- 1Food Science Department, Faculty of Agriculture, Saba Basha, Alexandria University, Alexandria, Egypt
- 2Food Industry Technology Program, Faculty of Industrial and Energy Technology, Borg Al Arab Technological University (BATU), Alexandria, Egypt
- 3Food Technology Department, Arid Lands Cultivation Research Institute, City of Scientific Research and Technological Applications (SRTA-City), Alexandria, Egypt
Protein complexes with a nutritional value, heat stability, and gelling properties with no negative impact on culture viability have promising application prospects in the fermentation industry. The aim of the study was to investigate the possibility of applying physical modification seeking high-protein-fortified yoghurt production using the nano casein–pectin NCP complex as an active colloidal system with enhanced structural and thermal properties and monitor the quality properties of the physicochemical, heat stability, rheological, starter culture viability and sensory evaluation of fortified products comparing with the plain control throughout the cold storage. High-energy ball milling (HEBM) technique was used to produce nanoparticles of casein powder and smaller particles of pectin individually, and particle size and zeta potential was assessed. Deferent Nano casein-pectin (NCP) complex formulations were prepared, their physicochemical properties were assessed including protein quality via Amino Acid Analyzer (AAA), viscosity, thermogravimetric analysis (TGA), and then used in fortification of skimmed milk and low-fat yoghurt to monitor the fortification effects. The particle sizes showed to be ≈166 nm and 602.6 nm for nano-casein and pectin, respectively. Milk fortification with the NCP complex has significantly increased the nutritional value represented in increased protein content (7.19 g/100 g in NCP5); Ca, P, and S content (2,193.11, 481.21, and 313.77 ppm); and amino acid content with first limiting amino acids; histidine (0.89 mg/g), methionine (0.89 mg/g), and low content of hydrophobic amino acids (HAAs) may cause aggregation. NPC fortification enhanced physicochemical properties announced in enhanced viscosity (62. mP.s in NCP5) and heat stability (up to 200°C) compared with control skimmed milk (SM). NCP yoghurt fortification significantly increased protein content to 11 mg/100 g in T5, enhanced viscosity to 48.44 mP.s in T3, decreased syneresis to 16% in T5, and enhanced LAB viability which was translated in preferable sensorial properties. Applying fortification with nanoparticles of the casein–pectin (NCP) complex balanced the amino acid content and improved physicochemical, rheological, nutritional, and sensorial properties and LAB viability, which can be recommended further in functional food applications.
1 Introduction
Biological capabilities of yoghurt are due to the presence of bioactive peptides that are formed during the fermentation process. The term “functional food” is often used to refer to food products with demonstrated physiological benefits that are useful to the human body in some way. Functional foods designed for athletes have emerged as a novel sector of special-purpose food products. High-protein yogurt types with high content of protein could be beneficial in sports nutrition due to the increased amino acids that can trigger muscle protein synthesis and help in a calorie-restricted diet (1). The importance of protein fortification in yoghurt production is based on its easier digestibility due to the bacterial pre-digestion of proteins in yoghurt. Bacterial cultures showed to have more proteolytic activity, especially Lactobacillus bulgaricus during milk fermentation and storage than Streptococcus thermophilus, affecting rheological behavior, acidity, and lactic acid bacteria (LAB) profile during the cold storage (2, 3).
Casein is the main component of bovine milk (80% of the total milk protein), which is an important source of essential amino acids, bioactive peptides, a natural carrier for calcium and phosphate, and stable against a variety of milk-processing procedures (4). Development of protein complex nanoparticles balances the amino acid content and improves rheological and nutritional properties. Pectins have an influence on the textural properties of protein-pectin systems as they can reinforce the matrix that may be an alternative to modified starch commonly used in the food industry (5). The combination of casein nanoparticles–polysaccharide complexes such as pectin increases thermal stability as complexation occurs as a result of electrostatic interactions between anionic polysaccharides and positively charged proteins. Depending on the electrostatic conditions, the complexes will remain as small, soluble complexes that better prevent occasional aggregation of casein in aqueous solutions with attractive functions (6–8).
Hydrocolloids have a wide array of functional properties in foods. These include thickening, gelling, emulsifying, and stabilization. The formation of acid casein gels has been previously studied with different protein substrates including skim milk and micellar casein. Due to the differences in processing conditions, the aggregation states are likely to be different in these casein-based milk protein powders (9). Physical methods were applied to modify casein crosslinking, seeking enhanced functionality and structural properties (10). An association colloid is a colloid whose particles are made up of even smaller molecules as nanoparticles, which was used to deliver polar, non-polar, and amphiphilic functional ingredients through which the gels are formed (11). One application of interest is in dairy products with the benefit of increased thermal stability, where the primary concern for this application would be colloidal stability, which would be predicted by a small particle size. This could be accomplished by combining protein–pectin complexes (7). To the best of our knowledge, no report has shown the effect of casein pectin complexes on the structural and sensory characteristics of low-fat dairy products. However, the interactions between casein and polysaccharides were reported to naturally occur in some beverages and fruity dairy products (6, 12).
The aim of this study was to investigate the possibility of applying physical modification seeking high-protein-fortified yoghurt production using the nano casein–pectin complex as an active colloidal system with enhanced structural and thermal properties and monitor the quality properties of the physicochemical, technological (heat stability and rheological), starter culture viability, and sensory evaluation of the fortified low-fat yoghurt matrix compared with a full-fat throughout 15 days of cold storage.
2 Materials and methods
2.1 Materials
Pectin was obtained from Fufeng Biotechnology Co., Ltd. (Inner Mongolia, China). Casein soluble powder (500 g) was obtained from Merck, KGaA (Darmstadt, Germany). Ammonia solution (33%) was obtained from Elnasr Pharmaceutical Chemicals (Alexandria, Egypt). Skimmed milk powder (0.5% fat, 12% TS, 3.37% protein, 0.84% ash) was purchased from the local market.
2.2 Nano casein and pectin preparation
The high-energy ball milling (HEBM) technique was used to produce nanoparticles of casein powder and smaller particles of pectin individually in steel cells using hardened steel balls (diameter 15 mm and weight 32 gm) in the ambient atmosphere in different batches ranging from 2 to 50 h. The mechanical milling was performed in the planetary ball mill (Retsch, PM 400, Germany) operating at 25 Hz. The milled materials were used directly with no added milling media.
2.3 Particle size and zeta potential
The particle size casein and pectin particles were measured using a dynamic light scattering instrument (DLS) (Mastersizer 2000, Malvern Instruments, Malvern, United Kingdom). The particle size of each sample was represented as the surface-weighted mean diameter (d32), which was calculated from the full particle size distribution. The droplet charge (zeta potential) of the particles measured using particle micro-electrophoresis (Zetasizer Nano ZS-90, Malvern Instruments, Worcestershire, United Kingdom) at Standard Operation Procedure (SOP) was 1.570 and 1.547 for casein and pectin, respectively (13).
2.4 Nano casein-pectin (NCP) complex formulations
Figure 1 illustrates nano casein–pectin (NCP) complex formulation preparation. Nano-casein (≈166 nm) and pectin (≈602.6 nm) were dissolved in 100 mL distilled water. Ammonia solution (80%) was added for alkaline conditions (pH 10–11) for gelation. The complex was then heated to 85°C/ 2 h with continuous stirring by a magnetic stirrer (JR-2, Tianjin Ouno Instruments Co., Ltd.) till fully dissolved. Different ratios of nano-casein: pectin (1:1, 2:1, 3:1, 4:1, 5:1) were used for NCP complex formulations, as illustrated in Table 1. The formulations were pasteurized at 85°C/25 min and cooled and aged at 5°C/24 h. As middle concentration, NCP3 was selected for comparison against skimmed milk. Skimmed milk (12%) was fortified with different levels of NCP complex formulations (1, 2, and 3%) to select the optimum level of fortification in yoghurt application.
2.5 Physicochemical analysis
Total solids and fat were determined according to the Official Methods of Analysis (14). pH was measured using an ADWA pH meter (model AD1030; ADWA Instruments, Romania). Titratable acidity was assessed by titrating with 0.1 NaOH using phenolphthalein indicators and expressed as an equivalent percentage of lactic acid according to Ling (15). Concentrations of minerals such as; calcium, phosphorus, and sulfur (Ca, P and S) were determined using Atomic Absorption Spectrometry (AAS) according to Beaty and Kerber (16).
2.6 Amino acid profile
Amino acid analysis was carried out by the Automatic Amino Acid Analyzer (AAA 400 INGOS Ltd) using the performic acid oxidation method according to INGOS (17) and Smith (18).
2.7 Viscosity determination
The viscosity of NCP complex formulations was determined using the J.P. Selecta Wide Range Rotary Viscometer (Model STS-2011, Valencia, Spain) set to 100 rpm/20°C, and spindles were changed accordingly from spindle L1 to L4 as viscosity increased. After 50 s of shearing, the results were reported in mPc (19).
