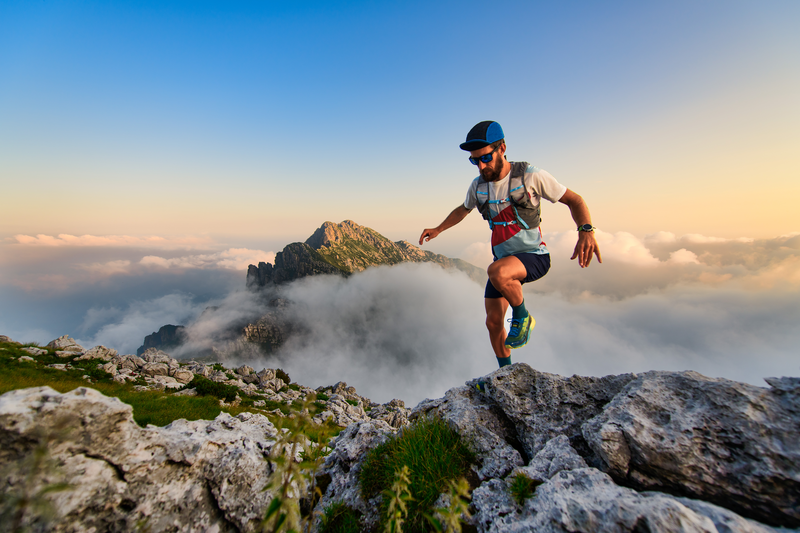
94% of researchers rate our articles as excellent or good
Learn more about the work of our research integrity team to safeguard the quality of each article we publish.
Find out more
REVIEW article
Front. Nutr. , 12 October 2023
Sec. Food Chemistry
Volume 10 - 2023 | https://doi.org/10.3389/fnut.2023.1287405
This article is part of the Research Topic Plant Terpenoids: Synthesis, Dynamics, Isolation, Structure, and Function View all 5 articles
With the continuous technological innovation in the high-value utilization of rice bran byproducts, rice bran oil retains a higher concentration of beneficial components such as a well-balanced composition of fatty acids and abundant phytosterols. This makes it a highly nutritious and healthy vegetable oil. This review provides an overview of the advancements made in separating, purifying, and processing phytosterols in rice bran oil. The review also introduces techniques for assessing the stability of rice bran oil. Moreover, the review emphasizes the nutritional value of phytosterols found in rice bran oil, highlighting their various health benefits, including their anticancer, anti-inflammatory, anti-allergic, antibacterial, cholesterol-lowering, skin-protective, anti-obesity, anti-diabetic, neuroprotective, gastroprotective, and immune-enhancing effects. Attaining a comprehensive understanding of the research progress made in phytosterols derived from rice bran oil can offer valuable guidance for the efficient utilization of rice bran.
Rice bran is the part of rice removed during the process of dehusking and milling, which transforms rough rice into polished rice (1, 2). It refers to the brownish outer layer of rice grains, including the outer husk, bran layer, aleurone layer, seed coat, and endosperm (3). It is also known as “rice husk” or “rice bran,” and typically constitutes about 8% of the total weight of the rice grain (4). As a byproduct of the rice milling process, rice bran often contains rice germ and broken rice. Furthermore, the composition of rice bran can be significantly influenced by different rice varieties and the precision of polished rice (5). Generally, regular rice bran contains around 34 to 62% carbohydrates, 11 to 15% protein, 15 to 20% fat, 7 to 11% dietary fiber, and 8 to 12% ash (6). Additionally, several bioactive compounds are found, such as tocopherols, tocotrienols, phenolic acids, flavonoids, oryzanol, octacosanol, α-lipoic acid, squalene, and ceramides, among others (7).
During the processing of brown rice, the mechanical actions disrupt the cellular structure of the rice bran layer, causing lipases from the endosperm to disperse into the rice bran (8). When exposed to the oil in the bran, hydrolysis reactions take place, leading to the rapid hydrolytic rancidity of rice bran, accompanied by producing a pungent odor that affects its development and utilization (9). Research has shown that rice bran requires stabilization techniques within 6 h to deactivate the lipases, in order to achieve its effective utilization and storage. Currently, stabilization techniques for rice bran are adopted widely, including dry heat treatment, low-temperature storage, microwave heating inactivation, chemical stabilization, ohmic dielectric heating, radiation treatment, extrusion puffing, enzymatic treatment, among others (10). Among these methods, dry heat treatment is the most commonly used for enzyme deactivation, although it may also affect activities of rice bran proteins (11). Nutrient loss can be easily caused by the extrusion puffing method, while chemical stabilization may result in the retention of chemical residues, so this method is less commonly employed (12).
Rice bran has an oil content ranging from 12 to 24%, and based on its lipid content, it can be categorized into three types: full-fat rice bran, low-fat rice bran, and defatted rice bran (13). Full-fat rice bran is commonly referred to as rice bran, which has not undergone any oil extraction process. It retains its natural oil content. Low-fat rice bran is the type of rice bran obtained after extracting rice bran oil, and it typically contains around 7% of oil. Defatted rice bran, also known as rice bran meal, is the residue left after extracting rice bran oil using organic solvents (14). It contains less than 1% of oil. Moreover, in actual production, rice bran can be further classified into “rough rice bran” and “polished rice bran” based on the number of times of rice milling processes and different techniques employed (15). Rough rice bran, with fewer milling processes, has a higher lipid content, making it suitable for oil extraction purposes. On the other hand, polished rice bran has lower lipid content but is rich in protein, making it suitable for the development of nutritious food products (16).
In order to preserve and retain the various bioactive compounds abundant in rice bran and avoid their destruction, the processing techniques of rice bran oil have been widely researched (17). These techniques include mechanical pressing, solvent extraction, supercritical CO2 extraction, membrane separation technology, and more. Rice bran oil has a balanced fatty acid composition, containing approximately 22% saturated fatty acids, 41% monounsaturated fatty acids, and 37% polyunsaturated fatty acids (18). The main components of these fatty acids are palmitic acid, oleic acid, and linoleic acid, respectively, with their proportions closely matching the dietary fatty acid recommendations of the World Health Organization (WHO) at 1:1.5:1 (19). Additionally, rice bran oil contains high levels of phytosterols, tocopherols, and tocotrienols, with concentrations reaching up to 10,500 mg/kg, 15,300 mg/kg, and 205.7 mg/kg, respectively, far surpassing those found in other plant oils. As a result, rice bran oil has been utilized in the development of functional blended oils, frying oils, and other products (20).
In addition to being used for oil extraction, rice bran has further expanded its applications in the food, health supplement, and pharmaceutical industries by extracting high-nutrition and high-economic-value products from the defatted byproduct, rice bran meal (21). These extracted products include polysaccharides, dietary fiber, proteins, phytic acid, inositol, and other valuable components. Numerous studies have demonstrated that rice bran extracts possess various nutritional and health benefits (22). For instance, rice bran polysaccharides exhibit anti-tumor, immune-enhancing, and blood glucose-lowering effects. Rice bran dietary fiber, being the highest polysaccharide content, can be added to various food products such as pasta and baked goods to improve their texture and nutritional value. Rice bran protein contains all essential amino acids and is easily digestible and absorbed by the human body. Further hydrolysis of rice bran protein yields biologically active peptides with rich nutritional properties (23). Research has shown that these peptides have effects such as lowering blood pressure, reducing cholesterol levels, anti-cancer properties, antimicrobial activities, and alleviating diabetes symptoms (24).
Overall, the existing research has provided a comprehensive understanding of rice bran dietary fiber, protein, and oil. There have also been comprehensive summarizations of rice bran oil-derived compounds such as oryzanol, tocopherols, and tocotrienols, which are present in significant quantities. However, compared to other plant oils, the remarkable benefits of plant sterols found in rice bran have received less attention in the literature. This review focuses on rice bran, a byproduct of rice processing, and review the current research and application status of the retained nutrients and accompanying compounds, particularly plant sterols, in the deep processing of rice bran oil.
