circulation. (2010) 121:2183–91. doi: 10.1161/CIRCULATIONAHA.109.896597
5. Lewington S, Clarke R, Qizilbash N, Peto R, Collins R. Age-specific relevance of usual blood pressure to vascular mortality: a meta-analysis of individual data for one million adults in 61 prospective studies. Lancet. (2002) 360:1903–13. doi: 10.1016/S0140-6736(02)11911-8
6. Evangelou E, Warren HR, Mosen-Ansorena D, Mifsud B, Pazoki R, Gao H, et al. Genetic analysis of over 1 million people identifies 535 new loci associated with blood pressure traits. Nat Genet. (2018) 50:1412–25. doi: 10.1038/s41588-018-0205-x
7. Slomski A. Effect of modified chinese diet is comparable to antihypertensives. JAMA. (2022) 328:700. doi: 10.1001/jama.2022.14322
8. Gruber T, Pan C, Contreras RE, Wiedemann T, Morgan DA, Skowronski AA, et al. Obesity-associated hyperleptinemia alters the gliovascular interface of the hypothalamus to promote hypertension. Cell Metab. (2021) 33:1155–70.e1110. doi: 10.1016/j.cmet.2021.04.007
9. Clemente JC, Ursell LK, Parfrey LW, Knight R. The impact of the gut microbiota on human health: an integrative view. Cell. (2012) 148:1258–70. doi: 10.1016/j.cell.2012.01.035
10. Floch MH. Intestinal microecology in health and wellness. J Clin Gastroenterol. (2011) 45(Suppl.):S108–10. doi: 10.1097/MCG.0b013e3182309276
11. Marques FZ, Mackay CR, Kaye DM. Beyond gut feelings: how the gut microbiota regulates blood pressure. Nat Rev Cardiol. (2018) 15:20–32. doi: 10.1038/nrcardio.2017.120
12. Li J, Zhao F, Wang Y, Chen J, Tao J, Tian G, et al. Gut microbiota dysbiosis contributes to the development of hypertension. Microbiome. (2017) 5:14. doi: 10.1186/s40168-016-0222-x
13. Ganesh BP, Nelson JW, Eskew JR, Ganesan A, Ajami NJ, Petrosino JF, et al. Prebiotics, probiotics, and acetate supplementation prevent hypertension in a model of obstructive sleep apnea. Hypertension. (2018) 72:1141–50. doi: 10.1161/HYPERTENSIONAHA.118.11695
14. Yan Q, Gu Y, Li X, Yang W, Jia L, Chen C, et al. Alterations of the gut microbiome in hypertension. Front Cell Infect Microbiol. (2017) 7:381. doi: 10.3389/fcimb.2017.00381
15. Bowden J, Davey Smith G, Burgess S. MR with invalid instruments: effect estimation and bias detection through Egger regression. Int J Epidemiol. (2015) 44:512–25. doi: 10.1093/ije/dyv080
16. Davies NM, Holmes MV, Davey Smith G. Reading MR studies: a guide, glossary, and checklist for clinicians. BMJ. (2018) 362:k601. doi: 10.1136/bmj.k601
17. Kurilshikov A, Medina-Gomez C, Bacigalupe R, Radjabzadeh D, Wang J, Demirkan A, et al. Large-scale association analyses identify host factors influencing human gut microbiome composition. Nat Genet. (2021) 53:156–5. doi: 10.1038/s41588-020-00763-1
18. Sudlow C, Gallacher J, Allen N, Beral V, Burton P, Danesh J, et al. UK biobank: an open access resource for identifying the causes of a wide range of complex diseases of middle and old age. PLoS Med. (2015) 12:e1001779. doi: 10.1371/journal.pmed.1001779
19. Palmer TM, Lawlor DA, Harbord RM, Sheehan NA, Tobias JH, Timpson NJ, et al. Using multiple genetic variants as instrumental variables for modifiable risk factors. Stat Methods Med Res. (2012) 21:223–42. doi: 10.1177/0962280210394459