2.8 Thermogravimetric analysis (TGA)
Nano casein–pectin complex formulation thermal stability was assessed using a thermogravimetric analyzer (Shimadzu TGA–50, Japan). All samples were heated at a rate of 20°C/ min underflow of N2 up to 200°C (20).
2.9 Preparation of fortified yoghurt
Pasteurized skimmed milk (12% SNF) was heat-treated at 85°C for 15 min. Milk then was divided into five equal portions, T1 with control plain yoghurt and four treatments, T2, T3, T4, and T5, of skimmed milk fortified with different NCP complex formulations NCP2, NCP3, NCP4, and NCP5, respectively. Different NCP complex formulations were added with a fortification percent of 3% (v/v) of skimmed milk. The mix was cooled to 42°C, then inoculated with (0.03 g/kg) of common yogurt cultures YO-PROX 003_100 UA (France), poured into 100 mL plastic cups, incubated at 42°C for 5 h until coagulation at pH ~4.6, and then cooled and stored at 4°C (21). Figure 2 exhibits a schematic diagram of fortified yoghurt preparations.
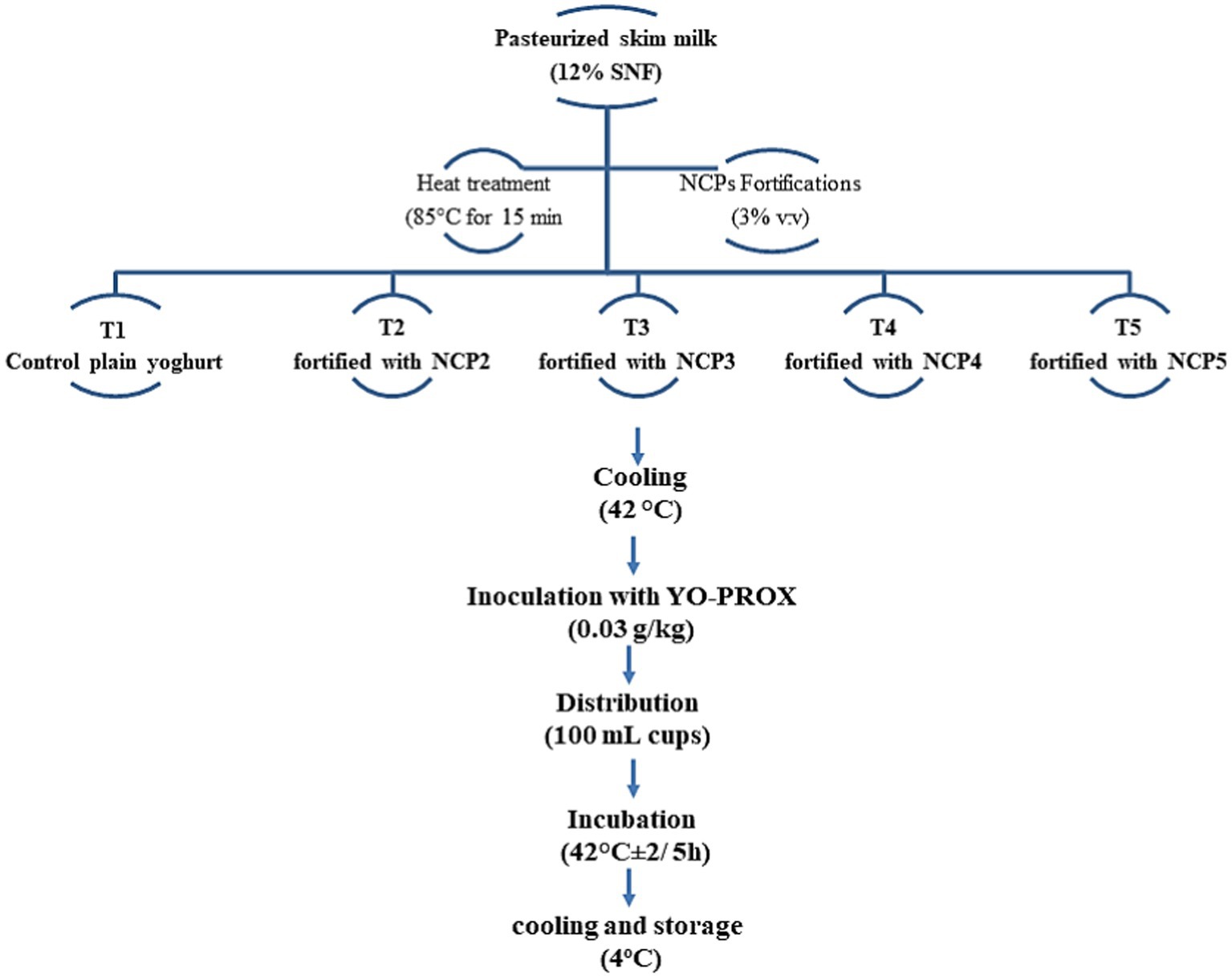
Figure 2. Schematic diagram of fortified yoghurt preparations. NCP1, NCP2, NCP3, NCP4, NCP5: nano–casein: pectin (1:1, 2:1, 3:1, 4:1, 5:1), respectively.
2.10 Syneresis determination
The yoghurt susceptibility to syneresis was determined by the method reported by Isanga and Zhang (22).
2.11 Microbiological analysis
The conventional diluting pouring plate technique was used for enumerating microbes in the samples. The microbiological analyzes were conducted as described by Standard Methods for the Examination of Dairy Products (23). For members of lactic acid bacteria count, MRS agar (Biolife, Italy) was used; for coliform counts, violet red bile agar (VRBA) (Biolife, Italy) was used, and the plates were incubated at 37°C for 48 h. The enumeration of yeast and mold was conducted using the rose bengal chloramphenicol agar (HIMedia, India), and the plates were incubated at 21°C for 5 days. The results were calculated directly as the colony forming unit (CFU/g).
2.12 Sensory evaluation
Under the supervision and approval of ethical committee, ten panelists (8 men and 2 women, aged between 27 and 58 years), conducted sensory evaluation on yoghurt samples at Food Science Department, Faculty of Agriculture, Saba Basha Alexandria University, Egypt, as described by Darwish et al. (24), Senaka Ranadheera et al. (25), and Ozturk et al. (26). The samples, which were stored at 4°C, were allowed to rest at room temperature (25°C) for 10 min before evaluation. The samples were evaluated using a 10-point Hedonic scale (27). This scale consisted of the test parameters of color, odor, flavor, taste, appearance, acidity, consistency, and overall acceptability, accompanied by a scale of ten categories as 1 = dislike extremely; 2 = dislike much; 3 = dislike moderately; 4 = dislike slightly, 5 = neither dislike nor like, 6 = like slightly; 7 = like moderately; 8 = like much; and 9 and 10 = like extremely. Sensory evaluation was conducted on NCP-fortified yoghurt on 1, 5, 10, and 15 days of cold storage.
2.13 Statistical analysis
IBM SPSS version 16.0 was used to examine the data given to the computer (28). All data were expressed as mean values ± SD. Statistical analysis was performed using one-way analysis of variance (ANOVA) followed by Duncan’s test. The obtained results were deemed significant at a p-value of <0.05 (29).
3 Results and discussion
3.1 Particle size and zeta potential characterization of nano casein and pectin particles
Casein as colloidal particles in milk represents approximately 65% of the total milk proteins. Milk casein which is made up of four proteins, αs1, β, αs2, and κ-casein (30), is promising to be used in a complex with polysaccharides or fibers. Figures 3A,B illustrates particle size of casein and pectin particles. Figure 3A showed that the majority of casein particle size was 166.2 nm with an intensity of 53.7%, while pectin particle size was 602.6 nm. Nanoparticles differ in behavior in the gastrointestinal tract compared with conventional particles due to the very small size and high surface area of nanoparticles. The toxicity is also related to factors such as particle size, zeta potential, surface group, and aggregation state (31). However, reports and regulatory organizations limited the term and the hazard to materials with particle size <100 nm which is not applied in the current research (32, 33).
