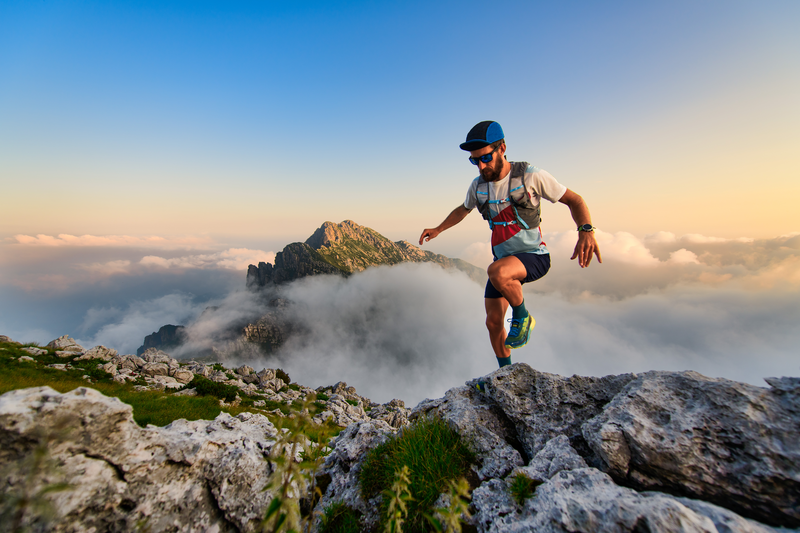
94% of researchers rate our articles as excellent or good
Learn more about the work of our research integrity team to safeguard the quality of each article we publish.
Find out more
ORIGINAL RESEARCH article
Front. Nutr. , 17 November 2023
Sec. Nutrition and Food Science Technology
Volume 10 - 2023 | https://doi.org/10.3389/fnut.2023.1283086
Idesia polycarpa Maxim protein was used as a substrate to prepare a novel food packaging material with bioactive functions for encapsulating and extending the postharvest shelf life of sweet cherries. The film-forming solution was prepared from a mixture of Idesia polycarpa Maxim protein, glycerol, and gelatin, and was cast to form a film at room temperature and evaluated for mechanical, optical, structural, crystallinity, thermal properties, morphology, and antioxidant activity. Idesia polycarpa Maxim protein composite film solution was applied as an edible coating on sweet cherries and evaluated for changes in physical and biochemical parameters of sweet cherries in storage at 20°C and 50% relative humidity for 9 days. The results showed that the film tensile strength increased from 0.589 to 1.981 Mpa and the elongation at break increased from 42.555% to 58.386% with the increase of Idesia polycarpa Maxim protein concentration. And in the in vitro antioxidant assay, IPPF-4.0% was found to have the best antioxidant activity, with scavenging rates of 65.11% ± 1.19%, 70.74% ± 0.12%, and 90.96% ± 0.49% for DPPH radicals, ABTS radicals, and hydroxyl radicals, respectively. Idesia polycarpa Maxim protein coating applied to sweet cherries and after storage at 20°C and 50% relative humidity for 9 days, it was found that the Idesia polycarpa Maxim protein coating significantly reduced the weight loss (54.82% and 34.91% in the Control and Coating-2.5% groups, respectively) and the loss of ascorbic acid content (16.47% and 37.14% in the Control and Coating-2.5% groups, respectively) of the sweet cherries, which can effectively extend the aging of sweet cherry fruits and prolong their shelf life. The developed protein film of Idesia polycarpa Maxim with antioxidant activity can be used as a new food packaging material in the food industry.
Sweet cherries are produced in Western Europe and are the source species for most table cherries, their fruit is sweet and tart and can be eaten fresh or made into jams, wines, and canned goods. The bright red color of European sweet cherries is due to iron ions, but also to the richness of polyphenolic antioxidants such as anthocyanins, proanthocyanidins, lutein, and zeaxanthin, which fight cell damage, reduce inflammation, and act as antioxidants, anti-aging, prevent chronic diseases and promote overall health (1). European sweet cherries are a better choice for people with diabetes and hypertension due to their low sugar content, glycemic index of 22, low calorie content, and no sodium (2, 3). European sweet cherries are seasonal fruit, picked when ripe in May or June to obtain more nutrition, color, and flavor. Sweet cherries can be stored for 5–7 days and are severely damaged by microbial infestation, spoilage due to microbial multiplication, and water loss during growth, harvest, and storage (4–6). The use of food packaging to extend the shelf life of European sweet cherries is particularly critical.
At present, the preservation technology of sweet cherries mainly adopts the traditional low-temperature packaging method, which can effectively inhibit the growth of microorganisms to delay the aging and spoilage of sweet cherries (7). Although low-temperature packaging extends the shelf life of sweet cherries, but the cost is too high, and the collision between the fruits in the process of transportation will also cause some damage (8, 9). In recent years, the proposed edible active packaging method has effectively solved this problem (10). Edible packaging typically uses sustainable, biodegradable materials that are wrapped or coated around food products as consumables and do not generate waste (11). Edible coatings can be safely consumed and retain the taste and appearance of fresh fruit, and the coatings have some nutritional value (12). In addition, they should act as an effective semi-permeable barrier between the surrounding atmosphere and the fruit, blocking water vapor and respiratory gases (13). Biocoatings control physical and biochemical changes in the fruit during long-term storage by reducing the rate of respiration and metabolic reactions and by modifying the internal atmosphere (14). Not only are biopolymers biodegradable, but the raw materials are renewable resources (15). Natural edible polymers generally include polysaccharides, lipids, and proteins (16). Proteins are widely used for the production of biodegradable films due to their excellent film-forming ability. Compared to films made from polysaccharides and lipids, protein-based films have better physical, nutritional, and functional activities (17). For example, edible film or coating treatments preserved the post-harvest quality of sweet cherries by minimizing weight loss and ascorbic acid loss, inhibiting fungal growth, and maintaining the firmness of the coated fruit (18, 19). Milk protein concentrate-based coatings successfully delayed the darkening, shriveling, and softening of the cherries (20). The edible film coating acts as a barrier, which can inhibit gas exchange, reduce respiration rates, and slow down fruit decay (21). Nigella sativa protein concentrate films by using grape juice have been applied to preserve the freshness of sweet cherries (22). The protein-based film improved the antimicrobial and antioxidant properties of the film by adding grape juice to it. Fungal growth was inhibited and the shelf life of fresh sweet cherries was extended (23). Edible Carboxymethyl Chitosan-Gelatin based coating was beneficial in delaying the decay of sweet cherries. After 33 days of storage, the fruit decay rate of the coated group only ranged from 3.0% to 15.3%, while that of the control group reached 17.7%–63.0% (24). It was shown that the protein-based edible coating can reduce the respiration and transpiration rates of sweet cherries, minimize firmness loss, and reduce fruit decay (25). Yuan et al. (26) found that the use of multifunctional electrostatically spun nanofibre films loaded with protein-based biopolymers for packaging sweet cherries effectively delayed fruit senescence and maintained postharvest quality. However, due to the slow speed of nanopreparation by electrostatic spinning and the high economic cost of raw material preparation, it is not suitable for large-scale production and application. Therefore, it is crucial to find a biodegradable, sustainable, inexpensive packaging material with a simple preparation process to prolong the freshness of sweet cherries.