20. Ziegler A, Pahlke F, König IR. Comments on 'MR: using genes as instruments for making causal inferences in epidemiology. Statist Med. (2008) 27:1133–63. doi: 10.1002/sim.3034
21. Pierce BL, Burgess S. Efficient design for MR studies: subsample and 2-sample instrumental variable estimators. Am J Epidemiol. (2013) 178:1177–84. doi: 10.1093/aje/kwt084
22. Bowden J, Davey Smith G, Haycock PC, Burgess S. Consistent estimation in MR with some invalid instruments using a weighted median estimator. Genet Epidemiol. (2016) 40:304–14. doi: 10.1002/gepi.21965
23. Verbanck M, Chen CY, Neale B, Do R. Publisher correction: detection of widespread horizontal pleiotropy in causal relationships inferred from MR between complex traits and diseases. Nat Genet. (2018) 50:1196. doi: 10.1038/s41588-018-0164-2
24. Burgess S, Thompson SG. Erratum to: interpreting findings from MR using the MR-Egger method. Eur J Epidemiol. (2017) 32:391–2. doi: 10.1007/s10654-017-0276-5
25. Egger M, Smith GD, Phillips AN. Meta-analysis: principles and procedures. BMJ. (1997) 315:1533–7. doi: 10.1136/bmj.315.7121.1533
26. Lavelle EC, Murphy C, O'Neill LA, Creagh EM. The role of TLRs, NLRs, and RLRs in mucosal innate immunity and homeostasis. Mucosal Immunol. (2010) 3:17–28. doi: 10.1038/mi.2009.124
27. Steimle A, Frick JS. Molecular mechanisms of induction of tolerant and tolerogenic intestinal dendritic cells in mice. J Immunol Res. (2016) 2016:1958650. doi: 10.1155/2016/1958650
28. Liu H, Wang J, He T, Becker S, Zhang G, Li D, et al. Butyrate: a double-edged sword for health? Adv Nutr. (2018) 9:21–9. doi: 10.1093/advances/nmx009
29. Wu W, Sun M, Chen F, Cao AT, Liu H, Zhao Y, et al. Microbiota metabolite short-chain fatty acid acetate promotes intestinal IgA response to microbiota which is mediation by GPR43. Mucosal Immunol. (2017) 10:946–56. doi: 10.1038/mi.2016.114
30. Louis P, Flint HJ. Formation of propionate and butyrate by the human colonic microbiota. Environ Microbiol. (2017) 19:29–41. doi: 10.1111/1462-2920.13589
31. Louis P, Flint HJ. Diversity, metabolism and microbial ecology of butyrate-producing bacteria from the human large intestine. FEMS Microbiol Lett. (2009) 294:1–8. doi: 10.1111/j.1574-6968.2009.01514.x
32. Che L, Hu Q, Wang R, Zhang D, Liu C, Zhang Y, et al. Inter-correlated gut microbiota and SCFAs changes upon antibiotics exposure links with rapid body-mass gain in weaned piglet model. J Nutr Biochem. (2019) 74:108246. doi: 10.1016/j.jnutbio.2019.108246
33. Lei Y, Tang L, Liu S, Hu S, Wu L, Liu Y, et al. Parabacteroides produces acetate to alleviate heparanase-exacerbated acute pancreatitis through reducing neutrophil infiltration. Microbiome. (2021) 9:115. doi: 10.1186/s40168-021-01065-2
34. Jaworska K, Koper M, Ufnal M. Gut microbiota and renin-angiotensin system: a complex interplay at local and systemic levels. Am J Physiol Gastrointest Liver Physiol. (2021) 321:G355–66. doi: 10.1152/ajpgi.00099.2021
35. Saha P, Mell B, Golonka RM, Bovilla VR, Abokor AA, Mei X, et al. Selective IgA deficiency in spontaneously hypertensive rats with gut dysbiosis. Hypertension. (2022) 79:2239–49. doi: 10.1161/HYPERTENSIONAHA.122.19307