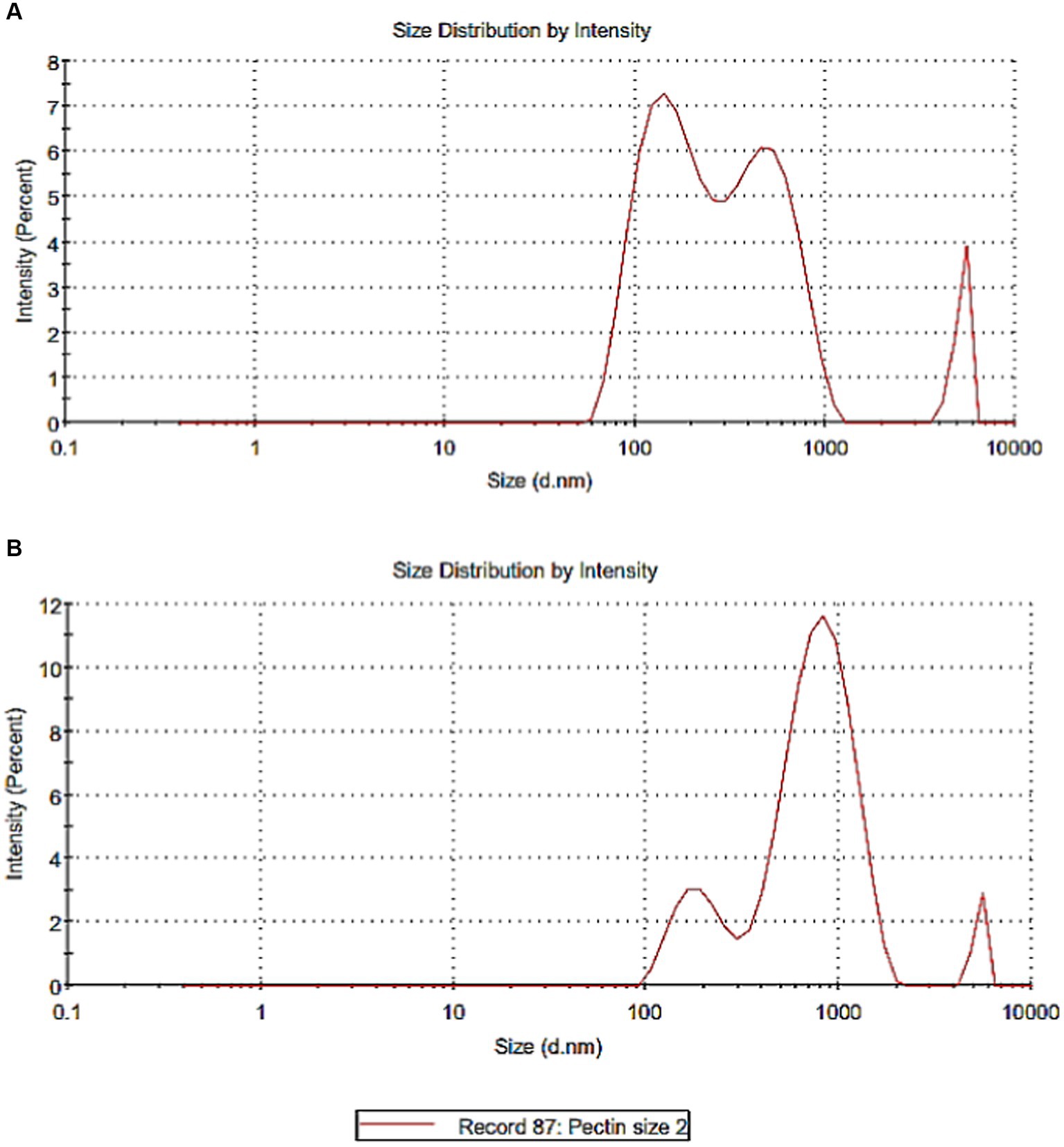
Figure 3. Particle size of casein and pectin particles. (A) Particle size of casein. (B) Particle size of pectin.
Zeta potentiometry was used to estimate the surface charge of the casein and pectin particles to determine their suspension stability (Figures 4A, B). The results showed that the zeta potential for casein and pectin particles were − 15.70 and − 29.10 mV, respectively, suggesting strong repulsive forces and electrostatic stabilization between them. Electrostatically stabilized nanosuspensions have a maximum zeta potential of −30 mV (34). Some studies reported possible interactions between starches and casein including electrostatic adhesion, steric stabilization, and hydrogen bond (6). The stability and structural behavior of complexes are controlled by many parameters such as the protein surface characteristics, charge density, degree of pectin esterification, pH, temperature, and biopolymer ratio; consequently, using nanosized casein can allow more stable interactions with pectin (6, 35).
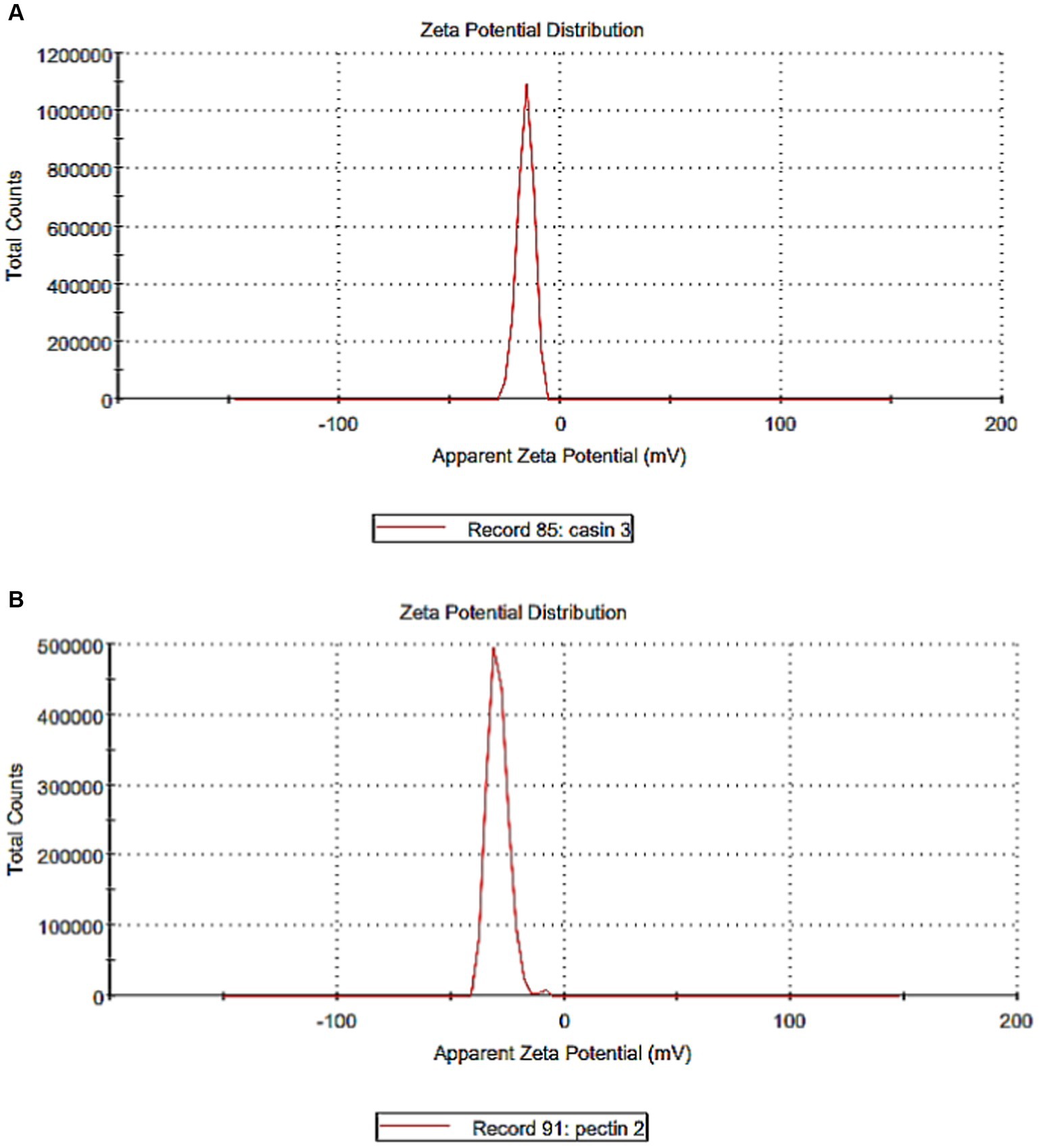
Figure 4. Zeta potential of casein and pectin particles. (A) Zeta potential of casein particles. (B) Zeta potential of pectin particles.
3.2 Chemical characterization of NCP complex fortified milk
3.2.1 Nutrients content of NCP complex fortified milk
Table 2 illustrates the chemical composition of NCP complex fortified milk with three fortification percent (1, 2, and 3%). Results indicated that protein represents the main component in NCP complex fortified milk (4.72–7.25 g/100 g). Insignificant changes concerning fat content were recorded (approx. 0.3 g/100 g), while significant increases in total solid (up to 12.79 g/100 g) and protein (up to 7.25 g/100 g) were noticed in the fortified milk formulations (NCP2–NCP5) compared to control (skimmed milk). Similar results were reported by Wilbanks et al. (36).
3.2.2 Mineral content
Main minerals such as calcium (Ca), phosphorus (P), and sulfur (S) of SM and NCP were assessed, as illustrated in Table 3. Results revealed that the three mineral contents are significantly increased in NCP than in SM. Casein was reported to be an important source of essential amino acids, phosphate, and calcium, to help in increased calcium absorption and bone mineral density, to inhibit muscle protein breakdown, and to contribute to moderately prolonged muscle protein synthesis (37, 38). Sulfur-containing amino acids are the first limiting amino acid in various dietary proteins including casein (39), which supports the obtained results of amino acid content (Table 3). Methionine, which is the main source of sulfur content (40), was increased from 0.71 mg/g in SM to 0.89 mg/g in NCP (Table 3). This was reflected in a significant increase in sulfur content from 304.78 ppm in SM to 313.77 ppm in NCP. These results agreed with (38) and support the nutritional role of NCP fortification targeting higher nutritional value functional foods products.