Idesia polycarpa cake meal is a by-product of Idesia polycarpa oil extraction in which Idesia polycarpa protein is the main nutrient. According to the preliminary experimental research in our laboratory, Idesia polycarpa fruit pomace is also rich in protein, and the preliminary in vitro antioxidant experiments have shown that Idesia polycarpa protein has excellent antioxidant functions. A composite film with antioxidant function was prepared by using Idesia polycarpa protein and the film was formed on the surface of European sweet cherry by a coating method. The mechanical properties, optical properties, and antioxidant activity of Idesia polycarpa protein composite films were also investigated. The biochemical properties of the Idesia polycarpa protein composite films were also studied for changes in the storage of European sweet cherries packaged with Idesia polycarpa protein composite films (9 d, 20°C), and the effect of Idesia polycarpa protein composite films on the shelf-life of European sweet cherries was investigated.
Idesia polycarpa was sourced from Enshi, Hubei, China, and the Idesia polycarpa cake meal used in the study was sourced from the by-products of Idesia polycarpa oil extraction. Ripe and fresh sweet cherries were obtained from Spain. Hexane and sodium hydroxide were analytical reagent grade and were purchased from Tianjin Hengxing Chemical Reagent Manufacturing Co., Ltd. Gelatin was purchased from Aladdin Reagent Database Inc. (Shanghai, China). Glycerol was purchased from Taiko Palm-Oleo (Zhangjiagang) Co., Ltd.
The protein was prepared according to the method of Das et al. (27) with some modifications. The Idesia polycarpa cake meal was crushed and passed through an 80 mesh sieve, then the Idesia polycarpa cake meal flour was defatted with n-hexane and finally air dried in a fume hood for 48 h to obtain defatted Idesia polycarpa cake meal flour. The defatted Idesia polycarpa cake meal flour was mixed with 0.06 mol/L NaOH solution in a material-liquid ratio of 1:55. The mixture was heated to 53°C using a magnetic stirrer (DF-101S, China) and then stirred for 2 h. The supernatant was collected by centrifugation (3,578×g, 10 min). The pH of the supernatant was adjusted to 3.2 with 1 mol/L HCl. After 2 h at room temperature, the precipitate was collected by centrifugation (3,578×g, 10 min), washed with distilled water to neutral, and dried in a freeze dryer (SCIENTZ-100F/A, China) for 24 h to obtain the Idesia polycarpa protein.
Idesia polycarpa protein (IPP) was dissolved in distilled water (2.5%, 3.0%, 3.5%, and 4%), the pH of the solution was adjusted to 12 with 2 mol/L NaOH, 30% (v/v) glycerol was added and stirred at 40°C for 1 h under magnetic stirring to mix well. The temperature was raised to 70°C and 0.25% (w/v) gelatin was added and stirred for 20 min. After the solution was cooled to room temperature, 8 mL of film-forming solution was evenly distributed on disposable Petri dishes (100 mm). The disposable Petri dishes were placed in an oven at 25°C for 24 h and then the films were removed (28). The composite films with concentrations of 2.5%, 3%, 3.5%, and 4% were recorded as IPPF-2.5%, IPPF-3.0%, IPPF-3.5% and IPPF-4.0%, respectively.
Film thickness was measured with a micrometer. The thickness of the film samples was determined by averaging six random measurements.
The tensile strength (TS) and elongation at break (EAB) of the film were tested with the help of a computerized tensile tester (DR-508A, China). The film was cut into long strips of 10 mm × 60 mm, equilibrated at 25°C and 30% relative humidity for 24 h and fixed on the tensile tester, stretched at a rate of 15 mm/min with an initial length of 35 mm, and the tensile strength and elongation at break were calculated according to the following equations.
Where F is the maximum tensile force that the film can withstand, d is the thickness of the film, and b is the width of the film.
where L is the length of the film when it breaks and L0 is the initial length of the film.
The moisture content of the film is tested by a moisture tester (HE53/02, China). 0.5–1 g of the film was placed in the moisture tester for testing.
Water vapor permeability was measured according to the method of Zhang et al. (29) with some modifications. 10 mL of distilled water was added to a permeable cup with an inner diameter of 6 cm, and the permeable cup was covered with a 7 cm × 7 cm film sample. The change in weight of the distilled water in the permeable cup was recorded after 24 h at 25°C and 30% relative humidity.
The color parameters (L*, a*, and b*) of six random points of the film samples were measured using a colorimeter (CS-820 N, China). The total color difference (∆E) and the whiteness index (WI) of the film were calculated using the following equation.
The opacity of the film was measured using a UV spectrophotometer (UV-8000H, China). The film was cut into rectangles (1 cm × 5 cm) and the absorbance at 600 nm was measured by fixing the film on the surface of the cuvette and an empty cuvette was used as a blank control.
where A600 is the film sample absorbance at 600 nm and t is the film sample thickness (mm).
FTIR spectra of the film were collected using an FTIR spectrometer (BRUKER, America) in the wave number range of 4,000–500 cm−1.
The samples were scanned from 5° to 70° at 2°/min with Cu Kα source at 40 kV and 40 mA by X-ray diffractometer (Bruker D8 advance, Germany).
DSC measurements (Netzsch DSC214, Germany) were performed on the film samples using a differential scanning calorimeter. Nitrogen gas was flushed at a rate of 20 mL/min (as cooling gas). The film samples (5–10 mg) were sealed in an aluminum tray and heated from 30°C to 200°C at a rate of 10°C/min.
The morphology of the film sample was imaged by SEM (TESCAN MIRA LMS, Czech Republic), and the morphology was recorded with an accelerating voltage of 3 kV. The sample was fractured in liquid nitrogen and the fracture surface was observed after gold sputtering.
The antioxidant activity of the Idesia polycarpa protein film was determined by immersing 100 mg of film samples in 10 mL of distilled water and stirring until complete dissolution.
Referring to the method of Maryam Adilah and Nur Hanani (30) with slight modifications. DPPH solution (0.1 mmol/L) was prepared in ethanol. 1 mL of film sample solution was mixed well with 3 mL of DPPH solution and the reaction was carried out at room temperature for 30 min protected from light, and the absorbance was measured at 517 nm as A1. The sample control group was substituted with distilled water for the DPPH solution and the absorbance value was measured as A2; the blank group was substituted with distilled water for the sample solution and the absorbance value was measured as A0; and the DPPH scavenging activity was obtained using the following equation.