Phytosterols, generally known as plant steroids, have similar structural and biological functions to cholesterol (25). With regard to health-promoting effects on human beings, it has been claimed from several scientific literatures that phytosterol presented remarkable anti-oxidant, anti-polymerization, anti-inflammatory, anti-tumor and other biological activities. At present, it is reported that more than 200 different types of phytosterols have been identified in a wild range of plant species with trace levels (26, 27). The chemical structures of identified phytosterols compounds from rice bran are displayed in Figure 1. Naturally, the basic skeleton of phytosterols is cyclopentane polyhydrophenanthrene with a hydroxyl group at C-3 position and an 8 to 10 carbon atoms side chain at C-17 position, which could significantly influence the physiochemical properties and biological properties of phytosterols. With regard to the number of methyl groups at the C-4 position, phytosterols can be divided into three categories: 4-demethyl sterols, 4a-monomethyl sterols, and lophenol; 4,4-dimethyl sterols. Given their inherent molecular structure, the main differences are that phytosterols usually have a methyl or ethyl group at the C-24 position, and some common phytosterols have an unsaturated double bond at the C-22 position. Moreover, the poor oil-solubility and water-solubility are contributed to the hydroxyl and hydrocarbon side chains at C-3 and C-17 (28). However, humans cannot synthesis phytosterols, all phytosterols in human blood and tissues are derived from diet (29). Due to their diverse physiological effects, phytosterols have great potential to use in functional foods, supplements, and pharmaceutical products designed to enhance human health. Therefore, the development and utilization of the phytosterols from rice bran is necessary.
The main traditional methods for phytosterols extraction from rice bran are cold-pressure extraction (CPE) (30) and solvent extraction (SE) (31). Nevertheless, traditional methods exhibit shortcomings in terms of time consumption, energy inefficiency, limited economic viability, and environmental contamination (32). Thus, researchers have reported novel techniques for extracting phytosterols, such as ultrasonication assisted extraction (33), supercritical fluid extraction (SFE) (34), subcritical extraction (SUBE) (35), and ohmic heating-aided mechanical extraction (36). The yields of γ-glutamin from rice bran obtained by SE, SFE, and SUBE and the concentrations in the extracts are presented in Table 1.
Supercritical fluid is any substance at a temperature and pressure above its critical point, where distinct liquid and gas phases do not exist (44). SFE often uses CO2 and water as solvents. In the study of Imsanguan et al., three methods (SC-CO2, SE, and Soxhlet extraction) were compared for the γ-oryzanol extraction and found that the maximum extraction rate of 11371.79 mg/kg of dry rice bran was achieved by SC-CO2 (37). Understanding the solubility of γ-oryzanol in SC-CO2 is the basis for optimization of the extraction conditions for SC-CO2. Bitencourt et al. found that the process parameters, such as CO2 flow rate, pressure and temperature, plays an important role in SC-CO2 extraction. Under the optimal conditions, the solubility of γ-oryzanol at 60°C/400 bar was close to 1 g/kg in CO2 (45). For instance, γ-oryzanol was reported to have a high recovery (39% (w/w)) using SC-CO2 at 30 MPa, 303 K, 270 min and 10 g CO2/min (41). Considering the techno-economy of SC-CO2, Kayathi et al. realized SC-CO2 extraction of γ-oryzanol from rice bran with low pressure operation (23.9 Mpa), which yielded up to 36,000 mg/kg of dry rice bran (38). In another study conducted by Chen et al., using SC-CO2 to extract rice bran oil from rice bran flour, followed by the concentration and isolation of γ-oryzanol by column partition purification (N-hexane with ethyl acetate in volume ratios of 90:10) can get high purity γ-oryzanol (39).
Subcritical liquified dimethyl ether (SUBLDME) extraction will consume less energy than SC-CO2, while also reducing greenhouse gas emissions. Compared to SC-CO2, SUBLDME yielded more γ-oryzanol (4865.25 mg/100 g of rice bran) (42). SUBLDME often uses propane, butane, dimethyl ether and water as solvents. Among them, dimethyl ether has a low boiling point (−24.8°C) and is not easy to remain in the extract. Dimethyl ether has been authorized by the European Food Safety Authority (EFSA) as a safe extraction solvent for the production of foodstuffs and food ingredients (43). Moreover, there is considerable experimental data suggesting that subcritical butane- and propane-extracted rice bran oil had high oxidative stability (46). Therefore, the use of subcritical solvents to create added value to agricultural wastes through the extraction of high-value food products is one of the strategies to reduce CO2 greenhouse gas emissions.
It is noteworthy that trace components may remain in the γ-oryzanol extracted from rice bran, such as cycloartenyl ferulate (CAFA) and campesteryl ferulate. Therefore, further isolation and purification of the extracted γ-oryzanol is also required. Currently, the commonly used separation and purification methods include countercurrent chromatography (CCC) (47) and normal-phase preparative scale high performance liquid chromatography (HPLC) (48). Recent evidence suggests that γ-oryzanol is separated by the preparative recycle HPLC system using a combination of octadecylsilane (ODS) silica and the purity of γ-oryzanol exceeds 99% (49). In addition, Sawada et al. used high performance liquid chromatography-ultraviolet-mass spectrometry (HPLC-UV-MS) and nuclear magnetic resonance (NMR) for the separation and purification of the isomerized substance of γ-oryzanol (50). The extraction process of phytosterols from rice bran are summarized in Figure 2.
Generally, the processing of phytosterols extraction starts from stabilization of rice bran. And then the stabilized rice bran was extracted by a number of extraction and separation methods. Rice bran is composed of numerous bioactive compounds, such as tocopherols, γ-oryzanol, anthocyanins, and flavonoids, which mainly contributed to the stability of rice bran. Among them, tocopherols could protect cell membranes, increase stability, and inhibit lipid peroxidation by acting as a trap for lipid peroxyl radicals. But tocopherols are highly susceptible to degradation at high temperatures. A strong relationship between combined bioactive and antioxidant capacity has been reported in the literature (51). They identify that high activity against free radicals and strong antioxidant capacity of tocopherols at lower concentration (0.04%). γ-oryzanol is second only to tocopherols in antioxidant power. When the ratio of rice bran to corn oil was 1:5 and the heating temperature was 180°C, the 2,2-diphenyl-1-picrylhydrazyl (DPPH) loss of corn oil decreased slowly, the p-anisidine value and CDA of corn oil increased the slowest, and corn oil stability was significantly improved during storage after recovery. Furthermore, BoRa Yi et al. suggested that the probable cause was the transfer of some of the γ-oryzanol from rice bran to corn oil (52). It is worth noting that a large number of studies have focused on the concentration of γ-oryzanol and tocopherols. However, the synergistic effect of antioxidant capacity depends not only on the concentration but also on the ratio. It was shown that the formation of FFA and secondary oxidation products was significantly inhibited while the optimal ratio of γ-oryzanol and tocopherols was 8:1, with the oxidative stability index of rice bran oil reached 10.26 h (53). Similarly, γ-oryzanol has a certain inhibitory effect on the degradation of tocopherols (54). When the concentration of tocopherols in rice bran oil was 500 mg/kg, higher concentrations of γ-oryzanol did not inhibit the degradation of tocopherols. In contrast, the higher concentration of γ-oryzanol, the more pronounced, respectively, was the inhibition of tocopherols degradation.
As a result, various methods of stabilizing rice bran have emerged, such as heat treatment, extrusion, γ-irradiation, refrigeration and acidification. Among them, the commonly used heat treatment methods mainly include dry-heating (DH), microwave heating (MH), ethanol vapor (EV), ohmic heating, and so on. Kim et al. (11) compared the storage stability of rice bran after DH, FDDH, MH, AC, and EV treatments. It was found that the FFA content of the heat-treated rice bran did not change significantly compared to that of the untreated group (FFA content gradually increased from 2.14 to 19.81%) during the storage period (24 weeks), and all of them were effective in stabilizing the oxidative rancidity of the rice bran, with DH being the most effective in delaying the formation of FFA. In addition, Bruscatto et al. (55) placed rice bran oil at 100°C and 180°C to determine the changes in its α-, (γ + β)-, and δ-tocopherol content with time, and the results of the experiment confirmed that the degradation of tocopherols increased significantly with increasing heating temperature. The highest percentage of α-tocopherol in rice bran oil was lost by 26.08% after heating at 100°C for 432 h, while it was no longer detected at 180°C for 240 h. The results showed that α-tocopherol was not detected in rice bran oil. The contents of (γ + β)-tocopherol in rice bran oil was 79.9 mg/100 g, and δ-tocopherol was 6.4 mg/100 g, respectively, when heated at 100°C for 432 h. At 180°C for 432 h, the contents of (γ + β)-tocopherol were 1.4 mg/kg and δ-tocopherol were 0.3 mg/kg. Srisaipet and Nuddagul found that the stability of rice bran oil was related to the content of γ-oryzanol in addition to the temperature during heat treatment (56). γ-oryzanol can react with KOH to form carbonyl compounds due to the breaking of double bonds and oxidation, which are oxidized to low molecular weight fatty acids during heating and lost through volatilization. In addition to the commonly used dry heat treatment, an infrared (IR) stabilization method to prevent rancidity of rice bran was developed by Yılmaz et al. (57). The FFA content of rice bran stabilized at 600 W IR power for 5 min remained below 5% for 165 days. There was no significant change in γ-oryzanol content or fatty acid composition in stabilized rice bran compared to raw rice bran. Therefore, IR can be used as a medium to inactivate lipase and extend the shelf life of rice bran.