3.2.3 Amino acid profile
To compare the chemical composition and nutritional value of SM and NCP3 (was chosen as a medium concentration), their amino acids profile and amino acid scores % were evaluated, as represented in Table 4. Results revealed that all amino acids showed to be more concentrated in NCP, with the first limiting amino acids, histidine (0.72, 0.89 mg/g) and methionine (0.71, 0.89 mg/g) in SM and NCP, respectively. Furthermore, the content of hydrophobic amino acids (HAAs), phenylalanine, leucine, isoleucine, tyrosine, valine, methionine, and proline, are 1/3rd proportion of the total amino acid content (33.82, 33.53%) in SM and NCP, respectively. Hydrophobic amino acids are an important cause of aggregation, which was not detected in the nano casein–pectin (NCP) complex formulations. It is worth noting that the essential amino acid EAA content of NCP was 12.26 g/100 g relatively higher than the content in SM (9.55 g/100 g), which can be applied as a high-quality protein dietary supplement for human consumption. Amino acids scores reflected the high quality of protein with a good balance of essential amino acids that achieve protein adequacy in adults, especially the NCP content of leucine (AAS/ 100 g) that can cover 18.07% of daily requirements (41, 44). The obtained results are in agreement with (45).
3.3 Physical properties of NCP complex fortified milk
The physical properties of NCP complex fortified milk with three fortification percentages (1, 2, and 3%) are exhibited in Table 5. Rheological parameters of NCP at room temperature reflected the effect of different nano-casein and pectin concentrations of NCP complex fortified milk formulations on viscosity. The results indicated that the concentrations of nano-casein and pectin are directly proportional to the viscosity values. A similar observation was previously reported (36). Milk casein is able to immobilize water per protein and shows voluminosity; however, a tendency towards more viscous gels for products with increased protein content on a casein basis was observed (46). Casein greatly contributes to micellar stabilization that may allow controlling acid gelation; thus, products fortified with casein may lead to dairy gels with innovative physicochemical and textural properties (47). Additionally, the presence of pectin may reduce intermolecular distances and enhance intermolecular interactions such as hydrogen bonding (11).
3.4 Viscosity monitoring during cold storage
Figure 5 illustrates viscosity monitoring of the five NCP complex formulations through 60 days of cold storage at 4°C. The viscosity values started on the 1st day of preparation at 8, 13, 19, 21, and 22 mPs for NCP1, NCP2, NCP3, NCP4, and NCP5, respectively. During storage, the values tended to increase gradually to end on the 60th day with 11, 23.3, 47.3, 53, 62. mP.s for NCP1, NCP2, NCP3, NCP4, and NCP5, respectively. The results indicated that the viscosity increased by time and by increasing the concentration of nano-casien concentration. This phenomenon may be explained by increasing concentration of pectins that may reduce intermolecular distances and enhance intermolecular interactions such as hydrogen bonding. On the other hand, at low temperatures during storage, the kinetic energy of molecules decreases; thus, intermolecular distances also decrease causing viscosity to increase (5). Consequently; the enhanced viscosity alongside the enhanced LAB viable counts (Figure 6) could contribute in improving the elasticity and viscosity of the yoghurt curd during coagulation that was reflected on decreased syneresis (Table 6) and the sensorial evaluation of yoghurt products (Figure 7) to be described with thickness, creaminess, and softness. These results came in agreement with Wang et al. (48).
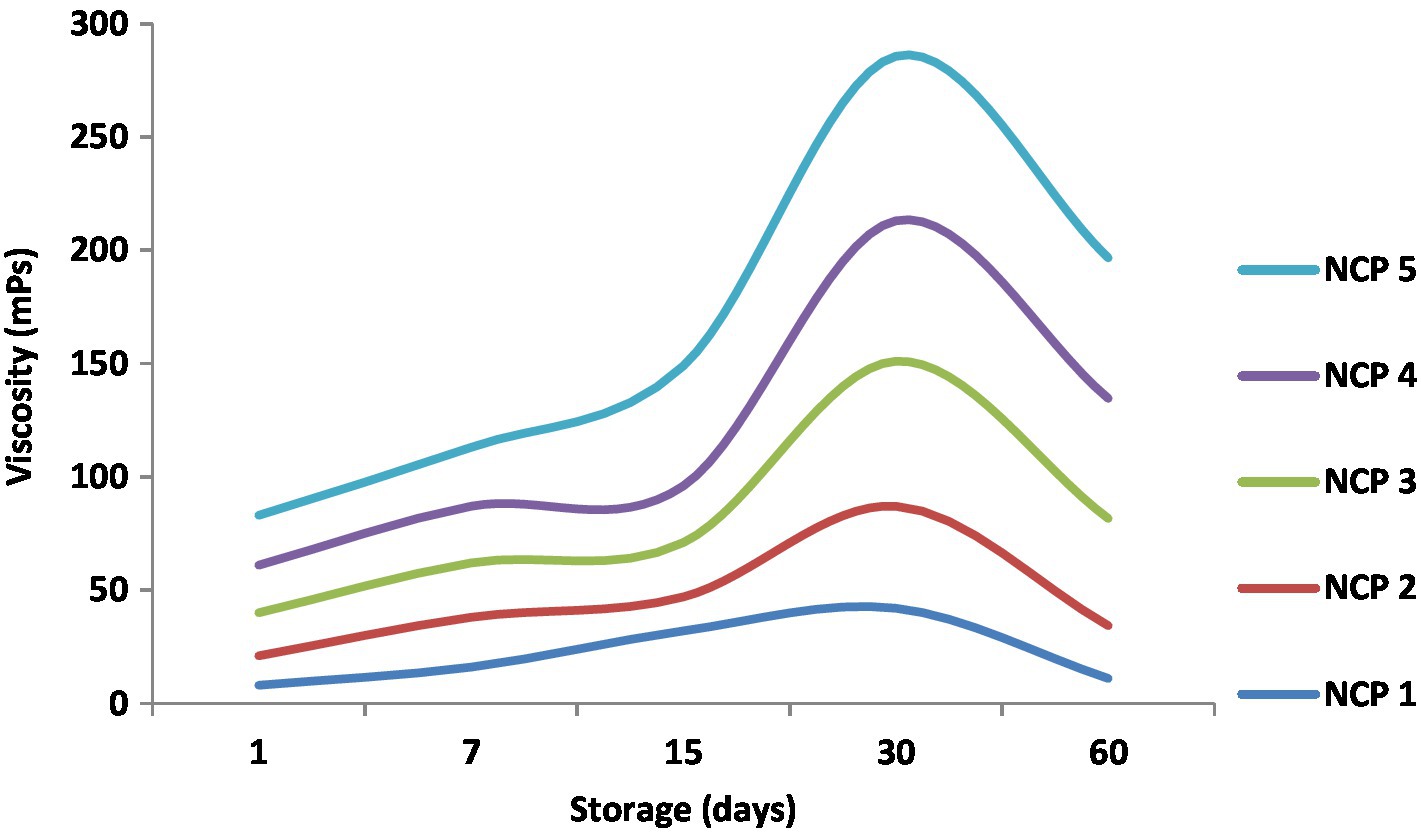
Figure 5. Viscosity of NCP complex formulations through 60 days of cold storage. NCP1, NCP2, NCP3, NCP4, NCP5: nano-casein: pectin (1:1, 2:1, 3:1, 4:1, 5:1), respectively.