According to the method of Moghadam et al. (31) with slight modifications. The aqueous ABTS solution (7 mmol/L) was mixed with an equal volume of potassium persulfate solution (2.45 mmol/L), placed in a refrigerator at 4°C for 16 h, and diluted with distilled water to an absorbance of 0.7 ± 0.02 at use. 0.2 mL of film sample solution was added to 4 mL of ABTS dilution solution, protected from light, and reacted for 6 min, and the absorbance value at 734 nm was measured as A1; the sample control group was treated with distilled water instead of ABTS solution and the absorbance was measured as A2; the blank group was measured as A0 with distilled water instead of film sample solution, and the ABTS scavenging activity was obtained using the following equation.
The method of Zhang et al. (32) was used with slight modifications. 1 mL of FeSO4 solution with a concentration of 6 mmol/L, 1 mL of salicylic acid solution with a concentration of 6 mmol/L, and 1 mL of sample solution were added to the test tube. After mixing, 1 mL of H2O2 solution with a concentration of 6 mmol/L was added. After mixing again, the reaction was carried out at 37°C for 30 min and the absorbance of the solution was measured at 510 nm as A1. The absorbance was measured as A2 for the sample control group using distilled water instead of H2O2 solution and as A0 for the blank group using distilled water instead of sample solution. The hydroxyl radical scavenging capacity was calculated as follows.
Sweet cherries were coated with reference to the method of Basiak et al. (33). Sweet cherries of uniform size and without mechanical damage were selected for experimental work. The sweet cherries were soaked in Idesia polycarpa protein film solution for 1 min and air-dried intermittently for 10 min, with three soaking times. The coating was formed by allowing the film solution on the surface of the sweet cherries to air dry. The effect of two different concentrations (2.5% and 4%) of Idesia polycarpa protein film solution on sweet cherries was evaluated and recorded as Coating-2.5% and Coating-4.0%. The untreated sweet cherries were recorded as the control group as Control. All fruits were placed in storage at 20°C and 50% humidity.
The weights of the control and coated sweet cherries were recorded daily. Differential weight loss was calculated and converted to percentages.
5 g of sweet cherry pulp was homogenized in distilled water (50 mL) and filtered. The pH of the filtrate was measured with a pH meter.
The TA of the samples was evaluated according to the method of Colussi et al. (34) with slight modifications. 5 g of the samples were stirred in distilled water (50 mL) at 80°C for 2 h and filtered. Then 20 mL of the filtrate was titrated with 0.1 mol/L NaOH solution to pH 8.1. TA was expressed as grams of citric acid per 100 g of sample weight and was determined as follows
where V(NaOH) is the volume of titrated NaOH(mL), 0.1 is the molar concentration of NaOH solution, 0.064 is the conversion factor of citric acid, and m(g) is the mass of the aliquot.
Total soluble sugar content was determined according to the method of Gharibzahedi et al. (35) with some modifications. 5 g of sweet cherry pulp was pounded in distilled water (50 mL), stirred for 2 h at 80°C, and filtered. 1 mL of filtrate, 1 mL of phenol solution (5%), and 5 mL of H2SO4 were taken in a test tube and mixed well and put the test tube into a 90°C water bath for 30 min reactions, and then the absorbance was measured at 490 nm using a UV spectrophotometer with distilled water as a blank. The regression equation used was y = 0.0086 x + 0.1412 (R2 = 0.9987).
The content of ascorbic acid was enumerated by using 2,6-dichlorophenol indophenol (DCPIP) dye (36). Sweet cherry pulp (1 g) was mashed in 10 mL of HPO3 (2%) and mixed with gentle shaking in the dark for 30 min. The supernatant was collected by centrifugation (3,578×g, 10 min). The supernatant (350 μL) was diluted 1:10 times with 2% HPO3 and mixed with DCPIP dye (650 μL), incubated in the dark for 3 min and the absorbance was measured at 518 nm with 80% ethanol as a blank. The regression equation used was y = −0.0946 x + 1.434 (R2 = 0.9966) and was correlated with absorbance (x) and ascorbic acid concentration (y).
All experiments were performed in triplicate (unless otherwise stated), and data in the text are reported as mean ± standard deviation and statistically evaluated using the OriginPro 2023 one-way analysis of variance (ANOVA) test and accepted as statistically significant at p < 0.05.
Mechanical properties are one of the criteria for assessing whether a film can be used as a food packaging material, and the film or coating material can act as a protective layer for the food, reducing the risk of mechanical damage to the food. The physicochemical properties of Idesia polycarpa protein films are shown in Table 1, where we can find that the thickness, TS, and EAB of the films increased with the increase in protein concentration. Also with increasing protein concentration WVP decreased from 9.26% ± 0.09% to 8.44% ± 0.09%, which indicates that the increase in protein concentration favors the decrease in WVP of the films. This could be because the tighter structures formed by the larger concentration of protein solutions during film formation block the passage of water molecules, which is consistent with the results reported by Bishnoi et al. (37) in wheat protein-alginate composite films. This result is also supported by the detection of film moisture content, which increased with increasing protein concentration, with 2.367% for IPPF-2.5% and 4.950% for IPPF-4.0%. As the protein concentration increases the film structure becomes more compact resulting in a slower evaporation of moisture, which is beneficial for applications in the food packaging industry.
Table 1. Thickness, tensile strength, elongation at break, moisture content, and water vapor permeability of films.
The overall brown color of the film was mainly due to the richness of natural pigments in Idesia polycarpa. In contrast, the Idesia polycarpa cake meal was not decolorized during the protein extraction process. The results of the optical properties of the films are shown in Table 2. The total color difference and whiteness index of the films showed a decreasing trend with increasing protein concentration. While opacity is positively correlated with protein concentration, this is because the introduction of pigment increases with protein concentration. The lightest brown color L* was 29.278 ± 0.365, a* was 4.210 ± 0.185, and b* was 6.645 ± 0.482 for the IPPF-2.5% films. The darkest brown color L* was 26.452 ± 0.116, a* was 3.028 ± 0.119, and b* was 2.455 ± 0.150 for the IPPF-4.0% films. This is because the natural color of Idesia polycarpa proteins is brown, so the color of the film will gradually darken with increasing protein content. This brown shadeable film and the film with high opacity are suitable for storing photosensitive foods (38). For some photosensitive foods, such films can block specific wavelengths of light and can effectively prevent the coated foods from oxidation reactions (39, 40).