Rice bran undergoes hydrolytic rancidity owing to the contact between lipids and active enzymes. Among them, lipase causes hydrolysis of rice bran oil to produce free fatty acids (FFA). This hydrolytic rancidity affects the flavor and odor of rice bran, thus limiting further development of rice bran. Conventional alkaline refining deacidification leads to loss of crude oil as well as other bioactive components (58, 59). There are a number of novel alternative methods (e.g., fractionation and enzymatic) that can effectively remove FFA while also stabilizing the antioxidants in rice bran. Dunford et al. focused on the potential of continuous countercurrent SC-CO2 fractionation for the deacidification of rice bran oil. The results showed that fractionation at a low pressure of 138 Bar and a high temperature of 80°C effectively removed FFA from rice bran oil without any oryzanol loss in the extract (60). According to the literature (61), when the CO2 filling pressure was 8.0 MPa, the FFA content of rice bran oil decreased to 1.1% and the phytosterol conversion reached 93.2%. Moreover, Xu et al. proposed and developed a tandem continuous flow reactor, which is used to deacidify rice bran oil and product functional oils. Compared to commercial Novozym 435, the tandem continuous-flow enzymatic reactor removed 91.4% of FFA and increased phytosterol esters and diacylglycerol in rice bran oil by 9- and 12-fold, respectively. Based on this, the research team innovated the method of removing FFA. They prepared a nanomagnetic Fe3O/SiOx-g-P lipase (magnetic enzyme) by immobilizing CALB on Fe3O4/SiOx-g-P polymer carrier. Using this as a catalyst, FFA from rice bran oil were used to esterify phytosterols. The FFA content in rice bran oil was reduced from 16.0 to 2.4%, and the conversion of phytosterols was 85.7% (62). In addition, the retention rate of γ-oryzanol is more than 40% higher than that of traditional alkaline refining method (63). In order to improve the reuse and value-added conversion of crude high acid rice bran oil, enzymes are often employed to obtain phytosterols by enzymatic esterification with FFA from rice bran. The literature review shows that immobilized Candida rugosa lipase (CRL) on SiO2@P (MAA-co-VBC-co-DVB) particles can get 92.1% conversions (64).
In order to simultaneously carry out the hydrolysis of rice bran oil and the esterification reaction with phytosterols, and have the advantages of high efficiency, sustainability, solvent-free process and multiple recovery, Yang et al. proposed a pH-switchable Pickering interface biocatalyst (PIB) system (65). The system was based on amine-functionalized mesoporous silica nanospheres, with PIB as the emulsifier and AYS@HMSS-N (AYS) as the carrier, and the relative conversion rate of phytosterols was maintained at 95%. In the search for greener, more efficient and less consumptive methods with high yields and high activity, numerous studies are now focusing on the application of microbial fermentation in the production of physiologically active secondary metabolites. Numerous studies are now focusing on the application of microbial fermentation in the production of physiologically active secondary metabolites. The microorganism Moraxella oviswas a probiotic that can utilize rice bran oil and selectively degrade phytosterols, and this strain can produce androst-4-ene-3,17-dione, androsta-1,4-diene-3,17-dione, testosterone, and estrone (66). Deodorization is also the key step of rice bran in the process of deacidification. Adopting nitrogen as the vapor extraction gas is more suitable for deodorization, it promotes the formation of phytosterols, reduces the production of phytosterols oxidation product, and improves the quality of oil (67). In addition, the loss of biological activity of rice bran is a concern. Compared to CPE, SE obtained higher levels of aminobutyric acid (97.37 mg/100 g), γ-oryzanol (3829.65 mg/100 g), phytosterols (599.40 mg/100 g), and polyglycol compounds (332.79 mg/100 g) (68). Afterwards, microwave pretreatment (440 W, 2.5 min) of rice bran increased antioxidant activity (0.5-fold), total phenolic content (1.3-fold), flavonoids (0.9-fold), total tocopherols (2.6-fold), total γ-oryzanol (1.6-fold), and total phytosterols (1.4-fold) (69).
As the second leading cause of death and morbidity worldwide, cancer generally refers to the uncontrolled cell growth that may spread to other parts of body (70, 71). These abnormal differentiation and proliferation may result in the dysregulated expression of genes involved in cell cycle control (72). The great costs and poor therapeutic effect associated with conventional therapies limit the clinic therapeutic efficacy. Diet has been identified as an important and modifiable risk factor for cancer (73, 74). Therefore, modification in dietary habits that involves the inclusion of functional food components with chemopreventive properties has been identified as a potential strategy for halting or reversing the early phases before the manifestation of a malignancy. Kim et al. confirmed that γ-oryzanol influence of speed in tumor regression via NK activity and macrophages activation and angiogenesis inhibition. Notably, this strategy has almost no side effect (75). Forster et al. investigated the inhibitory effect on human colorectal cancer (CRC) cells growth for the seven rice bran varieties. Significantly, α-tocotrienol, γ-tocotrienol, δ-tocotrienol, and tocopherols showed 1.3- to 15.2-fold differences, respectively. Based on the spearman correlation analysis of the content of γ-tocotrienol and total phenolics were positively correlated with the CRC cells inhibitory effect. Stoichiometric variation in rice bran components and differential effects on colorectal cancer viability merit further evaluation elucidate their role in dietary colorectal cancer chemoprevention (76). These combined considerations indicate that the tocotrienol-rich fraction-based diet with the potential anti-cholesterol and anti-cancer activity could be potentially good candidates in cancer prevention or treatment.
Inflammation functions as a protective reaction against multiple detrimental stimuli, potentially leading to tissue damage and infection, by means of targeted production of proinflammatory molecules (77, 78). In terms of duration, inflammation can generally be categorized into two primary classifications: acute inflammation and chronic inflammation (79). Mizushina isolated cycloartenyl trans-ferulate from the byproduct after the sake-brewing production. With the remarkably high selectivity for mammalian A, B, and X pol families, trans-ferulate has been observed to mitigte the TPA-induced inflammatory response. This finding implies both the byproduct and cycloartenyl trans-ferulate hold potential as an effective agent for anti-inflammatory health promotion (80). Akihisa et al. isolated two trans-ferulates and four cis-ferulates from edible rice bran methanol extract. Results of anti-inflammatory assay revealed that ferulates presented marked inhibitory effect with the 50% inhibition in the range of 0.1–0.8 mg per ear. Moreover, cycloartenol and 24-methylenecycloartanol presented remarkable inhibitory effect with the ID50 in the range of 0.2–0.3 mg per ear. Compared with the corresponding ferulates, eight types of free sterols had weaker activity in the range of ID50 0.7–2.7 mg per ear. Taken together, these evidence could facilitate the advanced utilization of rice derived products within the food-related industry (81).
As a chronic cardiovascular disease, atherosclerosis is the develop of narrowing and hardening of the abnormal cholesterol deposits in the inner layers of arteries (82). It is the result of hyperlipidemia and lipid oxidation and is the key factor leading to cardiovascular diseases such as stroke and heart attack in developed countries (83). Correlativity study showed that combination of hypocholesterolemic agents and functional compositions in diets may restrict the risk of developing atherosclerotic lesions, thus, Revilla et al. isolated enzymatically from rice bran, which consist of sterols, tocotrienols, γ-oryzanol, tocopherols, and peptides. The experimental data suggest that this new product may be a good source of functional food ingredient because of the advantage of water solubility, antioxidant and hypocholesterolemic capacity in comparison with rice bran (84).