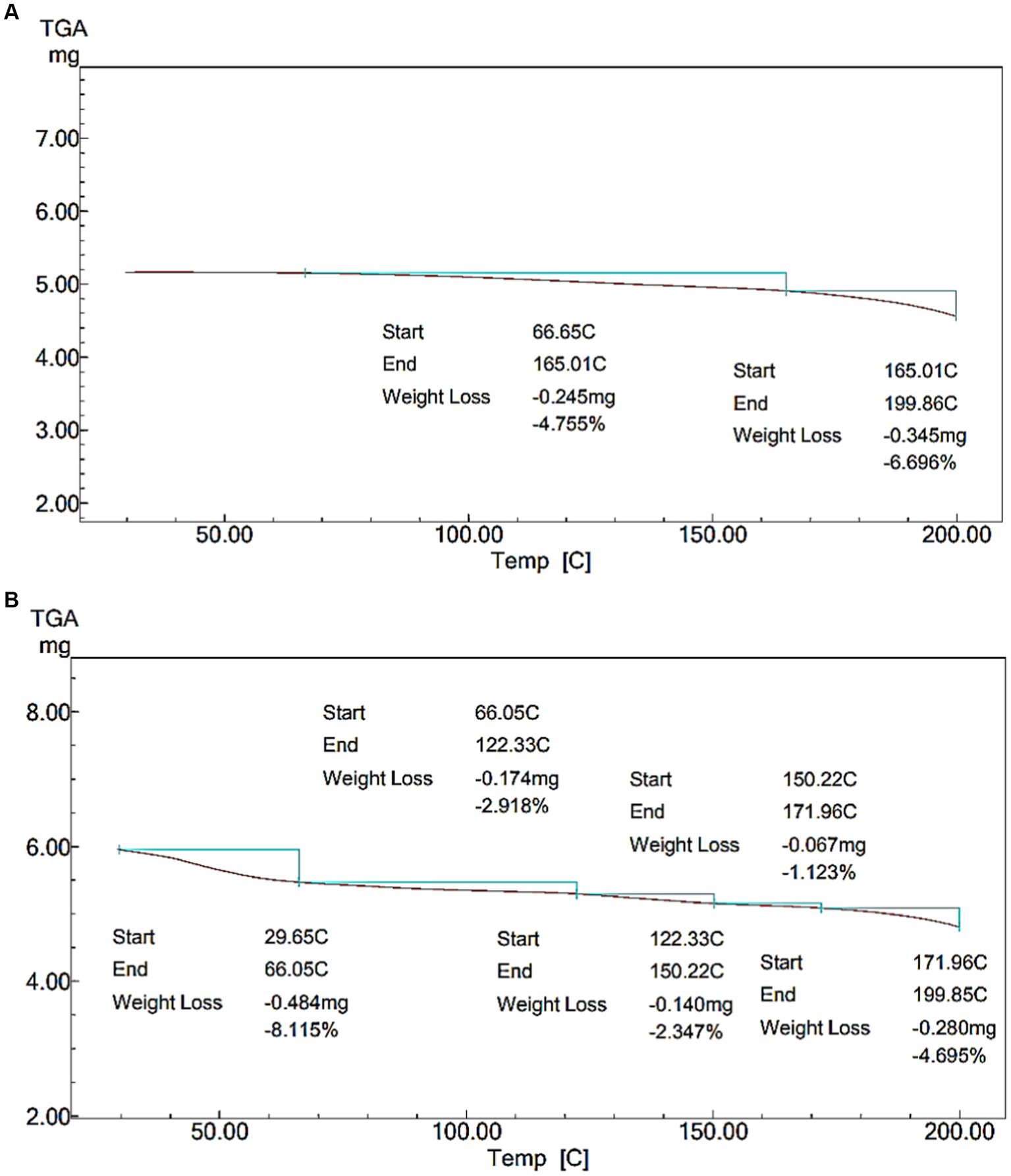
Figure 6. Thermal stability of NCP complex. (A) Thermal stability of skimmed milk. (B) Thermal stability of NCP3 complex.
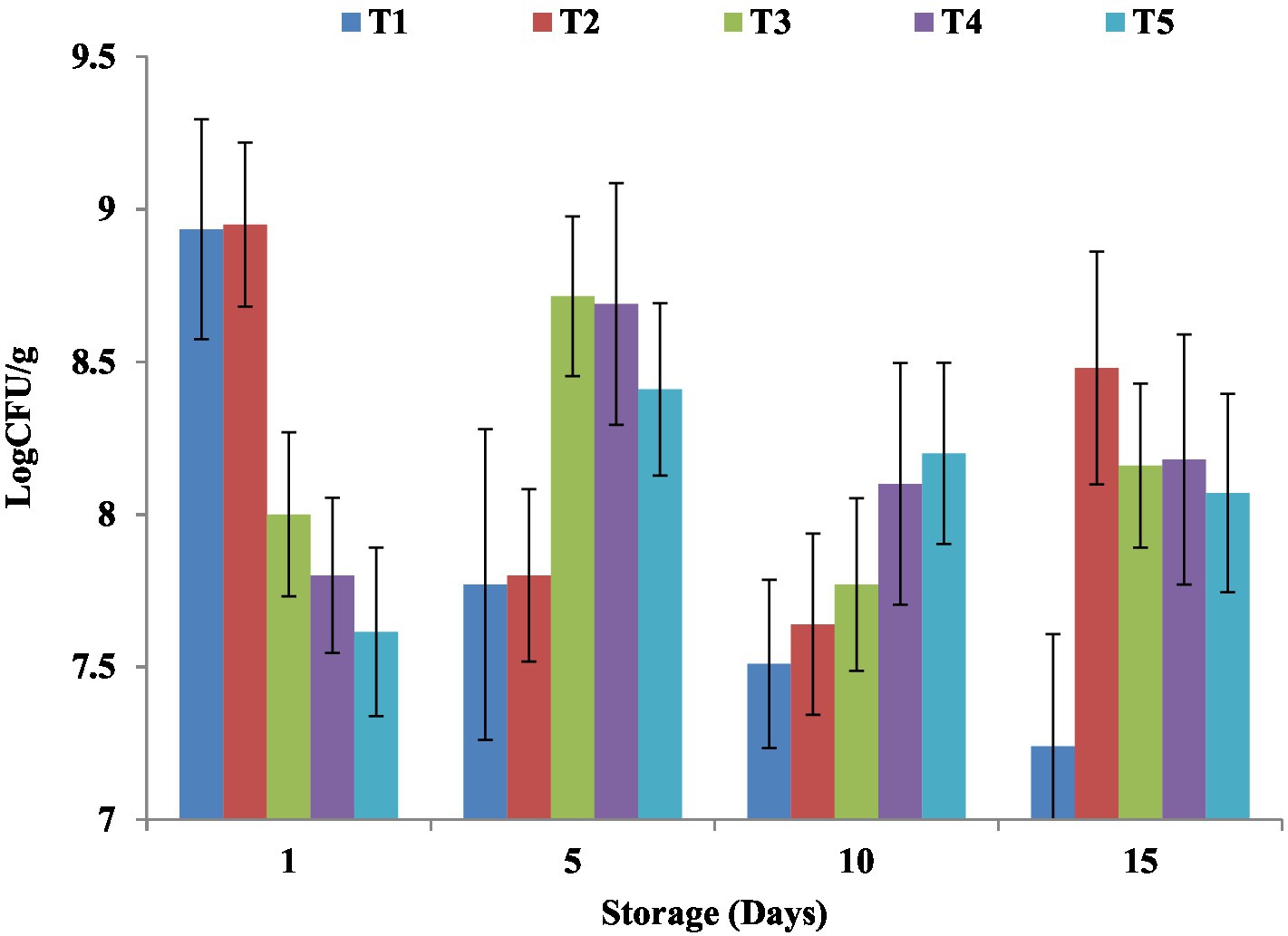
Figure 7. Impact of NCP complex fortification on LAB in yoghurt during cold storage. Results are means ± SD. T1, control plain yoghurt; T2, skimmed milk fortified with NCP2 complex; T3, skimmed milk fortified with NCP3 complex; T4, skimmed milk fortified with NCP4 complex; T5, skimmed milk fortified with NCP5 complex.
3.5 Thermal stability
Due to the significance of thermal stability in dairy applications, the thermal stability of the NCP3 complex was assessed. TGA curves show sample weight loss as a function of temperature. Figures 6A,B shows the thermal degradation of the NCP complex compared to skimmed milk (SM). Figure 6A shows that the weight loss of SM which attributed to the evaporation of the free water was (~ 4%) at (~66–165°C), followed by another stage of weight loss (~165–199°C) corresponding to 6% of weight loss. On the other hand, Figure 6B shows that the weight loss of the NCP3 complex (~ 8%) at (~29–66°C) was attributed to the evaporation of the free water (24). Followed by weight loss occurred in four stages (~66–122°C, ~122–150°C, ~150–172°C, and ~ 171–199°C) corresponding to a total of 10% weight loss which ranged from (2–4%) in each stage, the thermal stability of NCP3 was complex. These losses were attributed to the degradation of the polysaccharides into low-molecular-weight volatile compounds (49). The well thermal stability showed by the NCP3 complex (up to 200°C) can recommend the applicability of NCP complex formulations in high-temperature dairy processing.
3.6 Impact of NCP complex fortification on yoghurt properties
3.6.1 Physicochemical characteristics
The impact of the NCP complex on the chemical composition of fortified yoghurt is represented in Table 6. Results indicated that the NCP increased protein content significantly increased the total solids content without any significant effect on fat content. These results reflect the high-protein content of NCP, especially NCP3, NCP4, and NCP5 as illustrated in Table 2. Caseins as milk protein have high nutritional value compared to other proteins due to their high content of essential amino acids (Table 4), good digestibility, and low-lactose which could offer a good alternative to high-protein–low lactose for lactose intolerance cases (50).
Fat reduction in yogurt (0.4–0.5 g/100 g) (Table 6) has commonly been associated with poor texture. In addition to the role of casein in texture enhancement, the pectin in nano-casein–Pectin (NCP) fortification as a stabilizer achieved two basic functions, increased the water binding capacity, and increased hydrophilic properties of casein (51), which reflected in increased yoghurt viscosity and reduced a syneresis (Table 7).