The FTIR spectra of the films prepared from four different concentrations of Idesia polycarpa proteins are shown in Figure 1A, from which it can be clearly observed that the curves of the films with four different concentrations of Idesia polycarpa proteins are basically the same. Significant protein characteristic peaks can also be observed, with absorption bands at 1,653 cm−1 and 1,530 cm−1 for protein amide I and amide II bands, respectively (41). 1,447 cm−1 is the absorption peak for C-H stretching vibration and N-H bending vibration (amide III) (42). The FTIR spectra of CH at 3,253 cm−1 shows typical absorption bands associated with -OH groups and -NH2 groups associated with typical absorption bands (43). The absorption peak at 2,930 cm−1 is mainly the asymmetric stretching of the C-H bond in the CH3 group, and the absorption peak at 1,022 cm−1 is mainly the absorption peak of -C-O-C- in the glycosidic bond (44, 45). This phenomenon was attributed to the addition of gelatin to the membrane solution during the fabrication of the Idesia polycarpa protein membrane to obtain better mechanical properties, so the characteristic peaks of polysaccharides appeared in the infrared spectra of the films (46). According to Figure 1A, the four different concentrations of Idesia polycarpa protein complex membranes showed significant absorption peaks between 1,600 cm−1 and 1,700 cm−1, which is a region commonly used to study the secondary structure of amide I (47). The four different concentrations of Idesia polycarpa protein complex membranes showed obvious absorption peaks at 1,650 cm−1, which is mainly due to the strength of hydrogen bond between C=O and N-H bonds, indicating that Idesia polycarpa protein is mainly α-helical structure (48).
Figure 1. FTIR spectra (A) and X-ray diffractograms patterns (B) of IPPF-2.5%, IPPF-3.0%, IPPF-3.5%, and IPPF-4.0% films.
Figure 1B shows the X-ray diffraction patterns of the films prepared from different concentrations of Idesia polycarpa protein. For the thin film samples with various protein concentrations, it is evident from Figure 1B that characteristic peaks are visible around 2θ = 20°, which we analyzed to be primarily induced by the amorphous phase of the proteins, showing that the majority of the structures in all samples are amorphous. This is consistent with the results of Mir et al. (49) and Sarıcaoğlu and Turhan (50). Therefore, the XRD results showed that the Idesia polycarpa protein and glycerol were well-compatible in the preparation of edible membranes.
A differential scanning calorimeter measures the change in the heat flow rate of a sample. The relationship between the energy difference between the substance and the reference during the heating process and the temperature is measured. The DSC thermograms of the films treated with different Idesia polycarpa protein contents are shown in Figure 2, with a clear exothermic peak observed at 110–185°C, which is caused by the thermal degradation of the films (51). The heat flow rate of the films decreased with the increase of Idesia polycarpa protein concentration. The peak areas of 37.15 J/g, 67.45 J/g, 85.62 J/g, and 91.59 J/g for IPPF-2.5%, IPPF-3.0%, IPPF-3.5%, and IPPF-4.0% films, respectively, were positively correlated with the Idesia polycarpa protein concentration, which may be due to the higher hydrophilicity of Idesia polycarpa protein after denaturation (52). This resulted in a higher water binding capacity of the Idesia polycarpa protein film, which is consistent with the results of water content measurement.
Figure 2. Differential scanning calorimetric curves for IPPF-2.5% (A), IPPF-3.0% (B), IPPF-3.5% (C), and IPPF-4.0% (D) films.
Scanning electron microscopy (SEM) was used to reveal the morphological properties, internal structure, and interactions among the constituents of the edible films. SEM images of the films containing different concentrations of Idesia polycarpa proteins are shown in Figure 3. It is obvious from Figure 3 that the IPPF-2.5% film and IPPF-3.0% film are smoother and more uniform, which indicates that the protein can be well integrated with glycerol. While IPPF-3.5% and IPPF-4.0% films were relatively rough and appeared fine particles, which was related to the solubility of the protein. As the protein concentration increased, the viscosity of the film-forming solution also increased, and the protein became increasingly difficult to dissolve. The appearance of fine particles may be due to incomplete dissolution. A similar situation was found in the films prepared from Fenugreek Protein (53).
Figure 3. Scanning electron micrographs of IPPF-2.5% (A), IPPF-3.0% (B), IPPF-3.5% (C), and IPPF-4.0% (D) film samples.
Antioxidant packaging is one of the main types of active packaging that can extend the shelf life of food products. Figure 4 shows that Idesia polycarpa protein films have strong antioxidant activity. When the concentration was 10 mg/mL, the DPPH radical scavenging rate was 31.67% ± 0.24%, 39.74% ± 0.67%, 50.59% ± 0.23%, and 65.11% ± 1.19% for IPPF-2.5%, IPPF-3.0%, IPPF-3.5%, and IPPF-4.0%, respectively, and the ABTS radical scavenging rate was 47.31% ± 0.52%, 54.20% ± 0.83%, 57.79% ± 0.74% and 70.74% ± 0.12%, and hydroxyl radical scavenging rates were 67.47% ± 0.23%, 75.85% ± 0.41%, 78.44% ± 0.25%, and 90.96% ± 0.49%, respectively. The antioxidant activity of the film depends on the content of the Idesia polycarpa protein. The higher the protein concentration, the higher the antioxidant activity of the protein film. The protein content is positively correlated with the antioxidant activity of the protein film. According to the results of the team’s previous research, Idesia polycarpa protein has good antioxidant function and its hydrolyzed products are mainly aspartic acid and glutamic acid, which have good antioxidant activity (54). For example, Lu et al. (55) found that sesame protein hydrolysis products are mainly composed of aspartic acid and glutamic acid, which is associated with their significant antioxidant activity. This result is similar to the results of this paper. This phenomenon was attributed to the good antioxidant property of Idesia polycarpa protein membrane is that Idesia polycarpa protein is insoluble in water under neutral conditions and soluble in water under alkaline conditions. Hydrolysis of Idesia polycarpa protein under alkaline conditions produces aspartic acid and glutamic acid, two amino acids that promote peroxidase activity or directly scavenge free radicals (56). This leads to good antioxidant activity of the Idesia polycarpa protein membrane. When it is used in functional packaging materials, it can effectively slow down the rate of oxidative aging of packaging products.
Figure 4. DPPH, ABTS, and hydroxyl radical scavenging capacity of IPPF-2.5%, IPPF-3.0%, IPPF-3.5%, and IPPF-4.0% films.
The appearance and color of the fruit is an important fruit quality parameter that affects its marketability and consumer acceptability. In general, the shade of color represents the maturity of the fruit. During the whole storage period, the appearance of the fruit changed as shown in Figure 5. On day 5, it was observed that the sweet cherries in the Control group turned dark red in appearance, while the sweet cherries in the coated group had a bright red surface. The sweet cherries in the Control group aged faster than those in the coating group, and those in the Coating-2.5% group aged the slowest. The viscosity of the Coating suspension was critical in the dip coating process of the sweet cherries, and the Coating-4.0% suspension was very viscous, which prevented the dip coating process from achieving uniform film thickness, which would explain the fact that the Coating-2.5% group had a more uniform film thickness compared to the Coating-4.0% group has a better preservation function compared to the Coating-4.0% group.
Figure 5. Changes in the appearance of sweet cherries untreated (Control), coated with 2.5% protein film solution (Coating-2.5%), and coated with 4.0% protein film solution (Coating-4.0%) during 9 days of storage at 20°C and 50% relative humidity.