36. Hsu C-N, Yu H-R, Lin I-C, Tiao M-M, Huang L-T, Hou C-Y, et al. Sodium butyrate modulates blood pressure and gut microbiota in maternal tryptophan-free diet-induced hypertension rat offspring. J Nutr Biochem. (2022) 108:109090. doi: 10.1016/j.jnutbio.2022.109090
37. Drapkina OM, Yafarova AA, Kaburova AN, Kiselev AR. Targeting gut microbiota as a novel strategy for prevention and treatment of hypertension, atrial fibrillation and heart failure: current knowledge and future perspectives. Biomedicines. (2022) 10:82019. doi: 10.3390/biomedicines10082019
38. Shi H, Nelson JW, Phillips S, Petrosino JF, Bryan RM, Durgan DJ. Alterations of the gut microbial community structure and function with aging in the spontaneously hypertensive stroke prone rat. Sci Rep. (2022) 12:8534. doi: 10.1038/s41598-022-12578-7
39. Sun S, Lulla A, Sioda M, Winglee K, Wu MC, Jacobs DR Jr, et al. Gut microbiota composition and blood pressure. Hypertension. (2019) 73:998–1006. doi: 10.1161/HYPERTENSIONAHA.118.12109
40. Kim S, Goel R, Kumar A, Qi Y, Lobaton G, Hosaka K, et al. Imbalance of gut microbiome and intestinal epithelial barrier dysfunction in patients with high blood pressure. Clin Sci. (2018) 132:701–18. doi: 10.1042/CS20180087
41. Ko C-Y, Liu Q-Q, Su H-Z, Zhang H-P, Fan J-M, Yang J-H, et al. Gut microbiota in obstructive sleep apnea-hypopnea syndrome: disease-related dysbiosis and metabolic comorbidities. Clin Sci. (2019) 133:905–17. doi: 10.1042/CS20180891
42. Yu Y, Mao G, Wang J, Zhu L, Lv X, Tong Q, et al. Gut dysbiosis is associated with the reduced exercise capacity of elderly patients with hypertension. Hypertens Res. (2018) 41:1036–44. doi: 10.1038/s41440-018-0110-9
43. Toral M, Robles-Vera I, De la Visitacion N, Romero M, Yang T, Sánchez M, et al. Critical role of the interaction gut microbiota - sympathetic nervous system in the regulation of blood pressure. Front Physiol. (2019) 10:231. doi: 10.3389/fphys.2019.00231
44. Sharma RK, Yang T, Oliveira AC, Lobaton GO, Aquino V, Kim S, et al. Microglial cells impact gut microbiota and gut pathology in angiotensin II-induced hypertension. Circ Res. (2019) 124:727–36. doi: 10.1161/CIRCRESAHA.118.313882
45. Wang W, Zhu LJ, Leng YQ, Wang YW, Shi T, Wang WZ, et al. Inflammatory response: a crucial way for gut microbes to regulate cardiovascular diseases. Nutrients. (2023) 15:607. doi: 10.3390/nu15030607
46. Santisteban MM, Qi Y, Zubcevic J, Kim S, Yang T, Shenoy V, et al. Hypertension-linked pathophysiological alterations in the gut. Circ Res. (2017) 120:312–23. doi: 10.1161/CIRCRESAHA.116.309006
47. Dinarello CA, Simon A, van der Meer JWM. Treating inflammation by blocking interleukin-1 in a broad spectrum of diseases. Nat Rev Drug Discov. (2012) 11:633–52. doi: 10.1038/nrd3800
48. Liew FY, Pitman NI, McInnes IB. Disease-associated functions of IL-33: the new kid in the IL-1 family. Nat Rev Immunol. (2010) 10:103–10. doi: 10.1038/nri2692
49. Colotta F, Re F, Muzio M, Bertini R, Polentarutti N, Sironi M, et al. Interleukin-1 type II receptor: a decoy target for IL-1 that is regulated by IL-4. Science. (1993) 261:472–5. doi: 10.1126/science.8332913
50. McMahan CJ, Slack JL, Mosley B, Cosman D, Lupton SD, Brunton LL, et al. A novel IL-1 receptor, cloned from B cells by mammalian expression, is expressed in many cell types. EMBO J. (1991) 10:2821–32. doi: 10.1002/j.1460-2075.1991.tb07831.x