The effect of the NCP complex on physical properties of fortified yoghurt during 15 days of cold storage was monitored and exhibited in Table 7. Depending upon the composition of NCP, fortified yoghurt samples showed significant differences in rheological properties. The viscosity of T1 plain yoghurt and T2 fortified with NCP2 started from 28.6 mP.s, while it started from 46.6, 43.2, and 42.00 mP.s in T3, T4, and T5, respectively, which reflect that the viscosity was directly proportional with the increase in casein content. These increases may be related to the formation of aggregates of casein in the continuous phase. Similar observations were earlier reported (52). Syneresis and apparent viscosity are important physical properties that can determine the quality of yogurt because these characteristics can limit the shelf-life and acceptability of products (53). The syneresis showed to be significantly affected by viscosity (Figure 5) in an inverse manner as the least syneresis was observed in T3, T4, and T5 (18.00, 18.20, and 16.00% respectively). Syneresis (Whey separation) is a textural defect in yoghurt. Rearrangement of the network of casein micelles is the main cause of syneresis due to shrinkage of gel, which forces water to be expelled from the network. Increasing the protein content increases gel firmness and decreases syneresis. The formation of the coagulum and hence the viscosity of the product is directly proportional to the level of protein present, especially casein which is more effective in this regard (46). Furthermore, the fermentation rate results in lower viscosity. The acidification caused by LAB-producing lactic acid is an important mechanism for the process of yogurt production, which leads to a decrease in pH and thin, milk proteins aggregate to form yogurt gel (54). No significant effect was recorded concerning the acidity between the treatments except for insignificant increases, starting from the 10th day of cold storage in all treatments. This may be related to the resistance in pH change due to high-protein concentration, which agrees with the results of another study (1).
3.6.2 Impact on microbial quality and LAB viability
Lactic acid bacteria (LAB) viable counts of NCP complex fortified yogurt products during cold storage are exhibited in Figure 7. To receive health benefits associated with probiotics, LAB viability is mandatory. The results showed that on the 5th day of cold storage, NCP fortification enhanced the total LAB viable counts in T3, T4, and T5 (8.71, 8.69, and 8.20 Log CFU/ g) compared to the T1 and T2 (7.77, 7.8 Log CFU/ g) and along the cold storage period up to the 15th day of storage. Similar observations were recorded in a previous study (1). A higher lactic acid content in high-protein yogurt was reported to be in a range between 1.5 and 1.8% as the availability of high protein, amino acids, and peptides might alter the lactic acid-forming ability of the yoghurts (2). Increased Ca2+ concentration (Table 3) was reported to improve the activity of LAB during fermentation (55). Furthermore, the synergetic effect of pectin as oligosaccharide was revealed as a prebiotic which was reported to serve as a nutritional supplement for probiotic microorganisms that consequently enhance their survival chances (51, 56). Nevertheless, for all the products maintained viable for counts higher than 106 CFU/ g until the 15th day of storage, the optimum viable count had therapeutic merit (57). The viability of probiotics was reported to be affected by many factors such as storage time, oxygen content, temperature, low pH, and high concentration of salutes (58). No mold, yeast, or coliform growth was detected in all treatments till the 15th day of storage, which may be due to the aseptic condition followed through the preparation process.
3.6.3 Sensory evaluation
The effects of NCP complex fortification on the sensory properties of yoghurt treatments compared to the control plain yoghurt throughout 15 days of cold storage are illustrated in Figures 8A–D. Results showed that the most preferable treatments were T4 and T3 fortified with NCP4 and NCP3. On the other hand, T5 taste was described as cheesy compared to the plain control yoghurt T1. The texture and consistency of the fortified yoghurt treatments T4 and T3 was described as thick, compact, heterogeneous, and soft compared to the plain control yoghurt.
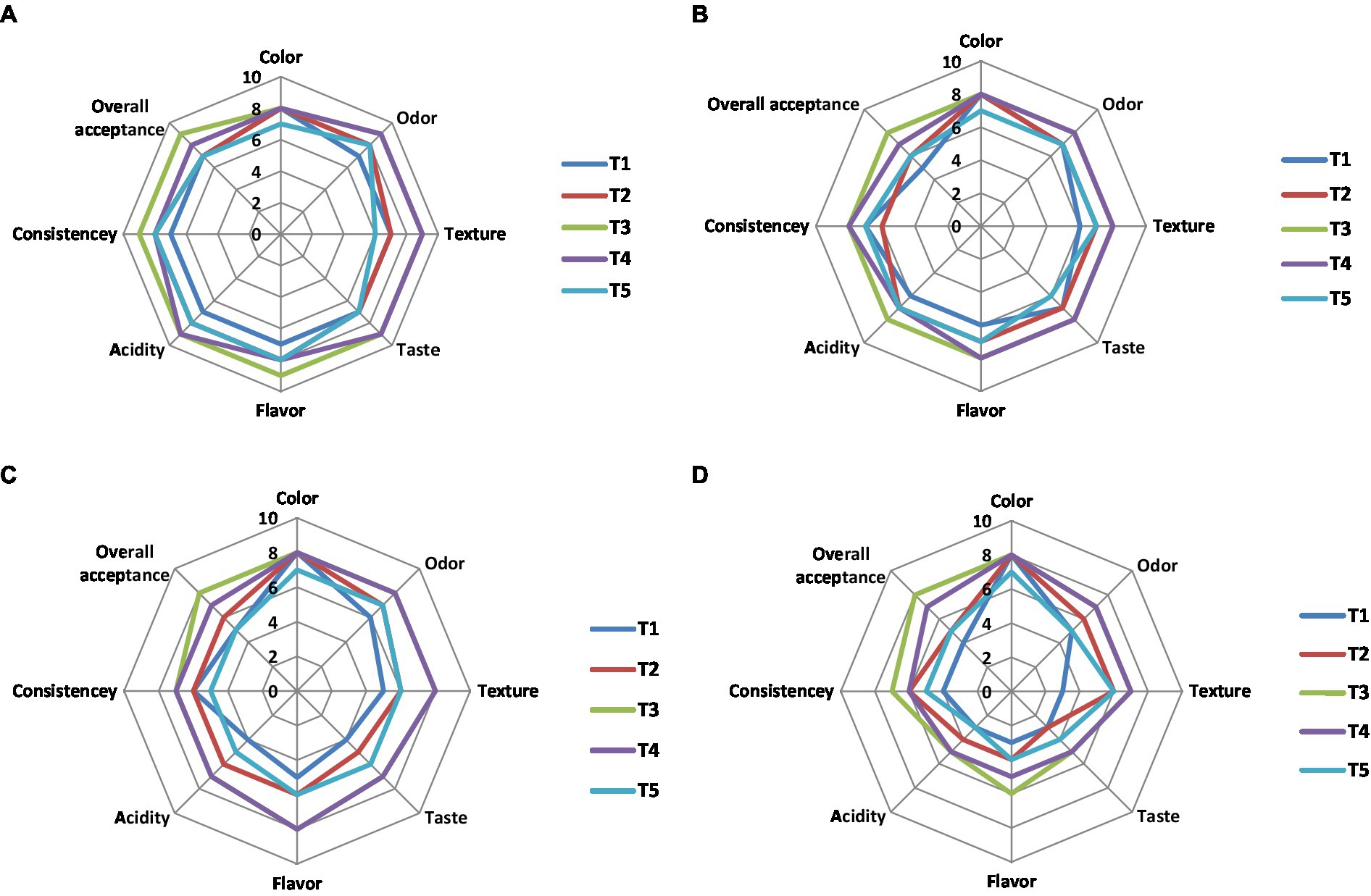
Figure 8. Sensory evaluation of NCP complex fortified yoghurt during storage. (A) 1st day; (B) 5th day; (C) 10th day; (D) 15th day of cold storage (4°C). Results are means of (10 n). T1, control plain yoghurt; T2, skimmed milk fortified with NCP2 complex; T3, skimmed milk fortified with NCP3 complex; T4, skimmed milk fortified with NCP4 complex; T5, skimmed milk fortified with NCP5 complex.
Concerning flavor, odor, and acidity, the treatments T4 and T3 tended to be creamy and buttery, while the acidic flavor of the plain control yoghurt affected the panelist evaluation negatively. The results showed that the organoleptic properties of the fortified yoghurt treatments are connected to the increased protein content (Table 6) and viscosity (Table 7) of these products. The obtained results came in consistency with another study (59), which also stated that attributes, such as thickness, creaminess, and softness, are important drivers of liking high-protein yoghurt products. All sensory parameters of all treatments including control T1 were declined on the 15th day of storage (Figure 8D), that encourage recommending the consumption of the products best before the 10th day of their cold storage.