The weight loss of sweet cherries in different treatment groups during storage is shown in Figure 6A, where all sweet cherry samples showed continuous weight loss during storage. At the end of the storage period (9 d), the weight loss was 54.82%, 34.91%, and 45.64% for the Control, Coating-2.5%, and Coating-4.0% coating groups, respectively. The Coating-2.5% coating treatment significantly retarded the weight loss of sweet cherries compared to the Control (p < 0.05). Weight loss of fruits during storage is mainly due to water loss due to respiration and transpiration of fruits (57). The barrier formed by the edible coating on the fruit surface between the fruit and the external environment was able to reduce gas exchange and water loss, which was closely related to the lower water vapor permeability of the Idesia polycarpa protein composite membrane (58). At the same time, we found that the WVP of the Idesia polycarpa protein composite film with a concentration of 4.0% was lower than that of the Idesia polycarpa protein composite film with a concentration of 2.5% in the process of film manufacture by plate spreading. We have analyzed that the main reason for this opposite phenomenon is due to the different ways of spreading the film. When the film is spread on a flat plate, the film liquid does not flow in the plate, so the viscosity of the film liquid has less influence on the film-forming effect. While the infiltration film deposition method was used in the study of weight loss of sweet cherries during storage, in this method of film deposition, due to gravity affecting the flow of film liquid on the surface of sweet cherries, and the concentration of 4.0% Idesia polycarpa protein composite film liquid was more viscous, and the film deposition was not uniform. This resulted in greater weight loss in the 4.0% coating group.
Figure 6. Changes in weight loss (A), pH (B), Titratable acidity (C), Total soluble sugars (D), and Ascorbic acid (E) content of untreated (Control), coated with 2.5% protein film solution (Coating-2.5%) and coated with 4.0% protein film solution (Coating-4.0%) of sweet cherries during 9 days of storage at 20°C and 50% relative humidity.
The pH, the titratable acidity of the fruit is an important quality parameter that reflects the ripeness of the fruit. The main organic acids found in sweet cherries are malic and citric acid, which are suitable for the acidity, taste, and flavor of the fruit. In this study, it was observed that regardless of the treatment done on sweet cherries, the pH values of sweet cherries were significantly higher and TA values (Figures 6B,C) were significantly lower (p < 0.05) throughout the storage period (9 d). At the end of the storage period at 20°C and 50% RH, the sweet cherries in the Control group had the highest pH (4.5), while the sweet cherries in the Coating-2.5% group had the lowest pH (4.3). This phenomenon can be explained by the fact that the high respiration rate of sweet cherries after harvesting converts starch and acid to sugar during storage, leading to an increase in sweet cherry pH (59). In contrast, the excellent barrier properties of the fruit coating reduced the amount of oxygen entering the fruit, effectively reducing the respiration rate, enzyme activity, and organic acid consumption of sweet cherries and delaying the decrease in acidity (60). TA values of sweet cherry samples increased significantly with pH (p < 0.05). The lowest TA value (0.41%) was observed in the control sweet cherries after 9 d of storage at 20°C and 50% RH. At this time, sweet cherry TA values in the Coating-2.5% and Coating-4.0% groups were 0.51% and 0.46%, respectively. As the sweet cherry fruit ripened and decayed, organic acids gradually decomposed into sugars, leading to a decrease in TA.
TSS is a parameter that measures fruit senescence, and the increase in respiration during senescence increases the TSS of the fruit, which means that the increase in TSS can be used as a proxy for the senescence process. The initial metabolic process in fruits converts carbohydrates into sugars and other soluble compounds, leading to the accumulation of TSS during storage (61). As shown in Figure 6D, the content of total soluble sugars in sweet cherries increased with storage time during storage at 20°C and 9 d as a result of hydrolysis of starch and other carbohydrates in sweet cherries to produce monosaccharides (e.g., sucrose, glucose, fructose, etc.). During 9 days of storage, the TSS content was 319.19 ± 5.00, 243.23 ± 3.94, and 264.97 ± 2.45 mg/g for Control, Coating-2.5% and Coating-4.0%, respectively. The key reason for this phenomenon was the barrier effect of the coating, resulting in its lower metabolic rate and starch hydrolysis rate processes.
Ascorbic acid is a water-soluble vitamin that decreases with the evaporation of water during storage. The different treatment groups had a significant effect (p < 0.05) on the change of ascorbic acid content (Figure 6E) in sweet cherries. The results showed that the ascorbic acid content of the Control, Coating-2.5%, and Coating-4.0% groups were 16.47%, 37.14%, and 23.96%, respectively, after 9 days of storage period. This was attributed to the effectiveness of coating in reducing the rate of fruit respiration, enzyme activity, and evaporation of water vapor, thus reducing the loss of ascorbic acid content (62). Meanwhile, Yousuf et al. (63) made similar findings in coating experiments on fresh-cut melons using fruit extracts and hydrocolloids. This finding can effectively delay the rate of fruit deterioration and increase the commercial value of the fruit.
The feasibility of using Idesia polycarpa Maxim protein as a substrate for the preparation of edible films was demonstrated by the solution casting method. Idesia polycarpa Maxim protein composite film with good mechanical properties, barrier properties, and antioxidant activity. After storing sweet cherries at 20°C and 50% relative humidity for 9 days, the Idesia polycarpa Maxim protein-based bioactive coatings were found to exert beneficial effects on the shelf-life parameters of sweet cherries, with the best preservation results in the Coating-2.5% group. Extend the shelf life of sweet cherries by maintaining their color appearance, reducing weight loss, and decreasing the loss of ascorbic acid content. In addition, despite the high antioxidant activity of the designed films, Coating-2.5% showed superior freshness preservation than Coating-4.0% when it was applied to sweet cherries. This is related to the manner of coating, which is susceptible to the effect of solution viscosity when coating is performed by infiltration, resulting in non-uniform coating. However, all the coated groups showed better freshness retention than the control group. Therefore, Idesia polycarpa Maxim protein composite film is a good biofunctional food packaging material, which is also beneficial for direct use as fruit food packaging, which will greatly exploit the value of the utilization of Idesia polycarpa by-products.
The original contributions presented in the study are included in the article/supplementary materials, further inquiries can be directed to the corresponding author.
WY: Conceptualization, Investigation, Writing – review & editing, Data curation, Methodology, Software, Validation, Visualization, Writing – original draft. ZZ: Conceptualization, Data curation, Investigation, Methodology, Validation, Visualization, Writing – original draft, Writing – review & editing, Supervision. YC: Investigation, Supervision, Writing – review & editing, Formal analysis, Project administration, Resources. KL: Formal analysis, Investigation, Project administration, Resources, Supervision, Conceptualization, Funding acquisition, Writing – review & editing.
The author(s) declare financial support was received for the research, authorship, and/or publication of this article. The authors would like to thank the financial support from the Natural Science Foundation of Hubei Province (2023AFD080); the National Forestry and Grassland Administration 2021 soft science projects of China (2021131009); the Postgraduate Innovation Project of Hubei Minzu University (MYK2023035).