It is known that skin health is not only significantly caused by overexposure to solar ultraviolet (UV) radiation, but also by contaminant, chemical oxidants, microorganisms, and inflammatory reactions (85). Skin tissue, which consist of the epidermis, dermis, and subcutaneous fat layers, plays a role in maintaining skin barrier function (86). While the damage of skin barrier function has been widely confirmed by an altered stratum corneum integrality, with a consequent increase in trans epidermal water loss and decrease in skin hydration (87). Thus, discovering new dietary bioactive to have a positive influence on skin health conditions is extremely important (88, 89). Earlier, Yasukawa et al. observed that the functional composition from rice bran remarkably inhibited the TPA-induced inflammation with IC50 at 0.2–0.3 mg per ear. Amongst 24-methylcholesterol ferulate, sitosterol ferulate, cycloartenol ferulate, and 24-methylenecycloartanol ferulate, cycloartenol ferulate presented the significant inhibition ability of TPA in 7,12-dimethylbenz[a]anthracene-initiated mouse (90). Recently, Vardhani et al. provided the sufficiently potent of black rice bran extract for utilization in the recipe for skin whitening. In vitro, the melanin levels reduced at 3- and 30 μM γ-oryzanol doses, specifically. Also, the ethanolic black rice bran extract excellently inhibited tyrosinase activity with an IC50 of 74.8% (91). Kobayashi et al. developed a method for producing phytosterol ester extract, which consist of phytosterols, triterpene alcohols, and fatty acids, from byproducts of the refine rice bran oil. Both the normal human fibroblasts cell assay and image analysis results suggested that phytosterol ester extract could have a positive impact on the deteriorated barrier function. Furthermore, the administration of phytosterol ester extract topically resulted in an anti-inflammatory response and enhanced moisturization by augmenting barrier function, consequently mitigating erythema and pore-related issues induced by inflammation (92).
Obesity has been correlated positively with an increased risk of developing various severe medical conditions, including diabetes, myocardial infarction, cerebrovascular accidents, as well as certain types of carcinomas (93). To take precautions against diet-induced obesity, Fukuoka et al. demonstrated that rice bran-derived, oil-soluble triterpene alcohol and sterol preparation (TASP) enhanced the efficiency of fat and attenuated diet-induced obesity via reducing the postprandial glucose-dependent insulinotropic polypeptide (GIP) content, which indicated that the enriched TASP product may be a promising approach to develop GIP-based obesity-controlling product (94). During energy restriction-induced weight loss in overweight and obese adults without cholesterol-lowering medication intake, Hongu et al. confirmed that plant sterols in rice bran could influence the obesity intervention on lipid profiles. Collectively the findings indicate that overweight and obese adults who followed a diet that was both nutrient-balanced and restricted in calories by 25%, while also using rice bran and plant sterols as supplements, had a notable reduction in their levels of LDL-C and total cholesterol (95).
Previous studies have confirmed that dietary supplementation, such as chlorella vulgaris (96), carotenoids (97), and salidroside (98), can protect against diabetes mellitus and its complications (99, 100). Nevertheless, the misunderstanding of the molecular mechanisms in regulating diabetes diseases and the potential utilization of nutritional supplementation is currently undergoing comprehensive researches. For example, Kaup and co-workers provided both in vitro and in vivo validation of stabilized rice bran extract had a concentration-dependent anti-diabetic effect, which was of interest that γ-oryzanol might be a candidate for anti-diabetic effect. Thus, it is reasonable to extrapolate that the prevalence of diabetes or at least a prediabetic situation can effectively retard via rice bran extract intake (101). On the basis of observations from streptozotocin/nicotinamide-induced type 2 diabetes rat model, the utilization of a rice bran oil diet by Chen could block the hyperinsulinemic and hyperlipidemic reaction processes. And the hypocholesterolemic mechanism of rice bran oil might due to the high concentrations of γ-oryzanol and γ-tocotrienol could enhance fecal neutral sterol and bile acid release via upregulating cholesterol synthesis and catabolism (102).
Neurodegenerative diseases, characterized by progressive loss in selected areas of the nervous system, are becoming one of the increasingly and common global health burdens (103, 104). Particularly in the elderly, aging-related neurological disorders are now the leading source of disability in the world and Parkinson’s disease is the fastest-growing of these disorders. Despite their diverse clinical manifestations, neurodegenerative diseases are multifactorial disorders with standard features and mechanisms such as abnormal protein aggregation, mitochondrial dysfunction, oxidative stress and inflammation. From rice bran and rice bran oil, Zhang et al. demonstrated that campesteryl ferulate, 24-methylenecycloartanyl ferulate, sitosterol, and cycloartenyl ferulate presented dopaminergic neuroprotective ability via the DAF-16/FOXO pathway activation and the inhibition of the apoptotic protein CED-3 expression. In terms of these findings, a significant insight into the potential of four phytosterols as therapeutics in protecting the dopaminergic neurons was provided (105). Thus, this rice bran-based food product might be considered as potential candidates to be used as dietary supplement, helping to prevent the development of the neurodegenerative diseases.
Peptic ulcers are one of the major human illnesses of the entire gastrointestinal tract, which affecting nearly 10% of the world’s population, among them, 5% suffer from gastric ulcers (106). The imbalance between endogenous aggressive factors and cytoprotective factors lead to the pathogenesis of gastric ulcers, which occur mainly in the stomach and the proximal duodenum (107). Recently, scientific evaluations on different types of phytosterols have been identified and revealed to exhibit potential prevention or cure activities of peptic ulcers. Therefore, the assessment of food-derived components potential as a source of an anti-ulcer agent is still one of the important challenges confronting medicine nowadays. Trinovita et al. obtained rice bran extract by ionic liquid-microwave-assisted method and calculated the effectiveness of rice bran extract as a gastroprotective in ethanol-induced acute gastric ulcer models. Results show that rice bran extract could suppress the ulcer formation by 66.75% and gastric acid levels, and increased the secretion of gastric mucus at the dose of 400 mg kg−1 BW. Thus, rice bran extract has potential to be considered as gastroprotective agents from rice bran in gastric ulcers treatments (108).
Nowadays, there is a renewed interest in developing and discovering anti-allergic phytochemicals for the prevention of allergic diseases, which is a serious health problem worldwide (109, 110). As one of the immune dysfunctions, hypersensitivity reactions can be divided into four general categories based on the mechanism of immunological response: (1) immediate or anaphylactic hypersensitivity mediated by IgE, (2) antibody-mediated cytotoxicity mediated by antibodies of the IgM or IgG classes and complement, (3) immune complex hypersensitivity mediated by IgG or IgM classes, and (4) cell mediated or delayed type hypersensitivities. Recently it has been pointed out that dietary change might contribute to the onset of allergic diseases. For instance, the essential mechanisms of vitamins, flavonoids and related compounds’ anti-allergic action were detailly investigated. In order to reveal interacting points between cycloartenyl ferulate and allergic reaction, Oka et al. building the passive cutaneous anaphylaxis rats model induced by DNP-HAS (111). After cycloartenyl ferulate and γ-oryzanol were injected intradermally with anti-DNP IgE into the dorsal skin of rats, the hypersensitive reaction was attenuated markedly. Overall, the finding regarding anti-allergic activity was mainly contributed by the cycloartenyl ferulate could binding with IgE and ultimately weaken mast cell degranulation.
In the study of Castanho et al., γ-oryzanol have extracted and quantified from three different rice bran varieties. Among Ketoconazole, Bifonazole, Ballatinao, Maluit, Dinordo, Arabon, Bora, NSICRC9, Azucena, Macarico, and Ceres, not only the Portuguese Ceres presented the highest bacterial inhibition ability, but also all the varieties could inhibit the majority of bacterial and fungal strains. The antimicrobial activity assays showed that Maluit and Dinorado demonstrated the highest fungal inhibition activity. Anti-tumor activity assay showed that Ballatinao with the highest content of γ-oryzanol also presented the greatest capability against all the tested tumor cell lines. Therefore, all the results indicated that rice extracts may be used as the potential candidates od natural antimicrobial and antitumor agents (112).
The human innate immune response is of prime importance in host defense system against pathogens, which consists of two branches: innate and adaptive immunity (113). The innate immune system recognizes pathogens and apoptotic cells through particular receptors and responds by activating immune competent. It is clear that recent studies indicate that phytosterols in Hypoxis erxtracts might ultimately influence the innate immune system response. By studying the immune protective activity of γ-oryzanol-rich extract from black rice bran, it was found that the enhancement of the phagocytic activity of RAW264.7 macrophages occurred, and the expression level of Toll-like receptor 4 and cluster of differentiation 14 elevated. Moreover, γ-oryzanol-rich extract and γ-oryzanol could promote the production of IL-8, and CCL2, which suggest that γ-oryzanol could be developed as a functional food components for improving the innate immune ability (114). Based on the research form Wilankar et al., tocotrienols possess sufficient immunomodulatory activities in murine lymphocytes. Compared with α-tocotrienol, novel findings are reported on the inhibition effect of γ-tocotrienol was more effectively contributed in cytokine formation and concanavalin A-induced T cell proliferation (115).