4 Conclusion
The present study succeeded in the production of high-protein-fortified yoghurt using nanoparticles of casein–pectin (NCP) complex as an active colloidal system. Milk fortification with NCP significantly increased the nutritional value by increasing protein, minerals (Ca, P, and S), and essential amino acids content, with low content of hydrophobic amino acids (HAAs) that may cause aggregation. Enhanced physicochemical properties were revealed in enhanced viscosity and heat stability compared to control skimmed milk (SM). NCP yoghurt fortification significantly increased protein content, enhanced viscosity, and hence decreased syneresis. Higher amino acids, calcium, and oligosaccharides content of NCP were reflected on microbial counts that showed enhanced LAB viability. All above properties were announced on the panelists’ acceptability to show preferable sensory properties. Obtained results encourage recommending NPC fortification in milk, fermented products, and other functional food applications. Future research should explore the potential of designing, producing, and developing more efficient nanosystems products to increase safety, quality, and consequently consumer acceptance of nanoscale formulations in functional foods industries, stating the required regulations to go from lab to large-scale commercial applications.
Data availability statement
The original contributions presented in the study are included in the article/supplementary material, further inquiries can be directed to the corresponding authors.
Author contributions
MG: Conceptualization, Data curation, Investigation, Methodology, Resources, Supervision, Visualization, Writing – original draft, Writing – review & editing. MA: Conceptualization, Formal analysis, Methodology, Supervision, Visualization, Writing – original draft. EM: Data curation, Formal analysis, Methodology, Writing – original draft. EA: Supervision, Visualization, Writing – original draft. SD: Supervision, Writing – original draft. AD: Conceptualization, Data curation, Methodology, Resources, Visualization, Writing – original draft, Writing – review & editing.
Funding
The author(s) declare that no financial support was received for the research, authorship, and/or publication of this article.
Conflict of interest
The authors declare that the research was conducted in the absence of any commercial or financial relationships that could be construed as a potential conflict of interest.
Publisher’s note
All claims expressed in this article are solely those of the authors and do not necessarily represent those of their affiliated organizations, or those of the publisher, the editors and the reviewers. Any product that may be evaluated in this article, or claim that may be made by its manufacturer, is not guaranteed or endorsed by the publisher.
References
1. Gomaa, MAE, Allam, MG, Haridi, AAIM, Eliwa, AEM, and Darwish, AMG. High-protein concentrated pro-yogurt (pro-WPI) enriched with whey protein isolate improved athletic Anemia and performance in a placebo-controlled study. Front Nutr. (2022) 8:1–10. doi: 10.3389/fnut.2021.788446
2. Naibaho, J, Jonuzi, E, Butula, N, Korzeniowska, M, Föste, M, Sinamo, KN, et al. Fortification of milk-based yogurt with protein hydrolysates from brewers’ spent grain: evaluation on microstructural properties, lactic acid bacteria profile, lactic acid forming capability and its physical behavior. Curr Res Food Sci. (2022) 5:1955–64. doi: 10.1016/j.crfs.2022.10.016
3. Aita, O, Husein, Y, Fayed, A, and El-Nawawy, M. Quality attributes of protein fortified yoghurt. J Food Dairy Sci. (2015) 6:227–41. doi: 10.21608/jfds.2015.48815
4. Zhao, X, Cui, YJ, Bai, SS, Yang, ZJ, Miao-Cai, MS, Aziz, T, et al. Antioxidant activity of novel casein-derived peptides with microbial proteases as characterized via Keap1-Nrf2 pathway in HepG2 cells. J Microbiol Biotechnol. (2021) 31:1163–74. doi: 10.4014/jmb.2104.04013
5. Gawkowska, D, Cybulska, J, and Zdunek, A. Structure-related gelling of pectins and linking with other natural compounds: a review. Polymers (Basel). (2018) 10:762. doi: 10.3390/polym10070762
6. Wang, Y, and Wusigale, LY. Colloidal nanoparticles prepared from zein and casein: interactions, characterizations and emerging food applications. Food Sci Human Wellness. (2023) 12:337–50. doi: 10.1016/j.fshw.2022.07.036
7. Wagoner, TB, and Foegeding, EA. Whey protein–pectin soluble complexes for beverage applications. Food Hydrocoll. (2017) 63:130–8. doi: 10.1016/j.foodhyd.2016.08.027
8. Du, Q, Zhou, L, Lyu, F, Liu, J, and Ding, Y. The complex of whey protein and pectin: interactions, functional properties and applications in food colloidal systems – a review. Colloids Surf B Biointerfaces. (2022) 210:112253. doi: 10.1016/j.colsurfb.2021.112253
9. Peng, Y, Serra, M, Horne, DS, and Lucey, JA. Effect of fortification with various types of milk proteins on the rheological properties and permeability of nonfat set yogurt. J Food Sci. (2009) 74:C666–73. doi: 10.1111/j.1750-3841.2009.01350.x
10. Salunke, P, Marella, C, Amamcharla, JK, Muthukumarappan, K, and Metzger, LE. Use of micellar casein concentrate and milk protein concentrate treated with transglutaminase in imitation cheese products—Unmelted texture. J Dairy Sci. (2022) 105:7891–903. doi: 10.3168/jds.2022-21852
11. Bdair, U, Esmail, SM, and Abdel-Whab, ME. The effect of pectin and pectin nanoparticles on some quality properties of meat sausage. Egypt J Agric Res. (2019) 97:627–43. doi: 10.21608/ejar.2019.152561
12. Everett, DW, and McLeod, RE. Interactions of polysaccharide stabilisers with casein aggregates in stirred skim-milk yoghurt. Int Dairy J. (2005) 15:1175–83. doi: 10.1016/j.idairyj.2004.12.004
13. Farrag, AF, Zahran, H, Al-Okaby, MF, El-Sheikh, MM, and Soliman, TN. Physicochemical properties of white soft cheese supplemented with encapsulated olive phenolic compounds. Egypt J Chem. (2020) 63:8–9. doi: 10.21608/EJCHEM.2020.23381.2388
14. AOAC International In: GW Latimer, editor. Official methods of analysis. 20th ed. Rockville, Maryland: AOAC International (2016)
16. Beaty, RD, and Kerber, JD. Concepts, instrumentation and techniques in atomic absorption spectrophotometry. Norwalk, CT: The Perkin-Elmer Corporation (1993).
18. Smith, AJ. Post column amino acid analysis In: AJ Smith, editor. Methods in molecular biology (Clifton, N.J.). Totowa, NJ: Humana Press Inc. 133–41.
19. Akalın, AS, Unal, G, Dinkci, N, and Hayaloglu, AA. Microstructural, textural, and sensory characteristics of probiotic yogurts fortified with sodium calcium caseinate or whey protein concentrate. J Dairy Sci. (2012) 95:3617–28. doi: 10.3168/jds.2011-5297
20. Cerqueira, MA, Souza, BWS, Simoes, J, Teixeira, JA, Domingues, MRM, Coimbra, MA, et al. Structural and thermal characterization of galactomannans from non-conventional sources. Carbohydr Polym. (2011) 83:179–85. doi: 10.1016/j.carbpol.2010.07.036
21. Tamime, AY, and Robenson, RK. Tamime and Robenson’s yoghurt science and technology. 3rd ed. Cambridge, England: Woodhead Publishing Ltd and CRC Press LLC (2007).
22. Isanga, J, and Zhang, G. Production and evaluation of some physicochemical parameters of peanut milk yoghurt. LWT - Food Sci Technol. (2009) 42:1132–8. doi: 10.1016/j.lwt.2009.01.014
23. Marth, EH. Standard methods for the examination of dairy products. 14th ed. Washington, DC: American Public Health Association (1978).
24. Darwish, AMG, Khalifa, RE, and El Sohaimy, SA. Functional properties of chia seed mucilage supplemented in low fat yoghurt. Alexandria Sci Exch J. (2018) 39:450–9. doi: 10.21608/asejaiqjsae.2018.13882
25. Senaka Ranadheera, C, Evans, CA, Adams, MC, and Baines, SK. Probiotic viability and physico-chemical and sensory properties of plain and stirred fruit yogurts made from goat’s milk. Food Chem. (2012) 135:1411–8. doi: 10.1016/j.foodchem.2012.06.025
26. Ozturk, B, Argin, S, Ozilgen, M, and McClements, DJ. Nanoemulsion delivery systems for oil-soluble vitamins: influence of carrier oil type on lipid digestion and vitamin D3 bioaccessibility. Food Chem. (2015) 187:499–506. doi: 10.1016/j.foodchem.2015.04.065
27. ISO 22935-3. IDF 099–3: 2009 – Milk and milk products – Sensory analysis – Part 3: Guidance on a method for evaluation of compliance with product specifications for sensory properties by scoring. First edit. © ISO and IDF 2009. Geneva: ISO (2009).