The authors declare that the research was conducted in the absence of any commercial or financial relationships that could be construed as a potential conflict of interest.
All claims expressed in this article are solely those of the authors and do not necessarily represent those of their affiliated organizations, or those of the publisher, the editors and the reviewers. Any product that may be evaluated in this article, or claim that may be made by its manufacturer, is not guaranteed or endorsed by the publisher.
1. Chockchaisawasdee, S, Golding, JB, Vuong, QV, Papoutsis, K, and Stathopoulos, CE. Sweet cherry: composition, postharvest preservation, processing and trends for its future use. Trends Food Sci Technol. (2016) 55:72–83. doi: 10.1016/j.tifs.2016.07.002
2. Papapetros, S, Louppis, A, Kosma, I, Kontakos, S, Badeka, A, Papastephanou, C, et al. Physicochemical, spectroscopic and chromatographic analyses in combination with chemometrics for the discrimination of four sweet cherry cultivars grown in northern Greece. Foods. (2019) 8:442. doi: 10.3390/foods8100442
3. Ricardo-Rodrigues, S, Laranjo, M, and Agulheiro-Santos, AC. Methods for quality evaluation of sweet cherry. J Sci Food Agric. (2023) 103:463–78. doi: 10.1002/jsfa.12144
4. Aglar, E, Ozturk, B, Guler, SK, Karakaya, O, Uzun, S, and Saracoglu, O. Effect of modified atmosphere packaging and ‘parka’ treatments on fruit quality characteristics of sweet cherry fruits (Prunus avium L. ‘0900 Ziraat’) during cold storage and shelf life. Sci Hortic. (2017) 222:162–8. doi: 10.1016/j.scienta.2017.05.024
5. Zehra, A, Wani, SM, Bhat, TA, Jan, N, Hussain, SZ, and Naik, HR. Preparation of a biodegradable chitosan packaging film based on zinc oxide, calcium chloride, nano clay and poly ethylene glycol incorporated with thyme oil for shelf-life prolongation of sweet cherry. Int J Biol Macromol. (2022) 217:572–82. doi: 10.1016/j.ijbiomac.2022.07.013
6. Zhang, C, Gong, H, and Liu, Y. Effects of postharvest coating using chitosan combined with natamycin on physicochemical and microbial properties of sweet cherry during cold storage. Int J Biol Macromol. (2022) 214:1–9. doi: 10.1016/j.ijbiomac.2022.06.057
7. Correia, S, Schouten, R, Silva, AP, and Gonçalves, B. Factors affecting quality and health promoting compounds during growth and postharvest life of sweet cherry (Prunus avium L.). Front Plant Sci. (2017) 8:2166. doi: 10.3389/fpls.2017.02166
8. Remón, S, Ferrer, A, Marquina, P, Burgos, J, and Oria, R. Use of modified atmospheres to prolong the postharvest life of Burlat cherries at two different degrees of ripeness. J Sci Food Agric. (2000) 80:1545–52. doi: 10.1002/1097-0010(200008)80:10<1545::AID-JSFA680>3.0.CO;2-X
9. Trajkovska Petkoska, A, Daniloski, D, D’Cunha, NM, Naumovski, N, and Broach, AT. Edible packaging: sustainable solutions and novel trends in food packaging. Food Res Int. (2021) 140:109981. doi: 10.1016/j.foodres.2020.109981
10. Nunes, C, Silva, M, Farinha, D, Sales, H, Pontes, R, and Nunes, J. Edible coatings and future trends in active food packaging–fruits’ and traditional sausages’ shelf life increasing. Foods. (2023) 12:3308. doi: 10.3390/foods12173308
11. Xie, Q, Liu, G, and Zhang, Y. Edible films/coatings containing bioactive ingredients with micro/nano encapsulation: a comprehensive review of their fabrications, formulas, multifunctionality and applications in food packaging. Crit Rev Food Sci Nutr. (2022) 12:1–38. doi: 10.1080/10408398.2022.2153794
12. Chavan, P, Lata, K, Kaur, T, Rezek Jambrak, A, Sharma, S, Roy, S, et al. Recent advances in the preservation of postharvest fruits using edible films and coatings: a comprehensive review. Food Chem. (2023) 418:135916. doi: 10.1016/j.foodchem.2023.135916
13. Maringgal, B, Hashim, N, Mohamed Amin Tawakkal, IS, and Muda Mohamed, MT. Recent advance in edible coating and its effect on fresh/fresh-cut fruits quality. Trends Food Sci Technol. (2020) 96:253–67. doi: 10.1016/j.tifs.2019.12.024
14. Romanazzi, G, and Moumni, M. Chitosan and other edible coatings to extend shelf life, manage postharvest decay, and reduce loss and waste of fresh fruits and vegetables. Curr Opin Biotechnol. (2022) 78:102834. doi: 10.1016/j.copbio.2022.102834
15. Perera, KY, Jaiswal, AK, and Jaiswal, S. Biopolymer-based sustainable food packaging materials: challenges, solutions, and applications. Foods. (2023) 12:2422. doi: 10.3390/foods12122422
16. Mohamed, SAA, El-Sakhawy, M, and El-Sakhawy, MA-M. Polysaccharides, protein and lipid-based natural edible films in food packaging: a review. Carbohydr Polym. (2020) 238:116178. doi: 10.1016/j.carbpol.2020.116178
17. Iversen, LJL, Rovina, K, Vonnie, JM, Matanjun, P, Erna, KH, ‘Aqilah, NMN, et al. The emergence of edible and food-application coatings for food packaging: a review. Molecules. (2022) 27:5604. doi: 10.3390/molecules27175604
18. Hassan, B, Chatha, SAS, Hussain, AI, Zia, KM, and Akhtar, N. Recent advances on polysaccharides, lipids and protein based edible films and coatings: a review. Int J Biol Macromol. (2018) 109:1095–107. doi: 10.1016/j.ijbiomac.2017.11.097
19. Mujtaba, M, Ali, Q, Yilmaz, BA, Seckin Kurubas, M, Ustun, H, Erkan, M, et al. Understanding the effects of chitosan, chia mucilage, levan based composite coatings on the shelf life of sweet cherry. Food Chem. (2023) 416:135816. doi: 10.1016/j.foodchem.2023.135816
20. Certel, M, Uslu, MK, and Ozdemir, F. Effects of sodium caseinate- and milk protein concentrate-based edible coatings on the postharvest quality of Bing cherries. J Sci Food Agric. (2004) 84:1229–34. doi: 10.1002/jsfa.1755
21. Tokatlı, K, and Demirdöven, A. Effects of chitosan edible film coatings on the physicochemical and microbiological qualities of sweet cherry (Prunus avium L.). Sci Hortic. (2020) 259:108656. doi: 10.1016/j.scienta.2019.108656