Rice bran oil, with its optimal proportion of fatty acids and rich nutrient content, can be utilized to produce functional blended oils. By incorporating rice bran oil with various other plant oils, the complementary advantages of different oils can create a more comprehensive nutritional profile. This review specifically highlights the presence of phytosterols, beneficial compounds found in rice bran oil, which are typically present in lower quantities in other plant oils. Consequently, the development of blended oils by combining multiple plant oils can significantly enhance the nutritional value of the final product, contributing to a healthier diet. In addition to its uses as a blending oil, rice bran oil can also be used as a frying oil and for the development of healthy food products. Additionally, further research is still needed to fully understand the biological effects of phytosterols present in rice bran oil. The thriving and fast-paced growth of the rice bran industry is expected to bring significant economic benefits and societal value.
In summary, rice bran oil offers numerous nutritional benefits due to its abundance of phytosterols, making it an excellent choice for the high-value utilization of rice bran, a byproduct of rice processing. Not only does it have the potential for application in functional food products, but ongoing advancements in extraction and processing techniques have resulted in rice bran oil products with outstanding stability and sensory attributes. As a result, this progress not only fuels further exploration and development of rice bran within the food industry but also opens doors for its application in pharmaceuticals, chemicals, and other diverse sectors.
ZL: Conceptualization, Writing – original draft, Data curation. XL: Data curation, Writing – original draft. ZM: Funding acquisition, Supervision, Writing – review & editing. TG: Funding acquisition, Conceptualization, Writing – original draft.
The author(s) declare financial support was received for the research, authorship, and/or publication of this article. This work was supported by the Jiangsu Provincial Research Foundation for Basic Research of China (Grant No. BK20200954) and the National Youth Talent Support Program of the China Association for Science and Technology (20220615ZZ07110388).
The authors declare that the research was conducted in the absence of any commercial or financial relationships that could be construed as a potential conflict of interest.
All claims expressed in this article are solely those of the authors and do not necessarily represent those of their affiliated organizations, or those of the publisher, the editors and the reviewers. Any product that may be evaluated in this article, or claim that may be made by its manufacturer, is not guaranteed or endorsed by the publisher.
The Supplementary material for this article can be found online at: https://www.frontiersin.org/articles/10.3389/fnut.2023.1287405/full#supplementary-material
2. Prakash, J, and Ramaswamy, H. Rice bran proteins: properties and food uses. Crit Rev Food Sci Nutr. (1996) 36:537–52. doi: 10.1080/10408399609527738
3. Ren, H, Qi, S, Zhang, L, Wang, L, Huang, J, Yang, H, et al. Variations in the appearance quality of brown rice during the four stages of milling. J Cereal Sci. (2021) 102:103344. doi: 10.1016/j.jcs.2021.103344
4. Raghav, PK, Agarwal, N, and Sharma, A. Emerging health benefits of rice bran – a review. Int J Multidiscip Res Mod Educ. (2016) 2:367–82. doi: 10.4172/2155-9600.1000108
5. Mohidem, NA, Hashim, N, Shamsudin, R, and Che Man, H. Rice for food security: revisiting its production, diversity, rice milling process and nutrient content. Agriculture. (2022) 12:741. doi: 10.3390/agriculture12060741
6. Handayani, O.W.K., and Kurnia, A.R., Rice bran based Vitabran sports vitality booster, ISMINA 2021: Proceedings of the 5th International Conference on Sports, Health, and Physical Education, ISMINA 2021, 28–29, 2021, Semarang, Central Java, Indonesia, European Alliance for Innovation, (2021), 400
7. Bigliardi, B, and Galati, F. Innovation trends in the food industry: the case of functional foods. Trends Food Sci Technol. (2013) 31:118–29. doi: 10.1016/j.tifs.2013.03.006
8. Esa, NM, Ling, TB, and Peng, LS. By-products of rice processing: an overview of health benefits and applications. J Rice Res. (2013) 4:2. doi: 10.4172/jrr.1000107
9. Sharif, MK, Butt, MS, Anjum, FM, and Khan, SH. Rice bran: a novel functional ingredient. Crit Rev Food Sci Nutr. (2014) 54:807–16. doi: 10.1080/10408398.2011.608586
10. Wu, X, Li, F, and Wu, W. Effects of rice bran rancidity on the oxidation and structural characteristics of rice bran protein. LWT. (2020) 120:108943. doi: 10.1016/j.lwt.2019.108943
11. Kim, S-M, Chung, H-J, and Lim, S-T. Effect of various heat treatments on rancidity and some bioactive compounds of rice bran. J Cereal Sci. (2014) 60:243–8. doi: 10.1016/j.jcs.2014.04.001
12. Rashid, MT, Liu, K, Hen, S, Jatoi, MA, and Sarpong, F. Nutritional composition and volatile compounds stability in dry-heat and extruded stabilised rice bran during storage. Int J Food Sci Technol. (2023) 58:3379–91. doi: 10.1111/ijfs.16323
13. Sivamaruthi, BS, Alagarsamy, K, Thangaleela, S, Bharathi, M, Kesika, P, and Chaiyasut, C. Composition, microbiota, mechanisms, and anti-obesity properties of Rice bran. Foods. (2023) 12:1300. doi: 10.3390/foods12061300
14. Sereewatthanawut, I, Prapintip, S, Watchiraruji, K, Goto, M, Sasaki, M, and Shotipruk, A. Extraction of protein and amino acids from deoiled rice bran by subcritical water hydrolysis. Bioresour Technol. (2008) 99:555–61. doi: 10.1016/j.biortech.2006.12.030
15. Choi, B-M, Lanning, S, and Siebenmorgen, T. A review of hygroscopic equilibrium studies applied to rice. Trans ASABE. (2010) 53:1859–72. doi: 10.13031/2013.35795
16. Gul, K, Yousuf, B, Singh, A, Singh, P, and Wani, AA. Rice bran: nutritional values and its emerging potential for development of functional food—a review. Bioact Carbohydr Diet Fibre. (2015) 6:24–30. doi: 10.1016/j.bcdf.2015.06.002
17. Zaky, AA, Abd El-Aty, A, Ma, A, and Jia, Y. An overview on antioxidant peptides from rice bran proteins: extraction, identification, and applications. Crit Rev Food Sci Nutr. (2022) 62:1350–62. doi: 10.1080/10408398.2020.1842324
18. Ghosh, M. Review on recent trends in rice bran oil processing. J Am Oil Chem Soc. (2007) 84:315–24. doi: 10.1007/s11746-007-1047-3
19. Shahidi, F. (Ed.). Bailey’s Industrial Oil and Fat Products, Industrial and Nonedible Products from Oils and Fats (Vol. 6). John Wiley & Sons (2005).
20. Fraterrigo Garofalo, S, Tommasi, T, and Fino, D. A short review of green extraction technologies for rice bran oil. Biomass Convers Biorefinery. (2021) 11:569–87. doi: 10.1007/s13399-020-00846-3