29. Kotz, JC, Treichel, P, and Weaver, GC. Chemistry and chemical reactivity. Pacific Grove: Thomson Brooks Cole (2006).
30. Swaisgood, HE. Review and update of casein chemistry. J Dairy Sci. (1993) 76:3054–61. doi: 10.3168/jds.S0022-0302(93)77645-6
31. Jain, K, Mehra, NK, and Jain, NK. Nanotechnology in drug delivery: Safety and toxicity issues. Curr Pharm Des (2015) 21:4252–61. doi: 10.2174/1381612821666150901103208
32. Chen, J, and Hu, L. Nanoscale delivery system for nutraceuticals: preparation, application, characterization, safety, and future trends. Food Eng Rev. (2020) 12:14–31. doi: 10.1007/s12393-019-09208-w
33. Khan, I, Saeed, K, and Khan, I. Nanoparticles: properties, applications and toxicities. Arab J Chem. (2019) 12:908–31. doi: 10.1016/j.arabjc.2017.05.011
34. Karami, K, Jamshidian, N, Hajiaghasi, A, and Amirghofran, Z. BSA nanoparticles as controlled release carriers for isophethalaldoxime palladacycle complex; synthesis, characterization, in vitro evaluation, cytotoxicity and release kinetics analysis. New J Chem. (2020) 44:4394–405. doi: 10.1039/c9nj05847h
35. Vaucher Ac Dos, S, PCM, D, Coimbra, PT, dos SM, CI, Marreto, RN, Dellamora-Ortiz, GM, et al. Microencapsulation of fish oil by casein-pectin complexes and gum arabic microparticles: oxidative stabilisation. J Microencapsul. (2019) 36:459–73. doi: 10.1080/02652048.2019.1646335
36. Wilbanks, DJ, Yazdi, SR, and Lucey, JA. Effects of varying casein and pectin concentrations on the rheology of high-protein cultured milk beverages stored at ambient temperature. J Dairy Sci. (2022) 105:72–82. doi: 10.3168/jds.2021-20597
37. Malekhosseini, P, Alami, M, Khomeiri, M, Esteghlal, S, Nekoei, AR, and Hosseini, SMH. Development of casein-based nanoencapsulation systems for delivery of epigallocatechin gallate and folic acid. Food Sci Nutr. (2019) 7:519–27. doi: 10.1002/fsn3.827
38. Jeong, EW, Park, GR, Kim, J, Baek, Y, Go, GW, and Lee, HG. Whey proteins-fortified Milk with adjusted casein to whey proteins ratio improved muscle strength and endurance exercise capacity without lean mass accretion in rats. Foods. (2022) 11:574. doi: 10.3390/foods11040574
39. Tanaka, K, and Sugano, M. Effect of addition of sulfer-containing amino acids and glycine to soybean protein abd casein on cholesterol levels of rats. J Nutr Sci Vitaminol (Tokyo). (1989) 35:323–32. doi: 10.3177/jnsv.35.323
40. Johns, PW. Determination of Sulfur amino acids in Milk and plant proteins. Food Anal Methods. (2021) 14:108–16. doi: 10.1007/s12161-020-01854-9
41. FAO/WHO/UNU. Energy and protein requirements - FAO/WHO/UNU expert consultation. Food and agriculture Organization of the United States/ World Health Organization/ United Nations University, energy and protein requirements. Report of a joint FAO/WHO/UNU meeting. Geneva: World Health Organization (1985).
43. National Research Council. Recommended dietary allowances - food and nutrition/ board commission on life/ sciences National Research Council. 10th ed. Washington, DC: National Academy Press (1989).
44. Darwish, AMG, Abd El-Wahed, AA, Shehata, MG, El-Seedi, HR, Masry, SHD, Khalifa, SAM, et al. Chemical profiling and nutritional evaluation of bee pollen, bee bread, and Royal Jelly and their role in functional fermented dairy products. Molecules. (2023) 28:227. doi: 10.3390/molecules28010227
45. Du, X, Jing, H, Wang, L, Huang, X, Mo, L, Bai, X, et al. pH-shifting formation of goat milk casein nanoparticles from insoluble peptide aggregates and encapsulation of curcumin for enhanced dispersibility and bioactivity. Lwt. (2022) 154:112753. doi: 10.1016/j.lwt.2021.112753
46. Rani, R, Unnikrishnana, V, Dharaiya, CN, and Singh, B. Factors affecting syneresis in yoghurt: a review. Indian J Dairy Biosci. (2012) 23:1–9.
47. Kern, L, Fabre, O, Scher, J, and Petit, J. Chemical fractionation of caseins by differential precipitation: influence of pH, calcium addition, protein concentration and temperature on the depletion in α- and β-caseins. Int J Food Sci Technol. (2019) 55:542–52. doi: 10.1111/ijfs.14283
48. Wang, Y, Aziz, T, Hu, G, Liu, J, Min, Z, Zhennai, Y, et al. Production, purification, and characterization of a novel milk-clotting metalloproteinase produced by Bacillus velezensis YH-1 isolated from traditional Jiuqu. Lwt. (2023) 184:115080. doi: 10.1016/j.lwt.2023.115080
49. Shehata, MG, Darwish, AMG, and El-Sohaimy, SA. Physicochemical, structural and functional properties of water-soluble polysaccharides extracted from Egyptian agricultural by-products. Ann Agric Sci. (2020) 65:21–7. doi: 10.1016/j.aoas.2020.05.004
50. Sindayikengera, S, and Xia, W. Nutritional evaluation of caseins and whey proteins and their hydrolysates from Protamex. J Zhejiang Univ Sci B. (2006) 7:90–8. doi: 10.1631/jzus.2006.B0090
51. Juniawati, US, and Budiyanto, A. Effect of stabilizer on the physicochemical and microbiology properties of Wheygurt. IOP Conf Ser Earth Environ Sci. 2022, 1024:12074. doi: 10.1088/1755-1315/1024/1/012074
52. Ziaeifar, L, Labbafi Mazrae Shahi, M, Salami, M, and Askari, GR. Effect of casein and inulin addition on physico-chemical characteristics of low fat camel dairy cream. Int J Biol Macromol. (2018) 117:858–62. doi: 10.1016/j.ijbiomac.2018.05.135
53. Rashwan, AK, Osman, AI, and Chen, W. Natural nutraceuticals for enhancing yogurt properties: a review. Environ Chem Lett. (2023) 21:1907–31. doi: 10.1007/s10311-023-01588-0
54. Hoxha, R, Evstatieva, Y, and Nikolova, D. Physicochemical, rheological, and sensory characteristics of yogurt fermented by lactic acid bacteria with probiotic potential and bioprotective properties. Foods. (2023) 12:2552. doi: 10.3390/foods12132552
55. Zhang, J, Cai, D, Yang, M, Hao, Y, Zhu, Y, Chen, Z, et al. Screening of folate-producing lactic acid bacteria and modulatory effects of folate-biofortified yogurt on gut dysbacteriosis of folate-deficient rats. Food Funct. (2020) 11:6308–18. doi: 10.1039/d0fo00480d
56. Sarwar, A, Al-Dalali, S, Aziz, T, Yang, Z, Din, JU, Khan, AA, et al. Effect of chilled storage on antioxidant capacities and volatile Flavors of Synbiotic yogurt made with probiotic yeast saccharomyces boulardii CNCM I-745 in combination with inulin. J Fungi. (2022) 8:713. doi: 10.3390/jof8070713
57. De LLA, D, Salgado, SM, Guerra, NB, AVS, L, SAC, A, and Ximenes Gn Da, C. Phisicochemical, sensory, and microbiological evaluation and development of symbiotic fermented drink. Food. Sci Technol. (2013) 33:805–11. doi: 10.1590/S0101-20612013000400030
58. Sarwar, A, Aziz, T, Al-Dalali, S, Zhao, X, Zhang, J, Ud Din, J, et al. Physicochemical and microbiological properties of synbiotic yogurt made with probiotic yeast saccharomyces boulardii in combination with inulin. Foods. (2019) 8:468. doi: 10.3390/foods8100468
Keywords: milk-protein fortification, casein-pectin nanoparticles (NCP), fermented dairy products, thermal stability, rheological properties
Citation: Gomaa MAE, Allam MG, Mokhtar E, Ayad EHE, Darwish SM and Darwish AMG (2024) Nano casein–pectin complex: exploring physicochemical, organoleptic properties, and LAB viability in skimmed milk and low-fat yoghurt. Front. Nutr. 10:1288202. doi: 10.3389/fnut.2023.1288202
Edited by:
Gaytán-Martínez Marcela, Autonomous University of Queretaro, MexicoReviewed by:
Dimas Rahadian Aji Muhammad, Sebelas Maret University, IndonesiaSwarup Roy, Lovely Professional University, India
Copyright © 2024 Gomaa, Allam, Mokhtar, Ayad, Darwish and Darwish. This is an open-access article distributed under the terms of the Creative Commons Attribution License (CC BY). The use, distribution or reproduction in other forums is permitted, provided the original author(s) and the copyright owner(s) are credited and that the original publication in this journal is cited, in accordance with accepted academic practice. No use, distribution or reproduction is permitted which does not comply with these terms.
*Correspondence: Mohamed A. E. Gomaa, m.gomaa@alexu.edu.eg; Amira M. G. Darwish, amiragdarwish@yahoo.com
†ORCID: Mohamed A. E. Gomaa, https://orcid.org/0000-0001-5777-9041
Marwa G. Allam, https://orcid.org/0000-0002-6653-1213
Esraa Mokhtar, https://orcid.org/0009-0002-9743-3162
Amira M. G. Darwish, https://orcid.org/0000-0003-3586-1575