22. Yaseen, D, Sabbah, M, Al-Asmar, A, Altamimi, M, Famiglietti, M, Giosafatto, CVL, et al. Functionality of films from Nigella sativa defatted seed cake proteins plasticized with grape juice: use in wrapping sweet cherries. Coatings. (2021) 11:1383. doi: 10.3390/coatings11111383
23. Sajimon, A, Edakkadan, AS, Subhash, AJ, and Ramya, M. Incorporating oregano (Origanum vulgare L.) essential oil onto whey protein concentrate based edible film towards sustainable active packaging. J Food Sci Technol-Mysore. (2023) 60:2408–22. doi: 10.1007/s13197-023-05763-7
24. Zhang, Y-L, Cui, Q-L, Wang, Y, Shi, F, Fan, H, Zhang, Y-Q, et al. Effect of edible carboxymethyl chitosan-gelatin based coating on the quality and nutritional properties of different sweet cherry cultivars during postharvest storage. Coatings. (2021) 11:396. doi: 10.3390/coatings11040396
25. Aday, MS, and Caner, C. Understanding the effects of various edible coatings on the storability of fresh cherry. Packag Technol Sci. (2010) 23:441–56. doi: 10.1002/pts.910
26. Yuan, Y, Tian, H, Huang, R, Liu, H, Wu, H, Guo, G, et al. Fabrication and characterization of natural polyphenol and ZnO nanoparticles loaded protein-based biopolymer multifunction electrospun nanofiber films, and application in fruit preservation. Food Chem. (2023) 418:135851. doi: 10.1016/j.foodchem.2023.135851
27. Das, D, Mir, NA, Chandla, NK, and Singh, S. Combined effect of pH treatment and the extraction pH on the physicochemical, functional and rheological characteristics of amaranth (Amaranthus hypochondriacus) seed protein isolates. Food Chem. (2021) 353:129466. doi: 10.1016/j.foodchem.2021.129466
28. Montes-de-Oca-Ávalos, JM, Altamura, D, Herrera, ML, Huck-Iriart, C, Scattarella, F, Siliqi, D, et al. Physical and structural properties of whey protein concentrate – corn oil – TiO2 nanocomposite films for edible food-packaging. Food Packag Shelf Life. (2020) 26:100590. doi: 10.1016/j.fpsl.2020.100590
29. Zhang, Z, Huang, X, Li, S, Zhang, C, and Luo, K. Preparation and characterization of zein-sulfated cardamine hupingshanensis polysaccharide composite films. Food Sci Nutr. (2021) 9:6737–45. doi: 10.1002/fsn3.2625
30. Maryam Adilah, ZA, and Nur Hanani, ZA. Storage stability of soy protein isolate films incorporated with mango kernel extract at different temperature. Food Hydrocoll. (2019) 87:541–9. doi: 10.1016/j.foodhyd.2018.08.038
31. Moghadam, M, Salami, M, Mohammadian, M, Khodadadi, M, and Emam-Djomeh, Z. Development of antioxidant edible films based on mung bean protein enriched with pomegranate peel. Food Hydrocoll. (2020) 104:105735. doi: 10.1016/j.foodhyd.2020.105735
32. Zhang, Y, Jing, X, Chen, Z, and Wang, X. Purification and identification of antioxidant peptides from millet gliadin treated with high hydrostatic pressure. LWT. (2022) 164:113654. doi: 10.1016/j.lwt.2022.113654
33. Basiak, E, Geyer, M, Debeaufort, F, Lenart, A, and Linke, M. Relevance of interactions between starch-based coatings and plum fruit surfaces: a physical-chemical analysis. Int J Mol Sci. (2019) 20:2220. doi: 10.3390/ijms20092220
34. Colussi, R, Ferreira da Silva, WM, Biduski, B, Mello El Halal, SL, da Rosa Zavareze, E, and Guerra Dias, AR. Postharvest quality and antioxidant activity extension of strawberry fruit using allyl isothiocyanate encapsulated by electrospun zein ultrafine fibers. LWT. (2021) 143:111087. doi: 10.1016/j.lwt.2021.111087
35. Gharibzahedi, SMT, Ahmadigol, A, Khubber, S, and Altintas, Z. Whey protein isolate/jujube polysaccharide-based edible nanocomposite films reinforced with starch nanocrystals for the shelf-life extension of banana: optimization and characterization. Int J Biol Macromol. (2022) 222:1063–77. doi: 10.1016/j.ijbiomac.2022.09.232
36. Muley, AB, and Singhal, RS. Extension of postharvest shelf life of strawberries (Fragaria ananassa) using a coating of chitosan-whey protein isolate conjugate. Food Chem. (2020) 329:127213. doi: 10.1016/j.foodchem.2020.127213
37. Bishnoi, S, Trifol, J, Moriana, R, and Mendes, AC. Adjustable polysaccharides-proteins films made of aqueous wheat proteins and alginate solutions. Food Chem. (2022) 391:133196. doi: 10.1016/j.foodchem.2022.133196
38. Sood, A, and Saini, CS. Red pomelo peel pectin based edible composite films: effect of pectin incorporation on mechanical, structural, morphological and thermal properties of composite films. Food Hydrocoll. (2022) 123:107135. doi: 10.1016/j.foodhyd.2021.107135
39. Samsi, MS, Kamari, A, Din, SM, and Lazar, G. Synthesis, characterization and application of gelatin–carboxymethyl cellulose blend films for preservation of cherry tomatoes and grapes. J Food Sci Technol. (2019) 56:3099–108. doi: 10.1007/s13197-019-03809-3
40. Zhang, W, and Rhim, J-W. Titanium dioxide (TiO2) for the manufacture of multifunctional active food packaging films. Food Packag Shelf Life. (2022) 31:100806. doi: 10.1016/j.fpsl.2021.100806
41. Garrido, T, Etxabide, A, Guerrero, P, and de la Caba, K. Characterization of agar/soy protein biocomposite films: effect of agar on the extruded pellets and compression moulded films. Carbohydr Polym. (2016) 151:408–16. doi: 10.1016/j.carbpol.2016.05.089
42. Kchaou, H, Jridi, M, Abdelhedi, O, Nasreddine, B, Karbowiak, T, Nasri, M, et al. Development and characterization of cuttlefish (Sepia officinalis) skin gelatin-protein isolate blend films. Int J Biol Macromol. (2017) 105:1491–500. doi: 10.1016/j.ijbiomac.2017.06.056
43. Chen, W, Ding, J, Yan, X, Yan, W, He, M, and Yin, G. Plasticization of cottonseed protein/polyvinyl alcohol blend films. Polymers. (2019) 11:2096. doi: 10.3390/polym11122096
44. Acquah, C, Zhang, Y, Dubé, MA, and Udenigwe, CC. Formation and characterization of protein-based films from yellow pea (Pisum sativum) protein isolate and concentrate for edible applications. Curr Res Food Sci. (2020) 2:61–9. doi: 10.1016/j.crfs.2019.11.008
45. Gagliarini, N, Figoli, CB, Piermaria, J, Bosch, A, and Abraham, AG. Unraveling molecular interactions in whey protein-kefiran composite films to understand their physicochemical and mechanical properties. Food Biosci. (2022) 50:102012. doi: 10.1016/j.fbio.2022.102012