51. Re F, Sironi M, Muzio M, Matteucci C, Introna M, Orlando S, et al. Inhibition of interleukin-1 responsiveness by type II receptor gene transfer: a surface “receptor” with anti-interleukin-1 function. J Exp Med. (1996) 183:1841–50. doi: 10.1084/jem.183.4.1841
52. Colotta F, Saccani S, Giri JG, Dower SK, Sims JE, Introna M, et al. Regulated expression and release of the IL-1 decoy receptor in human mononuclear phagocytes. J Immunol. (1996) 156:2534–41. doi: 10.4049/jimmunol.156.7.2534
53. Rückert F, Dawelbait G, Winter C, Hartmann A, Denz A, Ammerpohl O, et al. Examination of apoptosis signaling in pancreatic cancer by computational signal transduction analysis. PLoS ONE. (2010) 5:e12243. doi: 10.1371/journal.pone.0012243
54. Ricote M, García-Tuñón I, Bethencourt FR, Fraile B, Paniagua R, Royuela M. Interleukin-1 (IL-1alpha and IL-1beta) and its receptors (IL-1RI, IL-1RII, and IL-1Ra) in prostate carcinoma. Cancer. (2004) 100:1388–96. doi: 10.1002/cncr.20142
55. Pou J, Martínez-González J, Rebollo A, Rodríguez C, Rodríguez-Calvo R, Martín-Fuentes P, et al. Type II interleukin-1 receptor expression is reduced in monocytes/macrophages and atherosclerotic lesions. Biochim Biophys Acta. (2011) 1811:556–63. doi: 10.1016/j.bbalip.2011.05.014
56. Dan X, Mushi Z, Baili W, Han L, Enqi W, Huanhu Z, et al. Erratum: differential analysis of hypertension-associated intestinal microbiota. Int J Med Sci. (2021) 18:3748. doi: 10.7150/ijms.65972
57. Huart J, Leenders J, Taminiau B, Descy J, Saint-Remy A, Daube G, et al. Gut microbiota and fecal levels of short-chain fatty acids differ upon 24-hour blood pressure levels in men. Hypertension. (2019) 74:1005–13. doi: 10.1161/HYPERTENSIONAHA.118.12588
58. Verhaar BJH, Collard D, Prodan A, Levels JH, Zwinderman AH, Bäckhed F, et al. Associations between gut microbiota, faecal short-chain fatty acids, and blood pressure across ethnic groups: the HELIUS study. Eur Heart J. (2020) 41:4259–67. doi: 10.1093/eurheartj/ehaa704
Keywords: gut microbiome, hypertension, Mendelian randomization, interleukin, mediation
Citation: Zhao S, Zhang J, Ding F and Sun S (2023) Relationships among gut microbes, the interleukin family, and hypertension: a mediation Mendelian randomization study. Front. Nutr. 10:1293170. doi: 10.3389/fnut.2023.1293170
Received: 12 September 2023; Accepted: 31 October 2023;
Published: 28 November 2023.
Edited by:
Anil Kumar Sharma, Maharishi Markandeshwar University, IndiaReviewed by:
Nirmala Sehrawat, Maharishi Markandeshwar University, IndiaZhuoli Zhang, University of California, Irvine, United States
Copyright © 2023 Zhao, Zhang, Ding and Sun. This is an open-access article distributed under the terms of the Creative Commons Attribution License (CC BY). The use, distribution or reproduction in other forums is permitted, provided the original author(s) and the copyright owner(s) are credited and that the original publication in this journal is cited, in accordance with accepted academic practice. No use, distribution or reproduction is permitted which does not comply with these terms.
*Correspondence: Shougang Sun, c3Vuc2hnODA2QDEyNi5jb20=