21. Wang, Y., Applications of rice bran oil, rice bran and rice bran oil, Elsevier, (2019), 159–168.
22. Hoang, AT, Tabatabaei, M, Aghbashlo, M, Carlucci, AP, Ölçer, AI, Le, AT, et al. Rice bran oil-based biodiesel as a promising renewable fuel alternative to petrodiesel: a review. Renew Sust Energ Rev. (2021) 135:110204. doi: 10.1016/j.rser.2020.110204
23. Uraipong, C, and Zhao, J. In vitro digestion of rice bran proteins produces peptides with potent inhibitory effects on α-glucosidase and angiotensin I converting enzyme. J Sci Food Agric. (2018) 98:758–66. doi: 10.1002/jsfa.8523
24. Wang, N, Cui, X, Duan, Y, Yang, S, Wang, P, Saleh, AS, et al. Potential health benefits and food applications of rice bran protein: research advances and challenges. Food Rev Intl. (2023) 39:3578–601. doi: 10.1080/87559129.2021.2013253
25. Gylling, H, and Simonen, P. Phytosterols, phytostanols, and lipoprotein metabolism. Nutrients. (2015) 7:7965–77. doi: 10.3390/nu7095374
26. García-Llatas, G, and Rodríguez-Estrada, MT. Current and new insights on phytosterol oxides in plant sterol-enriched food. Chem Phys Lipids. (2011) 164:607–24. doi: 10.1016/j.chemphyslip.2011.06.005
27. Moreau, RA, Nyström, L, Whitaker, BD, Winkler-Moser, JK, Baer, DJ, Gebauer, SK, et al. Phytosterols and their derivatives: structural diversity, distribution, metabolism, analysis, and health-promoting uses. Prog Lipid Res. (2018) 70:35–61. doi: 10.1016/j.plipres.2018.04.001
28. Bacchetti, T, Masciangelo, S, Bicchiega, V, Bertoli, E, and Ferretti, G. Phytosterols, phytostanols and their esters: from natural to functional foods. Mediterr J Nutr Metab. (2011) 4:165–72. doi: 10.3233/s12349-010-0049-0
29. Hicks, KB, and Moreau, RA. Phytosterols and phytostanols: functional food cholesterol busters. Food technology. (2001) 55:63–7. doi: 10.1081/FRI-100108533
30. Mingyai, S, Srikaeo, K, Kettawan, A, Singanusong, R, Nakagawa, K, Kimura, F, et al. Effects of extraction methods on phytochemicals of rice bran oils produced from colored Rice. J Oleo Sci. (2018) 67:135–42. doi: 10.5650/jos.ess17122
31. Sahu, S, Ghosh, M, and Bhattacharyya, DK. Isolation of the unsaponifiable matter (squalene, phytosterols, tocopherols, γ-oryzanol and fatty alcohols) from a fatty acid distillate of rice bran oil. Int J Fats. (2018) 69:262. doi: 10.3989/gya.1112172
32. Lerma-García, M, Herrero-Martínez, J, Simó-Alfonso, E, Mendonça, CR, and Ramis-Ramos, G. Composition, industrial processing and applications of rice bran γ-oryzanol. Food Chem. (2009) 115:389–404. doi: 10.1016/j.foodchem.2009.01.063
33. Kumar, P, Yadav, D, Kumar, P, Panesar, PS, Bunkar, DS, Mishra, D, et al. Comparative study on conventional, ultrasonication and microwave assisted extraction of γ-oryzanol from rice bran. J Food Sci Technol. (2016) 53:2047–53. doi: 10.1007/s13197-016-2175-2
34. Sookwong, P, Suttiarporn, P, Boontakham, P, Seekhow, P, and Mahatheeranont, S. Simultaneous quantification of vitamin E, γ-oryzanols and xanthophylls from rice bran essences extracted by supercritical CO2. Food Chem. (2016) 211:140–7. doi: 10.1016/j.foodchem.2016.05.001
35. Wongwaiwech, D, Kamchonemenukool, S, Ho, C-T, Li, S, Majai, N, Rungrat, T, et al. Bioactives from crude rice bran oils extracted using green technology. Molecules. (2023) 28:2457. doi: 10.3390/molecules28062457
36. Sangpradab, J, Kamonpatana, P, Suwannaporn, P, and Huang, TC. Ohmic heating-aided mechanical extraction of gamma-oryzanol and phytosterols in rice bran oil. Food Bioprocess Technol. (2021) 14:1542–54. doi: 10.1007/s11947-021-02655-6
37. Imsanguan, P, Roaysubtawee, A, Borirak, R, Pongamphai, S, Douglas, S, and Douglas, PL. Extraction of α-tocopherol and γ-oryzanol from rice bran. LWT – Food Sci Technol. (2008) 41:1417–24. doi: 10.1016/j.lwt.2007.08.028
38. Kayathi, A, Chakrabarti, PP, Bonfim-Rocha, L, Cardozo-Filho, L, Bollampalli, A, and Jegatheesan, V. Extraction of γ-oryzanol from defatted rice bran using supercritical carbon dioxide (SC-CO2): process optimisation of extract yield, scale-up and economic analysis. Process Saf Environ Prot. (2021) 148:179–88. doi: 10.1016/j.psep.2020.09.067
39. Chen, CR, Wang, LY, Wang, CH, Ho, WJ, and Chang, CMJ. Supercritical carbon dioxide extraction of rice bran oil and column partition fractionation of γ-oryzanols. Sep Purif Technol. (2008) 61:358–65. doi: 10.1016/j.seppur.2007.11.003
40. Yoon, SW, Pyo, YG, Lee, J, Lee, JS, and Kim, IH. The concentrations of tocols and γ-oryzanol compounds in rice bran oil obtained by fractional extraction with supercritical carbon dioxide. J Oleo Sci. (2014) 63:47–53. doi: 10.5650/jos.ess13144
41. Jesus, SP, Grimaldi, R, and Hense, H. Recovery of γ-oryzanol from rice bran oil byproduct using supercritical fluid extraction. J Supercrit Fluids. (2010) 55:149–55. doi: 10.1016/j.supflu.2010.08.004
42. Kamchonemenukool, S, Wongwaiwech, D, Thongsook, T, and Weerawatanakorn, M. Subcritical liquified dimethyl ether and supercritical fluid carbon dioxide extraction of gamma oryzanol from rice bran acid oil. J Agric Food Res. (2023) 14:100672. doi: 10.1016/j.jafr.2023.100672
43. Wongwaiwech, D, Weerawatanakorn, M, and Boonnoun, P. Subcritical dimethyl ether extraction as a simple method to extract nutraceuticals from byproducts from rice bran oil manufacture. Sci Rep. (2020) 10:21007. doi: 10.1038/s41598-020-78011-z
44. Yamamoto, Y, and Hara, S. Novel fractionation method for squalene and phytosterols contained in the deodorization distillate of rice bran oil, Journal of oleo. Science. (2012) 33:126. doi: 10.5772/33126
45. Bitencourt, RG, Filho, WAR, Paula, JT, Garmus, TT, and Cabral, FA. Solubility of γ-oryzanol in supercritical carbon dioxide and extraction from rice bran. J Supercritical Fluids. (2015) 107:196–200. doi: 10.1016/j.supflu.2015.09.009
46. Liu, H-M, Wang, F-Y, Li, H-Y, Wang, X-D, and Qin, G-Y. Subcritical butane and propane extraction of oil from rice bran. Bioresources. (2015) 10:4652–62. doi: 10.15376/biores.10.3.4652-4662
47. Angelis, A, Urbain, A, Halabalaki, M, Aligiannis, N, and Skaltsounis, AL. One-step isolation of γ-oryzanol from rice bran oil by non-aqueous hydrostatic countercurrent chromatography. J Sep Sci. (2015) 34:2528–37. doi: 10.1002/jssc.201100192
48. Xu, Z, and Godber, JS. Purification and identification of components of gamma-oryzanol in rice bran oil. J Agric Food Chem. (1999) 47:2724–8. doi: 10.1021/jf981175j
49. Bao, Y, Yanase, E, and Nakatsuka, SI. Isolation of campesteryl ferulate and epi-campesteryl ferulate, two components of γ-oryzanol from rice bran. J Agric Chem Soc Japan. (2013) 77:877–9. doi: 10.1271/bbb.120938
50. Sawada, K, Nakagami, T, Rahmania, H, Matsuki, M, and Nakagawa, K. Isolation and structural elucidation of unique γ-oryzanol species in rice bran oil. Food Chem. (2021) 337:127956. doi: 10.1016/j.foodchem.2020.127956
51. Deepam, LSA, Sundaresan, A, and Arumughan, C. Stability of rice bran oil in terms of oryzanol, tocopherols, tocotrienols and sterols. J Am Oil Chem Soc. (2011) 88:1001–9. doi: 10.1007/s11746-010-1744-1
52. Yi, BR, Lee, JH, and Kim, MJ. Increasing oxidative stability in corn oils through extraction of γ-oryzanol from heat treated rice bran. J Cereal Sci. (2019) 91:102880. doi: 10.1016/j.jcs.2019.102880
53. Zhao, Z, Huang, J, Jin, Q, and Wang, X. Influence of oryzanol and tocopherols on thermal oxidation of rice bran oil during the heating process at Chinese cooking temperatures. LWT – Food Sci Technol. (2021) 142:111022. doi: 10.1016/j.lwt.2021.111022