46. Lin, L, Mei, C, Shi, C, Li, C, Abdel-Samie, MA, and Cui, H. Preparation and characterization of gelatin active packaging film loaded with eugenol nanoparticles and its application in chicken preservation. Food Biosci. (2023) 53:102778. doi: 10.1016/j.fbio.2023.102778
47. Hu, Y, Shi, L, Ren, Z, Hao, G, Chen, J, and Weng, W. Characterization of emulsion films prepared from soy protein isolate at different preheating temperatures. J Food Eng. (2021) 309:110697. doi: 10.1016/j.jfoodeng.2021.110697
48. Zhang, C, Wang, Z, Li, Y, Yang, Y, Ju, X, and He, R. The preparation and physiochemical characterization of rapeseed protein hydrolysate-chitosan composite films. Food Chem. (2019) 272:694–701. doi: 10.1016/j.foodchem.2018.08.097
49. Mir, NA, Riar, CS, and Singh, S. Effect of film forming solution pH on antibacterial, antioxidant and structural characteristics of edible films from modified quinoa protein. Food Hydrocoll. (2023) 135:108190. doi: 10.1016/j.foodhyd.2022.108190
50. Sarıcaoğlu, FT, and Turhan, S. Physical, chemical, thermal and microstructural characterization of edible films from mechanically deboned chicken meat proteins. J Polym Environ. (2019) 27:1071–85. doi: 10.1007/s10924-019-01410-5
51. Yuan, G, Jia, Y, Pan, Y, Li, W, Wang, C, Xu, L, et al. Preparation and characterization of shrimp shell waste protein-based films modified with oolong tea, corn silk and black soybean seed coat extracts. Polym Test. (2020) 81:106235. doi: 10.1016/j.polymertesting.2019.106235
52. He, M, Zhang, B, Dou, Y, Yin, G, and Cui, Y. Blend modification of feather keratin-based films using sodium alginate. J Appl Polym Sci. (2017) 134:44680. doi: 10.1002/app.44680
53. Kumari, N, Bangar, SP, Petrů, M, Ilyas, RA, Singh, A, and Kumar, P. Development and characterization of fenugreek protein-based edible film. Foods. (2021) 10:1976. doi: 10.3390/foods10091976
54. Yu, Y-P, Lai, S-J, Chang, C-R, Chen, W-C, Wu, S-H, and Lu, C-P. Peptidomic analysis of low molecular weight antioxidative peptides prepared by lotus (Nelumbo nucifera Gaertn.) seed protein hydrolysates. LWT-Food Sci Technol. (2021) 144:111138. doi: 10.1016/j.lwt.2021.111138
55. Lu, X, Zhang, L, Sun, Q, Song, G, and Huang, J. Extraction, identification and structure-activity relationship of antioxidant peptides from sesame (Sesamum indicum L.) protein hydrolysate. Food Res Int. (2019) 116:707–16. doi: 10.1016/j.foodres.2018.09.001
56. Xue, H-Y, Zhao, Y, Liu, Z-H, Wang, X-W, Zhang, J-W, Peng, X, et al. Recovery of yam soluble protein from yam starch processing wastewater. Sci Rep. (2020) 10:5384. doi: 10.1038/s41598-020-62372-6
57. Aguirre-Joya, JA, Ventura-Sobrevilla, J, Martínez-Vazquez, G, Ruelas-Chacón, X, Rojas, R, Rodríguez-Herrera, R, et al. Effects of a natural bioactive coating on the quality and shelf life prolongation at different storage conditions of avocado (Persea americana mill.) cv. Hass Food Packag Shelf Life. (2017) 14:102–7. doi: 10.1016/j.fpsl.2017.09.003
58. Xiao, Y, Liu, Y, Kang, S, and Xu, H. Insight into the formation mechanism of soy protein isolate films improved by cellulose nanocrystals. Food Chem. (2021) 359:129971. doi: 10.1016/j.foodchem.2021.129971
59. Costa, BP, Carpiné, D, Ikeda, M, Pazzini, IAE, da Silva Bambirra Alves, FE, de Melo, AM, et al. Bioactive coatings from non-conventional loquat (Eriobotrya japonica Lindl.) seed starch to extend strawberries shelf-life: an antioxidant packaging. Prog Org Coat. (2023) 175:107320. doi: 10.1016/j.porgcoat.2022.107320
60. Nandane, AS, Dave, RK, and Rao, TVR. Optimization of edible coating formulations for improving postharvest quality and shelf life of pear fruit using response surface methodology. J Food Sci Technol. (2017) 54:1–8. doi: 10.1007/s13197-016-2359-9
61. Soradech, S, Nunthanid, J, Limmatvapirat, S, and Luangtana-anan, M. Utilization of shellac and gelatin composite film for coating to extend the shelf life of banana. Food Control. (2017) 73:1310–7. doi: 10.1016/j.foodcont.2016.10.059
62. Mei, S, Fu, B, Su, X, Chen, H, Lin, H, Zheng, Z, et al. Developing silk sericin-based and carbon dots reinforced bio-nanocomposite films and potential application to litchi fruit. LWT. (2022) 164:113630. doi: 10.1016/j.lwt.2022.113630
Keywords: Idesia polycarpa Maxim, protein film, antioxidant activity, sweet cherry, shelf life
Citation: Yang W, Zhang Z, Chen Y and Luo K (2023) Evaluation of the use of Idesia polycarpa Maxim protein coating to extend the shelf life of European sweet cherries. Front. Nutr. 10:1283086. doi: 10.3389/fnut.2023.1283086
Received: 29 August 2023; Accepted: 31 October 2023;
Published: 17 November 2023.
Edited by:
Khalid Gul, University of Leeds, United KingdomReviewed by:
Wissam Zam, Wadi International University, SyriaCopyright © 2023 Yang, Zhang, Chen and Luo. This is an open-access article distributed under the terms of the Creative Commons Attribution License (CC BY). The use, distribution or reproduction in other forums is permitted, provided the original author(s) and the copyright owner(s) are credited and that the original publication in this journal is cited, in accordance with accepted academic practice. No use, distribution or reproduction is permitted which does not comply with these terms.
*Correspondence: Kai Luo, bHVva2FpXzc5QDE2My5jb20=
†These authors share first authorship
Disclaimer: All claims expressed in this article are solely those of the authors and do not necessarily represent those of their affiliated organizations, or those of the publisher, the editors and the reviewers. Any product that may be evaluated in this article or claim that may be made by its manufacturer is not guaranteed or endorsed by the publisher.
Research integrity at Frontiers
Learn more about the work of our research integrity team to safeguard the quality of each article we publish.