54. Huang, J, Chen, C, Zhao, Z, Li, X, Wang, X, Jin, Q, et al. Inhibition effect of oryzanol on the degradation of tocopherol and the oxidation kinetic of rice bran oils with different content of oryzanol and tocopherol. Eur J Lipid Sci Technol. (2022) 124:124. doi: 10.1002/ejlt.202100155
55. Bruscatto, MH, Zambiazi, RC, Sganzerla, M, Pestana, VR, Otero, D, Lima, R, et al. Degradation of tocopherols in rice bran oil submitted to heating at different temperatures. J Chromatogr Sci. (2009) 47:762–5. doi: 10.1093/chromsci/47.9.762
56. Srisaipet, A, and Nuddagul, M. Influence of temperature on gamma-oryzanol stability of edible rice bran oil during heating. Int J Chem Eng Appl. (2015) 5:303–6. doi: 10.7763/IJCEA.2014.V5.398
57. Yılmaz, NE, Tuncel, NB, and Kocabıyık, H. Infrared stabilization of rice bran and its effects on γ -oryzanol content, tocopherols and fatty acid composition. J Sci Food Agric. (2014) 94:1568–76. doi: 10.1002/jsfa.6459
58. Liu, R, Liu, R, Shi, L, Zhang, Z, and Wang, X. Effect of refining process on physicochemical parameters, chemical compositions and in vitro antioxidant activities of rice bran oil. LWT – Food Sci Technol. (2019) 109:26–32. doi: 10.1016/j.lwt.2019.03.096
59. Pestana, VR, Zambiazi, RC, Mendonça, CRB, Bruscatto, MH, Lerma-García, MJ, et al. Quality changes and tocopherols and γ-orizanol concentrations in rice bran oil during the refining process. J Am Oil. (2008) 85:1013–9. doi: 10.1007/s11746-008-1300-4
60. Dunford, NT, Teel, JA, and King, JW. A continuous countercurrent supercritical fluid deacidification process for phytosterol ester fortification in rice bran oil. Food Res Int. (2003) 36:175–81. doi: 10.1016/S0963-9969(02)00134-5
61. Yu, D, Wang, T, Chen, J, Tang, H, Li, D, Zhang, X, et al. Enzymatic esterification of rice bran oil and phytosterol in supercritical CO2. J Food Process Preserv. (2019) 43:14066. doi: 10.1111/jfpp.14066
62. Yu, D, Yu, C, Wang, T, Chen, J, and Wu, F. Study on the deacidification of rice bran oil esterification by magnetic immobilized lipase. Catal Lett. (2020) 150:1256–67. doi: 10.1007/s10562-019-02939-9
63. Xu, L, Zhang, Y, Zivkovic, V, and Zheng, M. Deacidification of high-acid rice bran oil by the tandem continuous-flow enzymatic reactors. Food Chem. (2022) 393:133440. doi: 10.1016/j.foodchem.2022.133440
64. Zhang, M, Zhu, J, Huang, F, Xiang, X, Shi, J, Deng, Q, et al. Enzymatic deacidification of the rice bran oil and simultaneous preparation of phytosterol esters-enriched functional oil catalyzed by immobilized lipase arrays. RSC Adv. (2015) 5:70073–9. doi: 10.1039/C5RA11533G
65. Yang, T, Zhang, Y, and Xu, L. pH-switchable pickering interfacial biocatalysis: one-pot enzymatic synthesis of phytosterol esters with low-value rice bran oil. ACS Sustain Chem Eng. (2022) 10:6963–72. doi: 10.1021/acssuschemeng.1c08719
66. Sallam, LAR, Osman, ME, Hamdy, AA, and Zaghlol, GM. Microbial transformation of phytosterols mixture from rice bran oil unsaponifiable matter by selected bacteria. World J Microbiol Biotechnol. (2008) 24:1643–56. doi: 10.1007/s11274-008-9659-9
67. Yu, D, Dong, T, Zhang, L, Zhou, X, Wang, L, Yang, F, et al. Effects of different deodorization methods on the oxidation of sterol components in rice bran oil. Food Chem. (2023) 404:134568. doi: 10.1016/j.foodchem.2022.134568
68. Donporn, W, Monthana, W, Somsak, T, and Chi-Tang, H. Comparative study on amount of nutraceuticals in by-products from solvent and cold pressing methods of rice bran oil processing. J Food Drug Anal. (2018) 6:6. doi: 10.1016/j.jfda.2018.06.006
69. Pokkanta, P, Yuenyong, J, Mahatheeranont, S, Jiamyangyuen, S, and Sookwong, P. Microwave treatment of rice bran and its effect on phytochemical content and antioxidant activity. Sci Rep. (2022) 12:7708. doi: 10.1038/s41598-022-11744-1
70. Dodia, S, Annappa, B, and Mahesh, PA. Recent advancements in deep learning based lung cancer detection: A systematic review. Engineering Applications of Artificial Intelligence (2022) 116:105490. doi: 10.1016/j.engappai.2022.105490
71. Yadav, AR, and Mohite, SK. Cancer-a silent killer: an overview. Asian J Pharmaceutical Res. (2020) 10:213–6.
72. Gómez-López, S, Lerner, RG, and Petritsch, C. Asymmetric cell division of stem and progenitor cells during homeostasis and cancer. Cell Mol Life Sci. (2014) 71:575–97. doi: 10.1007/s00018-013-1386-1
73. Wolk, A. Diet, lifestyle and risk of prostate cancer. Acta Oncol. (2005) 44:277–81. doi: 10.1080/02841860510029572
74. Grosso, G. Impact of nutritional risk factors on chronic non-communicable diseases. Eur J Pub Health. (2019) 29:197. doi: 10.1093/eurpub/ckz185.197
75. Kim, SP, Kang, MY, Nam, SH, and Friedman, M. Dietary rice bran component γ-oryzanol inhibits tumor growth in tumor-bearing mice. Mol Nutr Food Res. (2012) 56:935–44. doi: 10.1002/mnfr.201200057
76. Forster, GM, Raina, K, Kumar, A, Kumar, S, Agarwal, R, Chen, M-H, et al. Rice varietal differences in bioactive bran components for inhibition of colorectal cancer cell growth. Food Chem. (2013) 141:1545–52. doi: 10.1016/j.foodchem.2013.04.020
77. Serhan, CN, Brain, SD, Buckley, CD, Gilroy, DW, Haslett, C, O’Neill, LA, et al. Resolution of inflammation: state of the art, definitions and terms. FASEB J Off Publ Feder Am Soc Exp Biol. (2007) 21:325–32. doi: 10.1096/fj.06-7227rev
78. Chen, L, Deng, H, Cui, H, Fang, J, Zuo, Z, Deng, J, et al. Inflammatory responses and inflammation-associated diseases in organs. Oncotarget. (2018) 9:7204–18. doi: 10.18632/oncotarget.23208
79. Ahmed, AU. An overview of inflammation: mechanism and consequences. Front Biol. (2011) 6:274–81. doi: 10.1007/s11515-011-1123-9
80. Mizushina, Y, Kuriyama, I, Yamazaki, A, Akashi, T, and Yoshida, H. Cycloartenyl trans-ferulate, a component of the bran byproduct of sake-brewing rice, inhibits mammalian DNA polymerase and suppresses inflammation. Food Chem. (2013) 141:1000–7. doi: 10.1016/j.foodchem.2013.04.048
81. Akihisa, T, Yasukawa, K, Yamaura, M, Ukiya, M, Kimura, Y, Shimizu, N, et al. Triterpene alcohol and sterol ferulates from rice bran and their anti-inflammatory effects. J Agric Food Chem. (2000) 48:2313–9. doi: 10.1021/jf000135o
82. Frąk, W, Wojtasińska, A, Lisińska, W, Młynarska, E, Franczyk, B, and Rysz, J. Pathophysiology of cardiovascular diseases: new insights into molecular mechanisms of atherosclerosis, arterial hypertension, and coronary artery disease. Biomedicine. (2022) 10:1938. doi: 10.3390/biomedicines10081938
83. Nhung, BT, Tuyen, LD, Linh, VA, Nguyen, DV, Nga, TT, Thuc, VTM, et al. Rice bran extract reduces the risk of atherosclerosis in post-menopausal Vietnamese women. J Nutr Sci Vitaminol. (2016) 62:295–302. doi: 10.3177/jnsv.62.295
84. Revilla, E, Santa Maria, C, Miramontes, E, Bautista, J, García-Martínez, A, Cremades, O, et al. Nutraceutical composition, antioxidant activity and hypocholesterolemic effect of a water-soluble enzymatic extract from rice bran. Food Res Int. (2009) 42:387–93. doi: 10.1016/j.foodres.2009.01.010
85. Parrado, C, Mercado-Saenz, S, Perez-Davo, A, Gilaberte, Y, Gonzalez, S, and Juarranz, A. Environmental stressors on skin aging. Mechanistic insights. Front Pharmacol. (2019) 10:759. doi: 10.3389/fphar.2019.00759
86. Bennion, S., Structure and function of the skin. Dermatology Secrets E-Book; Elsevier: Amsterdam, The Netherlands (2020) 4690–4696.
87. Walters, K.A., and Roberts, M.S., The structure and function of skin, dermatological and transdermal formulations, CRC Press, (2002) 19–58.
88. Lee, C-J, Chen, L-G, Chang, T-L, Ke, W-M, Lo, Y-F, and Wang, C-C. The correlation between skin-care effects and phytochemical contents in Lamiaceae plants. Food Chem. (2011) 124:833–41. doi: 10.1016/j.foodchem.2010.07.003
89. Ribeiro, AS, Estanqueiro, M, Oliveira, MB, and Sousa Lobo, JM. Main benefits and applicability of plant extracts in skin care products. Cosmetics. (2015) 2:48–65. doi: 10.3390/cosmetics2020048
90. Yasukawa, K, Akihisa, T, Kimura, Y, Tamura, T, and Takido, M. Inhibitory effect of cycloartenol ferulate, a component of rice bran, on tumor promotion in two-stage carcinogenesis in mouse skin. Biol Pharm Bull. (1998) 21:1072–6. doi: 10.1248/bpb.21.1072
91. Vardhani, A, Jufri, M, and Purwaningsih, E. Potency of γ-Oryzanol rich black Rice bran (Oryza Sativa L. indica) extract for Tyrosinase inhibition. Int J Pharm Pharm Sci. (2020) 12:90–3. doi: 10.22159/ijpps.2020v12i5.37197
92. Kobayashi, M, Nakagawa, S, Nakamura, T, and Tsuno, T. Skin improvement effects of phytosterol ester derived from rice bran. Authorea Preprints. (2022)
93. Ellulu, MS, Patimah, I, Khaza’ai, H, Rahmat, A, and Abed, Y. Obesity and inflammation: the linking mechanism and the complications. Arch Med Sci. (2017) 4:851–63. doi: 10.5114/aoms.2016.58928
94. Fukuoka, D, Okahara, F, Hashizume, K, Yanagawa, K, Osaki, N, and Shimotoyodome, A. Triterpene alcohols and sterols from rice bran lower postprandial glucose-dependent insulinotropic polypeptide release and prevent diet-induced obesity in mice. J Appl Physiol. (2014) 117:1337–48. doi: 10.1152/japplphysiol.00268.2014
95. Hongu, N, Kitts, DD, Zawistowski, J, Dossett, CM, Kopeć, A, Pope, BT, et al. Pigmented rice bran and plant sterol combination reduces serum lipids in overweight and obese adults. J Am Coll Nutr. (2014) 33:231–8. doi: 10.1080/07315724.2013.869772
96. Panahi, Y, Darvishi, B, Jowzi, N, Beiraghdar, F, and Sahebkar, A. Chlorella vulgaris: a multifunctional dietary supplement with diverse medicinal properties. Curr Pharm Des. (2016) 22:164–73.
97. Moschos, MM, Dettoraki, M, Tsatsos, M, Kitsos, G, and Kalogeropoulos, C. Effect of carotenoids dietary supplementation on macular function in diabetic patients. Eye Visión. (2017) 4:23–6. doi: 10.1186/s40662-017-0088-4
98. Zheng, T, Bian, F, Chen, L, Wang, Q, and Jin, S. Beneficial effects of rhodiola and salidroside in diabetes: potential role of AMP-activated protein kinase. Mol Diagn Ther. (2019) 23:489–98. doi: 10.1007/s40291-019-00402-4
99. Prasathkumar, M, Becky, R, Anisha, S, Dhrisya, C, and Sadhasivam, S. Evaluation of hypoglycemic therapeutics and nutritional supplementation for type 2 diabetes mellitus management: an insight on molecular approaches. Biotechnol Lett. (2022) 44:203–38. doi: 10.1007/s10529-022-03232-3
100. Meng, X, Li, Q, Shi, R, Chang, J, Chang, H, and Li, M. Food supplements could be an effective improvement of diabetes mellitus: a review. J Future Foods. (2021) 1:67–81. doi: 10.1016/j.jfutfo.2021.09.003
101. Kaup, RM, Khayyal, MT, and Verspohl, EJ. Antidiabetic effects of a standardized Egyptian rice bran extract. Phytother Res. (2013) 27:264–71. doi: 10.1002/ptr.4705
102. Chen, C-W, and Cheng, H-H. A rice bran oil diet increases LDL-receptor and HMG-CoA reductase mRNA expressions and insulin sensitivity in rats with streptozotocin/nicotinamide-induced type 2 diabetes. J Nutr. (2006) 136:1472–6. doi: 10.1089/jmf.2006.9.290
103. Angeloni, C, Malaguti, M, Prata, C, Freschi, M, Barbalace, MC, and Hrelia, S. Mechanisms underlying neurodegenerative disorders and potential neuroprotective activity of agrifood by-products. Antioxidants. (2022) 12:94. doi: 10.3390/antiox12010094
104. Iriti, M, Vitalini, S, Fico, G, and Faoro, F. Neuroprotective herbs and foods from different traditional medicines and diets. Molecules. (2010) 15:3517–55. doi: 10.3390/molecules15053517
105. Zhang, J, Zhang, L, Wu, Z, Zhang, P, Liu, R, Chang, M, et al. The dopaminergic neuroprotective effects of different phytosterols identified in rice bran and rice bran oil. Food Funct. (2021) 12:10538–49. doi: 10.1039/D1FO01509E
106. de Lira Mota, KS, Dias, GEN, Pinto, MEF, Luiz-Ferreira, Â, Monteiro Souza-Brito, AR, Hiruma-Lima, CA, et al. Flavonoids with gastroprotective activity. Molecules. (2009) 14:979–1012. doi: 10.3390/molecules14030979
107. Kulshreshtha, M, Srivastava, G, and Singh, M. Pathophysiological status and nutritional therapy of peptic ulcer: an update. Environ Disease. (2017) 2:76–6. doi: 10.4103/ed.ed_11_17
108. Trinovita, E, Chany Saputri, F, and Mun’im, A. Potential gastroprotective activity of rice bran (Oryza sativa L.) extracted by ionic liquid-microwave-assisted extraction against ethanol-induced acute gastric ulcers in rat model. Sci Pharm. (2018) 86:35. doi: 10.3390/scipharm86030035
109. Tewtrakul, S, and Subhadhirasakul, S. Anti-allergic activity of some selected plants in the Zingiberaceae family. J Ethnopharmacol. (2007) 109:535–8. doi: 10.1016/j.jep.2006.08.010
110. Tewtrakul, S, Subhadhirasakul, S, and Kummee, S. Anti-allergic activity of compounds from Kaempferia parviflora. J Ethnopharmacol. (2008) 116:191–3. doi: 10.1016/j.jep.2007.10.042
111. Oka, T, Fujimoto, M, Nagasaka, R, Ushio, H, Hori, M, and Ozaki, H. Cycloartenyl ferulate, a component of rice bran oil-derived γ-oryzanol, attenuates mast cell degranulation. Phytomedicine. (2010) 17:152–6. doi: 10.1016/j.phymed.2009.05.013
112. Castanho, A, Lageiro, M, Calhelha, RC, Ferreira, IC, Sokovic, M, Cunha, LM, et al. Exploiting the bioactive properties of γ-oryzanol from bran of different exotic rice varieties. Food Funct. (2019) 10:2382–9. doi: 10.1039/C8FO02596G
113. Quintin, J, Cheng, S-C, van Der Meer, JW, and Netea, MG. Innate immune memory: towards a better understanding of host defense mechanisms. Curr Opin Immunol. (2014) 29:1–7. doi: 10.1016/j.coi.2014.02.006
114. Shin, SY, Kim, H-W, Jang, H-H, Hwang, Y-J, Choe, J-S, Lim, Y, et al. γ-oryzanol-rich black rice bran extract enhances the innate immune response. J Med Food. (2017) 20:855–63. doi: 10.1089/jmf.2017.3966
Keywords: rice bran, plant terpenoids, phytosterols, health benefits, processing technology, stability evaluation
Citation: Liu Z, Liu X, Ma Z and Guan T (2023) Phytosterols in rice bran and their health benefits. Front. Nutr. 10:1287405. doi: 10.3389/fnut.2023.1287405
Received: 01 September 2023; Accepted: 02 October 2023;
Published: 12 October 2023.
Edited by:
Zhengyi Wei, Guangxi Academy of Agricultural Sciences, ChinaReviewed by:
Fangwei Yang, Nanjing Forestry University, ChinaCopyright © 2023 Liu, Liu, Ma and Guan. This is an open-access article distributed under the terms of the Creative Commons Attribution License (CC BY). The use, distribution or reproduction in other forums is permitted, provided the original author(s) and the copyright owner(s) are credited and that the original publication in this journal is cited, in accordance with accepted academic practice. No use, distribution or reproduction is permitted which does not comply with these terms.
*Correspondence: Zheng Ma, MjAxODYyMDA4NTQyQGVtYWlsLnNkdS5lZHUuY24=; Tianzhu Guan, Z3VhbnR6QHl6dS5lZHUuY24=
Disclaimer: All claims expressed in this article are solely those of the authors and do not necessarily represent those of their affiliated organizations, or those of the publisher, the editors and the reviewers. Any product that may be evaluated in this article or claim that may be made by its manufacturer is not guaranteed or endorsed by the publisher.
Research integrity at Frontiers
Learn more about the work of our research integrity team to safeguard the quality of each article we publish.